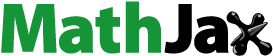
ABSTRACT
Comfort is a qualitative feature and one of the requirements that modern garment buyers place on their purchases. The purpose of this work is to study the effect of weft yarn type, weft yarn tension, warp yarn tension, and weft density on drape and surface roughness properties of cotton plain woven fabric. Each factor was treated at two levels such as yarn type (ring-spun and rotor-spun), warp tension (1 kN and 2 kN), weft tension (PFT/B- and PFT/B+T) and weft density (14 picks/centimeter (PPC) and 18 PPC). Full factorial design was used to design the experiment and analyze the results of the experiment. The results of the analysis showed that fabric produced from ring-spun yarn has smoother surface and better drape than fabric produced from rotor spun yarn. As weft and warp yarn tension increased, the surface roughness of the fabric increased and fabric drape decreased. On the other hand, fabric drape decreased as the weft density increased and the surface roughness of the fabric increase as weft density decreased.
摘要
舒适性是现代服装购买者在购买时的一个质的特征和要求之一. 研究了纬纱种类、纬纱张力、经纱张力和纬纱密度对纯棉平纹织物悬垂性和表面粗糙度的影响. 每个因素在两个水平上进行处理,如纱线类型(环锭纺和转杯纺)、经线张力(1kN和2kN)、纬线张力(PFT/B-和PFT/B+T)和纬线密度(14纬/厘米(PPC)和18PPC). 采用全因子设计对实验进行设计,并对实验结果进行分析. 分析结果表明,环锭纱织物比转杯纱织物具有更光滑的表面和更好的悬垂性. 当纬纱和经纱张力增加时,织物表面粗糙度增加,悬垂性降低. 另一方面,织物悬垂性随着纬纱密度的增加而降低,织物表面粗糙度随着纬纱浓度的降低而增加.
Introduction
Woven fabric has the biggest market share compared to non-woven and knitted fabrics (Adanur Citation2020). The method of manufacturing woven fabrics involves weaving warp and weft threads at right angles to one another. During the interlacement process, the warp yarns are twisted and stressed while the weft yarn is put between them (Walters et al. Citation2005). Woven fabric quality is determined by technical and technological elements, which must be altered during fabric design (Behera Citation2008). The physical, mechanical, and comfort characteristics of cloth are impacted by this shift in technical and technological factors.
Comfort is a qualitative feature of clothing, and it is one of the requirements that modern garment buyers place on their purchases. The sensation of comfort is a result of the accumulation of impulses that the brain’s many receptors send to the nerves. Fabric comfort is a basic requirement for a clothing system. Given that people interact with fabrics on a daily basis, it is a topic of discussion on a worldwide scale. The three primary requirements that people have for clothing are good comfort, affordability, and high-quality fabric. Fabric comfort is necessary while selecting proper apparel to wear (Edwin et al. Citation2013).
Clothing is chosen based on a variety of factors, including personal preferences and specific use, all of which are user-dependent. However, as illustrated in , people’s preferences might change depending on the season, surroundings, age, and type of activity. Comfort can be considered as one technique for measuring the performance of clothing by the wearer. In this regard, fabric engineers and clothing designers ought to consider clothing comfort as a quality component that contributes to overall garment performance and user pleasure.
Figure 1. The clothing comfort triad reviewed from (Kamalha et al. Citation2013).

Fabric comfort is classified as sensory and thermal comfort (N Nassif Citation2017). Sensorial comfort refers to the varied sensations of discomfort felt by the wearer when clothing contacts his or her skin entirely or partially. Sensorial comfort qualities characterize a fabric’s performance on skin contact, including fabric handle, drape, bending, and fabric surface features.
Surface friction, roughness, and stiffness are all qualities that contribute to sensory fabric comfort. It is a sensory reaction of nerves terminating to external stimuli such as temperature, pressure, and pain-producing neuro-physiological impulses that are delivered to the brain.
Fiber composition, yarn characteristics, threads density, and loom settings, influence sensory comfort attributes (Özdemir Citation2017). The influence of weft yarn twist level on sensory comfort qualities of 100% woven cotton fabric was investigated by (Atalie, Ferede, and Rotich Citation2019). The results showed that as the twist level grows, so did the shear and bending properties. Aliouche and Viallter (Citation2000) studied the yarn hairiness influences on sensory comfort qualities including surface roughness and fabric compression. According to (Yan, Höcker, and Schäfer Citation2000), bleached fabrics have a coarser handle and less fullness than unbleached fabrics. This study focused on the drape ability and surface roughness features of plain-woven fabrics as sensory comfort properties.
Subjective and objective metrics can be used to determine the cloth handling qualities. Fabric handles have features such as stiffness, drape, smoothness, weight, thickness, and compressibility. However, fabric stiffness and drape were the first qualities to be scientifically quantified (Özdil et al. Citation2014).
Drape ability is the degree to which a cloth deforms to position itself into folds when it is partially supported by other objects. It is one of the fabric qualities that influence the esthetics of clothes and interior textiles. Fabrics are distinguished from other sheet materials by their capacity to fold in two directions at the same time. This characteristic allows one to shape a fabric to the desired form (Joy Citation2021). The fabric’s ability to drape is influenced by a number of factors, including thickness, weight, fabric cover factor, and stiffness. Stiffness is one of the most commonly used metrics to assess fabric bending rigidity and drape quality. This attribute can have an impact on both comfort and esthetics. The stiffness of the cloth is determined by its geometrical properties (Özdil et al. Citation2014). Bending rigidity measures fabric stiffness, indicating its flexural capacity, resistance to bending forces, and ease of bending. This characteristic has a considerable impact on fabric comfort. Fiber type, yarn structure, fabric structure, and yarn density have an impact on the stiffness and drape of a fabric (Özdil et al. Citation2014).
Surface friction and roughness are essential and effective predictors of cloth tactile characteristics. Fabric friction, defined as resistance to motion, can be measured by rubbing a fabric mechanically against itself or tactually between the finger and thumb. It is critical to quantify fabric friction and roughness, as well as the elements that may influence them. Fiber morphological features influence fabric surface properties (Das, Kothari, and Vandana Citation2005). According to (Aliouche and Viallter Citation2000), yarn hairiness impacts the surface roughness and surface friction qualities of the fabric. Fabrics made from yarns with more twist have lower surface friction than fabrics made from yarns with less twist (Atalie, Ferede, and Rotich Citation2019). According to (Beyene and Kumelachew Citation2022), the surface roughness property values of woven fabrics rose consistently while switching from plain to sateen weave.
All of the studies thus far have concentrated on the effects of yarn properties such as twist, hairiness, and fines on the sensorial comfort properties of the fabric. Furthermore, a great deal of research has been done on the effects of fabric density and structure on sensory comfort. The sensory qualities of woven fabric are influenced by a wide range of factors in addition to yarn and fabric attributes. This study uses complete factorial design to investigate the effects of ring-spun and rotor-spun yarns, as well as loom settings such as weft and warp yarn tension, on the sensory comfort of plain woven fabric, which has not been examined previously to the best of our knowledge.
Materials and methodology
Materials
The required materials used for this study were ring and rotor-spun weft yarns and rotor-spun warp yarns. The same count of both ring and rotor spun weft yarns were used to produce the fabrics samples. The input yarns are produced using a Ring frame (Rieter G35 Model) and a Rotor spinning machine (Rieter-R 923) in Bahir Dar Textile Share Company, Bahir Dar, Ethiopia. The detailed properties of both rotor and ring spun yarns are described in .
Table 1. Input yarn characteristics.
Methodology
Experimental design
This research utilized full factorial designs, an advanced experimental design, to examine the relationship between multiple input variables and response variables. The factorial design allows researchers to look at how multiple factors affect a dependent variable (in this case how the weft yarns types, weft yarn tension, warp yarn tension, and weft density affect the sensorial comfort properties of fabric) both independently and together. The level of each factors are illustrated in .
Table 2. Experimental factors and their levels.
The experimental designs specified by the full factorial design (FFD) considering the four factors such as weft yarn types, weft yarn tension, warp yarn tension, and weft density and the respective experimental response results are given in .
Table 3. Sensorial comfort properties experimental results.
Fabric sample production and sampling
Sixteen samples of 1/1 168 cm width plain fabric were made by Picanol Air jet weaving machines (OMNIPLUS 800) in Ethiopia, Bahir Dar Textile Share Company as per the experimental design mentioned in .
The fabric samples for this investigation were taken 50 mm away from the selvage. The suggested methods for cutting laboratory specimens were taken into consideration for the various types of fabric variety in order to guarantee that the specimens were as true a representation of the fabric as possible.2.2.3 Characterizations of Sensorial comfort properties
Fabric drape test
Fabric drape is the extent to which a fabric deforms when it is allowed to hang under its weight. A Cusic drape tester is used to measure the drape property of the fabric using a parallel beam of light to cast a shadow from a circular piece of fabric supported by a small circular disk. Drape coefficient (D) describes the degree of fabric deformation by gravity when the fabric allows hanging under its weight. It is the ratio of the area of the projection of the draped ring of fabric to the area of the non-draped ring of fabric multiplied by 100. The low value of the drape coefficient means the fabric has a good drape. The samples are prepared with a circular disc diameter of 36 cm and the diameter of the paper ring for capturing the shadow of the draped fabric also have similar dimension. It is calculated using the formula given in EquationEquation 1(1)
(1) .
Fabric surface roughness test
Surface roughness is a critical quality for the tactile properties of fabrics used in healthcare settings, hygiene items, protective garments, and bedding that must meet specific requirements while in contact with the user. The SDL Atlas Fabric Touch Tester (FTT) is a cutting-edge testing device that measures several fabric hand qualities in a single test that takes about 2–3 minutes. According to preliminary research, FTT measurements have a significant association with human subjective touch experiences, allowing it to evaluate and discriminate fabric touch comfort qualities. SDL Atlas fabric Touch Tester (FTT) was used to evaluate the surface properties of fabrics. The system acquires data from sensors for measuring the surface roughness amplitude within fabric samples. The average values of five replicates were used for the analysis.
Data analysis
After the completion of the fabric properties test, the data was analyzed with the help of Design Expert 13 software and factorial design. The analysis techniques used in this experimental study are ANOVA, regression equation, and 3D plot. The regression analysis indicates the empirical relationship between the independent variables and dependent variables. A higher regression coefficient of a given response indicates that the response has been significantly affected by the factor. The sign (+,-) of the regression coefficient indicates that response increase or decrease as a result of changing a factor. On the other hand, the ANOVA provides 95% confidence limits on whether the factors had a substantial impact on the response variables.
Result and discussion
Sensorial comfort properties analysis
Test results of sensorial comfort properties such as fabric drape coefficient (DC) and surface roughness amplitude (SRA) of 16 fabric samples are described in .
Fabric drape
The drape of fabric was described by the drape coefficient percentage. The drape coefficient is the ratio of the projected area of the fabric sample to its undraped area. Fabric drape is inversely proportional to the drape coefficient. As shown in , the p-value for the model is 0.0007. This indicates the model is significant since the P-value is less than 0.05. The weft yarn types, warp tension, weft tension, weft density, and the interaction effect of warp tension and weft density have a significant effect on the fabric drape.
Table 4. The ANOVA (P) values of drape coefficient and surface roughness (in weft and warp direction).
As stated in , the R2 value for drape coefficient is 98.38%. This indicates the independent variable explains 98.38% of the drape coefficient of the fabric.
Table 5. The regression equation and R2 values for drape coefficient and surface roughness of fabric.
Effect of ring and rotor weft yarns on the fabric drape properties
Because the two weft yarns have different internal and external yarn structures as a result of the different manufacturing procedures used, the drape of fabric generated from ring and rotor spun weft yarns varies significantly. As shown in , the paired fabric samples are produced from a similar count (16Ne) of ring and rotor weft yarns. The fabric samples produced from rotor weft yarns have a higher drape coefficient than the fabric produced from ring weft yarn at constant yarn tension and weft density. This means the fabric produced from rotor weft yarns has less drape than fabrics produced from ring weft yarns. The three-dimensional bending process of the fabric depends on the bending rigidity of the threads and the mobility of warp/weft yarns within the fabric. The mobility of the threads in fabric made from rotor spun weft yarn is lower than that of fabric produced from ring weft yarn. This is because, during the formation of rotor spun yarns, the core of fibers that are aligned with the helix of the inserted twist forms the bulk of the yarns, and some of the fibers are wrapped around spun yarns. This identifiable bulky structure of the rotor yarns due to the presence of rapper fibers and irregular surface structure prevents the mobility of warp/weft yarns within the fabric and reduced the three-dimensional bending performance of the fabrics. This is also in agreement with (Göktepe et al. Citation2010). As shown in , the maximum drape coefficient was achieved in samples 2 and 16 at constant warp tension (2 kN), weft tension (PFT/B+T) and 18 PPC, whereas the minimum was attained in samples 8 and 12 at constant warp tension (1 kN), weft tension (PFT/B-) and 14 PPC.
Effect of weft and weft yarn tension on the fabric drape
As seen in , the drape coefficient increased as both weft and warp yarn tension increased while maintaining the same type of weft yarn and weft density. This is due to the fact that as yarn tension rises, the yarn becomes compacted within the fabric, diminishing mobility of the thread and fabric’s ability to flex. This made the fabric more resistant to three-dimensional bending, resulting in higher drape coefficients in the resultant fabrics. Low yarn tension enhances yarn mobility in textiles, making fabric less draping-resistant and increasing drape coefficient and fabric drape. Likewise, it agrees with the research of (Süle Citation2012).
Effect of weft density on the fabric drape
The weft density has a positive correlation with the drape coefficient. Compared to other factors, weft density has a higher regression coefficient, as shown in . shows the drape coefficient increased when the weft density increased from 14 PPC to 18 PPC at constant yarn tension and the same weft yarn type. Higher weft density fabrics prevent relative fiber mobility in yarn and fabric, resulting in reduced fabric bending ability. This is in accordance with (Özdil et al. Citation2014). Whereas the more widely spaced the threads, the lower the cover factor of the fabric and the higher the mobility of yarns due to the fewer threads in the specified area. This causes the fabric to have a less stiff handle. This leads to a lower drape coefficient and higher fabric drape.
Interaction effect of independent variables on fabric drape
The interaction effect of warp tension and weft density had a significant effect on the drape of fabric but the other interaction effect does not have a significant effect as shown in . As shown in the 3D surface plot described in , the minimum drape coefficient (high drape) was attained at lower weft density (14 PPC) and lower warp yarn tension (1 kN). Whereas, the maximum drape coefficient (low drape) was attained at higher weft density (18 PPC) and higher warp yarn tension (2 kN). This is because higher weft density with higher yarn tension produces stiffer fabrics. This makes the fabric more resistant to bending, but fabrics with less weft density and low yarn tension have a higher ability to drape. This is due to the higher mobility of warp/weft yarns within the fabrics. This was achieved at constant weft tension and the same weft yarn types.
Surface roughness properties
Roughness is defined as the fabric surface’s vertical divergence from its ideal shape and is used to measure the texture of the fabric surface. If these variations are significant, the surface is characterized as rough; if they are small, the surface is considered smooth. The surface texture of a textile material is described by two components: roughness and waviness. Roughness is the result of short wavelengths, whereas waviness is the result of longer wavelengths within the observed roughness profile. The surface roughness of the 16 fabric samples were measured both in the warp and weft directions. The weft yarn types (ring and rotor), weft and warp yarn tension, weft density, and the interaction effect of weft yarn types and weft density, weft tension and weft density have a significant effect on the surface roughness properties of the fabric in both warp, and weft direction as illustrated in .
The R2 values of 97.98% and 97.77% for warp and weft direction of the fabric indicates the strong correlation between the given factors and surface roughness.
Effects of ring and rotor weft yarns on the fabric surface roughness
Ring and rotor spun weft yarns have significant effects on the surface roughness properties of the fabrics due to the different arrangements of fibers in the yarn. As shown in , the surface roughness of fabric made from rotor yarn is higher than that of fabric produced from ring-spun weft yarn. This is because the ring-spun yarn is characterized by a cylindrical helix of well-oriented fibers with a hairy surface. The rotor-spun yarn shows a bipartite (two-zone) structure comprising a core of fibers aligned with the helix of the inserted twist and an outer zone of wrapper fibers, which occurs irregularly along the core length. The yarns have a more random positioning, and the direction of fiber to yarn length could result in more unequal yarn surfaces. This resulted in higher surface roughness properties than fabric made from ring-spun yarn. As shown from the graph, the surface roughness of the fabrics in the warp direction is higher than that of weft direction. This is due to the higher surface roughness of the sized rotor warp yarn as compared to weft yarn. This is also supported by the study (Anindya et al. Citation2008).
Effects of weft and warp yarn tension on the surface roughness properties of fabrics
As seen in , the weft and warp yarn tension positively affected the surface roughness properties. As the weft and warp yarn tension increased, the surface roughness properties of the fabric in the weft and warp direction increased. An increase in warp tension causes more pressure on the weft yarn and forces it to form more weft crimp and less warp crimp and an increase in weft tension forces the warp yarn to form more warp crimp and less weft crimp. This crimp variation in the length-wise and width-wise direction of the fabric creates structural fabric surface difference due to the formation of very high and very low peaks, which in turn increased surface roughness and decrease the drape of fabric significantly (Süle Citation2012).
Effect of weft density on the surface roughness properties of fabrics
The weft density negatively affected the surface roughness properties of the fabric. As shown in , when the weft density increased from 14 PPC to 18 PPC the surface roughness properties in the warp and weft direction decreased at constant yarn tension for the same weft yarn types. As both warp and weft density increased the fabric becomes more tightened and compacted resulting in higher surface roughness properties. However fabrics with low weft density has a less compacted structure due to less closeness between yarns in the fabric structure. As a result, a fabric with low weft and warp density has lower surface roughness. The lower the roughness, the smoother is the fabrics. This is in agreement with (Moorthy and Kandhavadivu Citation2015).
Independent variables interaction effect on surface roughness properties
Among the four factors interaction effect observed in , the interaction effect of weft yarn types (ring and rotor) and weft density has a significant effect on surface roughness of the fabric in the warp direction whereas, the interaction effect of weft yarn tension and weft density has significant effects on surface roughness of the fabric in the weft direction as shown in . The minimum surface roughness is obtained at higher weft density (18 PPC) and ring weft yarn. The maximum is achieved at a lower weft density (14 PPC) and rotor weft yarn in the warp direction. Whereas, in the weft direction the minimum surface roughness value of the fabric was obtained at higher weft density and low weft yarn tension. But the maximum is attained at low weft density and higher weft yarn tension.
Conclusion
Clothing is chosen based on a variety of factors, including personal preferences and specific use, all of which are user-dependent. Sensorial comfort refers to the varied sensations of discomfort felt by the wearer when clothing contacts with human skin entirely or partially. This research deals with the effect of ring and rotor spun weft yarns, weft yarn tension, warp yarn tension and weft density on drape and surface roughness of woven fabric. The results of the experiment revealed that fabric produced from ring-spun weft yarn shows better drape and surface roughness properties than fabric produced form rotor spun yarn.
An increase in warp tension causes more pressure on the weft yarn and forces it to form more weft crimp and less warp crimp and an increase in weft tension forces the warp yarn to form more warp crimp and less weft crimp. This crimp variation in the length-wise and width-wise direction of the fabric creates structural fabric surface difference due to the formation of very high and very low peaks, which in turn increased surface roughness and decreased the drape of fabric significantly. Moreover, while the weft density increases the fabric sample stiffness and surface roughness increase and vice versa. In general, fabric made from ring-spun yarn has smoother surface and highly drape able than fabric made from rotor-spun yarn.
The minimum drape coefficient (high drape) was achieved at lower weft density (14 PPC) and lower warp yarn tension (1 kN); however, maximum drape coefficient (low drape) was achieved at higher weft density (18 PPC) and higher warp yarn tension (2 kN).
Highlights
Comfort is a qualitative feature and one of the requirements that modern garment buyers place on their purchases.
The results of the experiment revealed that fabric produced from ring-spun weft yarn shows better drape and surface roughness properties than fabric produced form rotor spun yarn.
Fabric made from ring-spun yarn has smoother surface and highly drape able than fabric made from rotor-spun yarn.
When the warp and weft yarn tension increased, surface roughness properties of the fabric increased and, the drape of fabric decreased significantly.
While the weft density increases the fabric sample stiffness and surface roughness increased.
Author’s contribution
Author1: Andebet Kassaw: Data collection, designing the experiment, conducting the experiment, and analysis of data
Author 2: Million Ayele: Conception or design, writing the manuscript, and final approval of the version to be published.
Ethical approval
All content of this research didn’t involve human or animal use.
Acknowledgments
The authors would like to acknowledge the Ethiopian Institute of Textile and Fashion Technology, Bahir Dar University, Bahir Dar, Ethiopia for the support of this project.
Disclosure statement
No potential conflict of interest was reported by the author(s).
References
- Adanur, S. 2020. Handbook of Weaving (CRC Press). https://doi.org/10.1201/9780429135828.
- Aliouche, D., and P. Viallter. 2000. “Mechanical and Tactile Compression of Fabrics: Influence on Handle.” Textile Research Journal 70 (11): 939–12. https://doi.org/10.1177/004051750007001101.
- Anindya, G., R. D. A. Asis Patanaik, R. S. Rengasamy, and R. S. Rengasamy. 2008. “A Study on Dynamic Friction of Different Spun Yarns.” Journal of Applied Polymer Science 108 (5): 3233–3238. https://doi.org/10.1002/app.27633.
- Atalie, D., A. Ferede, and G. K. Rotich. 2019. “Effect of Weft Yarn Twist Level on Mechanical and Sensorial Comfort of 100% Woven Cotton Fabrics.” Fashion and Textiles 6 (2019): 1–12. https://doi.org/10.1186/s40691-018-0169-6.
- Behera, B. K. 2008. “Weaving Technology for Manufacturing High Performance Fabrics.” In Polyesters and polyamides, 253–278. Woodhead Publishing. https://doi.org/10.1533/9781845694609.2.253.
- Beyene, K. A., and D. M. Kumelachew. 2022. “An Investigation of the Effects of Weave Types on Surface Roughness of Woven Fabric.” Textile Research Journal 92 (13–14): 2276–2284. https://doi.org/10.1177/00405175211010683.
- Das, A., V. K. Kothari, and N. Vandana. 2005. “A Study on Frictional Characteristics of Woven Fabrics.” AUTEX Research Journal 5 (3): 133–140. https://doi.org/10.1515/aut-2005-050303.
- Edwin, K., Y. Zeng, J. I. Mwasiagi, and S. Kyatuheire. 2013. “The Comfort Dimension; A Review of Perception in Clothing.” Journal of Sensory Studies 28 (6): 423–444. https://doi.org/10.1111/joss.12070.
- Göktepe, F., M. Halász, P. Tamás, Ö. Göktepe, J. Gersak, B. Al-Gaadi, and D. Özdemir. 2010. “Twist Direction and Yarn Type Effect on Draping Properties.” ISN 171–177. https://doi.org/10.1080/00405167.2015.1117243.
- Joy, R. U. 2021. “Measurement and Prediction of Fabric and Garment Drape.” Textile Progress. https://doi.org/10.1080/00405167.2015.1117243.
- Kamalha, E., Y. Zeng, J. Mwasiagi, and S. Kyatuheire. 2013. “The Comfort Dimension; a Review of Perception in Clothing.” Journal of Sensory Studies 28. https://doi.org/10.1111/joss.12070.
- Moorthy, R. R., and P. Kandhavadivu. 2015. “Surface Friction Characteristics of Woven Fabrics with Nonconventional Fibers and Their Blends.” Journal of Textile and Apparel, Technology and Management 9 (3). https://doi.org/10.1111/joss.12070.
- Nassif, N. A. A. 2017. “Thermal Comfort Properties of Compact and Ring Yarn Woven Fabrics.” World 3: 31.
- Özdemir, H. 2017. “Thermal Comfort Properties of Clothing Fabrics Woven with Polyester/Cotton Blend Yarns.” AUTEX Research Journal 17 (2): 135–141. https://doi.org/10.1515/aut-2016-0012.
- Özdil, N., A. T. özgüney, G. S. Mengüç, and S. Sertsöz. 2014. “Influence of Yarn and Fabric Construction Parameters on Drape and Bending Behaviour of Cotton Woven Fabrics.” Textile & Apparel 24 (2): 169–179.
- Süle, G. 2012. “Investigation of Bending and Drape Properties of Woven Fabrics and the Effects of Fabric Constructional Parameters and Warp Tension on These Properties.” Textile Research Journal 82 (8): 810–819. https://doi.org/10.1177/0040517511433152.
- Walters, A., et al. 2005. An Overview of Textiles Processing and Related Environmental Concerns. UK: Greenpeace Research Laboratories, Department of Biological Sciences, University of Exeter.
- Yan, K., H. Höcker, and K. Schäfer. 2000. “Handle of Bleached Knitted Fabric Made from Fine Yak Hair.” Textile Research Journal 70 (8): 734–738. https://doi.org/10.1177/004051750007000812.