Abstract
Sea urchins are consumed in many parts of the world today. Human consumption of these marine invertebrates can be traced back to at least the Late Pleistocene in various archaeological sites from Island Southeast Asia. Makpan Cave, on Alor Island in Indonesia, is one of the earliest Wallacean prehistoric sites with an occupation record beginning ∼43,000 years ago. Coastal resources (i.e., shellfish, barnacle, crab, and sea urchin) play a dominant role in the subsistence strategy at this site, with urchins important throughout all occupation phases. In this study, we used three different quantification methods (weight, NISP, and MNI) to analyze the over 13 kg of sea urchin remains recovered from Makpan. Our analysis showed that sea urchins were collected during the initial occupation period, when other coastal resources were sparsely utilized. This indicates that sea urchins were intentionally targeted rather than being the result of subsidiary foraging during shellfish collection. The quantity and consistency of sea urchin exploitation at Makpan indicates that urchin was considered a staple food, especially during the initial phase of occupation. We hypothesize that a focus on sea urchins may have been a key strategy for the colonization of novel environments by the earliest human settlers in the region.
Introduction
Records of past sea urchin consumption
Archaeological records confirm that sea urchins have formed part of the human diet since prehistoric times. The earliest evidence, globally, for sea urchin consumption by humans comes from archaeological sites in Timor-Leste in southern Wallacea where urchin remains have been recovered from the coastal site of Asitau Kuru in layers dated to ∼40 ka (Boulanger et al. Citation2023; Shipton et al. Citation2019). Other Wallacean sites, including Laili in Timor-Leste and Leang Sarru in the Talaud Islands (Heterocentrotus mamillatus), have also recovered sea urchin remains as early as ∼25 ka and ∼35 ka, respectively (Hawkins et al. Citation2017; Ono, Soegondho, and Yoneda Citation2009).
In Europe, sea urchin (Paracentrotus lividus) was exploited from the Late Pleistocene at ∼15 ka alongside other coastal resources (Gutiérrez-Zugasti Citation2011; Gutiérrez-Zugasti et al. Citation2016). In North America, sea urchin (Strongylocentrotus spp.) exploitation is recorded from the Early Holocene ∼10–8 ka (Ainis Citation2022; Erlandson et al. Citation2005). Pacific islanders are also known to have widely exploited urchins from the time of first settlement in the Late Holocene (Kirch et al. Citation1995; Petchey and Kirch Citation2019; Weisler, Mihaljević, and Rogers Citation2020). People also used sea urchin products as tools and for decorative/spiritual purposes. The spines (e.g., H. mamillatus, Echinothrix spp., and Colobocentrotus atratus) were used as abrading tools in the Pacific (Burney and Kikuchi Citation2006; Dixon et al. Citation2022; Field et al. Citation2010; Sand Citation1998; Weisler, Mihaljević, and Rogers Citation2020), burial goods (Valentin et al. Citation2020), and were also sometimes carved into personal religious figurines (Titcomb et al. Citation1978). Sea urchin remains were also included in offerings placed at fishing shrines in the Hawaiian Islands (Weisler, Mihaljević, and Rogers Citation2020). On the northwest coast of Australia, a ground sea urchin spine from the site of Widgingarri 1 is evidence for the movement of this artifact over 200 km inland from the coast at least 30 ka based on recent redating (Norman et al. Citation2022; O’Connor Citation1999, 78). In Europe, sea urchin tests as well as spines, particularly fossil ones, were integrated into folklore and mythology and often subject to fanciful interpretations on their nature (Duffin Citation2006; McNamara Citation2006, Citation2007).
Sea urchin remains are usually highly fragmented and can be easily missed during excavation and post-excavation recovery. In archaeological contexts, sea urchin remains are mostly products of consumption waste (Ainis Citation2022; Kaharudin et al. Citation2019). In order to obtain the roe, people must break the test (i.e., the globular internal skeleton, sometimes erroneously referred to as echinoid “shell”) either with their bare hands or using tools. The easiest way to access the roe is from the base, where the urchin jaw apparatus (known as Aristotle’s lantern) is located (Grisolía, López, and de Dios Ortúzar Citation2012; HAFK personal observation). Due to this butchery practice, in addition to their natural fragility and skeleton consisting of numerous plates predominantly connected by connective tissue, sea urchin remains are extremely unlikely to be preserved intact in archaeological contexts (Campbell Citation2008a). Additionally, the spines of some species of sea urchin (e.g., Tripneustes gratilla) are relatively small, between ∼0.5 and 1 mm wide and from ∼4 to 7 mm long. Many archaeological excavations, especially early research endeavors, sieved through mesh sizes of 6–3 mm in size or larger. This large mesh size fails to recover the majority of small/fragmented materials, resulting in the loss of significant amounts of urchin remains during sieving (Cannon Citation1999; Weisler, Mihaljević, and Rogers Citation2020).
Limited recovery of sea urchins from archaeological contexts can also result from misidentification due to researcher unfamiliarity with the specimens. Sites which are not in close proximity to the coast and recover only a small number of urchin remains, in particular, are less likely to see a focused effort on urchin recovery and identification (Hawkins et al. Citation2017; Kaharudin et al. Citation2023). Some species of sea urchin (e.g., Mespilia globulus) have really thin spines with a red-brownish color that can be misidentified as plant remains or bone fragments, for example. Similarly, parts from the sea urchin jaw apparatus are difficult to recognize for anyone unfamiliar with echinoids. The demipyramids are often misidentified as a fragment of acorn barnacles (i.e., the tergum and scutum parts), while the epiphyses, teeth, and rotulae are often mistakenly identified as shell fragments (HAFK personal observation). Further many early excavators assigned greater research emphasis to bone and shellfish remains with less significance placed on the recovery of sea urchin (Boulanger et al. Citation2019; Samper Carro et al. Citation2016). This reduced focus meant that even if urchins were recovered, they were sometimes left out of the excavation report or publication, making it harder to track in the literature.
What can sea urchins tell us about past peoples?
In recent years, the recognition and recovery of sea urchins from archaeological excavations in proximity to the coast has increased (e.g., Ainis Citation2022; Anderson and Kennett Citation2012; Kaharudin et al. Citation2019; Weisler, Mihaljević, and Rogers Citation2020). This raises a number of questions regarding the role of sea urchin in prehistoric diets: (1) is the presence of sea urchin in the archaeological assemblage the result of focused collection or merely opportunistic behavior during shellfish harvesting?; (2) was sea urchin exploited as a staple subsistence food resource, or rather an occasional delicacy or ritual food?; and (3) how are different sea urchin habitats influenced by changing environmental conditions? (Gutiérrez-Zugasti et al. Citation2016; Milner Citation2009a, Citation2009b).
Dietary options have played a significant role in shaping cultural identities and food sources may be perceived beyond just the functional need to obtain nutritional values. Some foods can have social or symbolic meaning to communities (Hayden Citation2003; Kirch and O’Day Citation2003). Changes in ideas on how people see particular foods have also occurred throughout human history, with lobster, barnacle, and snail, being good examples (Dean Citation2010; Ghosh, Jung, and Meyer-Rochow Citation2016; Tye Citation2011). However, despite the frequency of these remains in archaeological contexts, their role in prehistoric diets is rarely fully understood.
Sea urchin gonad or “roe” quantity are heavily affected by seasons, reproductive cycle, water temperature, and ocean acidification, as demonstrated by studies of sea urchin gonad index (Seymour et al. Citation2013; Uthicke et al. Citation2014). The use of seasonal and lunar periodicity to maximize the yield of sea urchin gonads has been extensively studied, and people must harvest them at a particular time(s) of the year to obtain the maximum yield of sea urchin roe (Muthiga Citation2005; Ono Citation2010; Rocha et al. Citation2019). The seasonality of sea urchins can make them less favorable compared with other marine resources such as certain shellfish and fish that are available year-round. However, traditional communities often have their own knowledge regarding the best time to harvest sea urchin, both monthly and/or annually based on the most commonly exploited species and geographic location (Ono Citation2010; Titcomb et al. Citation1978). For example, the traditional Hawaiian proverb, “When the hala flowers are ripe, the sea eggs are fat” (Judd Citation1930, 9), indicates how the availability of sea urchins can be predicted. As with other food staples with limited seasonal harvesting times, such as whales (e.g., Egami and Kojima Citation2013) and red pandanus fruits (Bulmer Citation2005), the predictability of sea urchins makes them a reliable resource for many coastal communities, alongside year-round foods such as taro and breadfruit (Campbell Citation2015). The New Zealand Māori even developed techniques for preservation whereby they placed the urchins in freshwater or buried them underground enabling storage for several months (Whyte et al. Citation2001).
Sea urchin distribution covers various types of marine ecosystems from intertidal to bathyal (Mooi and Munguia Citation2014). Some species, such as C. atratus, commonly live in the shallow intertidal zone where they can be found in rock pools or fissures. Other species, including T. gratilla, Temnopleurus toreumaticus, and Salmacis belli, are frequently observed in subtidal zones that have a sandier environment with a higher abundance of seagrass and algae. Lastly, some species, such as H. mamillatus, prefer subtidal zones with a rich reef ecosystem. These ecosystem preferences mean sea urchin remains can be used as indicators to predict the type of paleo-coastal environment(s) people exploited in proximity to their occupation site (Kroh and Nebelsick Citation2003).
Sea urchin density is often used as an indicator of reef ecosystem health by marine biologists. High quantities of sea urchins will result in heavy grazing of kelp forests and can be a sign of an unhealthy reef ecosystem (Coyer et al. Citation1993; Ling et al. Citation2015; Steneck et al. Citation2002). On the other hand, an absence or low density of urchins in the ecosystem can indicate an over-harvesting or unstable non-human predator–prey relationships (Pfister and Bradbury Citation1996). Disturbances in reef ecosystem equilibrium are sometimes caused by changes in the abundance of sea urchin predators such as fish, crab, lobsters, and sea otters (Breen and Mann Citation1976; Hamilton and Caselle Citation2015; Lowry and Pearse Citation1973). Additionally, the dynamics of sea urchin populations can also be related to environmental changes such as ocean currents, temperature, red tides, and sometimes disease (Lessios Citation1988; Ling et al. Citation2009; Ohgaki et al. Citation2019; Sherman Citation2015). It is clear that the study of past sea urchin assemblages has the potential to shed light on a wide variety of topics from paleoenvironments and past coastal ecologies, to early human subsistence strategies and patterns of resource use (Ainis Citation2022; Erlandson et al. Citation2005; Gutiérrez-Zugasti Citation2011; Gutiérrez-Zugasti et al. Citation2016; Weisler, Mihaljević, and Rogers Citation2020).
Previous in-depth studies analyzing large sea urchin assemblages are mostly focused on Late Holocene sites and/or assemblages with low taxonomic diversity. For example, Weisler, Mihaljević, and Rogers (Citation2020) analyzed ∼11.7 kg of a single species, C. atratus, from human diet and ritual use in post-1500s AD Polynesia. Field, Lipphardt, and Kirch (Citation2016) recovered ∼1.71 kg total remains of five different species of sea urchin (C. atratus, Echinothrix diadema, Echinometra mathaei, H. mamillatus, and T. gratilla) from the Kohala residential deposits in Hawaii spanning the last 600 years. To date, prehistoric sea urchin consumption studies investigating sites dating back to the Late Pleistocene or Early Holocene show a significant lack of taxonomic diversity, mostly due to the limited number of sea urchin species available in the area. Among them are Gutiérrez-Zugasti (Citation2011) and Gutiérrez-Zugasti et al. (Citation2016) who reported ∼0.27 kg and ∼5.9 kg of P. lividus, respectively, from multiple sites on the Atlantic coast of the Iberian Peninsula, dated to ∼15–7 ka. Ainis (Citation2022) recovered ∼33 kg of Strongylocentrotus spp. from two archaeological sites in California’s Channel Islands, North America dated back to ∼8 ka. In contrast, here we present evidence of at least seven different sea urchin taxa that were regularly exploited at the single site of Makpan since ∼43 ka.
Sea urchins in Wallacean archaeological research
Wallacea, the tropical island archipelago between continental Sunda and Sahul, is a region with an abundance of prehistoric coastal sites and documents a long history of maritime resource use (Kealy, Louys, and O’Connor Citation2017; O’Connor, Ono, and Clarkson Citation2011). Because the offshore bathymetry of many of the Wallacean islands drops steeply to the continental shelf, the relative location of the position of the coastline has changed little during Late Pleistocene/Holocene sea level regression and transgression. Thus, many cave sites uniquely document maritime subsistence throughout this time. The region therefore has huge potential to contribute to our understanding of prehistoric coastal lifeways.
A number of archaeological sites in Wallacea have reported the recovery of sea urchin remains, including Leang Sarru (Talaud Island), Tron Bon Lei (Alor Island), Here Sorot Entapa (Kisar Island), Asitau Kuru, Lene Hara, Laili (Timor-Leste), and Kelo 6 (Obi Island) (per site respectively Ono, Soegondho, and Yoneda Citation2009; O’Connor et al. Citation2017; O’Connor et al. Citation2019; O’Connor Citation2015; idem; Hawkins et al. Citation2017; Shipton et al. Citation2020). However, so far there have been very limited attempts to explore these remains in more detailed analyses. Most of these sites recovered evidence for occupation from the Late Pleistocene to Early Holocene and preserve high quantities of marine faunal remains and artifacts. Some, such as Laili and Kelo 6, are located further inland but still close enough to have maintained access to marine resources ().
Figure 1. Map showing the location of Makpan (inset) and the surrounding archaeological sites in Wallacea (main) where recovery of sea urchin has been reported: (1) Leang Sarru; (2) Kelo 6; (3) Here Sorot Entapa; (4) Asitau Kuru; (5) Lene Hara; (6) Laili.
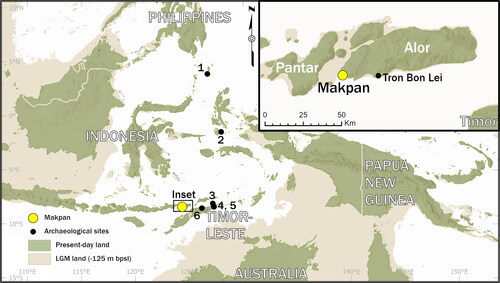
The recently reported archaeological site of Makpan Cave on Alor Island, where a high density of marine faunal remains were recovered, has one of the oldest occupation records in Wallacea. The lowest occupation layer dates back to 40,208–38,454 cal BP (median modeled date is 43,076 cal BP), with the excavation producing evidence for intermittent habitation through to the present day (Kealy et al. Citation2020). In total, the Makpan excavation recovered an impressive ∼13 kg of sea urchin remains from the 1 × 1 m test pit B, presenting the opportunity to conduct a thorough investigation of human and sea urchin interactions in prehistoric Wallacea, and the Indo-West Pacific in general.
Site description
Makpan is a large lava tube cave located in Alor Island, Indonesia. It is located about 2 km between the two nearby villages of Halmin and Ling Al. The villages are barely accessible by road, with the easiest access by sea from Kalabahi. Once reached, the cave can be approached from the villages by walking along the coast or taking a more inland, hilly path. Makpan Cave is located at the bottom of a hill, facing south across a wide, relatively flat savannah before stepping down toward the rocky-sandy beach approximately 1.8 km from the cave entrance (). The site looks out over the Pantar and Ombai Straits, with the island of Treweng visible from the beach ( and ).
Figure 2. (A) Approaching Makpan Cave; (B) view from inside the cave toward the coast; (C) view westward along the coastline from Makpan.
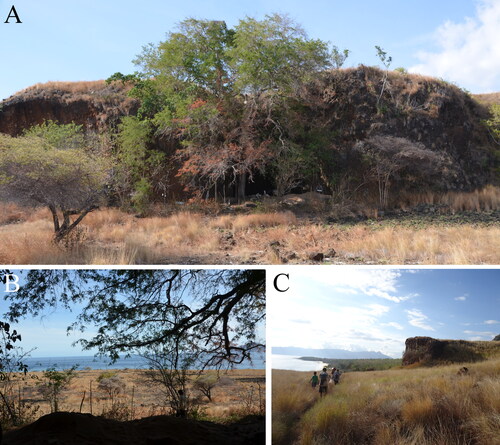
The word “Makpan” means “echo” in the local language, reflecting the size and depth of the chamber (Kealy et al. Citation2020). The site is currently used as a temporary resting spot providing a welcome refuge for people walking between the villages of Halmin and Ling Al (∼4.5 km). Large clam shells placed strategically collect fresh water dripping through the limestone (HAFK personal observation and personal communication from local villagers, July 23, 2016). Children from Ling Al regularly visit the cave to drink the shell water when returning home from school in Halmin. The local communities also use Makpan as a gathering spot during the seasonal deer hunt in the surrounding hills (personal communication of Halmin village elder to HAFK, July 23, 2016).
Makpan was excavated during June–July of 2016 as part of a collaborative research effort between the Australian National University (ANU) and Universitas Gadjah Mada (UGM) under the “From Sunda to Sahul” project led by author O’Connor. A 2 × 2 m test pit was opened near the cave entrance and divided into four adjoining 1 × 1 m squares: A, B, C, and D (Kealy et al. Citation2020: ). The excavation was undertaken in spits of ∼5 cm, following observable stratigraphic change. The 2 × 2 m test pit was dug to a depth of ∼1.5 m (spit 23), after which the excavation was reduced to just the B square, which was continued down to a depth of ∼3.5 m (spit 68). Culturally sterile beach sand was reached at spit 68 (Kealy et al. Citation2020).
Figure 3. Comparison between the three sea urchin quantification methods: weight, NISP, and MNI by spit recovered at Makpan. Occupation intensity represented by proxy from the total adjusted fauna weight (excluding micro-vertebrates). Dashed line indicates occupation phase boundary.
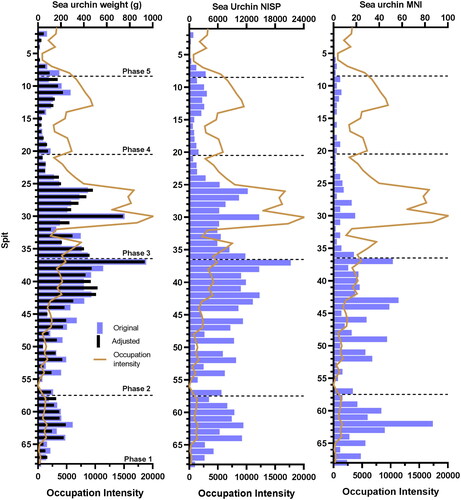
Makpan was revealed to have been used as an occupation shelter since around 43,000 cal BP, making it one of the oldest archaeological sequences in Wallacea (Kaharudin et al. Citation2020; Kealy et al. Citation2020). Both vertebrate and invertebrate faunal remains were recovered as part of Makpan’s past consumption waste, with the assemblage overwhelmingly dominated by the remains of marine fauna such as fish, turtle, shellfish, barnacle, crab, and sea urchin (Kealy et al. Citation2020). However, as revealed by Roberts et al.’s (Citation2020) isotopic analysis, the Makpan inhabitants at this time were still clearly incorporating a substantial amount of terrestrial foodstuffs into their diet, suggesting that the zooarchaeological record is rather a record of protein subsistence, lacking the complimentary archaeobotanical record.
Kealy et al. (Citation2020) identified five distinct phases of occupation at Makpan (see ): (1) initial occupation/the Late Pleistocene (∼43–15 ka); (2) the Terminal Pleistocene (∼14–12 ka); (3) the Pleistocene–Holocene transition (midden deposit; ∼12–11 ka); (4) the Early–Middle Holocene (∼10–7 ka); and (5) the Neolithic–Historic (∼4 ka–present). Sea urchin remains were recovered in all five occupation phases, albeit in different quantities and with variable species representation. This research analyzes the Makpan sea urchin assemblage to determine species abundance, the role of sea urchin exploitation, and what changes in urchin exploitation patterns might allow us to infer about corresponding changes in cultural and/or environmental conditions.
Table 1. Total abundance of sea urchin remains from Makpan by phase.
Material and methods
Sea urchin assemblages from all spits of Makpan square B (1–68) were analyzed. Unfortunately, material from spit 56 is missing, likely due to the long process of transportation from the site, so is excluded from this analysis. All the excavated sediment was weighed before receiving two types of sieving treatment: dry and wet. Wet sieving is crucial to reduce sediment volume and reveal the more easily distinguished colors of the faunal remains and other cultural materials during sorting. The deposit was sieved with a 1.5 mm mesh which was small enough to conserve the majority of fragmented artifacts and faunal remains. The sieved sediment went through multiple washing and sorting stages to minimize the loss of any overlooked or misidentified archaeological materials. For the Makpan sea urchin assemblage recovered as a result of this process, we then implemented taxonomical identification, quantification, and statistical analyses. Supplementary information is presented in files S1–S7.
Identification
Preliminary sorting was conducted on site to separate sea urchin from other faunal remains and cultural artifacts. Further identification and analyses were conducted back in the laboratory. Taxonomical identifications were based on morphological characteristics on the spines distinguished through comparisons with reference collections of sea urchins from the Australian National University (ANU), Australian Museum (AM), Indonesian Institute of Sciences (LIPI), as well as the Echinoid directory website of the UK Natural History Museum (NHMUK; Smith and Kroh Citation2011). The spines on most sea urchin taxa in the Indo-Pacific are quite distinctive, especially in an archaeological context where the flesh and its associated distinctive colors and patterns are no longer preserved (e.g., Miskelly Citation2002). Specifically, morphological characteristics of spine shape (e.g., C. atratus versus Echinometra spp.) and size (e.g., Salmacis spp. versus H. mamillatus) were utilized to identify the urchin taxa from Makpan (Supplementary material, Figure S2.1). Unfortunately, the fragmented nature of the remains and time constraints of the study meant we were unable to refine the taxonomic identification of the other sea urchin body parts (e.g., test, demipyramids, etc.) for the present study.
Quantification and statistical analyses
Number of identified specimens (NISP), minimum number of individuals (MNI), and weight of remains (weight) in grams to two decimal places were implemented as different types of quantification analyses. NISP was obtained for all identified sea urchin spines recovered from the excavation, both complete and fragmented, as these elements were the only ones to be consistently identified to the different taxa studied here. MNI was obtained by counting a specific sea urchin body part, depending on which one has the largest quantity across the assemblage (Ainis Citation2022; Weisler, Mihaljević, and Rogers Citation2020). The elements from Aristotle’s lantern and auricle were the most common. Since sea urchins have a fivefold symmetry, like most other echinoderms, each body part count is divided by five to get an MNI of 1 (except the madreporite). For instance, Aristotle’s lantern consists of 10 demipyramids (five left and five right), 10 epiphyses (five left and five right), five rotulae, five compasses, and five teeth (Carnevali et al. Citation1993). Demipyramids were the most common of these elements throughout the assemblage and so were used in our analysis for determining MNI (Supplementary material, Figure S1.2). Whichever demipyramid side (either left or right) was the most abundant in a spit, was divided by five to provide the MNI. Other body parts such as ocular plates, genital plates, and madreporite also have the potential to be used as MNI (Campbell Citation2008a, Citation2008b; Smith and Kroh Citation2011), but are less likely to be found in archaeological excavations due to their tiny size.
Two different weight methods were implemented: original and adjusted weight. Original weight is the raw weight of the specimens excavated, while adjusted weight standardizes this measure to account for variability in excavated sediment volume per spit. Throughout this paper we will now refer to this original weight as the “weight” while the adjusted weight is referred to as the “adjusted weight” (for a more detailed explanation of the method of adjusted weight applied in this study see Kaharudin et al. (Citation2019) and Kealy et al. Citation2020).
Pearson’s Correlation Coefficient was applied to measure the linear association between the quantification methods and faunal abundance variables. This analysis can show either a positive or negative correlation between variables. A significant positive correlation (P < 0.05) was recovered between all three methods (NISP, MNI, and weight), with the strongest correlation between NISP and weight, and weakest between MNI and weight (Supplementary Information 1/S1), indicating that comparisons between the three methods would produce comparable results. Pearson correlation coefficient was also used to compare the Makpan sea urchin remains with all other faunal groups identified from the assemblage (from Kealy et al. Citation2020), to measure the association between the exploited fauna and changing patterns of subsistence. As this initial correlation analysis identified two distinct groupings within the data, we ran a principal component regression analysis of the faunal weights to separate spits into two separate groups upon which we re-ran the correlation analysis (S3).
Results
Sea urchin overall abundance
A total of 13,532.74 g of sea urchin remains were recovered from Makpan Square B (S1). Spines are the most abundant body part (weight = 8891 g; NISP = 394,832), followed by test fragments (w = 4130 g; NISP not counted). Each element of Aristotle’s lantern was recovered, namely demipyramids (w = 326 g; NISP = 7510), epiphyses (w = 42 g; NISP = 3280), rotulae (w = 32 g; NISP = 2400), teeth (w = 1.86 g; NISP = 175), and compass elements (w = 0.18 g; NISP = 22). Lastly, other elements including the auricle fragments (w = 107 g; NISP = 4011), and madreporite (w = 0.08 g; NISP = 4) were identified. The majority of the spines, tests, auricle, and teeth are fragmented, while the majority of the demipyramids, epiphyses, rotulae, and madreporite plates are largely complete (S6).
The assemblage demonstrates sea urchin exploitation at Makpan since the Late Pleistocene (). This is the oldest known record for sea urchin consumption in an archaeological context. Our analysis () shows sea urchin exploitation was high during the initial occupation of the site in the Late Pleistocene (Phase 1/P1) comprising 14.59% of the total faunal assemblage or 1689.08 g, compared with the Terminal Pleistocene (P2) with 11.76% of the total faunal assemblage or 5806.94 g, and (P3) the Pleistocene–Holocene transition with 3.18% of the faunal assemblage or 4372.54 g. Intensity of urchin exploitation decreases significantly during the Early–Middle Holocene (P4) with 1.66% of the total faunal assemblage or 1241.35 g and in the Neolithic–Historic phase (P5) where it comprises merely 1.33% of the total faunal assemblages or 422.83 g (). Among the five phases of occupation the overall sea urchin consumption, based on weight, is highest in P2. However, when viewed in relation to the overall weight of faunal remains (including all non-sea urchin fauna) recovered from this phase, sea urchin in P2 is proportionally less abundant than in P1. Similarly, sea urchin abundance in P2 and P3 is not massively different by weight, but very different as a percentage of the total faunal assemblage, with urchin significantly lower during P3. This is due to the major increase in the exploitation of other fauna in P3.
The ratio of total sea urchin weight to MNI provides a rough estimate of individual sea urchin weight, a proxy for relative sea urchin size for each spit. This ratio would suggest sea urchin size peaked in P3 (). A Kruskal–Wallis test comparing the weight per sea urchin per spit indicated that sea urchin size changed significantly between each of the five occupation periods (H = 32.4205, P < 0.05). This roughly correlates with the natural differences in size in the various sea urchin taxa identified and their changing abundance throughout the assemblage, however changes in size may also reflect changing availability of mature adults.
Seven distinctive sea urchin taxa were identified (based on their spines) as most commonly consumed at Makpan ( and Supplementary material, Figure S5): Diadematidae indet. (NISP = 199), Salmacis spp. (NISP = 827), Colobocentrotus atratus (NISP = 556), Tripneustes gratilla (NISP = 3880), Heterocentrotus mamillatus (NISP = 21,465), Echinometra spp., (NISP = 124,131), and Stomopneustes variolaris (NISP = 240,135). Both S. variolaris and Echinometra spp. are the taxa with the highest abundance, followed by H. mamillatus and T. gratilla. Family Diadematidae is a group of sea urchins with particularly fragile test and spines. The high morphological similarity of many genera and species within this group (e.g., Coppard and Campbell Citation2006) make it very difficult to attribute disarticulated and fragmented remains to genera or species. In addition to these seven, a small number of remains of M. globulus (NISP = 3) and Eucidaris metularia (NISP = 1) were also identified in the assemblage (S4–5).
Figure 4. Weight (top) and NISP (bottom) of the seven major identified taxa recovered from Makpan. NISP is only based on the spine element. x-axis is not standardized across taxa.
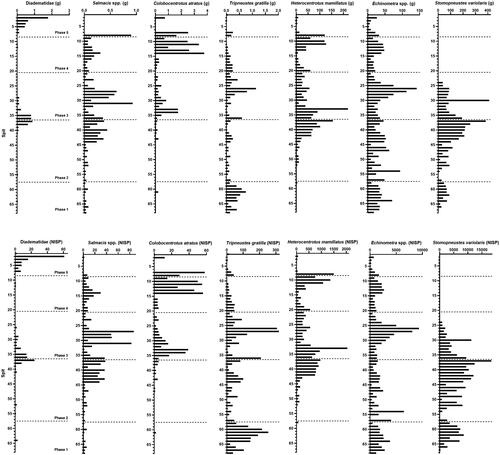
Thus, the Makpan assemblage also recovered the greatest diversity of sea urchins identified in a single archaeological context published so far. The diversity of sea urchins recovered from Makpan indicates that extensive coastal habitats were exploited. Most sea urchin taxa identified at Makpan inhabit the intertidal and subtidal zones or seaward slopes/reef ( and Supplementary material, Figure S2). All sea urchin taxa recovered at Makpan are also well-known as edible varieties (Archana and Babu Citation2016; Field, Lipphardt, and Kirch Citation2016; Murzina et al. Citation2021). While the spines of most species of Diadematidae are known to be poisonous, they are not fatal to humans, and Diadematidae are recorded as eaten by humans (Grisolía, López, and de Dios Ortúzar Citation2012; Szabó, Vogel, and Anderson Citation2012).
Figure 5. Percent NISP of the seven major identified taxa recovered from Makpan. NISP is only based on the spine element. Color spectrum approximately corresponds to habitat/depth (S2).
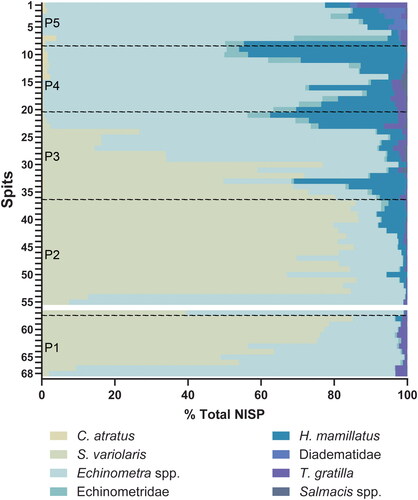
In general, there are two groups of sea urchin taxa at Makpan, based on their patterns of abundance. Group one consists of S. variolaris, Echinometra spp., and T. gratilla, and can be found since P1 and throughout most later periods of occupation. Group two (H. mamillatus, C. atratus, Salmacis spp., and Diadematidae) appears in the assemblage at the end of P2 ( and ). The increase in sea urchin diversity during P2, continuing toward P3, corresponds with the period of most intensive occupation of Makpan as indicated by a significant increase in shellfish, barnacle, crab, and fish exploitation (Kealy et al. Citation2020). This increase in urchin diversity in P2 may partly result from the larger urchin sample size for this phase which accounts for 42.9% by weight or 48.8% by MNI of the total sea urchin abundance in Makpan ().
Phase 1 (P1): initial occupation/the Late Pleistocene (∼43–15 ka)
The initial phase of occupation at Makpan recovered the lowest densities of faunal remains overall, with a small assemblage consisting mostly of shellfish, barnacle, sea urchin, and some terrestrial fauna—mostly rodents including a species of giant rat (Kealy et al. Citation2020; Louys et al. Citation2018). In contrast to the other marine resources found throughout the Makpan sequence, sea urchin exploitation is high in this earliest phase (MNI = 317; w = 1689.08 g), suggesting a greater focus on this resource immediately following initial occupation. Sea urchin makes up 14.59% of the total weight of faunal remains () in the phase. Taxonomic variability is generally limited in this early phase with a focus on S. variolaris (NISP = 47,907) and Echinometra spp. (NISP = 22,650). T. gratilla (NISP = 1358), H. mamillatus (NISP = 112), Salmacis spp. (NISP = 30), Diadematidae (NISP = 3), and C. atratus (NISP = 2) are present but in exceptionally small quantities. The P1 sea urchin assemblage suggests exploitation was mostly concentrated on the rocky intertidal zone with a focus on the easily accessed rock pools located above the low tide level, but with some exploration of seagrass habitats and out into waters above the reef (up to 30 m deep; see S2).
Phase 2 (P2): the terminal Pleistocene (∼14–12 ka)
An increase in occupation intensity at Makpan during the terminal Pleistocene is indicated by an increase in sedimentation rate and higher abundance of faunal remains recovered. Significant increases in terrestrial fauna, shellfish, barnacles, and sea urchins are recorded, while a minor increase is observed for fish and crab. This phase also recovered oldest shell artifacts including fishhooks and beads (Kealy et al. Citation2020; Langley et al. Citation2023a, Citation2023b). During this ∼3000-year phase of occupation, sea urchin exploitation reaches its highest intensity (MNI = 465; w = 5806.94 g). S. variolaris (NISP = 139,863) followed by Echinometra spp. (NISP = 32,736) are the dominant taxa ( and ), indicating a focus on rocky shore gleaning above the low tide level (S2).
A distinct increase in occupation intensity occurs toward the end of this phase (Kealy et al. Citation2020). Sea urchin follows this trend, increasing through P2. Some taxa such as Salmacis spp. (NISP = 273) and H. mamillatus (NISP = 6052) show a major rise in abundance toward the end of P2, continuing into the next phase. These shifts in intensity of sea urchin exploitation suggest a possible extension of coral reef ecosystems accessible by Makpan’s early inhabitants, toward the latter part of P2 (S2).
Phase 3 (P3): the Pleistocene–Holocene transition (∼12–11 ka)
The Pleistocene–Holocene transition is the most intensive phase of occupation recorded at Makpan, with a dense shell midden and multiple hearths throughout. This phase is marked by a decline in terrestrial fauna and a rise in most marine resources. Fish abundance by weight in particular soars and is associated with large numbers of fishhooks (Langley et al. Citation2023a). A major increase in shellfish, barnacle, and crab is also observed (Kealy et al. Citation2020). In contrast with these other marine resources, sea urchin exploitation shows a decline from the previous phase (MNI = 115; w = 4372.54 g). While as a proportion of total fauna sea urchin is much lower (down to 3.18%, ), the taxonomic diversity of sea urchins is highest in this phase compared to all other phases. Echinometra spp. (NISP = 46,771), H. mamillatus (NISP = 8302), Salmacis spp. (NISP = 386), and T. gratilla (NISP = 1423) reach their highest proportions, with a significant increase also seen for C. atratus (NISP = 168). Diadematidae (NISP = 49) and E. metularia (NISP = 1) are also recovered in this phase. This overall decline in sea urchins is driven by the decline in S. variolaris (NISP = 52,298) toward the end of P3. In contrast, Echinometra spp. increases notably to reach its peak in this phase (). The higher taxonomic diversity in the phase indicates exploitation of a wider range of habitats with Makpan’s inhabitants clearly foraging across all reaches of the littoral zone (S2).
Phase 4 (P4): the Early–Middle Holocene (∼10–7 ka)
P4 sees a decline in overall occupation intensity at Makpan, following the peak in P3 (Kealy et al. Citation2020). This decline is seen also in the sea urchin assemblage (MNI = 44; w = 1241.35 g). While intensity declines from the previous phase, the site’s occupants remained highly dependent on marine resources, considering the amount of fish, shellfish, barnacle, urchin, and crab remains recovered. Of all the marine fauna, crabs are the only group that show a relative increase in exploitation from the previous phase. There is a very slight increase in exploitation intensity toward the end of this phase (∼7 ka) portrayed by all marine resources except for fish (Kealy et al. Citation2020). This increase is also clearly observed in the sea urchin weights, but less evident from the MNI counts (). Echinometra spp. (NISP = 17,461), C. atratus (NISP = 266), and H. mamillatus (NISP = 5312) are the taxa that show an increase toward the end of P4 ( and ), while Salmacis spp. (NISP = 128), S. variolaris (NISP = 61), M. globulus (NISP = 3), and Diadematidae (NISP = 2) are present in small quantities.
Phase 5(P5): the Neolithic–Historic (∼4 ka–present)
This final occupation phase corresponds with the pottery-rich layers, the appearance of domestic animals, and an abundance of charcoal. During the first half of this phase (∼3–2 ka) all faunal groups are low, but then increase from ∼1 to 0.5 ka. Crabs show the most significant increase, peaking at the end of the phase (Kealy et al. Citation2020). Sea urchins are the only faunal group that do not increase toward the end of P5 (MNI = 12; w = 422.83 g). Echinometra spp. (NISP = 4513), Salmacis spp. (NISP = 10), C. atratus (NISP = 100), T. gratilla (NISP = 102), H. mamillatus (NISP = 1687), and S. variolaris (NISP = 3) were recovered but in smaller numbers than previous phases. Interestingly, Diadematidae (NISP = 106), which was rarely found in the previous phases, was common throughout this phase (). The broad range of habitats covered by the different sea urchins from this phase (S2), in addition to their low density, supports a shift away from the more focused sea urchin exploitation seen in previous phases.
Comparison with other fauna
Besides sea urchins, an abundance of other marine resources was recovered at Makpan, including fish, shellfish, barnacles, and crabs. Other vertebrate fauna were characterized by Kealy et al. (Citation2020) into macro-vertebrate (giant rats, fruit bats, and turtles) and micro-vertebrate (small–medium rodents, microbats, lizards, snakes, small birds, etc.) based on their size. Changes in exploitation intensity of fauna throughout the occupation period were determined by the different faunal category weights recovered per spit (see Kealy et al. Citation2020).
Pearson correlation coefficient was used to compare sea urchin abundance with every other fauna recovered from Makpan, to measure the strength in association (or disassociation) among the exploited fauna (Supplementary material, Figure S3.1 and Table S3.1). The two distinct groupings of correlation across the assemblage identified by the PCR showed a substantial degree of overlap with the five previously identified phases of occupation (). Pearson’s correlation of Group B (: Blue), correlating with P1, P2, and the beginning of P5, indicated a moderate (r = 0.5–0.7) positive correlation between urchins and shellfish, barnacle, macro-, and micro-vertebrates, and no significant correlation (P > 0.05) between sea urchins and fish or crab (). Group A (: Purple), correlating with P3, P4, and the end of P5, indicated a strong (r = 0.7–0.8) correlation between sea urchins and shellfish, barnacle, and fish, with no significant correlation with other vertebrates or crab.
Figure 6. Linear regression of Makpan terrestrial (micro- and macro-vertebrate) and marine (shellfish, barnacle, fish, and crab) fauna compared to sea urchin assemblage by weight (g) for each spit. Results of the statistical analyses (Pearson’s r) of the Makpan sea urchin assemblage by spit (n = 68) comparing to the other faunal groups, subdivided into Group A (positive PC score, Purple) and Group B (negative PC score, Blue). The “r” indicates “Pearson’s correlation coefficient.” Bar at bottom shows Groups A and B correlation to Phase 1–5. See Supplementary Information S3 and S7 for further results of this analysis.
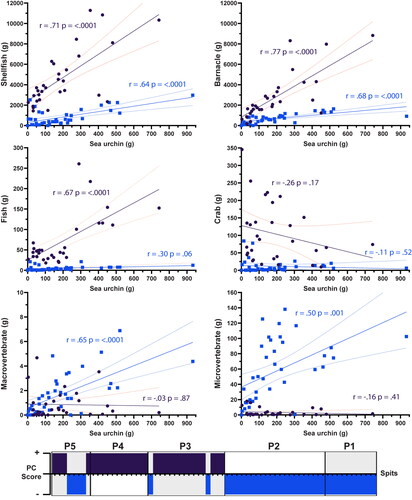
Comparison with barnacle recovered the highest Pearsons r value for each group (), suggesting that sea urchins and barnacles shared the most similar patterns in exploitation focus and intensity throughout the different occupation periods at Makpan. Shellfish are the only other taxa with a significant positive correlation to sea urchin in both groupings, supporting shared patterns in the exploitation of these three types of sessile marine invertebrates. Interestingly, the macro- and micro-vertebrate assemblages showed a very different pattern of correlation to the other fauna, with the strongest correlation in Group B rather than Group A. This result suggests a shared exploitation intensity between terrestrial vertebrates and sea urchin in P1 and P2, which appears to have been largely replaced by fish in the latter phases.
In contrast to the other faunal groups, crabs did not recover any significant correlation for either Group A or B. The weak negative correlation indicated for Group A, r(66) = −0.26, P = 0.17, may be somewhat related with the predator–prey association between the two (Breen and Mann Citation1976; Tarr, Williams, and Mackenzie Citation1996), directly or indirectly as waterborne cues from crabs can also disturb growth rate in sea urchins (Selden, Johnson, and Ellers Citation2009) making them less desirable for consumption. However, as the crab P-value is greater than 0.05, this result is not statistically significant (; Supplementary material, Table S3.2–3).
Discussion
Sea urchins and reef gleaning
Reef gleaning is an activity that can be performed by anyone, including men, woman, children, and the elderly. Utilizing the tidal cycle, it is often conducted in the early morning or evening at low tide. Each trip may target one, or multiple, types of fauna including fish, moray eel, shrimp, crabs, shellfish, octopus, squids, sea urchins, sea cucumbers, and peanut worms (De Guzman Citation2019; Tilley et al. Citation2021). Unlike fishing, that involves complex technology (e.g., watercraft, hooks, spears, lines, traps, and/or nets), and in some places is associated with spiritual beliefs, taboos, and gendered activities, reef gleaning only requires simple sticks, baskets, or just bare-hands (Chapman Citation1987). Reef gleaning also has a higher success rate for providing food, making it often a more reliable strategy than fishing (Tilley et al. Citation2021). Sea urchins are still targeted during gleaning trips at low tide on Alor’s neighboring islands including Pantar and Timor (; Fitriana and Stacey Citation2012; Tilley et al. Citation2021). Sea urchins are not as regularly suitable for consumption as shellfish or other sessile invertebrates however, as the only edible portion of the animal (their gonads/roe) are inherently linked with the reproductive cycle (Rocha et al. Citation2019; Smith and Berkes Citation1991).
Figure 7. Sea urchin (likely T. gratilla) harvested by reef gleaning during low tide in Timor-Leste, predominantly women and children (O’Connor, Pannell, and Brockwell Citation2013: ).
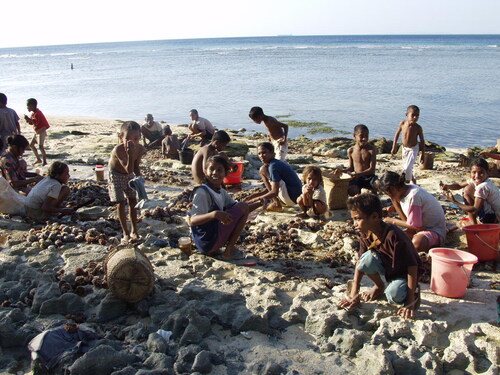
Barnacles and shellfish both recovered significant positively correlated patterns of exploitation to sea urchins in the Makpan record (). Similar to sea urchins, barnacles and shellfish are sessile coastal resources that can be easily exploited without complex hunting techniques. They share the same habitat, and all three faunal groups could have been targeted during a single reef gleaning trip. Barnacle and sea urchin in particular dominate the Makpan diet during P1 and toward P3 (Kealy et al. Citation2020), with the most abundant sea urchin taxa inhabiting the intertidal zone alongside most barnacles (Balanus spp.).
Unlike these sessile resources, we see distinctly different patterns of correlation between fish, other vertebrates, and crabs. Our results would suggest that the first two phases of occupation at Makpan focused on coastal gleaning of sessile marine invertebrates and exploitation of a naive terrestrial faunal assemblage. In P3, when occupation intensity peaked and more advanced maritime technologies were developed, we see the intensification of fishing and a shift away from intensive sea urchin exploitation toward a more diversified maritime subsistence.
Sea urchin over-exploitation
Unfortunately, easily accessed food also comes with the risk of over-exploitation. Sea urchin populations are vulnerable to depletion without appropriate resource management. Uncontrolled and nonselective harvesting can easily disrupt their reproductive cycle, with flow-on effects to population resilience (Smith and Berkes Citation1991). Over-exploitation is also a likely scenario for many prehistoric sites where we see high numbers of sea urchin exploited at initial occupation followed by a rapid decline. Some sea urchin taxa, including Echinometra, are tolerant of environmental stress and food competition but are very susceptible to predation and harvesting. Echinometra can easily colonize areas with minimum resources, but their nature of reproduction (broadcast spawning) means that they take longer to expand their population, since fertilization success is linked to abundance in most echinoids (see Lessios Citation1988; Levitan, Sewell, and Chia Citation1992). A short intense harvesting would likely impact these species and they would take a longer time to recover (McClanahan and Muthiga Citation2001).
While the greatest proportion of sea urchin remains were recovered from Makpan during P2 and P3, this corresponds with the increases in human occupation intensity and overall faunal exploitation across the site as a whole. P1, however, shows much more focused and intense sea urchin exploitation, despite much lighter occupation intensity, with a peak in MNI recovered from this phase (). The correlation between sea urchin and barnacle exploitation at Makpan, in particular the abundance of both in the earliest occupation phase, may be due to the fact that they are both sessile, and thus a predictable and easily targeted resource for coastal foragers arriving on new and unfamiliar shorelines. A similar case was also found at Here Sorot Entapa, Kisar, where sea urchin abundance is high during initial occupation (16–14.5 ka) alongside low levels of shellfish, barnacle, and crab remains (Kaharudin et al. Citation2019; O’Connor et al. Citation2019). This intense harvesting of sea urchins at initial occupation led Kaharudin et al. (Citation2019) and O’Connor et al. (Citation2019) to suggest that this was possibly the result of over-exploitation during initial occupation of novel environments by early seafaring communities. In the case of Makpan, when we look at the three popular taxa from P1 (S. variolaris, Echinometra spp., and T. gratilla), both T. gratilla and S. variolaris show a decline in exploitation at the beginning of P2, possibly reflecting the consequences of their over-exploitation in P1. S. variolaris in particular is even more vulnerable to continued harvesting than Echinometra spp., due to its slower growth and reproductive rates (Drummond Citation1993), supporting this hypothesis for its decline after P1.
In the Spanish Cantabrian region, nine prehistoric sites also show similar patterns where sea urchins are found early in the occupation record (since the Late Pleistocene), while other marine invertebrates (e.g., barnacles and crabs) were only exploited extensively from the Early Holocene (Gutiérrez-Zugasti Citation2011). Some Late Holocene sites occupied by the Pacific Islanders since ∼3–2 ka also indicate high exploitation of sea urchins during the initial occupation period followed by a significant decrease. This pattern could simply be the result of changing dietary preferences, which we do see in some areas of the Pacific and Indonesia today where some communities no longer even consider sea urchin to be part of their diet (Sand Citation1998; HAFK personal observation; HAFK personal communication from Stuart Bedford). However, sea urchin’s ease of harvesting and vulnerability to over-exploitation makes this hypothesis much more likely.
Sea urchins and ecological change at Makpan
Alternatively, changing intensities and focus on sea urchin harvesting during the different phases of occupation at Makpan may have been influenced by changing availability due to sea level and environmental fluctuations. In particular, the shift in exploitation of S. variolaris in Phases 1–3 to Echinometra spp. in Phases 4–5 ( and ), can be correlated with changing environmental conditions on Alor’s south coast.
Kealy et al. (Citation2020) modeled how sea level rise following the end of the LGM (Last Glacial Maximum) in the Late Pleistocene and into the Early Holocene may have greatly affected the population dynamics of local marine faunas due to the changing coastline of Alor and opening of the Pantar Strait. Post LGM, ∼22–16 ka, sea levels began to rise (Lambeck et al. Citation2014) at an average rate of ≤5 mm/year and accelerated to ∼46 mm/year during Meltwater Pulse 1 A (∼14.5–14 ka; Kim et al. Citation2023). After MWP1A, sea levels rose at ∼10 mm/year but increased rapidly to ∼22 mm/year during Meltwater Pulse 1B (∼11.5–11 ka; Kim et al. Citation2023). The steep bathymetric profile of Alor Island (Kealy et al. Citation2020) in conjunction with these dramatic changes in sea level, likely limited the establishment of stable ecosystems (in particular extensive coral reefs and sandy bays) during this period (Phases 1–3). Such conditions are supported by the abundance of S. variolaris, which is known to thrive in more disturbed and unpredictable rocky-shore ecosystems (Drummond Citation1993; Gayashan and Jayakody Citation2012).
Diversification in exploitation of sea urchins to incorporate more reefal habitats and deeper waters in the latter part of P2 and into P3 (i.e., increasing relative abundance of H. mamillatus, ), may reflect an expansion in availability of these systems. However, this increased diversification in sea urchin exploitation also corresponds with a significant increase in fish exploitation, of reef as well as pelagic species, which required more complex hunting technologies as reflected in the artifacts of fishing technology also recovered in this phase (Kealy et al. Citation2020; Langley et al. Citation2023a). Such changes indicate that Makpan’s inhabitants had developed advanced maritime subsistence strategies by this time, allowing them to exploit more diverse, changing, and deeper coastal environments, including a greater diversity of sea urchin taxa. These changes in habitat diversity and broadening exploitation focus were possibly driven by the continued rise in sea level and the creation of new/expanded habitats, which may have led to the intensification of occupation we see during this phase (Kealy et al. Citation2020). Alternatively, increasing population numbers of the local Makpan inhabitants at this time (Kealy et al. Citation2020) may have also driven this diversification in habitat exploitation and development of new maritime technologies.
It is toward the end of P3 that we see the shift away from S. variolaris dominating the assemblage to be replaced by Echinometra spp. as the most commonly exploited sea urchin taxa. This shift corresponds with the Holocene sea-level stabilization and establishment of the more stable ecosystems present along Alor’s south coast today. While the continued dominance of rocky-shore species highlights the prevalence of this ecosystem during all periods of occupation at Makpan, Echinometra spp. are known to preference a less intensely “survival-focused” life strategy than S. variolaris (Drummond Citation1993), supporting their abundance in the more established ecosystems of P4 and P5. However, as mentioned previously, while S. variolaris is better suited to unpredictable ecosystems, its slower reproductive strategy makes it more vulnerable to over-exploitation (Drummond Citation1993), which may have also occurred during P3 as a result of the overall increase in occupation intensity at Makpan during this time (Kealy et al. Citation2020).
Phases 4 and 5 also see steady increases in sea urchin diversity, reflecting both the increased diversity of available habitats but also likely an increased desire by people to harvest across a wider range of locations. This final period of occupation at Makpan is also marked by distinct changes in technologies and substance strategies (e.g., pottery and domesticates; Kealy et al. Citation2020), which may have influenced this diversification in exploited coastal ecosystems. While it is clear that environmental changes had a major impact on the availability of different sea urchin taxa at Makpan, it is also likely that changes in maritime technologies and harvesting methods influenced sea urchin exploitation. Furthermore, we consider intensive harvesting in P1 and P3, while not the sole event, likely combined with the dramatic environmental changes at the time to make local sea urchin populations particularly at risk of over-exploitation.
Sea urchin exploitation as a subsistence strategy
Discussions regarding non-mollusk invertebrate exploitation in archaeological contexts have debated whether they were intentionally targeted or only secondarily collected while people focused on harvesting shellfish (Gutiérrez-Zugasti et al. Citation2016). In relation to that debate, there is also the question of whether sea urchins were treated as a staple food source or rather a delicacy/occasional variety during prehistoric times (see Gutiérrez-Zugasti et al. Citation2016; Milner Citation2009a, Citation2009b). Sea urchin consumption at Makpan was high during the first three phases of occupation (Late Pleistocene ∼43–15 ka, terminal Pleistocene ∼14–12 ka, and toward the Pleistocene–Holocene transition ∼12–11 ka). More importantly for the question of targeted exploitation, the sea urchin assemblage at Makpan, when compared with other faunal remains, contributed a significant proportion of the diet of Makpan’s inhabitants during the first two phases of occupation.
During P1 and P2 at Makpan, terrestrial fauna, sea urchin, and some barnacle seem to have been the preferable sources for protein, with sea urchins particularly favored during initial occupation at Makpan. Given that barnacle and sea urchins could be easily collected together, but that sea urchins have a select window for collection, it is possible that sea urchins were the focus of the gleaning activity, with barnacle collected as the secondary/opportunistic resource. This is somewhat supported by the apparent broad range of barnacle size in the Makpan assemblage (HAFK personal observation) which would indicate a less focused strategy.
The Makpan record shows that sea urchin consumption can be substantial, seemingly regardless of abundance and availability of other marine invertebrates. It also strongly suggests that sea urchins were purposefully targeted, rather than acquired as a subsidiary resource during shellfish collection. The high and continuous abundance of urchin remains shows that sea urchin formed a regular staple part of the diet, especially during the early phases of occupation. The Makpan record suggests sea urchins were collected too intensively to have been just a minor or occasional resource, or delicacy in the diet of the early human inhabitants; perhaps even so intensively at both initial occupation and during P3 that it impacted the abundance and diversity of the local urchin population.
Conclusion
Sea urchin exploitation at Makpan was heavily influenced by the dynamics of occupation intensity as well as fluctuations in sea level and available habitat. Seven distinctive taxa were regularly consumed from ∼43 ka: Diadematidae, Salmacis spp., C. atratus, T. gratilla, H. mamillatus, Echinometra spp., and S. variolaris. Generally, sea urchins were more heavily exploited in the Late Pleistocene and consumption declined in the Holocene. During P1 (∼43–15 ka), sea urchins had a significant dietary role relative to the limited repertoire of other resources exploited. The emphasis on urchin exploitation and the taxonomic diversity of urchins increased during P2 (∼14–12 ka), in tandem with major increase in occupation intensity. In P3 (∼12–11 ka), quantities of urchin collected are still high but there is a relative decrease in their importance compared to other resources exploited, particularly fish, which was heavily exploited in this phase, coinciding with the appearance of fishhooks (Kealy et al. Citation2020). Sea urchin exploitation continued to decrease during the two final occupation phases, P4 (∼10–7 ka) and P5 (∼4 ka–present), in line with an overall decline in occupation intensity.
The high abundance of sea urchins recovered, their taxonomic diversity, and the continuity in their use throughout all occupation periods indicates that they were intentionally targeted as a major dietary resource by Makpan’s early inhabitants. Sessile, easily gathered resources, including barnacles, shellfish, and sea urchins, which can be collected from the intertidal zone, are the most commonly consumed fauna during initial habitation at the cave. Sea urchins, however, can be especially susceptible to over-exploitation and when there is intense reduction of the population, can take a long time to recover. Over-exploitation of the sessile, easily procured resources in close proximity to the site during the initial two occupation phases may have driven Makpan’s inhabitants to expand their coastal foraging tidal range and distance. The increase in urchin diversity during P3 suggests that Makpan’s early inhabitants explored a larger and more varied coastal ecosystem, corresponding with more intensive fishing and more complex fishing technologies. This may also have been in response to changing resource availability due to post-LGM sea level rise.
Supplemental Material
Download Zip (2.4 MB)Acknowledgements
Permission for the research was granted by the Indonesian government—RISTEK Foreign Research Permit (O’Connor1172/FRP/E5/Dit.KI/V/2016). Thanks to Dr Mahirta, Dr Widya Nayati, Dr Julien Louys, Dr Stuart Hawkins, Gendro Keling, and students from Universitas Gadjah Mada for their assistance in the field. We would also like to thank Yuni Suniarti and Devi M. Sari for their assistance during the preliminary sorting stage of the Makpan assemblages. Thank you to Dr Stephen Keable, for the access to the echinoid collection at the Australian Museum and Dr Cahyo Rahmadi, for their echinoid collection at Museum Zoologicum Bogoriense, LIPI. Our deepest gratitude to the landowners and villagers of Halmin and Ling Al for the help and our pleasant stay during Makpan excavation. Finally, we express our gratitude to the three anonymous reviewers whose comments have aided in improving this manuscript.
Supplemental Material
Supplemental S1. Method comparison: weight, MNI, and NISP. Supplemental S2. Sea urchins’ habitat details. Supplemental S3. Faunal comparison: Makpan sea urchin assemblage comparing to the other faunal groups. Supplemental S4. Sea urchins’ weight by element. Supplemental S5. Sea urchins Number of Identified Specimens (NISP) based on spines. Supplemental S6. Sea urchins elements for Minimum Number of Individuals (MNI). Supplemental S7. Principal Component (PC) score of Makpan faunal assemblages.
Disclosure statement
No potential conflict of interest was reported by the author(s).
Additional information
Funding
References
- Ainis, A. F. 2022. Using sea urchin remains to infer the health of kelp forest ecosystems in the past: Identification, quantification, and allometric reconstructions of harvested sea urchin (Strongylocentrotus spp.) on California’s Channel Islands. The Journal of Island and Coastal Archaeology 17 (2):246–73. doi:10.1080/15564894.2020.1774445
- Anderson, A. and D. J. Kennett, eds. 2012. Taking the high ground: The archaeology of Rapa, a fortified island in remote East Polynesia. Terra Australis 37. Canberra: ANU Press.
- Archana, A., and K. R. Babu. 2016. Nutrient composition and antioxidant activity of gonads of sea urchin Stomopneustes variolaris. Food Chemistry 197 (Pt A):597–602. doi:10.1016/j.foodchem.2015.11.003
- Boulanger, C., S. Hawkins, S. C. Samper Carro, R. Ono, and S. O’Connor. 2023. Continuity and variability in prehistoric fishing practices by Homo sapiens in Island Southeast Asia: New ichthyofaunal data from Asitau Kuru, Timor-Leste. World Archaeology 54 (2):288–316. doi:10.1080/00438243.2023.2192518
- Boulanger, C., T. Ingicco, P. J. Piper, N. Amano, S. Grouard, R. Ono, S. Hawkins, and A. F. Pawlik. 2019. Coastal subsistence strategies and mangrove swamp evolution at Bubog I Rockshelter (Ilin Island, Mindoro, Philippines) from the Late Pleistocene to the mid-Holocene. The Journal of Island and Coastal Archaeology 14 (4):584–604. doi:10.1080/15564894.2018.1531957
- Breen, P. A., and K. H. Mann. 1976. Changing lobster abundance and the destruction of kelp beds by sea urchins. Marine Biology 34 (2):137–42. doi:10.1007/BF00390755
- Bulmer, S. 2005. Reflections in stone: Axes and the beginnings of agriculture in the Central Highlands of New Guinea. In Papuan pasts: Cultural, linguistic and biological histories of Papuan-speaking peoples, ed. A. Pawley, R. Attenborough, J. Golson, and R. Hide, 387–450. Canberra: The Australian National University.
- Burney, D. A., and W. K. P. Kikuchi. 2006. A millennium of human activity at Makauwahi Cave, Māhāūulepū, Kauaūi. Human Ecology 34 (2):219–47. doi:10.1007/s10745-006-9015-3
- Campbell, G. 2008a. Sorry, wrong phylum: A neophyte archaeomalacologists experiences in analyzing a European Atlantic sea urchin assemblage. Archaeofauna 17:77–90.
- Campbell, G. 2008b. A preliminary study of methods for identifying archaeological sea urchin remains in the Pacific Northwest. Canadian Zooarchaeology 25 (15):15–35.
- Campbell, J. R. 2015. Development, global change and traditional food security in Pacific Island countries. Regional Environmental Change 15 (7):1313–24. doi:10.1007/s10113-014-0697-6
- Cannon, M. D. 1999. A mathematical model of the effects of screen size on zooarchaeological relative abundance measures. Journal of Archaeological Science 26 (2):205–14. doi:10.1006/jasc.1998.0317
- Carnevali, M. C., I. C. Wilkie, E. Lucca, F. Andrietti, and G. Melone. 1993. The Aristotle’s lantern of the sea-urchin Stylocidaris affinis (Echinoida, Cidaridae): Functional morphology of the musculo-skeletal system. Zoomorphology 113 (3):173–89. doi:10.1007/BF00394858
- Chapman, M. D. 1987. Women’s fishing in Oceania. Human Ecology 15 (3):267–88. doi:10.1007/BF00888026
- Coppard, S. E., and A. C. Campbell. 2006. Taxonomic significance of test morphology in the echinoid genera Diadema and Echinothrix. Zoosystema 28:93–112.
- Coyer, J. A., R. F. Ambrose, J. M. Engle, and J. C. Carroll. 1993. Interactions between corals and algae on a temperate zone rocky reef: Mediation by sea urchins. Journal of Experimental Marine Biology and Ecology 167 (1):21–37. doi:10.1016/0022-0981(93)90181-M
- De Guzman, A. B. 2019. Women in subsistence fisheries in the Philippines: The undervalued contribution of reef gleaning to food and nutrition security of coastal households. SPC Women Fisheries Bulletin 29:34–40.
- Dean, R. 2010. Delicacy or desperation? Eating peduncular barnacles in Neolithic Portugal. Journal of Ethnobiology 30 (1):80–91. doi:10.2993/0278-0771-30.1.80
- Dixon, B, M. Dega, D. Moore, and J. R. Amesbury. 2022. Archaeological research at the Early Pre-Latte Period site of San Roque on Saipan (ca. 1500–1100 BC). The Journal of Island and Coastal Archaeology. doi:10.1080/15564894.2022.2048284
- Drummond, A. E. 1993. Studies on the biology of three species of Sea urchin (Echinodermata: Echnoidea), on the South African East Coast. PhD diss., University of Natal.
- Duffin, C. J. 2006. Lapis Judaicus or the Jews’ stone: The folklore of fossil echinoid spines. Proceedings of the Geologists’ Association 117 (3):265–75. doi:10.1016/S0016-7878(06)80034-9
- Egami, T., and K. Kojima. 2013. Traditional whaling culture and social change in Lamalera, Indonesia: An analysis of the catch record of whaling. Senri Ethnological Studies 84:155–76.
- Erlandson, J. M., T. C. Rick, J. A. Estes, M. H. Graham, T. J. Braje, and R. L. Vellanoweth. 2005. Sea otters, shell, and humans: 10,000 years of ecological interaction on San Miguel Island, California. In Proceedings of the Sixth California Islands Symposium, ed. D. K. Garcelon and C. A. Schwemm, 58–69. Arcata, CA: Institute for Wildlife Studies.
- Field, J. S., P. V. Kirch, K. Kawelu, and T. N. Ladefoged. 2010. Households and hierarchy: Domestic modes of production in leeward Kohala, Hawai’i Island. The Journal of Island and Coastal Archaeology 5 (1):52–85. doi:10.1080/15564890903178663
- Field, J. S., J. N. Lipphardt, and P. V. Kirch. 2016. Trends in marine foraging in precontact and historic leeward Kohala, Hawai ‘i Island. Pacific Science 70 (3):287–307. doi:10.2984/70.3.2
- Fitriana, R., and N. Stacey. 2012. The role of women in the fishery sector of Pantar Island, Indonesia. Asian Fisheries Science Special Issue 25:159–75.
- Gayashan, M. A., and S. Jayakody. 2012. Diversity and density of sea urchins populations in rocky shores off Nilwella in Southern province of Sri Lanka. Sri Lanka Journal of Aquatic Sciences 17:35–46. doi:10.4038/sljas.v17i0.6854
- Ghosh, S., C. Jung, and V. B. Meyer-Rochow. 2016. Snail farming: An Indian perspective of a potential tool for food security. Annals of Aquaculture and Research 3 (3):1–6.
- Grisolía, J. M., F. López, and J. de Dios Ortúzar. 2012. Sea urchin: From plague to market opportunity. Food Quality and Preference 25 (1):46–56. doi:10.1016/j.foodqual.2012.01.004
- Gutiérrez-Zugasti, F. I. 2011. The use of echinoids and crustaceans as food during the Pleistocene–Holocene transition in Northern Spain: Methodological contribution and dietary assessment. The Journal of Island and Coastal Archaeology 6 (1):115–33. doi:10.1080/15564894.2010.487421
- Gutiérrez-Zugasti, F. I., E. Tong, A. García-Escárzaga, D. Cuenca-Solana, G. N. Bailey, and M. R. González-Morales. 2016. Collection and consumption of echinoderms and crustaceans at the Mesolithic shell midden site of El Mazo (northern Iberia): Opportunistic behaviour or social strategy? Quaternary International 407:118–30. doi:10.1016/j.quaint.2015.11.149
- Hamilton, S. L., and J. E. Caselle. 2015. Exploitation and recovery of a sea urchin predator has implications for the resilience of southern California kelp forests. Proceedings of the Royal Society B Biological Sciences 282 (1799):20141817. doi:10.1098/rspb.2014.1817
- Hawkins, S., S. O’Connor, T. R. Maloney, M. Litster, S. Kealy, J. N. Fenner, K. Aplin, C. Boulanger, S. Brockwell, R. Willan, et al. 2017. Oldest human occupation of Wallacea at Laili Cave, Timor-Leste, shows broad-spectrum foraging responses to late Pleistocene environments. Quaternary Science Reviews 171:58–72. doi:10.1016/j.quascirev.2017.07.008
- Hayden, B. 2003. Were luxury foods the first domesticates? Ethnoarchaeological perspectives from Southeast Asia. World Archaeology 34 (3):458–69. doi:10.1080/0043824021000026459a
- Judd, H. P. 1930. Hawaiian proverbs and riddles. Bernice P. Bishop Museum Bulletin 77. Hawaii: The Bishop Museum.
- Kaharudin, H. A. F., A. Alifah, L. Ramadhan, and S. Kealy. 2020. A review of archaeological dating efforts at cave and rockshelter sites in the Indonesian Archipelago. Journal of Indo-Pacific Archaeology 44:80–112.
- Kaharudin, H. A. F., G. A. R. Ananda, W. H. Prasetya, M. W. Wibisono, and J. S. E. Yuwono. 2023. Hunter-gatherers in labyrinth karst: An Early Holocene record from Gunung Sewu, Java. Archaeological Research in Asia 33:100427. doi:10.1016/j.ara.2022.100427
- Kaharudin, H. A. F., M. Mahirta, S. Kealy, S. Hawkins, C. Boulanger, and S. O'Connor. 2019. Human foraging responses to climate change; here Sorot Entapa rockshelter on Kisar Island. Wacana 20 (3):525–59. doi:10.17510/wacana.v20i3.783
- Kealy, S., J. Louys, and S. O’Connor. 2017. Reconstructing palaeogeography and inter‐island visibility in the Wallacean Archipelago during the likely period of Sahul colonization, 65–45 000 years ago. Archaeological Prospection 24 (3):259–72. doi:10.1002/arp.1570
- Kealy, S., S. O’Connor, D. M. Sari, C. Shipton, M. C. Langley, C. Boulanger, H. A. F. Kaharudin, E. P. B. G. G. Patridina, M. A. Algifary, A. Irfan, et al. 2020. Forty-thousand years of maritime subsistence near a changing shoreline on Alor Island (Indonesia). Quaternary Science Reviews 249:106599. doi:10.1016/j.quascirev.2020.106599
- Kim, H. L., T. Li, N. Kalsi, H. T. T. Nguyen, T. A. Shaw, K. C. Ang, K. C. Cheng, A. Ratan, W. R. Peltier, D. Samanta, et al. 2023. Prehistoric human migration between Sundaland and South Asia was driven by sea-level rise. Communications Biology 6 (1):150. doi:10.1038/s42003-023-04510-0
- Kirch, P. V., D. W. Steadman, V. L. Butler, J. Hather, and M. I. Weisler. 1995. Prehistory and human ecology in Eastern Polynesia: Excavations at Tangatatau rockshelter, Mangaia, Cook Islands. Archaeology in Oceania 30 (2):47–65. doi:10.1002/j.1834-4453.1995.tb00330.x
- Kirch, P., and S. J. O’Day. 2003. New archaeological insights into food and status: A case study from pre-contact Hawaii. World Archaeology 34 (3):484–97. doi:10.1080/0043824021000026468
- Kroh, A., and J. H. Nebelsick. 2003. Echinoid assemblages as a tool for palaeoenvironmental reconstruction—An example from the Early Miocene of Egypt. Palaeogeography, Palaeoclimatology, Palaeoecology 201 (1–2):157–77. doi:10.1016/S0031-0182(03)00610-2
- Lambeck, K., H. Rouby, A. Purcell, Y. Sun, and M. Sambridge. 2014. Sea level and global ice volumes from the Last Glacial Maximum to the Holocene. Proceedings of the National Academy of Sciences of the United States of America 111 (43):15296–303. doi:10.1073/pnas.1411762111
- Langley, M. C., S. Kealy, S. O’Connor, and Mahirta. 2023b. Sequins from the sea: The nautilus ornament technology of Makpan, Alor Island, Indonesia. Antiquity 97 (394):810–28 doi:10.15184/aqy.2023.97
- Langley, M. C., S. O’Connor, S. Kealy, and Mahirta. 2023a. Fishhooks, lures, and sinkers: Intensive manufacture of marine technology from the Terminal Pleistocene at Makpan Cave, Alor Island, Indonesia. The Journal of Island and Coastal Archaeology 18 (1):33–52. doi:10.1080/15564894.2020.1868631
- Lessios, H. A. 1988. Mass mortality of Diadema antillarum in the Caribbean: What have we learned? Annual Review of Ecology and Systematics 19 (1):371–93. doi:10.1146/annurev.ecolsys.19.1.371
- Levitan, D. R., M. A. Sewell, and F.-S. Chia. 1992. How distribution and abundance influence fertilization success in the sea urchin Strongylocentotus franciscanus. Ecology 73 (1):248–54. doi:10.2307/1938736
- Ling, S. D., C. R. Johnson, K. Ridgway, A. J. Hobday, and M. Haddon. 2009. Climate‐driven range extension of a sea urchin: Inferring future trends by analysis of recent population dynamics. Global Change Biology 15 (3):719–31. doi:10.1111/j.1365-2486.2008.01734.x
- Ling, S. D., R. E. Scheibling, A. Rassweiler, C. R. Johnson, N. Shears, S. D. Connell, A. K. Salomon, K. M. Norderhaug, A. Pérez-Matus, J. C. Hernández, et al. 2015. Global regime shift dynamics of catastrophic sea urchin overgrazing. Philosophical Transactions of the Royal Society B: Biological Sciences 370 (1659):20130269. doi:10.1098/rstb.2013.0269
- Louys, J., S. O’Connor, P. Higgins, S. Hawkins, T. Maloney, and Mahirta. 2018. New genus and species of giant rat from Alor Island, Indonesia. Journal of Asia-Pacific Biodiversity 11 (4):503–10. doi:10.1016/j.japb.2018.08.005
- Lowry, L. F., and J. S. Pearse. 1973. Abalones and sea urchins in an area inhabited by sea otters. Marine Biology 23 (3):213–9. doi:10.1007/BF00389487
- McClanahan, T. R., and N. A. Muthiga. 2001. The ecology of Echinometra. In Edible sea urchins: Biology and ecology, ed. J. M. Lawrence, 225–43. Amsterdam: Elsevier Science.
- McNamara, K. J. 2006. Star-crossed stones—The archaeology, mythology and folklore of fossil echinoids. Journal of the Royal Society of Western Australia 89 (29):83–5.
- McNamara, K. J. 2007. Shepherds’ crowns, fairy loaves and thunderstones: The mythology of fossil echinoids in England. In Myth and geology, ed. L. Piccardi and W. Masse, 279–94. London: Geological Society. doi:10.1144/GSL.SP.2007.273.01.22
- Milner, N. 2009a. Consumption of crabs in the Mesolithic, side stepping the evidence?. In Mesolithic and later sites around the Inner Sound, Scotland: The work of the Scotland’s First Settlers Project 1998–2004, ed. K. Hardy and C. Whickam-Jones, 401–7. Edinburgh: Scottish Archaeology Internet Publications.
- Milner, N. 2009b. Mesolithic consumption practices. Food for thought. Journal of Nordic Archaeological Science 16:49–63.
- Miskelly, A. 2002. Sea urchins of Australia and the Indo-Pacific. Sydney: Capricornica Publications.
- Mooi, R., and A. Munguia. 2014. Sea urchins of the Philippines. In The Coral Triangle: The 2011 Hearst Philippine Biodiversity Expedition, ed. G. C. Williams and T. M. Gosliner, 213–35. San Francisco: California Academy of Sciences.
- Murzina, S. A., P. Y. Dgebuadze, S. N. Pekkoeva, V. P. Voronin, E. S. Mekhova, and N. T. Thanh. 2021. Lipids and fatty acids of the gonads of sea urchin Diadema setosum (Echinodermata) from the coastal area of the Nha Trang Bay, Central Vietnam. European Journal of Lipid Science and Technology 123 (7):2000321. doi:10.1002/ejlt.202000321
- Muthiga, N. A. 2005. Testing for the effects of seasonal and lunar periodicity on the reproduction of the edible sea urchin Tripneustes gratilla (L) in Kenyan coral reef lagoons. Hydrobiologia 549 (1):57–64. doi:10.1007/s10750-005-4757-2
- Norman, K., C. Shipton, S. O’Connor, W. Malanali, P. Collins, R. Wood, W. M. Saktura, R. G. Roberts, and Z. Jacobs. 2022. Human occupation of the Kimberley coast of northwest Australia 50,000 years ago. Quaternary Science Reviews 288:107577. doi:10.1016/j.quascirev.2022.107577
- O’Connor, S. 1999. 30,000 years of aboriginal occupation in the Kimberley, North West Australia. Terra Australis 14. Canberra: ANU Press.
- O’Connor, S. 2015. Rethinking the Neolithic in Island Southeast Asia, with particular reference to the archaeology of Timor‑Leste and Sulawesi. Archipel 90 (90):15–47. doi:10.4000/archipel.362
- O’Connor, S., Mahirta, S. Kealy, C. Boulanger, T. Maloney, S. Hawkins, M. C. Langley, H. A. F. Kaharudin, Y. Suniarti, M. Husni, et al. 2019. Kisar and the archaeology of small islands in the Wallacean Archipelago. The Journal of Island and Coastal Archaeology 14 (2):198–225. doi:10.1080/15564894.2018.1443171
- O’Connor, S., R. Ono, and C. Clarkson. 2011. Pelagic fishing at 42,000 years before the present and the maritime skills of modern humans. Science 334 (6059):1117–21. doi:10.1126/science.1207703
- O’Connor, S., S. Pannell, and S. Brockwell. 2013. The dynamics of culture and nature in a “protected” Fataluku landscape. In Transcending the culture–nature divide in cultural heritage: Views from the Asia-Pacific region, ed. S. Brockwell, S. O’Connor, and D. Byrne, 203–33. Terra Australis 36. Canberra: ANU Press.
- O’Connor, S., S. C. Samper Carro, S. Hawkins, S. Kealy, J. Louys, R. Wood, and Mahirta. 2017. Fishing in life and death: Pleistocene fish-hooks from a burial context on Alor Island, Indonesia. Antiquity 91 (360):1451–68. doi:10.15184/aqy.2017.186
- Ohgaki, S. I., T. Kato, N. Kobayashi, H. Tanase, N. H. Kumagai, S. Ishida, T. Nakano, Y. Wada, and Y. Yusa. 2019. Effects of temperature and red tides on sea urchin abundance and species richness over 45 years in southern Japan. Ecological Indicators 96:684–93. doi:10.1016/j.ecolind.2018.03.040
- Ono, R. 2010. Ethno-archaeology and early Austronesian fishing strategies in near-shore environments. The Journal of the Polynesian Society 119:269–314.
- Ono, R., S. Soegondho, and M. Yoneda. 2009. Changing marine exploitation during late Pleistocene in Northern Wallacea: Shell remains from Leang Sarru Rockshelter in Talaud Islands. Asian Perspectives 48 (2):318–41. doi:10.1353/asi.2009.0002
- Petchey, F., and P. V. Kirch. 2019. The importance of shell: Redating of the To’aga site (Ofu Island, Manu’a) and a revised chronology for the Lapita to Polynesian Plainware transition in Tonga and Sāmoa. PLoS One 14 (9):e0211990. doi:10.1371/journal.pone.0211990
- Pfister, C. A., and A. Bradbury. 1996. Harvesting red sea urchins: Recent effects and future predictions. Ecological Applications 6 (1):298–310. doi:10.2307/2269573
- Roberts, P., J. Louys, J. Zech, C. Shipton, S. Kealy, S. S. Carro, S. Hawkins, C. Boulanger, S. Marzo, B. Fiedler, et al. 2020. Isotopic evidence for initial coastal colonization and subsequent diversification in the human occupation of Wallacea. Nature Communications 11 (1):2068. doi:10.1038/s41467-020-15969-4
- Rocha, F., A. C. Rocha, L. F. Baião, J. Gadelha, C. Camacho, M. L. Carvalho, F. Arenas, A. Oliveira, M. R. G. Maia, A. R. Cabrita, et al. 2019. Seasonal effect in nutritional quality and safety of the wild sea urchin Paracentrotus lividus harvested in the European Atlantic shores. Food Chemistry 282:84–94. doi:10.1016/j.foodchem.2018.12.097
- Samper Carro, S. C., S. O’Connor, J. Louys, S. Hawkins, and M. Mahirta. 2016. Human maritime subsistence strategies in the Lesser Sunda Islands during the terminal Pleistocene–early Holocene: New evidence from Alor, Indonesia. Quaternary International 416:64–79. doi:10.1016/j.quaint.2015.07.068
- Sand, C. 1998. Recent archaeological research in the Loyalty Islands of New Caledonia. Asian Perspectives 37 (2):194–223.
- Selden, R., A. S. Johnson, and O. Ellers. 2009. Waterborne cues from crabs induce thicker skeletons, smaller gonads and size-specific changes in growth rate in sea urchins. Marine Biology 156 (5):1057–71. doi:10.1007/s00227-009-1150-0
- Seymour, S., N. A. Paul, S. A. Dworjanyn, and R. de Nys. 2013. Feeding preference and performance in the tropical sea urchin Tripneustes gratilla. Aquaculture 400–401:6–13. doi:10.1016/j.aquaculture.2013.02.030
- Sherman, E. 2015. Can sea urchins beat the heat? Sea urchins, thermal tolerance and climate change. PeerJ 3:e1006. doi:10.7717/peerj.1006
- Shipton, C., S. O’Connor, N. Jankowski, J. O’Connor-Veth, T. Maloney, S. Kealy, and C. Boulanger. 2019. A new 44,000-year sequence from Asitau Kuru (Jerimalai), Timor-Leste, indicates long-term continuity in human behaviour. Archaeological and Anthropological Sciences 11 (10):5717–41. doi:10.1007/s12520-019-00840-5
- Shipton, C., S. O’Connor, S. Kealy, I. N, Syarqiyah, N. Alamsyah, M. Ririmasse, and Mahirta. 2020. Early ground axe technology in Wallacea: The first excavations on Obi Island. PLoS One 15 (8):e0236719. doi:10.1371/journal.pone.0236719
- Smith, A. B., and A. Kroh, eds. 2011. The Echinoid Directory. World Wide Web electronic publication. Accessed February 02, 2020. http://www.nhm.ac.uk/research‐curation/projects/echinoid‐directory.
- Smith, A. H., and F. Berkes. 1991. Solutions to the “Tragedy of the commons”: Sea-urchin management in St Lucia, West Indies. Environmental Conservation 18 (2):131–6. doi:10.1017/S0376892900021706
- Steneck, R. S., M. H. Graham, B. J. Bourque, D. Corbett, J. M. Erlandson, J. A. Estes, and M. J. Tegner. 2002. Kelp forest ecosystems: Biodiversity, stability, resilience and future. Environmental Conservation 29 (4):436–59. doi:10.1017/S0376892902000322
- Szabó, K., Y. Vogel, and A. Anderson. 2012. Marine resource exploitation on Rapa Island: Archaeology, material culture and ethnography. In Taking the high ground: The Archaeology of Rapa, a fortified island in remote East Polynesia, ed. A. Anderson and D. J. Kennett, 145–66. Terra Australis 37. Canberra: ANU Press.
- Tarr, R. J. Q., P. V. G. Williams, and A. J. Mackenzie. 1996. Abalone, sea urchins and rock lobster: A possible ecological shift that may affect traditional fisheries. South African Journal of Marine Science 17 (1):319–23. doi:10.2989/025776196784158455
- Tilley, A., A. Burgos, A. Duarte, J. dos Reis Lopes, H. Eriksson, and D. Mills. 2021. Contribution of women’s fisheries substantial, but overlooked, in Timor-Leste. Ambio 50 (1):113–24. doi:10.1007/s13280-020-01335-7
- Titcomb, M., D. B. Fellows, M. K. Pukui, and D. M. Devaney. 1978. Native use of marine invertebrates in old Hawaii. Pacific Science 32 (4):325–91.
- Tye, D. 2011. Lobster tales: Narratives of food, past, and place in Maritime Canada. Cuizine 3 (1). doi:10.7202/1004730ar
- Uthicke, S., M. Liddy, H. D. Nguyen, and M. Byrne. 2014. Interactive effects of near-future temperature increase and ocean acidification on physiology and gonad development in adult Pacific sea urchin, Echinometra sp. A. Coral Reefs 33 (3):831–45. doi:10.1007/s00338-014-1165-y
- Valentin, F., G. Clark, P. Parton, and C. Reepmeyer. 2020. Mortuary practices of the first Polynesians: Formative ethnogenesis in the Kingdom of Tonga. Antiquity 94 (376):999–1014. doi:10.15184/aqy.2020.89
- Weisler, M. I., M. Mihaljević, and A. J. Rogers. 2020. Sea urchins: Improving understanding of prehistoric subsistence, diet, foraging behavior, tool use, and ritual practices in Polynesia. The Journal of Island and Coastal Archaeology 15 (4):547–75. doi:10.1080/15564894.2019.1679293
- Whyte, R., J. A. Hudson, S. Hasell, M. Gray, and R. O’Reilly. 2001. Traditional Maori food preparation methods and food safety. International Journal of Food Microbiology 69 (3):183–90. doi:10.1016/s0168-1605(01)00547-5