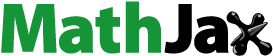
ABSTRACT
In this research work, we explore antibacterial activity and the UV-protection of cotton fabric functionalized with copper oxide-titanium dioxide nano composites particles (CuO+TiO2 NCPs). Cotton fabrics (Cot) were treated with L-methionine (Am) and functionalized with bimetallic CuO+TiO2 NCPs by pad and rolling technique. Cu(NO3)2 and titanium isopropoxide (TIP) were used as sources of Cu(OH)2 and TiO2 colloid respectively, which were then converted into CuO and TiO2 nanoparticles (NPs) for making antibacterial and UV-protective cotton. The method yielded a crystallite size of CuO and TiO2 NPs of 18.09 and 32.5 nm, respectively. FTIR, Raman spectroscopy, High-resolution scanning electron microscopy-Energy dispersive Spectroscopy (HRSEM), (EDS), X-ray photo spectroscopy (XPS), thermal gravimetric analysis (TGA), and XRD were used to analyze the functional group, morphology, chemical composition, thermal stability and crystalline structure of the functionalized cotton fabric samples. The results showed that an esterification reaction occurred between Am and OH in cotton fabric, which further enhanced the binding of NCPs. AmCot-CuO+TiO2 NCPs exhibit outstanding antibacterial activity against gram-positive S. aureus and gram-negative E. coli. The UV protection factor (UPF) for AmCot-CuO+TiO2 NCPs reached 50+; thus, multifunctional cotton fabrics with excellent antibacterial and anti-ultraviolet properties were obtained.
摘要
在这项研究工作中,我们探索了用氧化铜-二氧化钛纳米复合颗粒(CuO+TiO2-NCPs)功能化的棉布的抗菌活性和紫外线防护. 用L-甲硫氨酸(Am)对棉织物(Cot)进行处理,并用双金属CuO+TiO2-NCPs对其进行功能化处理. 分别以Cu(NO3)2和异丙氧基钛(TIP)作为Cu(OH)2和TiO2胶体的来源,将其转化为CuO和TiO2纳米粒子(NP),制备抗菌和防紫外线棉. 该方法产生的CuO和TiO2 NP的晶粒尺寸分别为18.09和32.5 nm. 采用傅立叶变换红外光谱、拉曼光谱、高分辨率扫描电子显微镜、能谱仪、X射线光电子能谱仪(XPS)、热重分析(TGA)和X射线衍射仪对功能化棉织物样品的官能团、形态、化学组成、热稳定性和晶体结构进行了分析. 结果表明,在棉织物中,Am和OH发生了酯化反应,进一步增强了NCPs的结合. AmCot-CuO+TiO2-NCPs对革兰氏阳性金黄色葡萄球菌和革兰氏阴性大肠杆菌表现出突出的抗菌活性. AmCot-CuO+TiO2-NCPs的紫外线防护因子(UPF)达到50+;从而制备出具有优异抗菌和抗紫外线性能的多功能棉织物.
Introduction
Infection caused by pathogens is one of the leading causes of fatalities in medical facilities across the globe (Murray et al. Citation2022). Infections caused by bacteria in general, and particularly in health care facilities, have prompted substantial research and industrial endeavors to develop antibacterial fabrics (Barman et al. Citation2022). Researchers identified Escherichia coli and P. aeruginosa as common nosocomial bacteria, while Staphylococcus aureus and Enterococci are gram-positive strains (Novais et al. Citation2018). Despite public awareness, there’s a growing demand for antibacterial materials in various industries, including medical devices (Wei et al. Citation2021; Li et al. Citation2017). Nanotechnology is being explored as a sustainable and eco-friendly alternative to traditional antimicrobial treatments due to growing resistance to microorganisms (Salem Citation2022). Textiles made of cotton fabric are widely utilized in industries and households (Agrawal et al. Citation2020). Cotton fabrics are ideal for microbe growth due to their temperature, moisture, and nutrients, causing negative health effects in humans due to their growth and reproduction (Xu et al. Citation2018). Efforts are being made to enhance the performance and market share of finished cotton fabric by adding additional functions like antibacterial properties (El-Naggar et al. Citation2021; Xu et al. Citation2018), UV protection (El-Naggar et al. Citation2016; Rehan et al. Citation2017), fire retardant (Rehman et al. Citation2021), and photocatalytic (Rezaie et al. Citation2018). The multi-functionalization of cotton fabric with nanoparticles (NPs) has gained significant attention, allowing for the imparting of multiple functions within the 1–100 nm size range (Jeevanandam et al. Citation2018). NPs with unique chemical, optical, and electrical properties due to their small size and extensive surface area hold immense potential in biomedical, energy, sensing, and photocatalytic applications. Various metal and metal oxide NPs, including TiO2 (El-Naggar et al. Citation2016), ZnO (Khosravian et al. Citation2015), Ag (Xu et al. Citation2018; Xu et al. Citation2019), and CuO (El-Nahhal et al. Citation2018), are extensively applied in the functionalization of cotton fabrics. NPs need to be tightly bonded to fabric surfaces for durable, multifunctional fabrics. Amino acid treatment can achieve wash-fast immobilization, reducing toxicity and stabilizing NPs against laundering. L-cysteine and L-methionine can also be used for textile substrate modification. For instance, L-cysteine grafted on the surface of cotton fabric resulted in long-lasting antimicrobial cotton textiles functionalized with silver nanoparticles (Xu et al. Citation2019). L-methionine was utilized for surface modification of cotton to bind Ag NPs generated by the in-situ method (Zhou et al. Citation2018). The report revealed that the adhesion of Ag NPs was highly achieved due to the formation of a coordination bond between the NPs and the amine and ether thiol groups on the surface of the fabric. Research highlights TiO2 and CuO NPs’ catalytic capabilities, anti-bacterial activity, and UV protection. Under UV light, nano-TiO2 produces hydrogen-oxygen-free radicals, killing microbes (Verma et al. Citation2022). The cotton fabric treated with TiO2 NPs shows excellent photocatalytic and antimicrobial properties and increased UV protection (Ibrahim et al. Citation2018). The synthesis of TiO2 NPs on cotton fabric resulted in UV hindrance properties. NanoCuO, a nontoxic and biocompatible modifier for cotton fabrics, exhibits good antibacterial efficiency and cost-effectiveness (El-Nahhal et al. Citation2021). In situ synthesized CuO NPs inhibit microbe growth, preventing delayed wound healing in humans (Parvathiraja and Shailajha Citation2021). CuO+TiO2 nanocomposite functionalized fabrics are biocompatible and have an advantage over Ag NPs in terms of functionalizing cotton fabric. Another advantage of the textiles functionalized by CuO+TiO2 NCPs is the affordability of CuO and TiO2 NPs in comparison to the more expensive Ag NPs. It is anticipated that these functionalized textiles will significantly lower nosocomial infections in healthcare and residential settings. To the best of the authors’ knowledge, no research has been conducted on the binding of CuO+TiO2 NCPs onto cotton fabrics modified with L-methionine as the binder. As a result, this paper describes the in-situ synthesis of CuO+TiO2 NCPs onto cotton textiles modified by the esterification of L-methionine and OH in cotton cellulose. The L-methionine-modified fabrics functionalized with the nanostructures were characterized by FTIR, micro-Raman spectroscopy, XRD, XPS, HRSEM-EDS, and TGA to identify the functional groups, crystallinity, size, and shape. The optical properties of functionalized and unfunctionalized cotton fabrics were evaluated by UV-DRS. The qualitative antibacterial activity, and UV-protection were assessed for the immobilization efficiency and performance of the nanostructures on the fabrics. The coating effect on the color of the fabrics and the mechanical properties of the functionalized cotton fabric were determined. Physical properties such as water absorption and vapor permeability rate were evaluated for any change in fabric nature due to modification and functionalization.
Materials and methods
Titanium isopropoxide (TIP), (Ti[OCH(CH3)2]4, 99.5% purity), L-methionine (C5H11NO2S, 98% purity) were purchased from Sisco Research Laboratories Pvt. Ltd., India. Copper nitrate trihydrate (Cu(NO3).3 H2O, 99.5% purity) was purchased from Guangdong Guanghua Sci-Tech Co Ltd, China. Hydrogen peroxide (H2O2, 35% purity) and ethanol (CH3CH2OH, purity 97%) were purchased from Sigma Aldrich. Sodium hydroxide (NaOH, 99.99% purity), Diamine tetra acetic acid (EDTA, 99% purity), Ammonia solution (NH3, 95% purity), magnesium sulfate (MgSO4, 99% purity), sodium carbonate (Na2CO3, 99.9% purity), glacial acetic acid (CH3COOH, 99.9% purity) and hydro chloric acid (HCl, 38% purity) were purchased from Loba Chemie Pvt. Ltd., India. The purchased chemicals were obtained in analytical grade and used without further purification. Cotton fabric-based textile produced by the cottage handloom sector was procured from the local market of Adama, Ethiopia and liquid detergent was purchased from Bekas Chemicals Plc, Adama, Ethiopia
Cleaning of cotton fabric
Cotton fabric was cleaned of impurities like pectin and wax using scouring agents like NaOH, Na2CO3, MgSO4, and EDTA, and then bleached with H2O2. The bleached fabric heated for a 45-minute drying process at 80°C, exposing the cellulose surface for esterification with L-methionine, and was coded as Cot.
Surface modification by L-methionine
The surface of a 30 mm × 30 mm cotton fabric was modified by immersing it in a 1.5% w/w L-methionine solution, rolling up to 80–90% weight pickup, and cured in an oven at 180 for 5 min for thermal fixation. AmCot was then assigned to the amine-modified fabric.
In-situ synthesis and deposition of AmCot-CuO+TiO2
A 5% TiO2 colloid solution was prepared by using TIP as a precursor in a beaker with deionized water, glacial acetic acid (15 mL), 37% HCl (4.2 mL), and TIP (15 mL), then heated at 60°C and stirred for 2 hours. CuO+TiO2 NCPs modified cotton fabric samples were prepared by placing 30 mm × 30 mm of AmCot into a mixture of 60 mL of 5% TiO2 colloid and 20 mL of 0.05 M Cu(NO3). H2O solution in an Erlenmeyer flask, then 10 mL of 25% NH3 solution was added dropwise into the mixture to adjust the pH (9) under stirring for 30 min, followed by 10 mL of 0.01 M NaOH to allow precipitation of Cu(OH)2 and TiO2 colloid. The solution mixture was left for 15 min. The fabric samples were washed with deionized water, heated at 75 for 4 h and cured at 180°C for 5 min. The treated fabric was coded as AmCot-CuO+TiO2.
Characterization
FTIR measurements were performed on (FTIR spectrometer Alpha-T, Bruker, Germany), transmission mode spectra 216 scan 4 cm−1 resolution were collected with a ZnSe ATR accessories having diamond crystal fixed at incident angle of 45. XRD analysis was performed using an X-ray diffractometer (XRD-7000 X-ray Diffractometer Shimadzu, Japan) using Cu k-alpha radiation (k = 1.54060 Å), steps at a scanning speed of 2θ 3°/min, an applied voltage of 40 kV and current of 30 mA in the 2θ range 10°–80° at room temperature. FEI Quanta FEG 250 SEM with EDX, SEM micrographs, and energy-dispersive spectroscopy, Thermo Fisher) were used to analyze the shape and chemical content. XPS analysis of the functionalized cotton fabrics with NCPs were performed by an AXIS multifunctional X-ray photoelectron spectrometer (Kratos Axis Ultra DLD, UK). Color analyses were conducted using a spectrophotometer with pulsed xenon lamps, minimizing specular and UV effects, and computing CIE L*, a*, b*, and K/S values. Tensile strength and elongation at break were measured using an ASTM D5034 tester, with samples conditioned for 24 hours. Thermal analysis was conducted using a TGA instrument [BJ HENVEN (ATAT 2012)] at a heating rate of 15 ◦C/min from 25 ◦C to 800°C, in a nitrogen atmosphere (flow rate of 100 mL/min). The cotton fabric sample’s water absorption was determined using the ASTM E-96 test method, and the vapor transmission rate was calculated using BS 3449: 1990. The vapor transmission rate was calculated by measuring the weight loss of water from the cup over 24 h (EquationEquation 1
(1)
(1) )
Where T is the vapor transmission rate (g/m2/d), mo and ma are the original weight of test assembly and the test assembly mass remained after 24 h, respectively, and r is the inside radius of the cup.
Antibacterial activity study
Mueller Hinton Agar AATCC 147 method was followed in conducting the qualitative test (MHA, OXOID CM0337). After the agar medium was sterilized, it was added to Petri plates and let to harden. Freshly grown broth containing 1 × 108 CFU ml−1, colony-forming units of gram-positive Staphylococcus aureus and gram-negative E. coli bacteria was streaked (about 6 cm) on the media surface and incubated for 12 hours at 37°C. After a 24-hour incubation period at 37°C, the UV-sterilized AmCot-CuO+TiO2 Petri plates were inspected for the presence of an inhibitory zone. After 24 hours of incubation, the growth of both bacterial species is determined using AmCot as the control sample.
Antibacterial laundering durability
The antibacterial activity of AmCot and AmCot-CuO+TiO2 was assessed for wash cycle durability against gram-negative E. coli and gram-positive S. aureus bacteria species according to the AATCC 100 2021 test method after every 10 wash cycles up to a total of 50 wash cycles. Each wash cycle was performed in a 5 g/L aqueous solution of liquid detergent at 45°C for 15 minutes and 150 rpm and dried at room temperature.
UV-Protection analysis
The optical properties of AmCot and Am-Cot-CuO+TiO2 samples were measured using a UV-vis DRS absorption spectrophotometer. The UV shielding character was evaluated using the ultraviolet protection factor (UPF), using the AS-NZS 4399:1996 method.
Where,
Sλ - is the spectral irradiation of the skin,
Eλ – is the erythemal spectral effectiveness,
Tλ - is spectral transmittance of the materials and Δλ is related to increment wavelength in (nm)
Result and discussion
ATR-FTIR spectroscopy
The ATR-FTIR spectra of Cot, AmCot and AmCot-CuO+TiO2 samples are shown in . The AmCot spectra show a significant signal at 3334 cm−1, indicating hydroxyl groups straining for intermolecular hydrogen bonding in cellulose and water (Yuce et al. Citation2022). The peak at 2918 cm−1 is attributed to the stretching vibration of C-H in cellulose (Agrawal et al. Citation2019), whereas the band at 1623 cm−1 is caused by water in the fibers (Cheng et al. Citation2018). The bands at 1431 and 1326 cm−1 (C-H deformation stretch vibrations and C-H in plane bending vibrations, respectively) (Markovic et al. Citation2018).
The polysaccharide in cellulose’s (CO) and (OH) stretching vibrations are linked to high peak vibrations at 1029 cm−1 while -glycosidic connections are evident at 897 cm−1 (Dave et al. Citation2014). The spectra of AmCot-CuO+TiO2 show identical characteristic peaks with minor changes, with a diminished hydroxyl group spectra suggesting -OH groups aid in CuO-TiO2 NCP deposition. New bands at 1732 cm−1 and 1567 cm−1 are observed in AmCot and AmCot-CuO+TiO2 due to asymmetric stretching of COOH, COOR, and -NH2 groups post-Am treatment which indicate that esterification reaction occurred between the hydroxyl groups of Cot and COOH groups of Am (Xu et al. Citation2018). The strong peaks at 510 and 432 cm−1 in the spectra of AmCot-CuO+TiO2 ascribed to metal-oxygen (Cu-O and O-Ti-O) vibrational modes respectively (Khashan et al. Citation2021).
Micro-raman spectroscopy
The micro-Raman spectra of AmCot and AmCot-CuO+TiO2, are shown in . The performance and durability of a modified cotton surface are significantly influenced by the chemistry at the interface of cellulose chains and the adhesion of NPs. The characteristic bands at 378 cm−1 and 460 cm−1 are due to the bending of CCC rings. In the range 900–1500 cm−1 only methylene (CH2) internal bending vibrations can be described as group modes of vibrations. The Raman peaks shown around 1093 cm−1 is attributed to the symmetric stretching of υ(COC) of glyosidic ring (Kavkler and Demšar Citation2011). A characteristic band associated with the C-S stretching vibration is observed at 653 cm−1 in the spectrum due to the L-methionine used to modify the cotton fabrics (Giesz et al. Citation2017). The HCC and HCO bending are present as weak intensity vibrations at 996 cm−1. The Raman peaks observed at 1460, 1375, and 1336, are due to the wagging and rocking of methylene (CH2) group. Comparing Figure for AmCot to AmCot-CuO+TO2 it is seen that the functionalization of AmCot by CuO-TiO2 NCPs does not cause any significant changes in the structure of cotton structure at short-range.
Xrd
The XRD pattern of AmCot-CuO+TiO2 is shown in . The strong diffraction peaks at 2θ equals to 17.2°, 22.8° and 25.6° are cellulose I crystalline form peaks (Klemm et al. Citation2005). The diffraction peaks at 35.5° and 38.8° correspond to (−111) and (111) Miller indices, which correspond to CuO crystalline planes (ICDD 01-089-5899). CuO NPs deposited onto the AmCot have an average crystallite size of around 18.09 nm ().
Table 1. Average crystallite size of CuO and TiO2 NPs on Am-Cot-CuO+TiO2 Scherrer equation (Scherrer, Citation1918).
Besides this, the diffraction pattern at 2θ of 36.06°, 41.24°, 56.5° 63.44° and 69.1° corresponds to Miller indices of (101), (111), (211) (002) and (301) plane are ascribed to TiO2 of the rutile phase, which matches well to JCPDS Card No: 21–1276. The average crystallite size of the TiO2 NPs deposited onto the fabric is ca. 32.51 nm using the Scherrer equation ().
Xps
shows the XPS spectrum of AmCot-CuO+TiO2 in wide (range of 150–1200 eV), Ti, Cu, O, C, S spectra, respectively. In the spectrum of AmCot-CuO+TiO2 in wide (), the peaks for elements as carbon (C 1s), copper (Cu 2p), titanium (Ti 2p), nitrogen (N 1s), oxygen (O 1s) and Sulphur (S 2p) are present. The high-resolution core-level XPS spectrum for Ti 2p detected two distinct peaks at BEs of 458.6 eV and 464.1 eV assigned for Ti 2p1/2 and Ti 2p3/2 respectively (). The splitting of energy about 5.5 eV between Ti 2p1/2 and Ti 2p3/2 is revealed the presence of Ti4+ which is in agreement with the reported result for TiO2 (Li et al. Citation2017). The Cu 2p spectrum () displays two strong Cu state peaks, Cu 2p3/2 (934.5 eV) and Cu 2p1/2 (954.3 eV), separated by 19.8 eV. Peaks at 954.3 and 934.5 eV are typical of the Cu2+ state (Shaheen et al. Citation2021).
Figure 4. XPS spectra of AmCot-CuO+TiO2, survey (a), Ti 2p (b), Cu 2p (c), C 1s (d), O 1s (e) and S 2P (f).
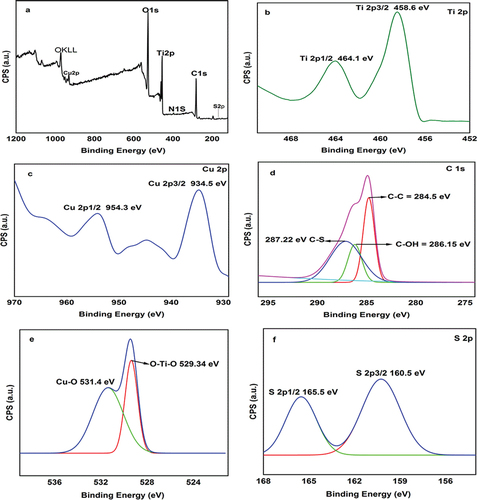
The deconvoluted C 1s peaks () show three peaks at 284.86 eV, 286.15 eV and 287.22 eV, corresponding to C-C, C-OH and C-S bonds, respectively (Xu et al. Citation2018).AmCot-CuO+TiO2 high-resolution O1s XPS spectra are shown in (). Cu-O and Ti-O are represented by two separate peaks centered at 531.7 eV and 529 eV, respectively (Parvathiraja and Shailajha Citation2021). The creation of coordination bonds between Ti atoms and Cu (110) with L-methionine is demonstrated by the peak at 160.5 eV (S 2p3/2) in the S 2p spectra, which corresponds to the strongly de-electronated state of the S element (). The S-C bonds are responsible for the second peak at 165.5 eV (S 2p1/2) (Méthivier et al. Citation2015).
Morphology
shows SEM micrographs of the surface morphology of AmCot, AmCot-CuO+TiO2, and AmCot-CuO+TiO2 after 50 washing cycles at low and high magnification. Micrographs a and e reveal visible space between cotton fiber weaves, indicating L-methionine binding and functionalization by CuO+TiO2 NCPs on the fiber surface. Micrographs (b-d) reveal AmCot fibers’ surface is clean, smooth, and free of contamination, with numerous folds and fibrils, due to scouring and bleaching processes that removed pectin and wax. AmCot-CuO+TiO2 (micrographs f-j) fiber surfaces show rougher texture and a cluster of smaller TiO2 and CuO NPs, confirming Scherrer’s equation-based size difference in XRD (Scherrer Citation1918). The SEM (micrograph j) findings show that even after 50 washing cycles, a significant amount of CuO+TiO2 NCPs remained on the AmCot-CuO+TiO2 surfaces. displays elemental mapping pictures of AmCot and AmCot-CuO+TiO2 taken from a specific region of relevant samples. The S and N element mapping in AmCot indicates that L-methionine is evenly distributed on the fabric. The mapping of Cu and Ti elements in AmCot-CuO+TiO2 in indicates that CuO-TiO2 NCPs are uniformly distributed on the fabric.
Thermal stability analysis
shows the TGA plot of the AmCot and AmCot-CuO+TiO2 NCPs. The thermogram shows AmCot and AmCot-CuO+TiO2 decompose in three stages, with the first stage causing 4.2–6% weight loss in cotton fabric samples at 50–100.6°C (Abidi et al. Citation2009). At the second stage, AmCot undergoes depolymerization, a significant weight loss process between 293.6°C and 379.3°C, triggered by the breakdown of glycosidic linkages in cellulose. In the last stage, weight loss rates are significantly slower between 379.3°C and 545°C, indicating an oxidative breakdown of the second-stage ash residues (Abidi et al. Citation2009). At 600°C, the AmCot residue weight is 3.8%. The thermogram of AmCot-CuO+TiO2 indicates that the second-stage ash residue oxidized at 552°C and 682°C, showing that CuO is more thermally stable than TiO2. At 552°C and 654°C, the ash levels of AmCot+CuO-TiO2 were 12.05% and 10.60%, respectively. Furthermore, the deposition of CuO+TiO2 NCPs on the amine-modified cotton fabric resulted in a significant increase in the residual weight of AmCot-CuO+TiO2 compared to the AmCot cotton sample. The residual weight of amine-modified cotton fabric deposited by CuO+TiO2 NCPs after a 50-washing cycle is AmCot-CuO+TiO2 (50), which is 8.4% (). The remaining amount of NCPs on AmCot-CuO+ TiO2 after 50 washing cycles is ca. 78.9%, when compared to the 0 wash cycle. The percentage of NCPs that were retained on the fabric surfaces indicates that utilizing amine as a binding material is efficient in terms of high laundry durability.
Color analysis
The deposition of CuO+TiO2 NCPs in cotton resulted in a light green hue on AmCot. CIELAB values for AmCot and AmCot-CuO+TiO2-decorated fabrics were not significantly different, as shown in . The table demonstrates that for AmCot-CuO+TiO2, the L* (lightness) reduces from 60.26 to 49.40. The deposition of CuO+TiO2, which gives a light green color to AmCot, causes minimal alteration in the fabric’s original hue. The a* values (red-green) rise from −2.06 to 3.36 for AmCot. This increase is attributed to some of the fibers’ reddish colors following functionalization of AmCot by CuO+TiO2. The b* values (yellow-blue) rise from 2.82 for AmCot to 12.56 in AmCot-CuO+TiO2. The increase in b* values after functionalization is due to a slight change of the original color into a greenish brown color due to the deposition of CuO+TiO2 NCPs. Color strength (K/S) at 425 nm, which is related to yellow color, rises from 0.07 for AmCot to 9.11 for AmCot-CuO+TiO2. The higher the K/S value of AmCot-CuO+TiO2, the more combined CuO and TiO2 NPs are deposited on the cotton fiber surfaces, resulting in less reflectance and more color strength (El-Naggar et al. Citation2021).
Table 2. Colorimetric data for AmCot and AmCot-CuO+TiO2.
Mechanical properties
The tensile strength as well as the elongation at break of AmtCot and AmCot-CuO+TiO2 in the warp and weft patterns are shown in . When compared to AmCot, the tensile strength of AmCot-CuO+TiO2 is lowered by approximately 5.8% in the warp direction and approximately 3.8% in the weft direction. The total reduction in elongation in the warp and weft directions is approximately 8.5%. The findings reveal that functionalizing cotton fabrics with CuO+TiO2 NCPs has no adverse effect on the mechanical properties, which are nearly comparable to those of AmCot. In-situ NCPs coated and bonded on fiber surfaces and between fibers behave like fillers rather than reinforcing agents. The patterns that have been observed are consistent with the literature, which indicates that the functionalization of cotton fibers by NPs has an insignificant effect on mechanical strength and is within the permitted limit for cotton textiles (Arenas-Chávez et al. Citation2022).
Table 3. Tensile strength and elongation of AmCot and AmCot-CuO+TiO2.
Antibacterial activity
exhibited the antibacterial activity of AmCot and AmCot-CuO+TiO2 samples against E. coli (gram-negative) and S. aureus (gram-positive) bacterial species. However, no growth is observed in AmCotCuO+TiO2 samples. These findings imply that AmCot-CuO+TiO2 has high antibacterial activity due to the antibacterial activity of CuO and TiO2 NPs (Subramanian et al. Citation2014). The zones of inhibition or bacterial ingrowth areas for the AmCot-CuO+TiO2 sample showed the presence of synergetic effects in NCPs (Hajipour et al. Citation2021).
Figure 8. Optical images of the antibacterial activity according to the AATCC 147 test method of (a) AmCot, (b) AmCot-CuO+TiO2 against E. coli, and (c) AmCot, (d) AmCot-CuO+TiO2 against S. aureus.
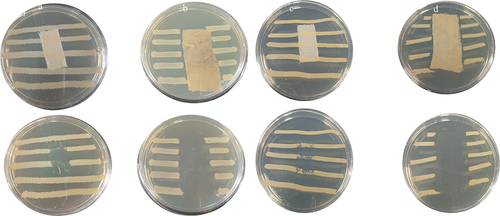
The antibacterial laundry durability of functionalized cotton fabrics
The Zones of inhibition (ZOI) of AmCot-CuO+TiO2 against E. coli and S. aureus are depicted in and . AmCot, the control sample, has no antibacterial activity, whereas AmCot-CuO+TiO2 has excellent antibacterial activity, as seen by their ZOIs. At 0 wash cycle, the ZOIs of AmCot-CuO+TiO2 against S. aureus are approximately 4.4% lower, respectively, than against E. coli. AmCot-CuO+TiO2 exhibits remarkable antibacterial activity retention after 50 wash cycles. After 50 wash cycles, the percentage decrease of ZOIs of AmCot-CuO+TiO2 against E. coli and S. aureus is approximately 22.2% and 24.5%, respectively. The result suggested that cotton fabric functionalized with CuO+TiO2 NCPs exhibited strong antibacterial activity after washing cycles (Khani and Talebian Citation2017; Pan et al. Citation2022)
Figure 9. Optical pictures of AmCot-CuO+TiO2 antibacterial washing durability upto 50 laboratory wash cycles.
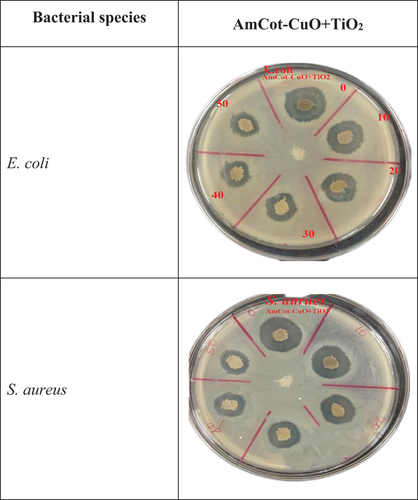
Table 4. AmCot-CuO+TiO2 inhibition zone diameter against E. coli and S. aureus.
The distinct properties of the CuO and TiO2 components have a large influence on the antibacterial and UV protection of the AmCot. However, significant improvements are expected when CuO or TiO2 NPs are incorporated into nanocomposites, particularly in terms of mechanical and thermal stability and increased antibacterial resistance after regular laundry washing. NPs with high surface/volume ratios exhibit bactericidal activity, but their exact mechanism against gram-positive and gram-negative bacteria is still being studied, with various hypotheses proposed in the literature (Salem Citation2022). Gram-negative E. coli cells have a layer of lipopolysaccharides and peptidoglycans with a higher negative charge, unlike gram-positive bacteria like S. aureus with a thicker peptidoglycan layer. E. coli’s lack of a thick peptidoglycan layer makes cell wall breakdown due to physical interaction between NPs and the cell wall more harmful (Slavin et al. Citation2017). The antibacterial activity of CuO and TiO2 nanoparticles is attributed to their small size, which allows interaction with bacteria’s cell walls and membranes (Usman et al. Citation2013). The medium’s production of OH can be toxic to live cells by destroying their nucleic acid helical structure or inactivating their enzymes through a reaction with thiol groups. The inactivation of proteins linked to the cytoplasmic membrane, which affects the transport system and inhibits the function of selective permeability, has also been proposed as a possible explanation for the inhibitory impact of NPs.
Ultra-violet protection factor (UPF)
For UV shielding performance, the ultraviolet protection factor (UPF) of AmCot AmCot-CuO+TiO2 was measured. Solar ultraviolet radiation in the ranges of (280–315 nm) (UVB) and (315–400 nm) (UVA) causes pigmentation in the skin, and prolonged exposure to sensitive skin causes photoallergies, a slight depression in the smoothness of a skin surface, and loss of elasticity (Rajaboopathi and Thambidurai Citation2018). The UV-visible absorbance and transmittance spectra of AmCot, AmCot-CuO+TiO2, and AmCot-CuO+TiO2 after 50 washing cycles are shown in . AmCot has weak UVA radiation blocking properties, as seen by its approximately 42% transmission of UVA radiation; in comparison, AmCot-CuO+TiO2 transmits only 18% and 19% of UVA radiation at 0 washing cycles and after 50 washing cycles, respectively (). The UPF values for AmCot and AmCot-CuO+TiO2 before and after 50 washing cycles are displayed in .
Figure 10. UV-DRS spectra of amine modified AmCot, AmCot-CuO+TiO2 and AmCot-CuO+TiO2 (50) after 50 washing cycles.
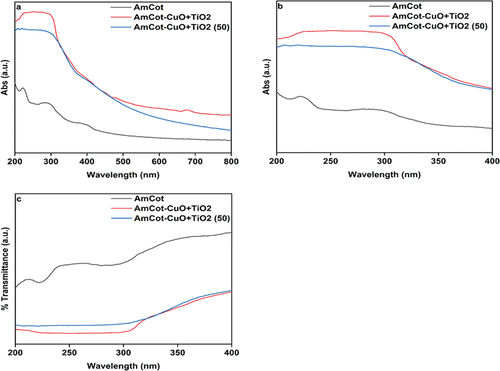
Table 5. UPF values of AmCot, AmCot-CuO+TiO2 and AmCot-CuO+TiO2 (50) after 50 washing cycles.
Water absorption and vapor permeability
Water vapor permeability and absorbability are physical properties of cotton fabric that determine the desirability of cotton fabrics to be well-suited for human skin. These properties of Cot, AmCot, and AmCot-CuO+TiO2 are shown in . It can be noted that AmCot and AmCot-CuO+TiO2 only slightly changed the characteristics of cotton fibers, by around 8.5% and approximately 10.5%, respectively. The formation of coordination bonds between the donor atoms in Am, the Cu2+ and the Ti2+ ions is thought to be the cause of the decrease in water permeability and absorbability. This, in turn, caused a steric hindrance effect, whereby the evenly dispersed NCPs were bound to the surface of the fabric. The gaps (pores) between the individual fibers were reduced so that water molecules had a longer path to pass through. Because of the drop in concentration of free primary and secondary OH groups, absorbability has decreased.
Conclusion
The study utilized a pad and rolling technique to examine the antibacterial resistance of laundered cotton fabrics functionalized with CuO+TiO2 NCPs, revealing excellent wash durability and UV protection. TR-FTIR peaks at 1732 cm−1 show L-methionine esterification with cotton hydroxyl groups, indicating that cotton fabric modification and functionalization do not affect its chemical characteristics. The XRD and XPS examinations demonstrated that the produced particles were of high purity, with typical crystallite sizes of 18.09 and 32.51 nm for CuO NPs and TiO2 NPs, respectively. The difference in particle size between CuO and TiO2 NPs determined by XRD was confirmed by HRSEM pictures. Am is equally grafted onto the cotton fibers, according to EDS mapping of the S and N components. The fabrics functionalized with CuO+TiO2 NCPs showed excellent antibacterial activity against E. coli and S. aureus strains after 50 wash cycles, providing excellent UV protection. In-situ deposition of CuO+TiO2 NCPs on amine-modified cotton results in a negligible alteration in fabric color. The functionalized fabrics’ essential qualities, such as vapor permeability and water absorbability, dropped by 8.5%, while tensile strength and elongation were reduced by 4–9%. Surface modification and functionalization techniques don’t affect cotton’s desired physical characteristics, demonstrating potential for large-scale multifunctional cotton fabric production due to its low cost, durability, and easy extension to other nanoparticles.
Highlights
Cotton fabric functionalized with copper oxide-titanium dioxide nano nanocomposite particles (CuO+TiO2 NCPs) were prepared by using basic pad and rolling technique.
CuO+TiO2 NCPs in-situ synthesized onto the surface of cotton fabric modified by esterification of L-methionine and OH group of cellulose
High crystalline purity of CuO+TiO2 of NCPs is synthesized
Cotton fabric coated with in-situ synthesized CuO-TiO2 of NCPs were exhibit excellent antibacterial activity with laundering durability of up to 50 wash cycles and excellent UV protection
The functionalized “fabrics” essential cotton properties, such as vapor permeability and water absorbability are maintained.
Author contribution statement
Tariku Bayisa: Conceptualization, Methodology, Writing – original draft. Gemechu D: Investigation, Supervision, Validation, Writing – review & editing. Zerihun F.: Graph preparation. Neeraj K.: Supervision, Writing – review & editing. Leta G.: Antimicrobial analysis and Validation.
Acknowledgments
The authors thank Adama Science and Technology University, Ethiopia; for providing the necessary support and facilities for sample preparation and characterization.
Disclosure statement
No potential conflict of interest was reported by the author(s).
References
- Abidi, N., L. Cabrales, and E. Hequet. 2009. “Functionalization of a Cotton Fabric Surface with Titania Nanosols: Applications for Self-Cleaning and UV-Protection Properties.” ACS Applied Materials & Interfaces 1 (10): 2141–18. https://doi.org/10.1021/am900315t.
- Agrawal, N., Low S. Tan, J. S. J. Fong, E.W.M. Lai, Y. and Z. Chen. 2020. “Durable Easy-Cleaning and Antibacterial Cotton Fabrics Using Fluorine-Free Silane Coupling Agents and CuO Nanoparticles.” Nano Materials Science 2 (3): 281–291. https://doi.org/10.1016/j.nanoms.2019.09.004.
- Arenas-Chávez, P., C. A. Limpe-Ramos, G. M. Marcela, C. R. Mejia, A. Del-Aguila-Arcentales, S. Alvarez-Risco, C. Vera-Gonzales, J A. Yáñez, and C. Vera-Gonzales. 2022. “Physical and Mechanical Characterization of a Functionalized Cotton Fabric with Nanocomposite Based on Silver Nanoparticles and Carboxymethyl Chitosan Using Green Chemistry.” Processes 10 (6): 1207. https://doi.org/10.3390/pr10061207.
- Barman, J., Tirkey, A. Batra, S. Paul, A. A. Panda, K. Deka, R. and P.J. Babu. 2022. “The Role of Nanotechnology Based Wearable Electronic Textiles in Biomedical and Healthcare Applications.” Materials Today Communications 32:104055. https://doi.org/10.1016/j.mtcomm.2022.104055.
- Cheng, D., M. He, G. Cai, X. Wang, J. Ran, and J. Wu. 2018. “Durable UV-Protective Cotton Fabric by Deposition of Multilayer TiO2 Nanoparticles Films on the Surface.” Journal of Coatings Technology and Research 15 (3): 603–610. https://doi.org/10.1007/s11998-017-0021-8.
- Dave, H., Ledwani, L. Chandwani, N. Chauhan, N. and S.K. Nema. 2014. “The Removal of Impurities from Gray Cotton Fabric by Atmospheric Pressure Plasma Treatment and Its Characterization Using ATR-FTIR Spectroscopy.” The Journal of the Textile Institute 105 (6): 586–596. https://doi.org/10.1080/00405000.2013.827900.
- El-Naggar, M.E., Khattab, T.A. Abdelrahman, M.S. Aldalbahi, A. and M.R. Hatshan. 2021. “Development of Antimicrobial, UV Blocked and Photocatalytic Self-Cleanable Cotton Fibers Decorated with Silver Nanoparticles Using Silver Carbamate and Plasma Activation.” Cellulose 28 (2): 1105–1121. https://doi.org/10.1007/s10570-020-03537-4.
- El-Naggar, M.E. Shaheen, T.I. Zaghloul, S. El-Rafie M.H. and A. Hebeish. 2016. “Antibacterial Activities and UV Protection of the in situ Synthesized Titanium Oxide Nanoparticles on Cotton Fabrics Industrial &.” Industrial & Engineering Chemistry Research 55 (10): 2661–2668. https://doi.org/10.1021/acs.iecr.5b04315.
- El-Nahhal, I.M. Elmanama, A.A. Amara N. Qodih, F.S. Selmane, M. and M.M. Chehimi. 2018. “The Efficacy of Surfactants in Stabilizing Coating of Nano-Structured CuO Particles Onto the Surface of Cotton Fibers and Their Antimicrobial Activity.” Materials Chemistry and Physics 215:221–228. https://doi.org/10.1016/j.matchemphys.2018.05.012.
- El-Nahhal, I.M., Salem, J. Kodeh, F. Anbar, R. and A. Elmanama. 2021. “Preparation of CuO-NPs Coated Cotton, Starched Cotton and Its CuO-Ag Nanocomposite, Cu (II) Curcumin Complex Coated Cotton and Their Antimicrobial Activities Nanomed.” Nanotechnol 12:566. https://doi.org/10.1016/j.matchemphys.2022.126099.
- Giesz, P., Mackiewicz, E. Nejman, A. Celichowski, G. and M.Cieślak. 2017. “Investigation on Functionalization of Cotton and Viscose Fabrics with AgNws.” Cellulose 24 (1): 409–422. https://doi.org/10.1007/s10570-016-1107-7.
- Hajipour, P., Eslami, A. Bahrami, A. Hosseini-Abari, A. Saber, F. Mohammadi, R. and M. Mehr. 2021. “Surface Modification of TiO2 Nanoparticles with CuO for Visible-Light Antibacterial Applications and Photocatalytic Degradation of Antibiotics.” Ceramics International 47 (23): 33875–33885. https://doi.org/10.1016/j.ceramint.2021.08.300.
- Ibrahim, N.A., Nada, A.A. Eid, B.M. Al-Moghazy, M. Hassabo, A.G. and N.Y. Abou-Zeid. 2018. “Nano-Structured Metal Oxides: Synthesis, Characterization and Application for Multifunctional Cotton Fabric.” Natural Sciences: Nanoscience and Nanotechnology 9 (3): 035014. https://doi.org/10.1088/2043-6254/aadc2c.
- Jeevanandam, J., Barhoum, A. Chan, Y.S. Dufresne, A. and M.K. Danquah. 2018. “Review on Nanoparticles and Nanostructured Materials: History, Sources, Toxicity and Regulations.” Beilstein Journal of Nanotechnology 9:1050–1074. https://doi.org/10.3762/bjnano.9.98.
- Kavkler, K., and A. Demšar. 2011. “Examination of Cellulose Textile Fibres in Historical Objects by Micro-Raman Spectroscopy.” Spectrochimica Acta Part A: Molecular and Biomolecular Spectroscopy 7878 (2): 740–746. https://doi.org/10.1016/j.saa.2010.12.006.
- Khani, A., and N. Talebian. 2017. “In vitro Bactericidal Effect of Ultrasonically Sol–Gel-coated Novel CuO/TiO2/PEG/cotton Nanocomposite for Wound Care.” Journal of Coatings Technology and Research 14 (3): 651–663. https://doi.org/10.1007/s11998-016-9870-9.
- Khashan, K. S., Sulaiman, G. M. Abdulameer, F. A. Albukhaty, S. Ibrahem, M. A., Al-Muhimeed, T. and A.A. AlObaid. 2021. “Antibacterial Activity of TiO2 Nanoparticles Prepared by One-Step Laser Ablation in Liquid.” Applied Sciences 11 (10): 4623. https://doi.org/10.3390/app11104623.
- Khosravian, S., Montazer, M., Malek, R.M. and T. Harifi. 2015. “In situ Synthesis of Nano ZnO on Starch Sized Cotton Introducing Nano Photo Active Fabric Optimized with Response Surface Methodology.” Carbohydrate Polymer 132:126–133. https://doi.org/10.1016/j.carbpol.2015.05.085.
- Klemm, D., Heublein, B. Fink, H.P, and A. Bohn. 2005. “Cellulose: Fascinating Biopolymer and Sustainable Raw Material.” Angewandte chemie international edition 44 (22): 3358–3393. https://doi.org/10.1002/anie.200460587.
- Li, S. Zhu T. Huang, J. Guo, Q. Chen, G. and Y. Lai. 2017. “Durable Antibacterial and UV-Protective Ag/TiO2@ Fabrics for Sustainable Biomedical Application.” International Journal of Nanomedicine 12:2593. https://doi.org/10.2147/IJN.S132035.
- Marković, D., Korica, M. Kostić, M. Radovanović, Ž. Šaponjić, Z. Mitrić, M. and M. Radetić. 2018. “In situ Synthesis of Cu/Cu2O Nanoparticles on the TEMPO Oxidized Cotton Fabrics.” Cellulose 25 (1): 829–884. https://doi.org/10.1007/s10570-017-1566-5.
- Méthivier, C., V. Humblot, and C. M. Pradier. 2015. “L-Methionine Adsorption on Cu (110), Binding and Geometry of the Amino Acid as a Function of Coverage.” Surface Science 632:88–92. https://doi.org/10.1016/j.susc.2014.09.007.
- Murray, C. J. Ikuta, K. S Sharara, F. Swetschinski, L. Aguilar, G. R. Gray, A.and N. Tasak, Bisignano, C., Rao, P., Wool, E., Johnson, S C. 2022. “Global Burden of Bacterial Antimicrobial Resistance in 2019: A Systematic Analysis.” The Lancet 399 (10325): 629–655. https://doi.org/10.1016/S0140-6736(21)02724-0.
- Novais, J. S. Moreira, C. S. Silva, A. C. J. Loureiro, R. S., Figueiredo, A. M. S., Ferreira, V. F. and D.R. Rocha, da Rocha, D R. 2018. “Antibacterial Naphthoquinone Derivatives Targeting Resistant Strain Gram-Negative Bacteria in Biofilms.” Microbial Pathogenesis 118:105–114. https://doi.org/10.1016/j.micpath.2018.03.024.
- Pan, C., Shen, H., Liu, G., Zhang, X., Liu, X., Liu, H. and Y. Guo. 2022. “CuO/TiO2 Nanobelt with Oxygen Vacancies for Visible-Light-Driven Photocatalytic Bacterial Inactivation.” ACS Applied Nano Materials 5 (8): 10980–10990. https://doi.org/10.1021/acsanm.2c02226.
- Parvathiraja, C., and S. Shailajha. 2021. “Bioproduction of CuO and Ag/CuO Heterogeneous Photocatalysis-Photocatalytic Dye Degradation and Biological Activities.” Applied Nanoscience 11 (4): 1411–1425. https://doi.org/10.1007/s13204-021-01743-5.
- Rajaboopathi, S., and S. Thambidurai. 2018. “Evaluation of UPF and Antibacterial Activity of Cotton Fabric Coated with Colloidal Seaweed Extract Functionalized Silver Nanoparticles.” Journal of Photochemistry and Photobiology B: Biology 183:75–87. https://doi.org/10.1016/j.jphotobiol.2018.04.028.
- Rehan, M., Barhoum, A., Van Assche, G. Dufresne, A. Gätjen, L. and R. Wilken. 2017. “Towards Multifunctional Cellulosic Fabric: UV Photo-Reduction and in-Situ Synthesis of Silver Nanoparticles into Cellulose Fabrics.” International Journal of Biological Macromolecules 98:877–886. https://doi.org/10.1016/j.ijbiomac.2017.02.058.
- Rehman, Z.U., Huh, S.H. Ullah, Z. Pan, Y.T. Churchill, D.G. and B.H. Koo. 2021. “LBL Generated Fire Retardant Nanocomposites on Cotton Fabric Using Cationized Starch-Clay-Nanoparticles Matrix.” Carbohydrate Polymer 274:118626. https://doi.org/10.1016/j.carbpol.2021.118626.
- Rezaie, A.B. Montaze, M. Rad, M.M. 2018. “Environmentally Friendly Low Cost Approach for Nano Copper Oxide Functionalization of Cotton Designed for Antibacterial and Photocatalytic Applications.” Journal of Cleaner Production 204:425–436. https://doi.org/10.1016/j.jclepro.2018.08.337.
- Salem, S.S. 2022. “Baker’s Yeast-Mediated Silver Nanoparticles: Characterisation and Antimicrobial Biogenic Tool for Suppressing Pathogenic Microbes.” BioNanoscience 12 (4): 1220–1229. https://doi.org/10.1007/s12668-022-01026-5.
- Scherrer, P. 1918. “Göttinger nachrichten math.” Physics 2:98–100.
- Shaheen, T. I., A. Fouda, and S. S. Salem. 2021. “Integration of Cotton Fabrics with Biosynthesized CuO Nanoparticles for Bactericidal Activity in the Terms of Their Cytotoxicity Assessment.” Industrial & Engineering Chemistry Research 60 (4): 1553–1563. https://doi.org/10.1021/acs.iecr.0c04880.
- Slavin, Y.N., Asnis, J. Häfeli, U.O. and H. Bach. 2017. “Metal Nanoparticles: Understanding the Mechanisms Behind Antibacterial Activity.” Journal of Nanobiotechnology 15 (1): 65. https://doi.org/10.1186/s12951-017-0308-z.
- Subramanian, B., Priya, K. Rajan, S. Dhandapani, P. and M. Jayachandran. 2014. “Antimicrobial Activity of Sputtered Nanocrystalline CuO Impregnated Fabrics.” Materials Letters 128:1–4. https://doi.org/10.1016/j.matlet.2014.04.056.
- Usman, M.S., Zowalaty, M. E. Shameli, K. Zainuddin, N. Salama, M. and N.A. Ibrahim. 2013. “Synthesis, Characterization, and Antimicrobial Properties of Copper Nanoparticles.” International Journal of Nanomedicine 8:4467–4479. https://doi.org/10.2147/IJN.S50837.
- Verma, V., Al-Dossari, M. Singh, J. Rawat, M. Kordy, M.G.M. and M. Shaban. 2022. “A Review on Green Synthesis of TiO2 NPs: Synthesis and Applications in Photocatalysis and Antimicrobial.” Polymers 14 (7): 1444. https://doi.org/10.3390/polym14071444.
- Wei, T., Qu, Y. Zou, Y. and Y. Zhang Y, Yu, Q. 2021. “Exploration of Smart Antibacterial Coatings for Practical Applications.” Current Opinion in Chemical Engineering 34:100727. https://doi.org/10.1016/j.coche.2021.100727.
- Xu, Q., Ke, X. Cai, D. Zhang, Y. Fu, F. Endo, T. and X. Liu. 2018. “Silver-Based, Single-Sided Antibacterial Cotton Fabrics with Improved Durability via an L-Cysteine Binding Effect.” Cellulose 25 (3): 2129–2141. https://doi.org/10.1007/s10570-018-1689-3.
- Xu, Q., Li, R. Shen, L. Xu, W. Wang, J. Jiang, Q. Zhang, L. Fu, F. Fu, Y. and X. Liu. 2019. “Enhancing the Surface Affinity with Silver Nano-Particles for Antibacterial Cotton Fabric by Coating Carboxymethyl Chitosan and L-Cysteine.” Applied Surface Science 497:143673. https://doi.org/10.1016/j.apsusc.2019.143673.
- Yuce, I., Canoglu, S. Yukseloglu, S.M. Li Voti, R. Cesarini, G. Sibilia, C. and M.C. Larciprete. 2022. “Titanium and Silicon Dioxide-Coated Fabrics for Management and Tuning of Infrared Radiation.” Sensors 22 (10): 3918. https://doi.org/10.3390/s22103918.
- Zhou, J., Cai, D. Xu, Q. Zhang, Y. Fu, F. Diao, H. and X. Liu. 2018. “Excellent Binding Effect of L-Methionine for Immobilizing Silver Nanoparticles Onto Cotton Fabrics to Improve the Antibacterial Durability Against Washing.” RSC advances 8 (43): 24458–24463. https://doi.org/10.1039/C8RA04401E.