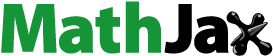
ABSTRACT
In order to achieve the goal of preparing functional textiles using tea saponin, in this work, carboxymethylated tea saponin (CTS) was used to modify cotton fabrics by an industrial rolling and baking process, and then functional textiles could be obtained after coordination with Fe3+, which could remove Cr species from water by photocatalytic reduction and adsorption processes. High CTS concentrations and higher treatment temperatures led to an increase in the carboxyl group content (QCOOH) and Fe content (QFe) of the obtained Fe-CTS-Cotton. The increase in the QFe value of the functional textiles favored the photocatalytic reduction of Cr(VI). Fe-CTS-Cotton with high QCOOH values showed excellent adsorption performance for the generated Cr(III) ions during the adsorption process. Therefore, the high photocatalytic reduction efficiency and strong Cr(III) ion trapping ability were the reasons for the recycling of Fe-CTS-cotton as a hybrid photocatalyst for enhanced removal of Cr(VI).
摘要
为了实现利用茶皂素制备功能性纺织品的目的,本工作采用羧甲基化茶皂素(CTS)对棉织物进行轧-烘-焙工业化改性,然后与Fe3+配位得到功能性纺织品,然后通过光催化还原和吸附过程完全去除水中的Cr物种. 较高的CTS浓度和处理温度使得所获得的Fe-CTS棉的羧基含量(QCOOH)和Fe含量(QFe)增加。功能性纺织品QFe值的增加有利于Cr(VI)的光催化还原. 具有高QCOOH值的Fe-CTS-棉在吸附过程中对生成的Cr(III)离子表现出优异的吸附性能. 因此,Fe-CTS-棉具有很高的光催化还原效率和很强的捕获 Cr(III) 离子的能力,这是将其作为增强去除 Cr(VI) 的光催化剂进行回收利用的原因。
Introduction
Tea saponin (TS) is extracted from tea seed which is considered as a residue after the tea seed is extracted for oil (Mao et al. Citation2010). On average, the production of 15 million tons of tea seed oil will obtain 50 million tons of residues annually in China (Feng et al. Citation2013). The basic structure of TS is sapogenin (C30H50O6) which is mainly a derivative of oleanane and links with one or more sugar moieties containing galactose, xylose, arabinose or glucuronic acid (Wang, Ye, and Liu Citation2012). Its relative molecular weight is about 1222.54 and the structure of TS is shown in (Hao et al. Citation2010). TS has been used as the most common finishing agent for foaming, emulsification, and decentralization (Yan et al. Citation2011; Yuan et al. Citation2008); moreover, additional functions of TS were found such as diminishing inflammation, easing pain and resisting epiphyte (Guo et al. Citation2008; Hayashi et al. Citation2000; Sagesaka et al. Citation1996) because of its bioactivities. In particular, several studies have reported that TS is naturally occurring triterpenoid saponins which has reducing power relating to the degree of hydroxylation in its structure (Kim et al. Citation2012; Wang, Ye, and Liu Citation2012). Modification of tea saponin would be an effective way to introduce functional groups to fulfil various demands for green finishing agent in miscellaneous industries. However, owing to its structural complication, studies on modification of tea saponin have little carried out, especially preparing environmental care material.
Chromium (Cr) has been declared group A of human carcinogen by the United States Environmental Protection Agency (USEPA) (Costa Citation2003) and introduced into aquatic systems by a number of human activities, chief of which is tanning of leather (Jacques et al. Citation2007; Wang et al. Citation2013). Other effluent sources of Cr are electroplating, textile, metal processing, paint and pigments, dyeing and steel fabrication. Trivalent (Cr(III)) and hexavalent (CrO42-, HCrO4- and Cr2O72-) species of Cr in several oxidation states (e.g., divalent, trivalent, pentavalent, and hexavalent) are primarily found in these industrial effluents. Cr(VI) is a powerful oxidizing agent, highly toxic to plants and animals, and a suspected potential carcinogen (Iftikhar et al. Citation2009). Although small amounts of Cr(III) are known to be essential for glucose metabolism in the human body, higher levels may lead to symptoms of toxicity, such as allergic skin reactions (Marín et al. Citation2009; Pan, Jiang, and Xu Citation2013). The USEPA has reported that the maximum level of Cr in drinking water should not exceed 0.1 mg/l (Lazaridis and Asouhidou Citation2003), while the World Health Organization has set an upper limit of 0.01 mg/l.
Cotton fiber is the most commonly used cellulose fiber in modern textiles industry. Cotton fiber, with annual production of more than 27 million tons, accounts for 30% of the world’s total fiber production (Wanassi, Azzouz, and Hassen Citation2016). The huge production capacity of the cotton textile industry has resulted in a higher output of waste cotton fibers, as different cotton wastes are generated from the initial stage of harvesting the cotton crop to the final stage of finished textile products. Industrial cotton waste has been considered one of the primary sources of cellulose waste that can be recycled in the last few years. There have been several attempts to re-utilize waste cotton for the production of porous carbonaceous materials (Hassan and Carr Citation2021), biofuels (Sharma, Kodgire, and Kachhwaha Citation2019) or cellulose nanocrystals and fibers (Meyabadi et al. Citation2014; Wang et al. Citation2017), among others. However, these recovery processes are normally carried out at high temperatures or require complex, costly and energy-intensive facilities.
In our earlier research, we successfully developed a novel catalytic/adsorptive bifunctional material (ethylenediaminetetraacetic acid (EDTA)-modified cotton fiber iron complexes) for the removal of Cr from water through a hydrothermal reaction and coordination principle (Dong et al. Citation2019). Inspired by this pioneering study, we speculated on a novel strategy to use TS with natural reduction capacity as an alternative to EDTA, which is expensive and hard to biodegrade (Barona, Aranguiz, and ElıElıAs Citation2001). In order to prepare functional textiles with TS, used for removing Cr species, the functional group of TS needs to be introduced efficiently, because TS lacks covalent bonding to the surface of cotton fibers, which does not allow for desirable durability of cotton fibers. In previous studies, polysaccharide derivatives were carboxymethylated in alkaline aqueous media employing monochloroacetic acid (MCA) as an etherifying agent (Silva et al. Citation2004; Wang, Zhang, and Zhao Citation2015; Xu et al. Citation2009). Hence, we considered that the TS esterified by MAC can conveniently introduce carboxyl groups through the oligosaccharide portion and form covalent bonds with cotton. The purpose of this work was to graft TS with strong natural reducing power and coordination ability onto waste cotton fabrics through continuous industrial means (pad-dry-cure process), and then subsequent form complexes with Fe ions, so that it could achieve detoxification (reducing power) of Cr(VI) in water and effectively adsorbed all Cr species. An investigation of selected important factors affecting the preparation and application of complexes for better aqueous Cr removal was performed. A discussion of the mechanisms by which aqueous Cr species may be removed by the complex is also presented.
Experimental
Materials and reagents
The pure tea saponin powder was purchased by Xi’An TongZe BiotechCo., Ltd (Xian, China). Bleached cotton commercially available fabrics (118.2 g−2) were used in this research. A solution of 2.0 g L−1 Na2CO3 and 2.0 g L−1 soap was boiled and the fabrics were treated for 30 min, after which the fabrics were thoroughly cleaned with tap water and dried at room temperature before use. K2Cr2O7, KMnO4, CH₃COOH, ClCH₂COOH (MCA), NaH2PO4·12 H2O, FeCl3·6 H2O, H2O2 (30%, w/w) were of analytical grade and used as received. Deionized water was used in whole work.
Preparation of carboxymethylated tea saponin
There are almost no related records about the carboxymethylation of tea saponin (CTS), due to the structure of tea saponin and polysaccharide is similar which contains one or more sugar chains, the carboxymethylation of tea saponin was carried out using the method about the carboxymethylation of polysaccharide with modification (Silva et al. Citation2004). One gram of tea saponin powder was added to a 25 mL sodium hydroxide solution and agitated at ambient temperature for 10 min to fully dissolve. The MAC was then thoroughly mixed with the solution and heated at a given temperature for a varying duration of time with continuous stirring. After the reaction was completed, the mixture was cooled to room temperature and the pH of the solution is adjusted to 7 using acetic acid. The resulting product was given a dialysis treatment using a semipermeable membrane (MWCO 500) to remove any chloride ion for 24-72 h and dried at 60°C. Finally, the CTS was obtained.
The degree of substitution (DS) of CTS
The total degree of substitution (DS) is the average number of functional groups introduced in the polymer, which can define the properties of the polymer after carboxymethylation. The exact determination of DS and functionalization pattern is a prerequisite for the optimization of reaction conditions as well as for understanding of structure property relationship. In this work, the DS of DTS was determined to use potentiometric titration. The dried sample (0.1 g) was firstly mixed adequately with aqueous sodium hydroxide solution (0.1 M, 10 ml) under nitrogen atmosphere and stirred for 2 h at 40°C. Finally, it was titrated with dilute aqueous hydrochloric acid solution (0.1 M). DS was then calculated by the following formula (Li, Dong, and Li Citation2015; Silva et al. Citation2004):
Where WA is the milliequivalent of acid consumed per gram of sample, C1 (mL) is the amount of NaOH solution added, V1 is the normality of the NaOH solution, C2 (mL) is the amount of HCl solution used for titration, V2 is the normality of the HCl solution, and m (g) is the CTS sample mass, 162 g/mol is the molar mass of an anhydroglucose unit (AGU), 58 g/mol is the net increase in the mass of an AGU for each carboxymethyl group substituted.
Synthesis of CTS-modified cotton fiber Fe complex
The detailed steps for modification of cotton fabrics using CTS were that: Firstly, an aqueous solution containing the various amount of CTS (5–25 gL−1) and NaH2PO4 (0–5% w/w) was prepared, and the cotton fabric was immersed in it. After 10 min, the impregnated cotton fabric was then padded with a laboratory padder (maintained a wetting rate of 75%−80%). Immediately thereafter, the samples were preliminary dried at 80°C for 5 min and 100–180°C (cure temperature) at for 1.5 min. The CTS modified cotton fabrics (CTS-Cotton) were obtained by rinsing and drying finally.
Carboxyl group content (QCOOH, mmolg−1) of CTS-Cotton sample was measured according to a titration method reported by Li et al. (Li, Dong, and Li Citation2015). Briefly, added 0.50 g of dried CTS-Cotton fragments into 50 mL of NaOH aqueous solution (100 mmolL−1) and stirred at 50°C for 2 h. A standard aqueous solution of 100 mmolL−1 HCl was then titrated with a burette to determine the amount of NaOH in the solution that had not yet reacted with the COOH group. The formula for calculating QCOOH value of CTS-Cotton was QCOOH=(V1C1 - V2C2)/m, where C1 and C2 were the molar concentrations of NaOH and HCl aqueous solution (molL−1), respectively. V1 is the volume of NaOH solution (50 ml). V2 is the volume of HCl solution consumed in titration (ml), and m is the mass of dry CTS-Cotton used (g).
The next step is to coordinate the CTS-cotton using Fe ions. The Fe complexes of CTS-cotton (referred to as Fe-CTS-Cotton) were first prepared by placing 1.0 g of CTS-Cotton into 50 mL of aqueous solutions of different concentrations of Fe(III) ions (FeCl3, 0.01 molL−1−0.2 molL−1) with continuous stirring for 3 h at 50°C, then removed for washing and drying. The concentration of Fe ions in the treatment solution was measured using a Varian Vista-MPX inductively coupled plasma optical emission spectroscopy (ICP-OES) during this process, the Fe content (QFe) on Fe-CTS-Cotton was calculated from the difference between the initial and residual concentrations of Fe ions.
Characterization methods
Cotton fabric was analyzed using SEM, FTIR, XPS and DRS techniques, respectively before and after modification and coordination. For SEM observation, a Zeiss Gemini 500 scanning electron microscope was adopted to investigate the surface structure of the samples with operation at 15 kV. For FTIR analysis, a Nicolet iS50 FT-IR spectrometer was used for testifying to their surface chemical constitution. A K-alpha X-ray photoelectron spectrometer (Thermo Fisher Scientific Inc., USA, XPS) was employed to record the core-level spectra of several elements on fibrous samples under 300 W A1 K conditions. A Hitachi UH4150 UV-vis-NIR spectrometer was used to assess the light adsorption ability of fibrous samples by obtaining their diffuse reflectance UV–Vis spectra (DRS) with Barium sulfate as a reference.
Cr species removal experiments
Firstly, a precise weight of K2Cr2O7 was put into a beaker and made to completely dissolve in 50 ml of distilled water to configure an aqueous solution with a concentration of 0.040 mmol/L of Cr6+ ions, and then 1.0 g of sheared Fe-CTS-Cotton was added into the above solution, and the system pH was adjusted by using dilute HCl or NaOH aqueous solution. Then the beaker was placed in a photo-reactor, and at regular intervals, the concentrations of Cr6+ ions and total Cr species in test solution were measured using a PinAAcle 900 H atomic absorption spectrometer (Pekin-Elmer Inc., USA). The reduction percentage (R%) of Cr6+ ions and the removal percentage of total Cr species (ƞ%) were calculated according to EquationEquations (3)(3)
(3) and (Equation4
(4)
(4) ), respectively.
where C06 and Ct6 were the initial and residual concentrations of Cr6+ ions, respectively. C0t and Ctt were the initial and residual concentrations of total Cr species, respectively during the reaction.
Results and discussion
Preparation of CTS
The amount of tea saponin was always kept at 1.0 g in the experiments, and the optimization of the carboxymethylation process was performed by varying the parameters of the process such as the dosage of sodium hydroxide and monochromatic acid, the temperature, and the duration of the reaction. Each parameter is varied while the others are kept constant as shown in .
It could be observed in that the DS value of CTS increased gradually with the increase in the amount of sodium hydroxide until it reaches 0.1 g where the DS value decreased sharply. This is due to the fact that the process of carboxymethylation of TS consists of two main reactions (Goyal, Kumar, and Sharma Citation2007). The main reaction involves sodium hydroxide first reacting with the hydroxyl group in the TS molecular structure to form an alkoxide group, which then undergoes a dimer nucleophilic substitution reaction with MAC (ClCH2COOH) to produce carboxymethyl groups. The reaction process can be represented by EquationEquations (5)(5)
(5) and (Equation6
(6)
(6) ):
A side reaction is the formation of sodium glycolate from MCA and sodium hydroxide during the reaction, which affects the degree of substitution of the carboxymethyl group on the TS molecular chain. This reaction is shown in EquationEquation (7)(7)
(7) :
At the initial stage of the reaction, EquationEquations (5)(5)
(5) –(Equation6
(6)
(6) ) were the dominant reactions, and their reaction rates were significantly greater than those of EquationEquation (7)
(7)
(7) , resulting in a growing number of carboxymethyl groups on the TS molecular chain. However, when the mass of sodium hydroxide was greater than 0.1 g, the reaction rate of EquationEquation (7)
(7)
(7) gradually increased, the MAC in the system was consumed by sodium hydroxide, and the amount of sodium glycolate increased, causing a decrease in the DS value of the CTS sample. shows that DS increases with increasing the amount of MCA at 0.1 g of NaOH and reaches an optimum at 0.119 g. At a mass of MCA more than 0.119 g, a side reaction (EquationEquation (7)
(7)
(7) ) occurred, which was responsible for the decrease in the DS value. It was found in that the DS value of CTS increases almost linearly as a result of the temperature increase, and the DS value is maximum at 60°C, which is attributed to the fact that the increase in temperature promotes the swell of CTS, which favorably affects the diffusion and adsorption of the reactants, and ultimately leads to a closer contact between the MAC and the CTS. The DS value decreased at 70–80°C, which was the most likely also the case for higher rates of side reactions hindering the carboxymethylation reaction of CTS, thus making 60°C the optimum reaction temperature. Effect of treating time on DS value is shown in . The higher the treatment time, the higher the DS value for the carboxymethylation reaction to reach equilibrium, and the DS value decreased after further increasing the treatment time beyond 3 h. The reason for the longer reaction time may be that the carboxymethylation reaction proceeds more adequately. The possible explanation for the decrease of DS value after 3 h was the degradation of the CTS produced by the reaction after an excessively long reaction time (Pushpamalar et al. Citation2006).
Photocatalyst preparation by CTS
CA-Cotton with different QCOOH was firstly prepared by treating the cotton fabrics through a dyeing and finishing traditional pad-dry-cure technique using different concentrations of CTS finishing solution and NaH₂PO₄ (1% wt) as a catalyst. It was then immersed into 0.10 mol L−1 aqueous FeCl₃ solution and allowed to carry out a coordination reaction, and the QCOOH and QFe of the resulting Fe-CTS-Cotton were determined, and the results are shown in .
shows that the increased concentration of CTS (CCTS) gave rise to an approximately linear increase in the QCOOH value of CTS-Cotton, indicating that deposition of CTS molecules on cotton fabrics was enhanced by high CCTS levels. One explanation for this could be that an increase in the amount of CTS facilitated their contact with cellulose chains and thus the formation of links between them. The possible principle is depicted in (Rowland and Brannan Citation1968; Welch Citation1988). In addition, higher levels of CCTS resulted in increased QFe values for Fe-CTS-cotton, with the highest QFe value (1.82 mmolg−1) at a CCTS level of 25 gL−1 (QCOOH = 2.51 mmolg−1 for CTS-cotton). This suggests that high levels of CCTS significantly increased the QCOOH value of the generated CTS-Cotton and preferred its coordination with Fe3+ to form complexes.
When the concentrations of CTS and NaH2PO4 were 20 gL−1 and 1% wt, respectively, the temperature elevation from 100°C to 180°C resulted in a sharp increase in the QCOOH value of CTS-Cotton, as shown in . This indicates that the modification of cotton fibers with CTS was considerably improved by high temperatures in the presence of NaH2PO4 as a catalyst. This may be due to the remarkable improvement of solubility and activation of CTS in aqueous solution at high temperatures, which catalyzed the dehydration of carboxyl groups with NaH2PO4 to form anhydrides in acidic medium, and the resulting anhydrides readily react with cotton fibers (Rowland and Brannan Citation1968; Welch Citation1988). On the other hand, high temperatures also increased the swelling and wettability of cotton fibers, which resulted in the enhancement of the reaction of the anhydride with the hydroxyl groups of the cellulose chain, generating CTS-cotton with a high QCOOH value, which immobilized more Fe3+ ions onto the cotton fibers. It is noteworthy that the presence of the high dose of NaH2PO4 also enhanced the QCOOH value of the CTS-cotton obtained in . The highest QFe values were observed for CTS-Cotton prepared in the presence of NaH2PO4 at 1% wt. A further increase in NaH2PO4 led to a decrease in the QFe value, mainly due to the excess density of carboxyl groups generated by NaH2PO4 on the surface of the fibers, thus limiting the reaction between them, as described above. exhibits the results of the CTS modification reaction on cotton fabrics in different pH environments. The QCOOH and QFe values of CTS-cotton showed a tendency to increase and then decreased with increasing pH, with both values reaching a maximum at pH 3. This indicates that lowering the pH of the finishing solution significantly promoted the modification reaction of CTS on cotton fibers, while high pH in the system limited the esterification reaction of CTS with cotton fibers. This is because too much alkalinity in the reaction system not only makes it difficult for CTS to form anhydride but also weakens the positive charge and reactivity of the carbon-positive ion on the formed anhydride, which hinders the esterification reaction of CTS with cotton fiber (Zhou and Lu Citation2012).
Surface morphology
SEM images representative of the three fabric samples in showed that the surface of the original cotton fabric was slightly smooth with many blurry lines or wrinkles on the surface, which became blurred after modification by CTS. Moreover, the coordination with Fe3+ further led to the appearance of a rough thick layer on the surface of Fe-CTS-Cotton.
Photocatalyst chemical characterization
FTIR
As could be seen from , TS had characteristic absorption peaks at 3314 cm−1, 2932 cm−1, 1722 cm−1, 1603 cm−1, 1404 cm−1, 1366 cm−1 and 1044 cm−1, which belonged to the stretching vibration of –OH, -CH2, C=O, C=C, -CH3 and C-O-C on the TS molecular chain, respectively (He et al. Citation2014). It was worth noting that the FTIR spectra of TS and CTS were very similar; however, when TS was modified by carboxymethylation, the intensity of characteristic absorption peaks at 1602 cm−1 and 1406 cm−1, which were attributed to the symmetric and antisymmetric stretching vibration of the COO− group, respectively (Xu et al. Citation2009), was significantly enhanced in the spectral lines b and c. In addition, the spectral line of TS at 1366 cm−1 was subordinated to the stretching vibration of the C-H bond on the carboxymethyl group in the TS molecular chain, and the absorption peak of CTS here is also significantly enhanced. Moreover, the spectral line c at 1602 cm−1, 1406 cm−1 and 1366 cm−1 were clearly stronger than those of spectral line b, which indicated that the carboxymethyl group (-OCH2COOH) on the MCA molecule had been successfully grafted on the TS molecular chain when the TS was modified by carboxymethylation, and the number of -OCH2COOH grafted on the TS molecular chain increased with the increased DS value of CTS, which was consistent with the changes in FTIR spectra before and after the carboxymethylation reaction of polysaccharide from cyclocarya paliurus (Z. J. Wang et al. Citation2016).
Figure 4. (A) FTIR spectra of TS and CTS with different DS, (b) FTIR spectrum of cotton, CTS-Cotton and Fe-CTS-Cotton.

From FTIR spectra in , there were some characteristic absorption bands of untreated cotton located at 3335 cm−1, 2900 cm−1, 1316–1432 cm−1 and 1030–1167 cm−1, which might be due to the stretching of OH, CH, CO and C-O-C at b-1,4 glycosidic linkages, correspondingly (Li, Dong, and Li Citation2015). It should be noted that all characteristic absorption bands of CTS were slightly intensified in the spectrum of CTS-cotton. More importantly, a new absorption peak at 1706 cm−1 appeared in the spectrum of CTS-cotton, which might originate from the characteristic absorption peaks of carboxyl and ester groups generated by the modification reaction between the CTS molecule and the cotton fiber molecular chain. This indicated that the hydroxyl group in the molecular structure of cotton fiber had been esterified with the carboxymethyl group in the molecular structure of CTS and then ester group had been formed (Gupta and Haile Citation2007). In addition, the characteristic absorption peak of the Fe-CTS-Cotton was shifted and the absorption peak at 1706 cm−1 disappeared, which means that the carboxyl group on the surface of CTS-Cotton had ligated with the Fe3+ ion to form a complex.
DRS
The DRS spectra in shows that cotton fibers had only faint absorption peaks in the UV region (200–400 nm). However, a strong absorption peak of CTS-Cotton in the UV region suggested that the light absorption properties of cotton fabrics had improved after CTS modification, which was mainly attributed to the unsaturated π–π transition of carboxylic acid groups in the macromolecular structure of CTS-Cotton, and the increase in the number of carboxymethyl groups enhanced the light absorption ability. Significantly, Fe-CTS-Cotton exhibited a much stronger light absorption than CTS-Cotton at UV and visible region, and higher QFe value further improved its light absorption. Moreover, after Fe3+ coordination, the peak at 414 nm for CTS-Cotton shifted high position, which might be the d–d transitions of Fe3+ and ligand-to-metal charge transfer (LMCT) transitions (Luo et al. Citation2017; Sun et al. Citation2011) from CTS-Cotton toward Fe3+ in the complex. Accordingly, it was expected that Fe-CTS-Cotton might be used as a solar light-driven photocatalyst.
Analysis of photocatalytic reduction process of aqueous Cr(VI)
The Fe-CTS-Cotton (QFe = 0.182 mmolg−1) was used to reduce 0.04 mmolL−1 of Cr(VI) in aqueous solution at pH 6.0 and 25°C under darkness or Hg lamp irradiation. Meanwhile, a control experiment with CTS-Cotton was also carried out, and then the reduction rate (R%) of Cr(VI) during the experimental process under different conditions was determined, as shown in .
Figure 6. Reduction efficiency of Cr(VI) with Fe-CTS-Cotton in the dark or under irradiation or under irradiation, and CTS-Cotton under irradiation.

shows that the R% value was less than 20% for 60 min under light irradiation when only CTS-Cotton was present in the system. This indicates that Cr(VI) were quite stable in the water and that CTS-Cotton had a weak reducing capacity for Cr(VI) under light irradiation. This might be attributed to the reduction of Cr(VI) by the two reducing groups (C-H and C=O) of CTS on cotton fibers, which gave CTS-Cotton a relatively stronger light absorption capacity than cotton in the UV and visible regions (as shown in ). It was noteworthy that the low R% value (12.13%) observed within 60 min under dark conditions when using Fe-CTS-Cotton may be due to the occupation of the carboxyl group position by Fe ions. Once light irradiation was introduced, the R% value increased dramatically and reached 91.53% within 60 min, indicating that the reduction of Cr(VI) using Fe-EDTA-Cotton was highly dependent on light irradiation. This was mainly due to the fact that Fe-CTS-Cotton has a stronger light absorption capacity than the corresponding CTS-Cotton. Upon light irradiation, Fe-EDTA-Cotton absorbed incident photons, which was then excited to form Fe(II) ions due to the LMCT process (Dong et al. Citation2019; Luo et al. Citation2017). The created Fe(II) ion was an effective reductant that converts Cr(VI) to Cr(III) through a series of single-electron transfer processes (Luo et al. Citation2017), while Cr(VI) re-oxidized Fe(II) to Fe(III) ions, completing the cycle of Fe(III)/Fe(II) ions. This process was represented by EquationEquations (8)(8)
(8) and (Equation9
(9)
(9) ).
Moreover, the XPS result in shows the changes in the chemical composition of the surface of Fe-CTS-Cotton before and after the experiments of Cr(VI) removal by reduction. Two weak peaks at 577.38 eV and 587.28 eV, corresponding to 2p3/2 and 2p1/2, respectively, were identified in the Cr 2p narrow scan spectrum of Fe-CTS-Cotton after the experiment, and both of them belonged to the Cr(III) ions (Jing et al. Citation2017). This further confirmed that the removal of Cr(VI) by Fe-CTS-Cotton began with the reduction of Cr(VI) to Cr(III) by the Fe complex, which was then immobilized by the carboxyl groups on the Fe-CTS-Cotton surface. Furthermore, reveals that the binding energy peaks of Fe(III) ions almost remained unchanged before and after the reaction, proposing that Fe-CTS-Cotton exhibited a better stability in the removal process of Cr(VI) species.
Optimised Cr(VI) removal process of Fe-CTS-cotton
To investigate the removal ability of Fe-CTS-cotton for Cr(VI), five samples with different QFe values were prepared by optimizing the treatment process and treating cotton with different concentrations of CTS. Subsequently, these samples were used to reduce Cr(VI) (0.04 mmolL−1) in an aqueous solution at pH 6 and a temperature of 25°C. The concentrations of Cr(VI) and total Cr species were measured over time, respectively, and the reduction of Cr(VI) (R%) and removal of total Cr species (ƞ%) during the reaction were calculated, and the results of the experiments are shown in .
shows that the R% value increased gradually with the increase of QFe value of Fe-CTS-Cotton, which was related to the fact that higher QFe could enhance its photocatalytic ability, which was beneficial to the reduction of Cr(VI). Therefore, the Fe(II)/Fe(IIII) photocatalytic cycle on CTS-Cotton enhanced the conversion of Cr(VI) to Cr(III) under light conditions. It was found from that the higher the QCOOH level of the complexes, the larger the ƞ% value, which indicated that except for the stronger reducing ability, the Cr removal ability of Fe-CTS-Cotton mainly depended on its higher QCOOH value, and with higher QCOOH value, the larger the number of remaining carboxyl groups, which enhanced the electrostatic attraction to the Cr(III) ions in the water. shows that the R% and ƞ% values of Fe-CTS-Cotton under different acidic and alkaline environments varied with the pH of the system. Lower pH led to a remarkable increase in both values, suggesting the reduction removal of Cr(VI) is significantly facilitated by Fe-CTS-Cotton in acidic media. In general, Cr(VI) species include H2CrO4, HCrO4-, and CrO42- in aqueous solution, which depended on their concentration and solution pH, whereas HCrO4− is the predominant form of H2CrO4 in the pH range of 1.0–6.8 (Deng et al. Citation2017). The adsorption of Cr(VI) species by both wool fibers and CTS in Fe-CTS-Cotton was enhanced in the acidic range, especially for CTS, where H+ ions in the acidic solution partially neutralized the negatively charged carboxyl groups in the structure of CTS, thus weakening the diffusion barriers for the Cr(VI) anion, which is mainly present in the form of dichromate (Cr2O72-), and increasing the chances of contact between Cr2O72- and Fe-CTS-Cotton. In addition, the high concentration of protons in the system also contributed to the enhanced reduction of Cr(VI), the principle of which could be formulated in EquationEquations (10)(10)
(10) and (Equation11
(11)
(11) ) (Luo et al. Citation2017; Marinho et al. Citation2016).
Although deprotonation of carboxyl groups on the surface of Fe-CTS-Cotton improved its adsorption capacity for Cr(III) at elevated pH (especially in alkaline media), the increase in pH also decreased the concentration of protons in solution, thus limiting the reduction of Cr(VI) to Cr(III). For further investigation of the effect of irradiation on Cr(VI) reduction and total Cr species removal, photocatalytic reduction of Cr(VI) was also performed on Fe-CTS-cotton (QFe = 1.58 mmolg−1) using different lamps (LED-l, LED-m, LED-h and Hg lamp) as the irradiation source, respectively. The R% and ƞ% values during the reaction were then measured and are shown in . From , it could be seen that the R% values of the Hg lamp (Containing UV light) were higher than those of the LED visible light lamp, which indicates that although the irradiation intensity of the Hg lamp is lower than that of the LED-h visible light lamp, the former could promote the reduction of Cr(VI) more effectively than the latter. This was mainly due to the fact that Fe-CTS-Cotton absorbs UV light more strongly than visible light, which accelerates the reduction of Cr(VI), as shown in . In addition, high-intensity LED visible light irradiation increased the R% and ƞ% values, suggesting that LED visible light lamps could be used to improve the reduction of Cr(VI) and the removal of total Cr. The more energy efficient of LED visible lamps compared to Hg lamps could be used as an alternative to Fe-CTS-Cotton mediated Cr(VI) reduction by increasing the irradiation intensity of LED lamps.
Cr (VI) removal kinetics
The pseudo-second-order kinetic model was adopted to evaluate the data in . The linear form of pseudo-second-order kinetic model was described as:
Where k was the rate constant of the pseudo-second-order model for Cr(VI) reduction (kR) or Cr(III) adsorption (kƞ) by Fe-CTS-Cotton. qe and qt were the amounts of Cr(VI) reduction or Cr(III) adsorption per unit mass of Fe-CTS-Cotton at steady state and any time t, respectively. The slope and intercept of the linear plot of t/qt against t yielded the values of qe and k values. The regression results of by EquationEquation (12)(12)
(12) for the Cr(VI) reduction or Cr(III) adsorption by Fe-CTS-Cotton are summarized in .
Table 1. Cr(VI) reduction and Cr(III) adsorption pseudo-second-order kinetic model parameters for Fe-CTS-Cotton.
As seen from and , the correlation coefficients (r2) values for the second-order kinetic models at Cr(VI) reduction and Cr(III) adsorption were >0.98 in case of Fe-CTS-Cotton. Moreover, the theoretical amounts of Cr(VI) reduction and Cr(III) adsorption at equilibrium were only slightly different from the experimental values () in the case of pseudo-second order kinetic. These results confirmed that the entire removal process followed a pseudo-second-order model for Fe-CTS-Cotton. It was obvious that qeR and qeƞ values increased gradually with the increase of QFe, which implied that the Fe ions on cotton favored the reductive removal of Cr ions. In addition, the kR value showed an increase with increasing QFe value, whereas the opposite was true for the kƞ value, suggesting that the presence of Fe ions on cotton may affect the adsorption rate of Cr(VI) after its reduction to Cr(III).
Recycling capacity study
The used Fe-CTS-Cotton was taken out from the solution after reaction, and it was then reused by water washing and drying ().
Comparing the R% and η% curves of Cr(VI) in first run and four successive runs, slight decrease in R% and η% curves was found in five successive runs. This proposed that after reuse for five times, Fe-CTS-Cotton still showed reusability as a photocatalyst for enhancing the reduction removal of Cr species.
Comparison with other Cr(VI) removal materials
A traditional photocatalytic material (Carbon dots coupled TiO2 (CD/TiO2) (Zhang et al. Citation2018)) and both biological materials (pineapple leaves (PL723) (Ponou et al. Citation2011) and apple peels (ACAP) (Enniya, Rghioui, and Jourani Citation2018)) were selected for comparison with Fe-CTS-Cotton, their Cr(VI) removal capacities are presented in .
Table 2. Comparison of Fe-CTS-Cotton with several Cr(Vl) removal materials.
It is seen from that R% and η% values of Fe-CTS-Cotton were slightly higher than the corresponding values of CD/TiO2, PL723 or ACAP. Importantly, Fe-CTS-Cotton could be used in neutral environments, expanding the pH ranges of Cr(VI) removal materials. Additionally, both biological materials were prepared by using electric furnace at high temperature, thus increasing production cost. Another issue was other three materials difficult separation and recovery after Cr(VI) removal process because they were applied in the form of power. On the basis of the above results, it could be summarized that Fe-CTS-Cotton was the comparatively desirable Cr(VI) removal materials because of their several advantages such as low cost, high effectiveness, environmental friendliness and better reuse performance.
Conclusions
Fe-CTS-Cotton as a photocatalyst with functional roles could be prepared by the surface grafting of cotton fiber with carboxymethyl tea saponin and subsequent coordination of Fe(III) ions. The as-prepared Fe-CTS-Cotton could effectively remove the Cr species through the photocatalytic reduction of Cr(VI) species to Cr(III) ions and subsequent adsorption of the generated Cr(III) ions on its surface, its reduced rate of Cr(VI) is 99.9% (80 min) and adsorption removal rate of Cr(III) is 96.25% (180 min). In this process, increasing the Fe content of the photocatalyst or stronger irradiation favored the photocatalytic reduction removal of Cr species. After reuse for many times, Fe-CTS-Cotton still showed favorable reusability. All experiments in this article were conducted in triplicate with well-reproducible results, there ported data was obtained by averaging the values of three replicated experiments, this indicated that Fe-CTS-Cotton would have a great potential in the industrial-scale treatment of Cr(VI)-bearing toxic waste waters.
Acknowledgments
This research was supported in part by the Jiangsu Province Industry-University-Research Cooperation Project (BY2022864), in part by the Jiangsu Advanced Textile Engineering Technology Center Funds (XJFZ/2022/1 and XJFZ/2022/3), in part by the Nantong Basic Science Research Program (MSZ2022162, JC22022049 and JCZ2022109), in part by the Research Project of Jiangsu College of Engineering and Technology (GYKY/2023/6, GYKY/2022/4, GYJY202303 and GYJY202307), in part by Natural Science Foundation of the Jiangsu Higher Education Institutions of China (23KJB540002) and in part by Science and Technology Project of China National Textile And Apparel Council (2022030).
Disclosure statement
No potential conflict of interest was reported by the author(s).
Additional information
Funding
References
- Barona, A., I. Aranguiz, and A. ElıElıAs. 2001. “Metal Associations in Soils Before and After EDTA Extractive Decontamination: Implications for the Effectiveness of Further Clean-Up Procedures.” Environmental Pollution 113 (1): 79–18. https://doi.org/10.1016/S0269-7491(00)00158-5.
- Costa, M. 2003. “Potential Hazards of Hexavalent Chromate in Our Drinking Water.” Toxicology and Applied Pharmacology 188 (1): 1–5. https://doi.org/10.1016/S0041-008X(03)00011-5.
- Deng, L., Z. Shi, L. Wang, and S. Zhou. 2017. “Fabrication of a Novel NiFe2o4/zn-Al Layered Double Hydroxide Intercalated with EDTA Composite and Its Adsorption Behavior for Cr (VI) from Aqueous Solution.” Journal of Physics and Chemistry of Solids 104:79–90. https://doi.org/10.1016/j.jpcs.2016.12.030.
- Dong, Y., P. Wang, L. Gan, B. Li, and H. Wen. 2019. “Enhanced Removal of Aqueous Cr (VI) Using Optimized Fe Complex on EDTA Modified Cotton Fiber via Photocatalytic Reduction and Adsorption Hybrid Functions.” Cellulose 26 (12): 7383–7397. https://doi.org/10.1007/s10570-019-02598-4.
- Enniya, I., L. Rghioui, and A. Jourani. 2018. “Adsorption of Hexavalent Chromium in Aqueous Solution on Activated Carbon Prepared from Apple Peels.” Sustainable Chemistry and Pharmacy 7:9–16. https://doi.org/10.1016/j.scp.2017.11.003.
- Feng, Y., J. Jiang, L. Zhu, L. Yue, J. Zhang, and S. Han. 2013. “Effects of Tea Saponin on Glucan Conversion and Bonding Behaviour of Cellulolytic Enzymes During Enzymatic Hydrolysis of Corncob Residue with High Lignin Content.” Biotechnology for Biofuels 6 (1): 1–8. https://doi.org/10.1186/1754-6834-6-161.
- Goyal, P., V. Kumar, and P. Sharma. 2007. “Carboxymethylation of Tamarind Kernel Powder.” Carbohydrate Polymers 69 (2): 251–255. https://doi.org/10.1016/j.carbpol.2006.10.001.
- Guo, Y. Q., J. X. Liu, Y. Lu, W. Y. Zhu, S. E. Denman, and C. S. McSweeney. 2008. “Effect of Tea Saponin on Methanogenesis, Microbial Community Structure and Expression of mcrA Gene, in Cultures of Rumen Micro‐Organisms.” Letters in Applied Microbiology 47 (5): 421–426. https://doi.org/10.1111/j.1472-765X.2008.02459.x.
- Gupta, D., and A. Haile. 2007. “Multifunctional Properties of Cotton Fabric Treated with Chitosan and Carboxymethyl Chitosan.” Carbohydrate Polymers 69 (1): 164–171. https://doi.org/10.1016/j.carbpol.2006.09.023.
- Hao, W., G. Zhong, M. Hu, J. Luo, Q. Weng, and M. Rizwan-Ul-Haq. 2010. “Control of Citrus Postharvest Green and Blue Mold and Sour Rot by Tea Saponin Combined with Imazalil and Prochloraz.” Postharvest Biology and Technology 56 (1): 39–43. https://doi.org/10.1016/j.postharvbio.2009.10.003.
- Hassan, M. M., and C. M. Carr. 2021. “Biomass-Derived Porous Carbonaceous Materials and Their Composites As Adsorbents for Cationic and Anionic Dyes: A Review.” Chemosphere 265:129087. https://doi.org/10.1016/j.chemosphere.2020.129087.
- Hayashi, K., Y. M. Sagesaka, T. Suzuki, and Y. Suzuki. 2000. “Inactivation of Human Type a and B Influenza Viruses by Tea-Seed Saponins.” Bioscience, Biotechnology, and Biochemistry 64 (1): 184–186. https://doi.org/10.1271/bbb.64.184.
- He, J., Z. Y. Wu, S. Zhang, Y. Zhou, F. Zhao, Z. Q. Peng, and Z. W. Hu. 2014. “Optimization of Microwave‐Assisted Extraction of Tea Saponin and Its Application on Cleaning of Historic Silks.” Journal of Surfactants and Detergents 17 (5): 919–928. https://doi.org/10.1007/s11743-013-1523-8.
- Iftikhar, A. R., H. N. Bhatti, M. A. Hanif, and R. Nadeem. 2009. “Kinetic and Thermodynamic Aspects of Cu (II) and Cr (III) Removal from Aqueous Solutions Using Rose Waste Biomass.” Journal of Hazardous Materials 161 (2–3): 941–947. https://doi.org/10.1016/j.jhazmat.2008.04.040.
- Jacques, R. A., E. C. Lima, S. L. Dias, A. C. Mazzocato, and F. A. Pavan. 2007. “Yellow Passion-Fruit Shell As Biosorbent to Remove Cr (III) and Pb (II) from Aqueous Solution.” Separation and Purification Technology 57 (1): 193–198. https://doi.org/10.1016/j.seppur.2007.01.018.
- Jing, F., R. Liang, J. Xiong, R. Chen, S. Zhang, Y. Li, and L. Wu. 2017. “MIL-68 (Fe) as an Efficient Visible-Light-Driven Photocatalyst for the Treatment of a Simulated Waste-Water Contain Cr (VI) and Malachite Green.” Applied Catalysis B: Environmental 206:9–15. https://doi.org/10.1016/j.apcatb.2016.12.070.
- Kim, Y. A., C. S. Kong, J. Im Lee, H. Kim, H. Y. Park, H. S. Lee, and Y. Seo. 2012. “Evaluation of Novel Antioxidant Triterpenoid Saponins from the Halophyte Salicornia Herbacea.” Bioorganic and Medicinal Chemistry Letters 22 (13): 4318–4322. https://doi.org/10.1016/j.bmcl.2012.05.017.
- Lazaridis, N. K., and D. Asouhidou. 2003. “Kinetics of Sorptive Removal of Chromium (VI) from Aqueous Solutions by Calcined Mg-Al-CO3 Hydrotalcite.” Water Research 37 (12): 2875–2882. https://doi.org/10.1016/S0043-1354(03)00119-2.
- Li, B., Y. Dong, and L. Li. 2015. “Preparation and Catalytic Performance of Fe (III)-Citric Acid-Modified Cotton Fiber Complex As a Novel Cellulose Fiber-Supported Heterogeneous Photo-Fenton Catalyst.” Cellulose 22 (2): 1295–1309. https://doi.org/10.1007/s10570-015-0562-x.
- Luo, Z., J. Wang, L. Qu, J. Jia, S. Jiang, X. Zhou, and Z. Wu. 2017. “Visible-Light-Driven Photocatalytic Reduction of Cr (VI) on Magnetite/carboxylate-Rich Carbon Sheets.” New Journal of Chemistry 41 (21): 12596–12603. https://doi.org/10.1039/C7NJ01800B.
- Mao, H. L., J. K. Wang, Y. Y. Zhou, and J. X. Liu. 2010. “Effects of Addition of Tea Saponins and Soybean Oil on Methane Production, Fermentation and Microbial Population in the Rumen of Growing Lambs.” Livestock Science 129 (1–3): 56–62. https://doi.org/10.1016/j.livsci.2009.12.011.
- Marín, A. P., M. I. Aguilar, V. F. Meseguer, J. F. Ortuno, J. Sáez, and M. Lloréns. 2009. “Biosorption of Chromium (III) by Orange (Citrus Cinensis) Waste: Batch and Continuous Studies.” Chemical Engineering Journal 155 (1–2): 199–206. https://doi.org/10.1016/j.cej.2009.07.034.
- Marinho, B. A., R. O. Cristovao, J. M. Loureiro, R. A. Boaventura, and V. J. Vilar. 2016. “Solar Photocatalytic Reduction of Cr (VI) Over Fe (III) in the Presence of Organic Sacrificial Agents.” Applied Catalysis B: Environmental 192:208–219. https://doi.org/10.1016/j.apcatb.2016.03.061.
- Meyabadi, T. F., F. Dadashian, G. M. M. Sadeghi, and H. E. Z. Asl. 2014. “Spherical Cellulose Nanoparticles Preparation from Waste Cotton Using a Green Method.” Powder Technology 261:232–240. https://doi.org/10.1016/j.powtec.2014.04.039.
- Pan, J., J. Jiang, and R. Xu. 2013. “Adsorption of Cr (III) from Acidic Solutions by Crop Straw Derived Biochars.” Journal of Environmental Sciences 25 (10): 1957–1965. https://doi.org/10.1016/S1001-0742(12)60305-2.
- Ponou, J., J. Kim, L. P. Wang, G. Dodbiba, and T. Fujita. 2011. “Sorption of Cr (VI) Anions in Aqueous Solution Using Carbonized or Dried Pineapple Leaves.” Chemical Engineering Journal 172 (2–3): 906–913. https://doi.org/10.1016/j.cej.2011.06.081.
- Pushpamalar, V., S. J. Langford, M. Ahmad, and Y. Y. Lim. 2006. “Optimization of Reaction Conditions for Preparing Carboxymethyl Cellulose from Sago Waste.” Carbohydrate polymers 64 (2): 312–318. https://doi.org/10.1016/j.carbpol.2005.12.003.
- Rowland, S. P., and M. A. F. Brannan. 1968. “Mobile Ester Cross Links for Thermal Creasing of Wrinkle-Resistant Cotton Fabrics.” Textile Research Journal 38 (6): 634–643. https://doi.org/10.1177/004051756803800611.
- Sagesaka, Y. M., T. Uemura, Y. Suzuki, T. Sugiura, M. Yoshida, K. Yamaguchi, and K. Kyuki. 1996. “Antimicrobial and Anti-Inflammatory Actions of Tea-Leaf Saponin.” Yakugaku Zasshi: Journal of the Pharmaceutical Society of Japan 116 (3): 238–243. https://doi.org/10.1248/yakushi1947.116.3_238.
- Sharma, A., P. Kodgire, and S. S. Kachhwaha. 2019. “Biodiesel Production from Waste Cotton-Seed Cooking Oil Using Microwave-Assisted Transesterification: Optimization and Kinetic Modeling.” Renewable and Sustainable Energy Reviews 116:109394. https://doi.org/10.1016/j.rser.2019.109394.
- Silva, D. A., R. C. de Paula, J. P. Feitosa, A. C. de Brito, J. S. Maciel, and H. C. Paula. 2004. “Carboxymethylation of Cashew Tree Exudate Polysaccharide.” Carbohydrate Polymers 58 (2): 163–171. https://doi.org/10.1016/j.carbpol.2004.06.034.
- Sun, C., C. Chen, W. Ma, and J. Zhao. 2011. “Photodegradation of Organic Pollutants Catalyzed by Iron Species Under Visible Light Irradiation.” Physical Chemistry Chemical Physics 13 (6): 1957–1969. https://doi.org/10.1039/C0CP01203C.
- Wanassi, B., B. Azzouz, and M. B. Hassen. 2016. “Value-Added Waste Cotton Yarn: Optimization of Recycling Process and Spinning of Reclaimed Fibers.” Industrial Crops and Products 87:27–32. https://doi.org/10.1016/j.indcrop.2016.04.020.
- Wang, W., X. Wang, X. Wang, L. Yang, Z. Wu, S. Xia, and J. Zhao. 2013. “Cr (VI) Removal from Aqueous Solution with Bamboo Charcoal Chemically Modified by Iron and Cobalt with the Assistance of Microwave.” Journal of Environmental Sciences 25 (9): 1726–1735. https://doi.org/10.1016/S1001-0742(12)60247-2.
- Wang, Z. J., J. H. Xie, M. Y. Shen, W. Tang, H. Wang, S. P. Nie, and M. Y. Xie. 2016. “Carboxymethylation of Polysaccharide from Cyclocarya Paliurus and Their Characterization and Antioxidant Properties Evaluation.” Carbohydrate Polymers 136:988–994. https://doi.org/10.1016/j.carbpol.2015.10.017.
- Wang, Z., Z. Yao, J. Zhou, and Y. Zhang. 2017. “Reuse of Waste Cotton Cloth for the Extraction of Cellulose Nanocrystals.” Carbohydrate Polymers 157:945–952. https://doi.org/10.1016/j.carbpol.2016.10.044.
- Wang, J. K., J. A. Ye, and J. X. Liu. 2012. “Effects of Tea Saponins on Rumen Microbiota, Rumen Fermentation, Methane Production and Growth Performance—A Review.” Tropical Animal Health and Production 44 (4): 697–706. https://doi.org/10.1007/s11250-011-9960-8.
- Wang, X., Z. Zhang, and M. Zhao. 2015. “Carboxymethylation of Polysaccharides from Tremella Fuciformis for Antioxidant and Moisture-Preserving Activities.” International Journal of Biological Macromolecules 72:526–530. https://doi.org/10.1016/j.ijbiomac.2014.08.045.
- Welch, C. M. 1988. “Tetracarboxylic Acids as Formaldehyde-Free Durable Press Finishing Agents: Part I: Catalyst, Additive, and Durability Studies.” Textile Research Journal 58 (8): 480–486. https://doi.org/10.1177/004051758805800809.
- Xu, J., W. Liu, W. Yao, X. Pang, D. Yin, and X. Gao. 2009. “Carboxymethylation of a Polysaccharide Extracted from Ganoderma Lucidum Enhances Its Antioxidant Activities in vitro.” Carbohydrate Polymers 78 (2): 227–234. https://doi.org/10.1016/j.carbpol.2009.03.028.
- Yan, J., Z. Wu, Y. Zhao, and C. Jiang. 2011. “Separation of Tea Saponin by Two-Stage Foam Fractionation.” Separation and Purification Technology 80 (2): 300–305. https://doi.org/10.1016/j.seppur.2011.05.010.
- Yuan, X. Z., Y. T. Meng, G. M. Zeng, Y. Y. Fang, and J. G. Shi. 2008. “Evaluation of Tea-Derived Biosurfactant on Removing Heavy Metal Ions from Dilute Wastewater by Ion Flotation.” Colloids and Surfaces A: Physicochemical and Engineering Aspects 317 (1–3): 256–261. https://doi.org/10.1016/j.colsurfa.2007.10.024.
- Zhang, Y., M. Xu, H. Li, H. Ge, and Z. Bian. 2018. “The Enhanced Photoreduction of Cr (VI) to Cr (III) Using Carbon Dots Coupled TiO2 Mesocrystals.” Applied Catalysis B: Environmental 226:213–219. https://doi.org/10.1016/j.apcatb.2017.12.053.
- Zhou, T., and D. Lu. 2012. “Study on Anti-Crease and Flame Retardant Finish of Cotton Fabrics with Phosphino Polycaboxylic Acid.” China Text Leader 10:82–85. https://doi.org/10.3969/j.issn.1003-3025.2012.10.023.