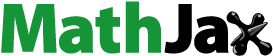
ABSTRACT
These days, green composites are getting a lot of attention for their biodegradability, sustainability, and affordability. This study applies polylactic acid as the matrix and untreated and surface-treated kenaf fibers as reinforcement to fabricate kenaf-reinforced PLA-based composites. Kenaf fibers are exposed to three different chemical treatments (sodium hydroxide, potassium permanganate, and potassium dichromate) and investigate the effect of surface modifications on the mechanical properties of kenaf/PLA composites. The surface modified fibers were characterized by FTIR Spectroscopy. The consequences of processing variables such as fiber volume percent ranging from 25% to 50% and molding temperature range of 160°C to 180°C were also examined on the mechanical characteristics of developed composites and are validated using SEM analysis. Surface modifications result in improved tensile and flexural characteristics of developed treated kenaf/PLA composites. Alkali treated woven kenaf fiber composites with greater matrix adhesion have optimum tensile and flexural strength of 72.81 MPa and 94.45 MPa respectively. The present study revealed that composites (fabricated at 170°C molding temperature) have maximal tensile and flexural strength at 35% fiber volume fraction.
摘要
如今,绿色复合材料因其生物降解性、可持续性和可负担性而备受关注. 本研究以聚乳酸为基体,未经处理和表面处理的红麻纤维为增强材料,制备了红麻增强聚乳酸基复合材料. 将红麻纤维暴露于三种不同的化学处理(氢氧化钠、高锰酸钾和重铬酸钾)中,研究表面改性对红麻/PLA复合材料力学性能的影响. 用红外光谱对表面改性纤维进行了表征. 还研究了加工变量(如纤维体积百分比在25%至50%范围内和成型温度在160°C至180°C范围内)对所开发复合材料的机械特性的影响,并使用SEM分析进行了验证. 表面改性改善了所开发的经处理的红麻/PLA复合材料的拉伸和弯曲特性. 基体附着力较大的碱处理红麻编织纤维复合材料的最佳拉伸强度和弯曲强度分别为72.81MPa和94.45MPa. 本研究表明,复合材料(在170°C成型温度下制造)在纤维体积分数为35%时具有最大的拉伸和弯曲强度.
Introduction
Composites are used worldwide and have become an important material which is used to develop different components that are used in our daily lives such as in spaceships, rubber tires, and bricks. The stronger, stiffer, and lighter composite material, which may be utilized for a variety of applications including transportation, aerospace, medical applications, etc., has always been in demand since 1950 (Bajpai, Singh, and Madaan Citation2014; John, Virk, and Wayne Citation2013). Due to the high strength-to-weight ratio and similar mechanical properties of traditional metals and composite materials, there is considerable interest in replacing traditional metals with these alternatives. In general, composite material constituents have physical and chemical characteristics which are still present while yet offering a mix of attributes that none of the constituents alone could have. However, managing synthetic composites presents some significant difficulties due to their reusability (Bajpai, Singh, and Madaan Citation2014). Hence, there is a need to develop the sustainable material i.e., Green Composites. Green composites are those whose constituents, either one or both, came from a natural resource (John, Virk, and Wayne Citation2013). According to the type of constituents they are formed of, completely biodegradable composites and partially biodegradable green composites. Completely biodegradable composites consist of two or more constituents which are derived from the natural resource. Partially Biodegradable composites consists of only one constituent is derived from the natural resource. There has been a lot of interest generated in the area of green composites in order to solve important issues including the difficulty of petroleum-based composites to biodegrade, global warming, the depletion of fossil resources, etc. Reinforcement in the composite material is one of the major constituents used as load bearing member. In Green composites, natural fibers are the generally used as reinforcement. The primary three categories of natural fibers are plant, animal, and mineral fibers (Mukherjee and Kao Citation2011). Many studies have been conducted using biodegradable polymers and natural fibers. Unidirectional fiber-reinforced composites show tensile and flexural strengths of 223 MPa and 254 MPa, respectively, according to S. ochi’s (Ochi Citation2008) development of the kenaf/PLA composites and investigation of its mechanical characteristics. P. Penhju et al (Pengju et al. Citation2007). developed the kenaf fiber in kenaf/PLA composites and investigated the variation of fiber content on the crystallization behavior and mechanical characteristics. Results showed that adding 30% more kenaf fiber to the composite reinforced it, improving its mechanical characteristics by 30%. With the aid of the melt blending technique, Y. F. Shih and C.C. Huang (Shih and Huang Citation2011) produced the banana fiber-reinforced green composites and looked into their thermal and mechanical characteristics. The tensile and flexural strengths of the composites rose at 40 phr, reaching 78.6 MPa and 65.4 MPa, respectively. The best compression molding parameters for attaining the best mechanical characteristics of bamboo/PLA composites were found using the Taguchi technique. The largest pressure (1.05 MPa) and shortest duration are shown to be the keys to achieving the best tensile and flexural strength (3 minutes) (Rawi, Jayaraman, and Bhattacharyya Citation2013). According to research by W. Smitthi pong et al (Smitthipong, Tanthatherdtam, and Chollakup Citation2013), the hybridization of TPS and pineapple leaf fiber with PLA matrix exhibits improved mechanical properties and then TPS-reinforced PLA-based composites. P.K. et al (Bajpai et al. Citation2013). constructed nettle fiber-reinforced polypropylene composites and investigated the effects of sunlight, cold temperatures, diesel oil, and river water on their tensile strength. The findings demonstrated that all exposed circumstances had an impact on tensile strength, with sunlight and river water settings lowering maximum tensile strength. The maximum tensile and flexural strengths were found to be at 100°C and were 32.5 MPa and 41.9 MPa, respectively. Jaiinder et al. (Jaiinder, Singh, and Dhawan Citation2018). examined the impact of molding temperature range on the mechanical parameters of jute-reinforced epoxy-based composites. By using a variety of fiber surface treatment techniques, researchers have tried to enhance the mechanical characteristics of green composites (Bulut and Aksit Citation2013; Jaafar et al. Citation2012; Jaiinder et al. Citation2017; Jaiinder, Singh, and Dhawan Citation2017, Citation2019a). Prior studies have been reported (Saba, Paridah, and Jawaid Citation2015) with the contributions of several scientists to the study of kenaf fiber-reinforced polymer composites were emphasized (Jaiinder, Singh, and Dhawan Citation2019b). Investigations into the possessions of molding temperature and fiber volume fraction on the mechanical characteristics of Jute/PLA composites revealed that 30% fiber fraction by volume and 160°C molding temperature produced the greatest mechanical characteristics. A Gomes (Gomes Citation2007) proposed the surface modification as the noval technique improving the tensile properties of green composites.
Hamidon et al. (Hamidon et al. Citation2019) reviewed the different surface treatments of kenaf fiber on its mechanical properties. The study revealed that all surface treatment methods have a positive impact in terms of enhancement of mechanical properties and fiber-matrix adhesion. F.S. Shahar et al. (Shahar et al. Citation2022) investigated the effect of reinforced kenaf particles ranging from 3%, 5%, and 7% by weight on fatigue and impact strength using 3D printing technology. It concludes that fatigue and impact strength increase with the increase in fiber content. Azlin et al. (Azlin et al. Citation2022) developed woven kenaf/polyester fiber reinforced PLA-based hybrid laminated composites using hot compression molding technique. Different reinforced stacking sequences are used to investigate their impact on mechanical properties. Maximum tensile strength of 103 MPa with 17.88% elongation and 84 kJ/m2 was observed with the S4 type of sequence sample. J. Fajrin et al. (Fajrin, Akmaluddin, and Gapsari Citation2022) utilize the kenaf fiber waste as reinforcement for the development of polyester-based composites. The effect of fiber configuration on mechanical properties was investigated. Results revealed that uni-directional oriented kenaf fiber exhibits the highest tensile strength of 76.5 MPa and 151.3 MPa as flexural strength.
Nurazzi et al. (Nurazzi et al. Citation2020) investigated the impact of alkali treatment and hybridization on the thermal characteristics of composites reinforced with glass fibers and sugar palm yarn. The thermal properties of the hybrid composites were assessed using DMA and TGA. Results concealed that alkali-treated sugar palm fiber reinforcement with the highest glass fiber content shows the highest storage modulus and lowest damping factor. The study also reveals that alkali treatment and hybridization have a positive impact on the thermal properties of polymer-reinforced composites (Lu et al. Citation2022). Yusoff et al. (Yusoff, Takagi, and Nakagaito Citation2016) developed the kenaf, bamboo, and coir fibers reinforced PLA-based composites and investigated their effect on their mechanical properties. It is concluded that Kenaf-coir/PLA-based composites exhibit 70% higher flexural strength in comparison with bamboo-coir/PLA and Kenaf-bamboo-coir/PLA composites. M.M. Owen et al. (Owen, Achukwu, Shuib, et al. Citation2023) developed the epoxy resin coated kenaf fiber reinforced PET composites for high temperature engineering applications. M.M. Owen et al. (Owen, Achukwu, Hazizan, et al. Citation2023) developed the recycled polyethylene terephthalate composites reinforced with the surface treated kenaf fiber. Results revealed that the recycled polyethylene terephthalate composites possessed similar thermal properties as of its virgin counterparts. Lot of researchers did the research on the surface modifications of the kenaf fibers and found a positive improvement in the mechanical properties (Owen et al. Citation2018a; Owen et al. Citation2018b; Achukwu et al. Citation2023; Owen, Achukwu, Arukalam, et al. Citation2022).
Moreover, the interest in biodegradable, sustainable, and affordable green composites has led to the research of natural fibers as polymer matrix reinforcements. Keenaf-reinforced PLA composites are developed using polylactic acid (PLA) as the matrix and kenaf fibers as reinforcement. In particular, the study examines how sodium hydroxide, potassium permanganate, and potassium dichromate surface treatments affect composite mechanical characteristics. The study investigates how to develop kenaf/PLA composites with enhanced mechanical characteristics. Additionally, the necessity for sustainable materials is emphasized by the substantial database of research on green composites. Since they might solve petroleum-based composites, global warming, as well as natural depletion of resources, green composites composed of natural fibers including kenaf have been gaining interest. To reinforce biodegradable polymers like PLA, natural fibers like kenaf have been extensively investigated. Sustainability merits, and potential substitution for composites motivate natural fiber selection. Reusability issues with synthetic composites are the research problem. The review covers works on natural fibers and biodegradable polymers such kenaf/PLA composites. Fiber surface treatments are important in fiber-reinforced composite mechanical characteristics, according to studies. As demonstrated in prior studies, surface modifications strengthen green composite’s mechanical characteristics. Alkali treatment, potassium permanganate, and potassium dichromate can enhance green composite’s physicomechanical characteristics when applied to the surface. The study takes into account and broadens on previous studies on jute and flax-reinforced PLA composites (Bulut and Aksit Citation2013; Jaiinder et al. Citation2017; Jaiinder, Singh, and Dhawan Citation2019a, Citation2019b; Saba, Paridah, and Jawaid Citation2015).
Green composites’ mechanical characteristics have been studied, however kenaf-reinforced PLA composites’ surface modifications and processing parameters during manufacturing required meticulously investigation and thoroughly researched (Azlin et al. Citation2022; Gomes Citation2007; Hamidon et al. Citation2019; Shahar et al. Citation2022). Alkali, potassium permanganate, and potassium dichromate treatments have modified fiber surfaces in investigations. Composite mechanical characteristics have been assessed to determine the efficacy of various treatments. Woven kenaf fiber composites treated with alkali exhibit superior matrix adherence bonding-strength, and optimal tensile and flexural strength.
Research on jute and flax-reinforced PLA composites preceded this study. With PLA as the matrix, this study uses woven untreated and surface-treated kenaf fibers as reinforcements. How the chemical treatments of surfaces (sodium hydroxide, potassium permanganate, and potassium dichromate), fiber volume fractions (ranging from 25% to 50%), and molding temperatures (ranging from 160°C to 180°C) have an impact on kenaf/PLA composite’s mechanical characteristics, interface adherence bond-strength, chemical, and microstructural-morphology analysis based-characterizations have been emerged as the subject of investigation.
In terms of scientific-novelty is concerned, the research ventures into an innovative analysis into the modification of the surfaces of kenaf fibers using three distinct chemical treatments: potassium dichromate, sodium hydroxide, and potassium permanganate. Moreover, this study makes a valuable contribution to the field by treating kenaf fibers with three distinct chemical treatments, whereas previous research has primarily examined surface modifications of natural fibers. Initial trials as well as literature validation provide support for the treatment selection process. Additionally, the research utilizes water-absorption, physicomechanical, interface-adherence, bond-strength and FTIR examinations which certainly is a novel approach to analyze untreated and surface-treated kenaf fibers, thereby offering valuable insights into chemical transformations. Utilisation of FTIR analysis to comprehend the chemical interactions among fibers as well as chemical treatments on surfaces. An exhaustive comprehension of structure-related modifications is the outcome of an in-depth examination regarding chemical bonds as well as functional groups.
Additionally, the investigation employs compression molding to produce kenaf/PLA composites, taking into account variables including molding temperature as well as fiber volume percentage. Although the fabrication of composites from natural fibers and biodegradable polymers is a prevalent practice, this research introduces novelty by methodically manipulating the volume percentage of the fibers (from 25% to 50%) and the molding temperature (from 160°C to 180°C). The investigation reveals the most favorable optimal circumstances for attaining maximum tensile as well as flexural strength. Furthermore, the research endeavors to thoroughly examine the mechanical characteristics by conducting an extensive array of testing, such as tensile, flexural, hardness, and water absorption. As the surface treatment influences the mechanical properties, and this research methodically assesses the effects of various surface treatments on the flexural and tensile characteristics of composites composed of kenaf and PLA.
The research highlights the significance of various surface treatments in relation to mechanical properties, with a specific emphasis on the substantial enhancements in tensile and flexural strength that are achieved through alkali treatment. An innovative aspect regarding surface modifications in green composites is brought to light by the order or arrangement of the sequence of chemical surface treatments in accordance with their corresponding efficacy ((Azlin et al. Citation2022; Gomes Citation2007; Hamidon et al. Citation2019; Jaiinder, Singh, and Dhawan Citation2019b; Shahar et al. Citation2022)).
In terms of scientific-innovation is concerned, the valuable insights are gained from the methodical comparison of surface treatments (potassium permanganate, potassium dichromate, and alkali treatment) and their effects on mechanical strengths. In terms of increasing tensile and flexural strength, alkali treatment is specifically recognized as the most efficacious method. The research indicates that the kenaf/PLA composites that were developed have the potential to be utilized in various applications, including the replacement of PVC wall panels.
Finally, the current research endeavor is an expansion of a previous investigation into Jute and Flax-reinforced PLA composites (Jaiinder, Singh, and Dhawan Citation2019b). In this study, woven untreated and surface-treated woven kenaf fiber were fabricated as reinforcement while PLA was used as the matrix material. The impact of surface treatments (Alkali treatment, potassium permanganate, and potassium dichromate), molding temperatures between 160°C and 180°C and fiber volume fractions between 25% and 50% on the mechanical characteristics of kenaf/PLA composites were investigated using compression molding. Untreated and surface-treated kenaf fibers were examined under FTIR spectroscopy to examine any structural change due to chemical interactions. Mechanical characterization and water absorption tests were performed in accordance with ASTM guidelines. Tensile-cracked specimens were examined using scanning electron microscopy (SEM) to examine the interfacial adhesion between the fiber and matrix.
Experimentation
Materials
A woven kenaf fiber that was procured from Chander Prakash Pvt Ltd in India was utilized as reinforcement. From Natur Tec India Pvt Ltd, Tamil Nadu, India, granulated polylactic acid (PLA) of grade 3025D was acquired. Various properties of polylactic acid and woven kenaf fiber are mentioned in and (Jaiinder, Singh, and Dhawan Citation2019b). From scientific chemicals, in India, many chemicals were bought, including potassium permanganate KMnO₄, potassium dichromate K2Cr2O7, sodium hydroxide NaOH, and acetic acid CH3COOH.
Table 1. Properties and its magnitude for polylactic acid (Jaiinder, Singh, and Dhawan Citation2019b).
Table 2. Properties and its magnitude for kenaf fiber (Jaiinder, Singh, and Dhawan Citation2019b).
Surface modifications of fibers
Before the use of woven kenaf fiber for the development of kenaf/PLA composites, they were exposed to the surface treatment as alkali treatment, Potassium dichromate, and Potassium permanganate with 5%, 0.02%, and 0.02% concentration, respectively. The selection of optimum concentrations of all the three chemicals were selected on the basis of initial trials (Jaiinder, Singh, and Dhawan Citation2019a) and also found that the selected optimum ranges are highly corroborated with the literature also (Bulut and Aksit Citation2013).
Alkali Treatment: Alkaline treatment is most commonly reported when vegetable fibers are changed on the surface, also known as mercerization. This treatment involves immersing the fiber for 24 hours in a solution of sodium hydroxide (NaOH), and then wash the fiber until it reaches equilibrium. Kenaf fibers were immersed in 5% concentrated NaOH solution in this investigation as shown in . The liquor ratio remained unchanged at 15:1. The fibers were treated with alkali, removed with an acetic acid solution, and then left to dry in the sun for 24 hours to ensure the homogenous drying of treated fiber. The chemical interactions between the alkali-treated fibers and the alkali solution were subsequently examined using FTIR spectroscopy.
Potassium Permanganate Treatment: This treatment produces highly reactive permanganate (Mn3+) ions that react with the groups of hydroxyl cellulose and form cellulose – manganese to induce graft co-polymerization. By using this method, the interface’s chemical interlocking is improved, which enhances the matrix’s adherence. In this investigation, woven kenaf fibers were soaked for 2 min at room temperature in a 0.02% concentrated potassium permanganate solution as shown in , then washed with tap water and finally held for drying purposes under the sunlight.
Potassium Dichromate Treatment: In this investigation, Kenaf fibers were immersed in 0.02% concentrated potassium dichromate solution for 2 minutes at room temperature. shows the dipping of kenaf fibers in potassium dichromate solution, pounded with tap water and eventually kept to dry under the sunlight.
FTIR analysis of kenaf fibers
A Fourier transform infrared spectrometer was used to obtain FTIR spectra of the untreated and surface-treated kenaf fibers. ASTM E168 standard is used for the FTIR analysis of Kenaf fiber. The research was conceded to analyze the chemical interactions between fibers. With a resolution of 1 cm−1 each spectrum was measured within the range of 500–4000 cm−1.
Processing of kenaf/PLA composites
Compression molding was utilized to develop kenaf/PLA composites, with a woven kenaf fiber serving as reinforcement and polylactic acid (PLA) serving as the matrix material. For the development of these composites, the experimental apparatus includes a Teflon sheet, a compression molding machine, heating rods, thermocouples, control panels, and a metallic die. The bottom die and upper die of a metallic die were developed and constructed in two pieces. It was made to produce rectangular laminates with dimensions of 320 mm by 120 mm and a thickness of 4 mm. Teflon sheets were employed as a releasing agent to keep the PLA from adhering to the metal. The metallic dies were heated by heating rods, which further melted the PLA at the desired temperature. J-type thermocouples were used to control the temperature of both dies. The temperature is recorded by these thermocouples, which also send feedback to the control panel so that it may be changed as necessary. To remove the moisture from it, woven kenaf fiber and a certain quantity of PLA were both put in the oven for drying at the temperature of 80°C. Woven kenaf fiber and PLA were weighed using a weighing machine after 4 hours. An adequate grade of PLA was poured into the rectangular cavity of a Teflon sheet, which was then covered with a first layer of woven kenaf fiber and a second layer of PLA to create a sandwich construction.
The same process was used to create a sandwich construction that included six layers of polylactic acid and five layers of woven kenaf fibers. The sandwich construction was eventually covered with a Teflon sheet, and the upper die was utilized to seal the entire die before it was inserted into the compression molding machine. At a steady molding temperature, 4 MPa of pressure was initially applied for 4 minutes, and then 6 MPa for an additional 11 minutes. The formation of composites is given a total molding duration of 15 minutes. After being developed, composites were air cooled to 80°C while being loaded. Future, composites were held away from the die for additional testing. The method for creating kenaf/PLA composites is depicted in .
Kenaf/PLA composites were fabricated using a range of fiber volume fractions (25%, 30%, 35%, 40%, 45%, and 50%) and molding temperatures (160°C, 170°C, and 180°C). The melting point of neat PLA was determined by a differential scanning calorimetry (DSC) test to be 160°C as evident by the (Jaiinder, Singh, and Dhawan Citation2019b). It was discovered from the early tests that fibers burn out at a temperature of 190°C as reported by from Jaiinder, Singh, and Dhawan (Citation2019b).
In light of these preliminary testing results, it has been agreed to maintain the 160°C to 180°C molding temperature range. Equation 1 has been used to compute the volume of fiber reinforcement in the composites (Gomes Citation2007).
Where Vf is fiber volume fraction, Wf is the weight of the fiber in composite, W and V is abbreviated for the weight and volume, respectively, of the produced composite., and pm is the density of matrix material. For mechanical characterization, kenaf/PLA laminates were further cut in accordance to ASTM standards. The specific ASTM standards used are mentioned in the section 2.5. Surface-treated kenaf/PLA based composites with 35% fiber volume fraction were developed at different curing temperatures ranging of 160°C, 170°C, and 180°C. Before development, woven kenaf fibers were chemically treated with sodium hydroxide, potassium permanganate, and potassium dichromate and used as reinforcements. The same procedure was adopted to develop all three types of treated composites as stated above.
Mechanical characterization
Tensile test
According to ASTM D3039, tensile testing was carried out (ASTM Standard D 3039/D 3039M–07 Citation2008) in order to comprehend how kenaf/PLA will behave for engineering applications under axial force. The test specimens were fabricated with dimensions 250 mm × 25 mm × 4 mm. The various test was carried out using a universal testing apparatus (UTM) (Tinius Olsen, USA). The load-cell capacity of the UTM for the tensile tests is 1000 Kg. A strain rate of 2 mm/min was used for the test. An average of 5 specimens was taken as the final reading.
Flexural test
ASTM standard D790–02 was followed for conducting cross-breaking tests (ASTM Standard D 790–02 Citation2002). Two parallel V-shaped supports kept the specimen in place within the apparatus. The sample was 120 mm by 15 mm by 4 mm in size. The supports were always 16 times the thickness of the test specimen apart. Strain rate of 2 mm/min was used for the test and is conducted on the same UTM machine as used for tensile test.
Hardness test
) illustrates how the hardness of kenaf/PLA composites was evaluated using a Barcol hardness tester. The Barcol hardness number indicates the quality of well-cured composites. According to ASTM standard D2583 (ASTM Standard D 2583 Citation1995) and guidelines kenaf/PLA composites were conducted with a hardness test.
Water absorption test
As per ASTM D570–98 (ASTM Standard D 570 Citation1999), a water absorption analysis was conducted. The laminate was sliced into a 38 mm square test specimen. The specimen was weighed in air (W1) before spending 24 hours submerged in distilled water. The specimen was carefully wiped after being removed from the water and weighed within two minutes of being removed from the water. The designation of this weight is W2. According to equation 2, the amount of water absorption was noted.
Surface morphology
To study the interface between the matrix material (PLA) and reinforcement (Kenaf fiber), tensile fractured surfaces underwent scanning electron microscopy (SEM) analysis. The investigation evaluated the impact of processing factors (fiber volume fraction and molding temperature) on the interfacial bonding between the two materials using samples of kenaf/PLA composites with the lowest and greatest tensile strengths (Ashok et al. Citation2021; Chandel et al. Citation2021; Jha et al. Citation2021, Citation2022; Kumar et al. Citation2022; J. I. P. Singh et al. Citation2021; J. P. Singh et al. Citation2020; Yeswanth et al. Citation2022).
Results and discussions
Characterization of surface modified kenaf fibers
Surface treatment of kenaf fibers with different chemicals such Sodium hydroxide, potassium dichromate (PD), and potassium permanganate (PP) are analyzed using FTIR spectroscopy. FTIR spectrum is used to analyze the functional groups by the effect of chemical treatments on the kenaf fiber ). As unveiled from the , the absorption band located between 3300 and 3340 cm−1 is caused by the hydrogen-bonded O–H stretching the cellulose vibration (Lu et al. Citation2022), which is generally found in all naturally occurring fibers. This band was measured at 3306 cm−1 for untreated fibers, and it was discovered that the intensity of the band varied slightly on treated fibers i.e 3365, 3402 and 3515 cm−1 for alkali, Potassium Dichromate and Potassium permanganate treatment respectively. This demonstrates the decrease in hydrophilic behavior due to a reduction in hydrogen bonding in cellulosic hydroxyl groups (Owen et al. Citation2018; Owen, Achukwu, Rahman, et al. Citation2023; Owen, Achukwu, Shuib, et al. Citation2023), and the free -OH presence in carboxylic groups does not participate in the formation of hydrogen bonding through the alkali treatment. The breakdown of ester or ether bonding brought about by the NaOH treatment causes hydrolysis, which extracts lignin or hemicelluloses from fibers and releases a free OH group. The untreated fiber exhibited a distinct band peak located at 1732 cm−1 (Lu et al. Citation2022). reported that this band showed C=O stretching in the ester linkages of lignin’s carboxylic groups. Potassium Dichromate (PD) and Potassium Permanganate (PP) treated fiber also exhibits a distinct band peak at 1740 and 1740 cm−1 respectively. From FTIR spectra, it is observed that this band was not present in alkali treated kenaf fiber which might be due to the dissolution of hemicellulose in the alkali solution. The peak at 1740 cm-1 associated with the C=O stretching vibration of hemi-celluloses in the alkali-treated kenaf fiber vanished as a result of structural change, as seen in . Hemi-cellulose removal strengthens the link between the fiber matrix and increases the mechanical properties of the composites (Owen, Achukwu, Akil, et al. Citation2022; Owen, Achukwu, Romli, et al. Citation2023). In addition, the strengthened tensile characteristics ensuing from the alkali treatment are attributed to the elimination of the hemicellulose from the kenaf-fiber, as evidenced by FTIR spectroscopy.
Table 3. FTIR transmittance readings for untreated and surface-treated kenaf fibers.
Mechanical characterization of developed composites
Impact of fiber loadings, processing temperature, and surface treatment on the tensile properties of kenaf/PLA composites
The values of tensile strength and Young’s modulus of kenaf-reinforced PLA composites are shown in and . The results depicted the kenaf/PLA composite’s behavior when the temperature and fiber volume fraction are changed.
Kenaf/PLA test results recorded the maximum tensile strength of 65.158 MPa at a fiber volume fraction of 35% at a molding temperature of 170°C, as shown in . The test also shows that adding woven kenaf fibers (less than 45% content) has increased the tensile strength of kenaf/PLA composites in comparison to clean PLA. It is anticipated that the tensile strength of kenaf/PLA composites will increase with kenaf fiber integration up to 35% of the volume fraction of the fiber and subsequently decline with additional increases in fiber loading up to 50% (Owen, Achukwu, Zafir Romli, et al. Citation2023).
Young’s modulus of kenaf-reinforced PLA composites is affected by fiber loading and molding temperature, as depicted in . Young’s modulus of kenaf-reinforced PLA composites has been seen to grow as the proportion of fiber increases up to 35% before declining as fiber loading climbs further. For kenaf/PLA composites, a maximum Elastic modulus of 3.29 GPa is discovered at a volume percentage of 35% fibers produced at a molding temperature of 170°C. The results obtained are highly corroborated with the similar literature study by M.M. Owen (Owen, Achukwu, Shuib, et al. Citation2023) which claimed that surface treated kenaf fibers improve the thermal stability and the interfacial adhesion between the coated kenaf fiber and the engineering plastic matrix.
The SEM pictures of the broken tensile specimen of the kenaf/PLA composite, which comprises 35% fiber loading by volume and was cured at 160 degrees Celsius, 170 degrees Celsius, and 180 degrees Celsius molding temperatures, are exposed in , respectively. Selection of the tensile specimens for SEM analysis was done on the basis of composites with maximum and minimum tensile strength. Hence, the composites with 35% fibre loading and 50% fiber loading were selected for analysis purposes on different molding temperatures.
Figure 7. SEM images of tensile failed specimens of kenaf reinforced PLA-based bio-composites for (a) 160°C (b) 170°C and (c) 180°C at 500× magnifications respectively.
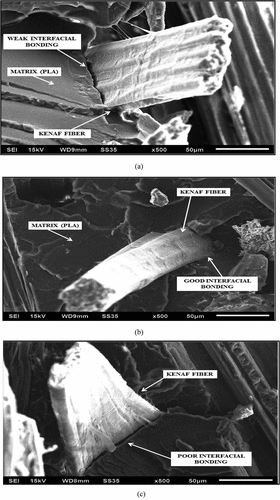
After analyzing the SEM images for kenaf/PLA composites, it has been inferred that for composites developed at molding temperatures of 160°C and 180°C, the poor interfacial-adherence and bond-strength has been apparent between the PLA-matrix and kenaf fiber. A lower load-bearing capability results from weak fiber-matrix interfacial bonding. Weak fiber-matrix interfacial bonding at 180°C can be due to higher temperature thermal degradation of the material.
Although poorer wettability of kenaf fiber with PLA matrix at 160°C may be the source of weak interfacial bonding, this indicates that the matrix is more viscous at this temperature during molding. Kenaf/PLA composites produced at 170 degrees Celsius with a 35% fiber volume percentage showed robust interfacial adhesion between the reinforcement and matrix material (Owen Citation2022). SEM pictures of tensile-broken samples of developed composites generated at 170°C with 35% and 50% fiber volume fractions, respectively, are shown in , respectively. Additionally, it has been determined that Kenaf/PLA composites with 50% fiber loading by volume generated at 170°C molding temperature show scrawny interfacial bonding between the matrix and fiber. In composites with 50% fiber volume percentage, complete fiber pull-out was seen, which may be due to the fibers’ poorer wettability with the matrix material and weak interfacial adhesion.
Figure 8. Tensile fractured Kenaf/PLA composite specimens as depicted in a SEM picture that was obtained at 170°C with a) 35% fiber volume percent, b) 50% fiber volume percent at 750× magnification respectively.
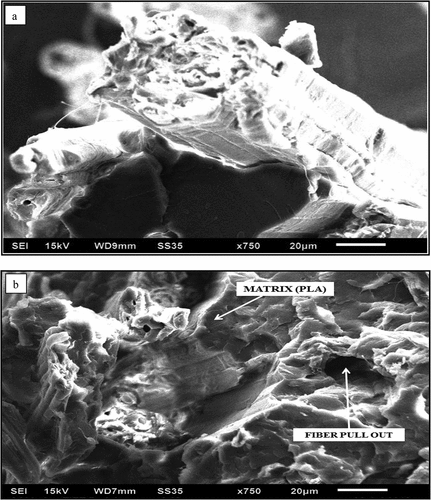
mentioned the outcome of different surface treatments on the tensile strength of the composites Kenaf/PLA. After all, three types of surface treatments, the tensile strength of kenaf fiber-reinforced PLA composites is increased. Alkali-treated kenaf-reinforced PLA composites created at 170°C curing temperature reported a maximum tensile strength of 72.81 MPa. The strengthened tensile characteristics ensuing from the alkali treatment are attributed to the elimination of the hemicellulose from the kenaf-fiber, as evidenced by FTIR spectroscopy.
It has been observed that after the surface treatments, the tensile strength of kenaf/PLA composites is improved. Similar results were obtained by M.M Owen et al (Owen et al. Citation2018; Owen, Achukwu, Shuib, et al. Citation2023). epoxy-coated and chemical-treated kenaf fibers generally improved the thermal stability as well as the mechanical properties of the composite samples compared to untreated-uncoated kenaf composites. Increment of the tensile strength follows the order: alkali treatment > potassium permanganate > potassium dichromate > untreated.
displays Young’s Modulus of surface-treated kenaf/PLA-based composites. Recorded values of Elastic Modulus show the maximum of 3.95 GPa for alkali-treated kenaf/PLA composites developed at 170°C curing temperature. M.M. Owen (Owen et al. Citation2018) presented the various surface treatment techniques for improving the mechanical and thermal properties of kenaf/polymer composites and the results are highly inline with the present study.
All in all, a higher tensile and flexural strength for the alkali-treated kenaf-fiber is attributable to the removal of hemi-cellulose content which leads to the strengthening of the interfacial-bonding, and adhesion-strength with well bonded adherence binding among the PLA-matrix and reinforcement.
Impact of fiber loadings, processing temperature, and surface treatment on the flexural properties of Kenaf/PLA composites
The 3-Point bend test was performed on kenaf/PLA composites with the help of a universal testing machine. illustrates how changes in the fiber volume fraction and molding temperature affect the flexural strength of kenaf/PLA composites.
According to the results of the current study, flexural strength increases as fiber volume fraction increases up to a point of 35%, after which it declines as fiber content increases to 50%. For kenaf-reinforced PLA composites with 35% fiber loading by volume and 170°C molding temperature, the maximum flexural strength was measured to be 86.956 MPa. shows the flexural modulus of kenaf/PLA composites. According to test results, kenaf-reinforced PLA composites with 35% fiber loading and 170°C molding temperature have a maximum flexural modulus of 7.99 GPa. This is also in agreement with other studies on natural fiber composites (Owen, Achukwu, Shuib, et al. Citation2023).
represents the impact of different surface treatments on the bending strength of the Kenaf/PLA based composites. It is recorded that the flexural strength of kenaf fiber-reinforced PLA-based composites is increased following the fiber surface treatments. Increment of the flexural strength follows the order: alkali treatment > potassium permanganate > potassium dichromate > untreated. Highest bending strength of 95.45 MPa was recorded for NaOH treated kenaf reinforced PLA composites developed at 170°C molding temperature. show the Flexural Modulus of surface treated kenaf reinforced PLA composites. Recorded values of Flexural Modulus show the maximum of 9.37 GPa for alkali treated kenaf reinforced PLA composites fabricated at 170°C curing temperature.
Hardness properties of kenaf/PLA composites
The hardness of kenaf/PLA composites is shown in . According to the findings, the Barcol hardness rises linearly as the fiber volume fraction rises before sharply falling off once it reaches its maximum value of 34.38. The composites produced at a molding temperature of 170°C with a volumetric fiber loading of 35% are found to have the greatest Barcol hardness numbers.
Water absorption properties of kenaf/PLA composites
At various molding temperatures, the proportion of water input for kenaf/PLA composites formed with varying fiber volume fractions was calculated. displays the kenaf/PLA composites’ water absorption. The amount of water absorbed has been seen to rise as the amount of fiber loading is increased. As the percentage of fiber volume increased from 45% to 50%, a significant shift in water absorption was seen. For kenaf/PLA composites with a 50% fiber volume percentage, a maximum of 16.77% was noted.
Conclusions
Untreated and surface treated Kenaf/PLA composites with different fiber volume fractions between 25% and 50% were prepared using compression molding process. The impact of surface treatments (Alkali treatment, potassium permanganate, and potassium dichromate), molding temperatures between 160°C and 180°C and different fiber loading on the mechanical characteristics of kenaf/PLA composites were investigated. It can be concluded that:
Composites made of kenaf and PLA that had a 35% volume percentage of fiber and were molded at a temperature of 170°C demonstrated the maximum tensile and flexural strength. In association to neat PLA.
Kenaf/PLA composites with a 35% fiber volume percentage demonstrated increases in flexural and tensile strength of 44.92% and 62.95%, respectively.
FTIR spectroscopic studies showed that surface modifications of the kenaf fibers takes place after each fiber treatment.
When compared to other fiber surface treatments, alkali treatment of kenaf fibers results in the greatest increase in tensile and flexural strength.
Alkali treated woven kenaf fiber composites with greater matrix adhesion have optimum tensile and flexural strength of 72.81 MPa and 94.45 MPa, respectively.
The increment of tensile and flexural strengths follows an order of Alkali treatment > Potassium permanganate > Potassium Dichromate > untreated, for kenaf/PLA composites.
To achieve the best mechanical properties while molding Kenaf/PLA composites, a temperature of 170°C is recommended.
With regard to their mechanical qualities, Kenaf/PLA composite laminates have the potential to displace the PVC wall panels now sold in the market.
Author contributions
Conceptualization, JIPS, VS, Sehijpal Singh (SS), VD, AB, RK, Shubham Sharma (SS); methodology, JIPS, VS, Sehijpal Singh (SS), VD, AB, RK, Shubham Sharma (SS); formal analysis, JIPS, VS, Sehijpal Singh (SS), VD, AB, RK, Shubham Sharma (SS); investigation, JIPS, VS, Sehijpal Singh (SS), VD, AB, RK, Shubham Sharma (SS); writing – original draft preparation, JIPS, VS, Sehijpal Singh (SS), VD, AB, RK, Shubham Sharma (SS); writing – review and editing, Shubham Sharma (SS), AK, FAA, MIK, EAAI; supervision, Shubham Sharma (SS), AK, FAA, MIK, EAAI; project administration, Shubham Sharma (SS), AK, FAA, MIK, EAAI; funding acquisition, Shubham Sharma (SS), AK, FAA, MIK, EAAI. All authors have read and agreed to the published version of the manuscript.
Consent to publish
All authors have read and approved this manuscript.
Highlights
The study has examined how Alkali, potassium permanganate, and potassium dichromate affect untreated and treated kenaf fibers employed in PLA composite reinforcement.
The study revealed that alkali treatment of kenaf fibers increases tensile and flexural strength, suggesting that surface treatments can enhance composite characteristics.
The research has discovered that kenaf/PLA composites that have a 35% fiber volume fraction and molded at 170°C have the highest tensile and flexural strength.
FTIR spectroscopic studies has showed that surface modifications of the kenaf fibers takes place after each fiber treatment.
The investigation has evaluated the impact of processing factors on the interfacial bonding between the kenaf/PLA composites with the lowest and greatest tensile strengths.
Kenaf/PLA composite laminates can replace PVC wall panels in commercial applications due to their mechanical characteristics.
Acknowledgments
Researchers Supporting Project number (RSPD2024R576), King Saud University, Riyadh, Saudi Arabia.
Disclosure statement
No potential conflict of interest was reported by the author(s).
Data availability statement
My manuscript has no associate data.
References
- Achukwu, E. O., M. M. Owen, A. Danladi, B. M. Dauda, A. Z. Romli, U. S. Ishiaku, and H. M. Ali. 2023. “Effect of Glass Fiber Loading and Reprocessing Cycles on the Mechanical, Thermal, and Morphological Properties of Isotactic Polypropylene Composites.” Journal of Applied Polymer Science 140 (10): 10. https://doi.org/10.1002/app.53588.
- Ashok, N. A., R. Kumar, M. Singh, J. Singh, P. Gulati, and J. Singh. 2021. “Development Method, Manufacturing Process of Fibre Reinforced Polymer Composite Type Helical Springs: A Review.” In Recent Trends in Engineering Design. Lecture Notes in Mechanical Engineering, edited by A. Parey, R. Kumar, and M. Singh. Singapore: Springer. https://doi.org/10.1007/978-981-16-1079-0_29.
- ASTM Standard D 2583. 1995. Standard Test Method for Indentation Hardness of Rigid Plastics by Means of a Barcol Impressor 1. West Conshohocken, PA: ASTM International. https://doi.org/10.1520/D2583-13A. www.astm.org.
- ASTM Standard D 3039/D 3039M – 07. 2008. Standard Test Method for Tensile Properties of Polymer Matrix Composite Materials 1. West Conshohocken, PA: ASTM International. https://doi.org/10.1520/D3039. www.astm.org.
- ASTM Standard D 570. 1999. Standard Test Method for Water Absorption of Plastics 1. West Conshohocken, PA: ASTM International. https://doi.org/10.1520/D0570-98R18. www.astm.org.
- ASTM Standard D 790 – 02. 2002. Flexural Properties of Unreinforced and Reinforced Plastics and Electrical Insulating Materials 1. West Conshohocken, PA: ASTM International. https://doi.org/10.1520/D0790-10. www.astm.org.
- Azlin, M. N. M., S. M. Sapaun, M. Y. M. Zuhri, and E. S. Zainudin. 2022. “Effect of Stacking Sequence and Fiber Content on Mechanical and Morphological Properties of Woven Kenaf/Polyester Fiber Reinforced Polylactic Acid (PLA) Hybrid Laminated Composites.” Journal of Materials Research and Technology 16:1190–23. https://doi.org/10.1016/j.jmrt.2021.12.046.
- Bajpai, P. K., D. Meena, S. Vatsa, and I. Singh. 2013. “Tensile Behavior of Nettle Fiber Composites Exposed to Various Environments.” Journal of Natural Fibers 10 (3): 244–256. https://doi.org/10.1080/15440478.2013.791912.
- Bajpai, P. K., I. Singh, and J. Madaan. 2014. “Development and Characterization of PLA-Based Green Composites: A Review.” Journal of Thermoplastic Composite Materials 27 (1): 52–81. https://doi.org/10.1177/0892705712439571.
- Bulut, Y., and A. Aksit. 2013. “A comparative study on chemical treatment of jute fiber: potassium dichromate, potassium permanganate and sodium perborate trihydrate.” Cellulose 20 (6): 3155–3164. https://doi.org/10.1007/s10570-013-0049-6.
- Chandel, P. S., Y. K. Tyagi, K. Jha, R. Kumar, S. Sharma, J. Singh, and R. A. Ilyas. 2021. “Study of Mode II Interlaminar Fracture Toughness of Laminated Composites of Glass and Jute Fibres in Epoxy for Structural Applications.” Functional Composites and Structures 3 (4): 044002. https://doi.org/10.1088/2631-6331/ac376e.
- Fajrin, J., A. Akmaluddin, and F. Gapsari. 2022. “Utilization of Kenaf Fiber Waste As Reinforced Polymer Composites.” Results in Engineering 13:100380. https://doi.org/10.1016/j.rineng.2022.100380.
- Gomes, A. 2007. “Development and Effect of Alkali Treatment on Tensile Properties of Curaua Fibers Green Composites.” Composites Part A, Applied Science and Manufacturing 38 (8): 1811–1820. https://doi.org/10.1016/j.compositesa.2007.04.010.
- Hamidon, H., T. H. Sultan, A. H. Ariffin, and U. M. Shah. 2019. “Effects of Fibre Treatment on Mechanical Properties of Kenaf Fibre Reinforced Composites: A Review.” Journal of Materials Research and Technology 8 (3): 3327–3337. https://doi.org/10.1016/j.jmrt.2019.04.012.
- Jaafar, W., S. N. Surip, N. N. Azmi, N. N. Bonnia, H. Anuar, H. N. Azlina, and S. B. Razak. 2012. “Thermal Properties of PLA/Kenaf Green Nanocomposites: Effect of Chemi-Mechanical Treatment.” Advanced Materials Research 576:342–344. https://doi.org/10.4028/www.scientific.net/AMR.576.342.
- Jaiinder, S. P., S. Singh, and V. Dhawan. 2017. “Study of Effect of Surface Treatment on Mechanical Properties of Natural Fiber Reinforced Composites.” Materials Today: Proceedings 4 (2): 2793–2799. https://doi.org/10.1016/j.matpr.2017.02.158.
- Jaiinder, S. P., S. Singh, and V. Dhawan. 2018. “Effect of Curing Temperature on Mechanical Properties of Natural Fiber Reinforced Polymer Composites.” Journal of Natural Fibers 15 (5): 687–696. https://doi.org/10.1080/15440478.2017.1354744.
- Jaiinder, S. P., S. Singh, and V. Dhawan. 2019a. “Effect of Alkali Treatment on Mechanical Properties of Jute Fiber-Reinforced Partially Biodegradable Green Composites Using Epoxy Resin Matrix.” Polymers & Polymer Composites. https://doi.org/10.1177/0967391119880046.
- Jaiinder, S. P., S. Singh, and V. Dhawan. 2019b. “Influence of Fiber Volume Fraction and Curing Temperature on Mechanical Properties of Jute/PLA Green Composites.” Polymers & Polymer Composites. https://doi.org/10.1177/0967391119872875.
- Jaiinder, S. P., S. Singh, V. Dhawan, A. Pannu, and S. Sarath. 2017. “Effect of Alkali Treatment on Mechanical Properties of Jute, Sisal, Banana, Hemp and Abaca Fibers for Polymer Composite Reinforcement.” International Journal of Mechanical Engineering 8 (7): 1775–1784.
- Jha, K., P. Tamrakar, R. Kumar, S. Sharma, J. Singh, R. A. Ilyas, S. M. Rangappa, et al. 2022. “Effect of Hybridization on Physio-Mechanical Behavior of Vetiver and Jute Fibers Reinforced Epoxy Composites for Structural Applications: Studies on Fabrication, Physicomechanical, Water-Absorption, and Morphological Properties.” Journal of Industrial Textiles 51 (2_suppl): 2642S–2664S. https://doi.org/10.1177/15280837221098573.
- Jha, K., Y. K. Tyagi, R. Kumar, S. Sharma, M. R. M. Huzaifah, C. Li, R. A. Ilyas, S. P. Dwivedi, A. Saxena, and A. Pramanik. 2021. “Assessment of Dimensional Stability, Biodegradability, and Fracture Energy of Bio-Composites Reinforced with Novel Pine Cone.” Polymers 13 (19): 3260. https://doi.org/10.3390/polym13193260.
- John, S., A. Virk, and H. Wayne. 2013. “A Review of Bast Fibres and Their Composites: Part 3 – Modelling.” Composites Part A, Applied Science and Manufacturing 44 (1): 132–139. https://doi.org/10.1016/j.compositesa.2012.08.018.
- Kumar, R., J. Singh, S. Sharma, C. Li, G. Królczyk, and S. Wojciechowski. 2022. “Neutrosophic Entropy-Based Ingenious Measurement for Fast Fourier Transforms Based Classification of Process Parameters and Wear Resistance of Friction-Stir Processed Hybrid AA7075- B4C Aluminium Metal-Matrix Composites.” Journal of Materials Research and Technology 20:720–739. https://doi.org/10.1016/j.jmrt.2022.07.026.
- Lu, C., C. H. Wang, C. H. Li, J. F. Tong, and Y. F. Sun. 2022. “Structural and Mechanical Properties of Hemp Fibers: Effect of Progressive Removal of Hemicellulose and Lignin.” Journal of Natural Fibers 19 (16): 13985–13994. https://doi.org/10.1080/15440478.2022.2113851.
- Mukherjee, T., and N. Kao. 2011. “PLA Based Biopolymer Reinforced with Natural Fibre.” Journal of Polymers and the Environment 19 (3): 714–725. https://doi.org/10.1007/s10924-011-0320-6.
- Nurazzi, N., A. Khalina, S. M. Sapuan, R. A. Ilyas, S. A. Rafiqah, and Z. M. Hanafee. 2020. “Thermal Properties of Treated Sugar Palm Yarn/Glass Fiber Reinforced Unsaturated Polyester Hybrid Composites.” Journal of Materials Research and Technology 9 (2): 1606–1618. https://doi.org/10.1016/j.jmrt.2019.11.086.
- Ochi, S. 2008. “Mechanical Properties of Kenaf Fibers and Kenaf/PLA Composites.” Mechanics of Materials 40 (4): 446–452. https://doi.org/10.1016/j.mechmat.2007.10.006.
- Owen, M. M. 2022. “Emmanuel Okechukwu Achukwu, and Hazizan Md Akil. “Preparation and Mechanical Characterizations of Water Hyacinth Fiber Based Thermoset Epoxy Composite.” Journal of Natural Fibers 19 (16): 13970–13984.
- Owen, M. M., E. O. Achukwu, H. M. Akil, A. Z. Romli, M. S. Z. Abidin, I. O. Arukalam, and U. S. Ishiaku. 2022. “Effect of Epoxy Concentrations on Thermo-Mechanical Properties of Kenaf Fiber – Recycled Poly (Ethylene Terephthalate) Composites.” Journal of Industrial Textiles 52:152808372211274. https://doi.org/10.1177/15280837221127441.
- Owen, M. M., E. O. Achukwu, I. O. Arukalam, and A. Z. Romli. 2022. “Effect of Varying Processing Temperatures on the Mechanical and Microstructural Properties of Kenaf Fibre-ABS Composites for Moderate Temperature Applications.” Polymers from Renewable Resources 13 (3): 154–169. https://doi.org/10.1177/20412479221122676.
- Owen, M. M., E. O. Achukwu, A. K. Hazizan, Z. R. Ahmad, A. H. Abdullah, and U. S. Ishiaku. 2023. “Characterization of Recycled and Virgin Polyethylene Terephthalate Composites Reinforced with Modified Kenaf Fibers for Automotive Application.” Polymer Composites 43 (11): 7724–7738. https://doi.org/10.1002/pc.26866.
- Owen, M. M., E. O. Achukwu, A. A. A. Rahman, A. Z. Romli, M. R. Ahmad, S. B. Shuib, and H. M. Akil. 2023. “Mechanical and Morphological Characterizations of Epoxy Composites Reinforced with Surface Modified Woven Cotton Structures Using Vacuum Bagging Technique.” The Journal of the Textile Institute 1–15. https://doi.org/10.1080/00405000.2023.2258047.
- Owen, M. M., E. O. Achukwu, A. Z. Romli, and H. M. Akil. 2023. “Recent Advances on Improving the Mechanical and Thermal Properties of Kenaf Fibers/Engineering Thermoplastic Composites Using Novel Coating Techniques: A Review.” Composite Interfaces 30 (8): 849–875. https://doi.org/10.1080/09276440.2023.2179238.
- Owen, M. M., E. O. Achukwu, S. B. Shuib, Z. R. Ahmad, A. H. Abdhullah, and U. S. Ishiaku. 2023. “Effects of High-Temperature Optimization and Resin Coating Treatment on the Mechanical, Thermal, and Morphological Properties of Natural Kenaf Fiber-Filled Engineering Plastic Composites.” Polymer Composites 44 (4): 2512–2529. https://doi.org/10.1002/pc.27260.
- Owen, M. M., E. O. Achukwu, S. B. Shuib, Z. R. Ahmad, A. H. Abdullah, and U. S. Ishiaku. 2023. “Effects of High-Temperature Optimization and Resin Coating Treatment on the Mechanical, Thermal, and Morphological Properties of Natural Kenaf Fiber-Filled Engineering Plastic Composites.” Journal of Natural Fibers 44 (4): 2512–2529. https://doi.org/10.1002/pc.27260.
- Owen, M. M., E. O. Achukwu, A. Zafir Romli, A. Halim Bin Abdullah, M. Hanif Ramlee, and S. Bin Shuib. 2023. “Thermal and Mechanical Characterization of Composite Materials from Industrial Plastic Wastes and Recycled Nylon Fibers for Floor Paving Tiles Application.” Waste Management 166:25–34. https://doi.org/10.1016/j.wasman.2023.04.038.
- Owen, M. M., U. S. Ishiaku, A. Danladi, B. M. Dauda, and A. Z. Romali. 2018. “Mechanical Properties of Epoxy-Coated Sodium Hydroxide and Silane Treated Kenaf/Recycled Polyethylene Tereph-Thalate (RPET) Composites: Effect of Chemical Treatment.” AIP 1985 (1): 030001. https://doi.org/10.1063/1.5047159.
- Owen, M. M., U. S. Ishiaku, A. Danladi, B. M. Dauda, A. Z. Romli, 2018a. The Effect of Surface Coating and Fibre Loading on Thermo-Mechanical Properties of Recycled Polyethylene Terephthalate (Rpet)/epoxy-Coated Kenaf Fibre Composites. AIP Conference Proceedings, 1985:1, https://doi.org/10.1063/1.5047160.
- Owen, M. M., U. S. Ishiaku, A. Danladi, B. M. Dauda, A. Z. Romli, 2018b. Mechanical Properties of Epoxy-Coated Sodium Hydroxide and Silane Treated Kenaf/Recycled Polyethylene Tereph-Thalate (RPET) Composites: Effect of Chemical Treatment, AIP Conference Proceedings, 1985:1, https://doi.org/10.1063/1.5047159.
- Pengju, P., B. Zhu, K. Weihua, S. Serizawa, M. Iji, and Y. Inoue. 2007. “Crystallization Behavior and Mechanical Properties of Bio-Based Green Composites Based on Poly (L-Lactide) and Kenaf Fiber.” Journal of Applied Polymer Science 105 (3): 1511–1520. https://doi.org/10.1002/app.26407.
- Rawi, N. F. M., K. Jayaraman, and D. Bhattacharyya. 2013. “A Performance Study on Composites Made from Bamboo Fabric and Poly (Lactic Acid).” Journal of Reinforced Plastics & Composites 32 (20): 1513–1525. https://doi.org/10.1177/0731684413498296.
- Saba, N., M. T. Paridah, and M. Jawaid. 2015. “Mechanical Properties of Kenaf Fiber Reinforced Polymer Composite: A Review.” Construction and Building Materials 76:87–96. https://doi.org/10.1016/j.conbuildmat.2014.11.043.
- Shahar, F. S., H. Sultan, A. Safri, J. Md, T. Abd, A. Basri, and A. Md Shah. 2022. “Fatigue and Impact Properties of 3D Printed PLA Reinforced with Kenaf Particles.” Journal of Materials Research and Technology 16:461–470. https://doi.org/10.1016/j.jmrt.2021.12.023.
- Shih, Y. F., and C. C. Huang. 2011. “Polylactic acid (PLA)/banana fiber (BF) biodegradable green composites.” Journal of Polymer Research 18 (6): 2335–2340. https://doi.org/10.1007/s10965-011-9646-y.
- Singh, J. I. P. 2021. “Kenaf-Fiber-Based Bio-Materials: A Review on Processing and Mechanical Properties”. In Recent Trends in Engineering Design. Lecture Notes in Mechanical Engineering In A. Parey, R. Kumar, and M. Singh edited by, Singapore: Springer. https://doi.org/10.1007/978-981-16-1079-0_10.
- Singh, J. P. 2020. “Influence of Surface Treatment and Molding Temperature on Mechanical Properties of Jute/PLA-Based Green Composites”. In Advances in Materials Science and Engineering. Lecture Notes in Mechanical Engineering In C. Prakash, S. Singh, G. Krolczyk, and B. Pabla edited by, Singapore: Springer. https://doi.org/10.1007/978-981-15-4059-2_12.
- Smitthipong, W., R. Tanthatherdtam, and R. Chollakup. 2013. “Effect of Pineapple Leaf Fiber-Reinforced Thermoplastic Starch/Poly (Lactic Acid) Green Composites: Mechanical, Viscosity and Water Resistance Properties.” Journal of Thermoplastic Composite Materials 28 (5): 717–729. https://doi.org/10.1177/0892705713489701.
- Yeswanth, I. V. S., K. Jha, S. Bhowmik, R. Kumar, S. Sharma, and A. I. Rushdan. 2022. “Recent Developments in RAM Based MWCNT Composite Materials: A Short Review.” Functional Composites & Structures 4 (2): 024001. https://doi.org/10.1088/2631-6331/ac5730.
- Yusoff, R. B., H. Takagi, and A. N. Nakagaito. 2016. “Tensile and Flexural Properties of Polylactic Acid-Based Hybrid Green Composites Reinforced by Kenaf, Bamboo and Coir Fibers.” Industrial Crops and Products 94:562–573. https://doi.org/10.1016/j.indcrop.2016.09.017.