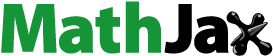
Abstract
In this study, we analysed the spatiotemporal characteristics of precipitation in Korea in connection with weakening of tropical cyclones (TCs) around the Korean Peninsula (32°–36° N, 122°–132° E) over the past 30 years (1979–2015). Weakened TCs were classified into two categories: TCs weakened to extratropical cyclones (WEC) and TCs weakened to tropical depressions (WTD). In WEC, precipitation was evenly distributed over all of the Korean Peninsula, with the greatest precipitation recorded on the southern coast. In WTD, the amount of precipitation was the greatest on the southern coast, while low precipitation was recorded in the central and inland areas of Korea. In Region 1 (Central Province, Jeollabuk-do, and the inland region of Gyeongsangbuk-do), greater precipitation was observed in WEC than in WTD. In Region 2 (Jeollanam-do, Gyeongsangnam-do, Southeast Gyeongsangbuk-do, and Jeju-do), little difference in precipitation was found between WEC and WTD. In WEC, potential vorticity developed in the upper-level troposphere with TCs approaching Korea along with a thermal trough in the lower-level troposphere to the northwest of the TCs. Meanwhile, an upper-level troposphere jet and a strong upper-level troposphere divergence region were observed to the northeast of the TCs. It was assumed that these meteorological factors had induced baroclinic disturbance and nondiabatic process, creating a wide precipitation area around the TCs. In contrast, in WTD, none of the intense potential vorticity, thermal trough, and upper-level troposphere jet was observed around the TCs. Consequently, only a narrow precipitation area was created around the TCs. The upper-level troposphere divergence region was relatively small, and no intense precipitation area was formed around the TCs.
1. Introduction
When a tropical cyclone (TC) moves north to mid-latitudes and then weakens, it reduces to a tropical depression (TD) or an extratropical cyclone (EC). A TD is defined as a TC that shares the same thermal and dynamic properties as typhoons but has a maximum sustained wind speed of less than 17 m s−1 around the centre. Meanwhile, an EC has completely different thermal and dynamic properties from those of typhoons. The weakening of a TC to an EC is referred to as extratropical transition, and it is often treated differently.
Previous studies have researched the concept of extratropical transition. Sekioka (Citation1956a, Citation1956b) and Matano and Sekioka (Citation1971) reported that while heading north, TCs interact with a mid-latitude trough or front and are then weakened to ECs at that front. Since then, with the introduction of the three-dimensional concept, it was found that both upper-level troposphere divergence and potential vorticity advection around TCs play a significant role in the TC to EC transition (DiMego and Bosart, Citation1982a, Citation1982b; Sinclair, Citation1993; Foley and Hanstrum, Citation1994; Klein et al., Citation2000; Harr et al., Citation2000; Ritchie and Elsberry, Citation2007; Rantanen et al., Citation2020). Another study showed that an extratropical transition occurs when cold and dry air are introduced to the warm and humid centre of a TC, resulting from the interaction between the TC and stratosphere potential vorticity (Browning et al., Citation1998; Yuan and Wang, Citation2014).
Other studies have investigated the distribution and characteristics of precipitation associated with extratropical transition. Shi et al. (Citation1997) reported that when hurricane Florence (1988) moved north to mid-latitudes, an upper-level troposphere jet and an upper-level troposphere trough were present to the northeast of the hurricane, which strengthened ascending flows and increased the relative humidity around the hurricane, resulting in strong precipitation intensity. In general, when a TC degrades to an EC, the precipitation intensity tends to be weakened but the precipitation range tends to become wider (Harr and Elsberry, Citation2000; Skok et al., Citation2013). However, if the weakened EC is re-enforced, strong precipitation intensity can be sustained over a wide area for a certain period of time (Ritchie, Citation2005). Yang and Oh (Citation2001) conducted a study on TC-related precipitation in Korea. They sorted every TC case that has affected Korea from 1996 to 2000 by regions of Korea according to the amount of precipitation, and they characterized the lower- and upper-level troposphere convergence and divergence, and western North Pacific subtropical high. There are other studies on the relationship between the characteristics of the large-scale environments around TCs and heavy precipitation in the Yeongdong region of Kangwon Province and the east coastal region of Gyeongbuk Province (Kim and Yoo, Citation2002; Jang, Citation2002). Meanwhile, Kwon and Kim (Citation2005) showed that the development of potential vorticity to the northwest of a TC around Korea and the penetration of the dry region could cause the weakening of the TC into an EC, leading to high precipitation.
In the current study, Typhoon Mindulle (T0407) in 2016 was selected as a case study, in which only the precipitation around the typhoon was analysed yet precipitation within Korea was not considered. This study aims to investigate the spatiotemporal distribution of precipitation associated with the weakening of TCs around Korea. First, 25 TCs weakened around Korea over the last 37 years (1979–2015) are classified into TCs weakened and transformed to ECs (WEC) and TCs weakened into TDs (WTD) then disappeared. The characteristics of individual TCs are compared, and then the spatiotemporal distribution of precipitation is compared and analysed. Next, the characteristics of individual rainfalls are explained in relation to the large-scale environments, and then the validity of the analysis conducted in the previous section is determined based on the case studies of WEC and WTD. Finally, the results of this study are summarized.
2. Data and methods
2.1. Data
TC data were supplied from the Regional Specialized Meteorological Centers, Tokyo Typhoon Center (http://www.jma.go.jp/jma/jma-eng/jma-center/rsmc-hp-pub-eg/trackarchives.html). The data consisted of names of TCs, location of latitude and longitude, minimum central pressure, and maximum sustained wind speed (MSWS), which were observed every 6 h. TC intensity was classified into four stages according to MSWS: tropical depression (TD, MSWS < 34 knots kts]), tropical storm (TS, 34 kts ≤ MSWS ≤ 47 kts), severe tropical storm (STS, 48 kts ≤ MSWS ≤ 63 kts), and typhoon (TY, MSWS ≥ 64 kts).
Data obtained from 61 weather observation stations () affiliated with the Korea Meteorological Administration (KMA) were used as the precipitation data for Korea. We analysed the 500 hPa geopotential height, 200 hPa jet stream, 200 hPa divergence region, 850 hPa convergence region, 300–200 hPa potential vorticity, 850 hPa air temperature, and vertical flows using the reanalysis data set of the National Center for Environmental Prediction–National Center for Atmospheric Research, which was composed of the latitude/longitude and time data with the spatiotemporal resolution at intervals of 2.5° × 2.5° and 6 h, respectively (Kalnay et al., Citation1996; Kistler et al., Citation2001; http://www.esrl.noaa.gov). In addition, we analysed the distribution of the precipitation intensity around both Typhoon Mindulle (T0407) WEC and Typhoon Neil (T9905) WTD using the precipitation intensity dataset of the Tropical Rainfall Measuring Mission (TRMM) satellite at intervals of 0.25° × 0.25° and 3 h (http://trmm.gsfc.nasa.gov).
2.2. Classification of weakened TCs
Of the TCs that have occurred in the western North Pacific over the last 37 years, 25 weakened around Korea (32°–36°N, 122°–132°E). These 25 TCs were classified into TCs WEC (14 cases) and TCs WTDs (11 cases; ). For individual cases, the spatiotemporal distribution of precipitation in Korea was compared and analysed.
Fig. 2. Points of tropical cyclones (TCs) weakened around Korea (32–36°N, 122–132°E) during the period 1979–2008, and their tracks for (a) WEC (weakened to extratropical cyclone), (b) WTD (weakened to tropical depression).

The examples used as WEC and WTD are shown below. The bold sections represent the TC-weakened sections used in this study.
WEC (weakened to extratropical cyclone)
TY (typhoon) -> STS -> TS -> EC -> Dissipation
TY -> STS -> TS -> TD -> EC -> Dissipation
WTD (weakened to tropical depression)
TY -> STS -> TS -> TD -> Dissipation
2.3. Definition of the TC-approaching time point toward Korea
When a TC is heading north toward Korea, the time point at which it first crosses 32°N was defined as the affecting time point in Korea (). Twelve hours before this time point was defined as the TC-approaching time point. The affecting time points were defined using the latitude of 32°N as a reference in consideration of the ranges of the latitudes of 32°–40°N and the longitudes of 120°–138°E (KMA, Citation1996), which are used as the references for typhoons affecting Korea. In this study, the differences in the large-scale environments that appear at the approaching time points of individual WEC and WTD cases were compared, and then the relationship between such differences and the precipitation distribution patterns was analysed.
Table 1. Definition of affecting time point and approaching time point of TC.
3. Characteristics of TCs weakened around Korea
3.1. Weakening time point of TCs
The average weakening time point for TCs that belong to WEC was +7.7 h from the affecting point in Korea and for WTD was +24.0 h (). The weakening time point of the WTD type was significantly later than that of the WEC type.
Table 2. Weakened time point of all TCs for WEC and WTD.
3.2. Traveling path of TCs
In WEC, the average traveling path of TCs approaching Korea is moving northeast from Shanghai, China, crossing Korea, and then exiting to the East Sea (). In WTD, the average traveling path of TCs approaching Korea is moving northwest from the south sea of Kyushu, Japan, neither crossing Korea nor exiting to the East Sea (). There was a clear difference in the 500 hPa geopotential height at the TC-approaching time point (–12 h). In WEC, the subtropical ridge lined up from the east of the TC to the southeast–northwest direction, and a trough was clearly observed to the west of the TC (). That is, the traveling path of the TC was directed north-eastward. By contrast, in WTD, the subtropical ridge lined up from the northeast of the TC to the east–west direction, and no trough was seen (). This pressure system pattern may slow the traveling speed of the TC.
3.3. Traveling speed of TCs
When a TC of the WEC type degrades to an EC, it moves northeast at a rapid speed due to mid-latitude westerlies (). When a TC of the WTD type degrades to a TD, it moves at a slow speed under the influence of the ridge around the TC rather than of mid-latitude westerlies (). The average traveling speed of the TC of the WEC type is 33 m s−1 for 45 h from the TC-approaching time point (–12 h), and the average traveling speed of the TC of the WTD type was 15 m s−1, indicating that the traveling speed was twice as fast in WEC than in WTD.
3.4. Monthly frequency of weakened TCs
As shown in the characteristics of the mean field of 500 hPa geopotential height, the subtropical ridge that appeared in the north of 35°N in WTD represents the pressure system pattern of mid-summer (). In fact, the weakened TCs of the WTD type occurred only in July and August over the last 37 years and most frequently in August (). In contrast, the incidence of WEC was lowest in August and highest in June and September, appearing evenly throughout June to October. In the next section, the effect of large-scale environments, which determine the distinction between WEC and WTD, on precipitation in Korea is analysed.
4. Spatiotemporal distribution of rainfall according to northbound traveling of TCs
4.1. Spatial distribution of rainfall
Analysis of the cumulative precipitation for 48 h from the TC-approaching time point (–12 h) revealed that in WES, precipitation was evenly distributed throughout Korea, with the highest cumulative precipitation in the southern regions (). The average precipitation measured from 61 weather observation stations in Korea was 63.3 mm, and the highest was 110.3 mm in Sancheong (47,289; ). In WTD, a large amount of precipitation was observed only in the southern regions and the eastern coastal regions, and very low precipitation was recorded in the central and inland regions (). The average precipitation from the 61 weather observation stations was 39.2 mm, and the highest was 115.0 mm in Goeje (47,294; ).
Fig. 5. Spatial distribution of rainfall accumulated over 48 h from approaching time point (–12 h) of TC for (a) WEC, (b) WTD and (c) their difference (WEC minus WTD). Dashed lines indicate negative value.

Table 3. Mean rainfall accumulated over 48 h at 61 weather observation stations from the approaching time point (–12 h) of TC and maximum rainfall of all the weather observation stations.
The difference in precipitation between WEC and WTD was most apparent in the central and inland regions (). In the central region, more precipitation was observed in WEC than in WTD, while in the southeastern region, including Busan in the centre, more precipitation was observed in WTD than in WEC (47,159). These differences in precipitation were tested through a Student’s t-test (at a significance level of 0.1; ). Stations marked with black circles indicate that significantly more precipitation was observed in WEC than in WTD, and stations marked with white circles indicate that there was no difference in precipitation between WEC and WTD. It was found that there was no station showing much higher precipitation in WTD than in WEC. Therefore, as shown in the figure, Region 1 was defined as regions with more precipitation in WEC than in WTD, and Region 2 was defined as regions with no difference in precipitation between WEC and WTD.
Fig. 6. Verification using Student’s t-test (0.1 significance level) for the rainfall difference shown in . Closed circles denote areas (Region 1) that there is a difference in rainfall while open circles denote areas (Region 2) that a difference in rainfall is similar.

Considering that a TC tends to get exposed more to the precipitation region when it moves slowly rather than quickly, more precipitation may result when TCs move slowly. However, although the traveling speed of TCs in WEC was faster than that in WTD, more precipitation was observed in WEC. Looking into the cumulative precipitation by latitude zone where the TC was located, in WTD, a greater precipitation was observed in Region 2 as TCs approached (), but a significantly smaller amount of precipitation was observed in Region 1 in WTD than in WEC, even though the cumulative precipitation time was longer in WTD than in WEC (). In other words, the precipitation in Region 1 had little correlation with the traveling speed or path of the TC. In the following section, the reasons why such differences in precipitation occur are identified.
4.2. Temporal distribution of rainfall
In WTD, it is evident that precipitation gradually increased in both Region 1 and Region 2 as a TC approached (). On the other hand, in WEC, a large amount of precipitation was concentrated in both Region 1 and Region 2 at the beginning (–12 h to +9 h) when the TC approached Korea. At this time, the distance between the TC and Korea was similar in WEC and WTD, nevertheless, much more precipitation was observed in WEC. In addition, in both Region 1 and Region 2, the precipitation peak time, whether the TC was WEC or WTD, was closer to the TC weakening time point than the time point at which the TC was closest to Korea (straight line in ). This seems to have been caused by the nondiabatic process, in which energy is converted to precipitation as the TC weakens. In the next section, the characteristics of potential vorticity advection, which represents the nondiabatic process well, and other large-scale environments are analysed by comparing WEC and WTD.
Fig. 8. Time evolution of 3-h interval accumulated rainfall (bars) from the approaching time point (–12 h) of TC to 48 h later for (a) Region 1 and (b) Region 2. Lines with an open circle indicate distances between the TC center and Seoul or Gwangju (star-marked point in embedded map). Solid vertical line indicates the mean time (+7.7 h) that TCs were weakened to EC and dashed vertical line indicates mean time (+24 h) that TCs were weakened to TD.

5. Large-scale environments that affect rainfall
5.1. Potential vorticity advection and upper-level troposphere divergence
The 300–200 hPa potential vorticity and 850 hPa thermal trough developed at the TC-approaching time point (–12 h) were located to the northwest of the TC in WEC, so that conditions that are favourable for the development of baroclinic disturbance and nondiabatic heating were formed around the TC (). In contrast, in WTD, neither potential vorticity nor thermal trough was observed around the TC (). Potential vorticity equation is as below.
Fig. 9. Potential vorticity of 300–200 hPa (shaded every 0.2 PVU starting at 0.4 PVU, where 1 PVU = 10−6 K kg−1 m2 s−1), 850 hPa air temperature (dashed lines, °C) and mean location of TCs at the approaching time point (–12 h) for (a) WEC and (b) WTD. Jet stream of 200 hPa (shaded every 5 m s−1 starting at 30 m s−1), 200 hPa divergence (thick contours, every 3 × 10−6 s−1 starting at 3 × 10−6 s−1) and 850 hPa convergence (thin contours, every –2 × 10−6 s−1 starting at –2 × 10−6 s−1) at the approaching time point (–12 h) for (c) WEC and (d) WTD. Dashed vertical line is used for the analysis of the vertical cross section in .

The front and ECs tend to develop further when energy is supplied from the upper-level troposphere jet and then amplified (Newton and Trevisan, Citation1984). In addition, when a TC approaches mid-latitudes, if the entrance and trough of the upper-level troposphere (200 hPa) jet stream are located to the northeast of the TC, the ascending flows become stronger in the centre of the TC and the relative humidity increases around the TC, resulting in strong precipitation intensity (Shi et al., Citation1997; Kim et al., Citation2006). On the other hand, Shapiro and Kennedy (Citation1981), Holton (Citation1992), and Ziv and Paldor (Citation1999) demonstrated that a divergence region was induced on the right side of the entrance of the upper-level troposphere jet stream through the ageostrophic factor and vorticity advection. When a TC degrades to an EC, it is usually accompanied by an upper-level troposphere jet, and an upper-level troposphere jet is accompanied to the northeast of the TC in WEC (). A strong divergence region was induced to the south of the entrance of the upper-level troposphere jet, which corresponded to all around the TC. Therefore, a heavy rainfall area was formed over a large area around the TC, and as a result, Korea was exposed to heavy rainfall for a long time and a large amount of precipitation was recorded. In contrast, in the WTD, neither an upper-level troposphere jet nor a strong upper-level troposphere divergence region was observed in WEC (). However, only a weak upper-level troposphere divergence was observed near the TC centre. Therefore, only a weak precipitation area was formed over a narrow area around the TC, and as a result, Korea was exposed to weak precipitation for a short time and only a small amount of precipitation was recorded.
As shown in , the incidence of WEC was higher in early summer and autumn than in mid-summer, while the incidence of WTD was rather higher in mid-summer, which seems to have resulted from the seasonal differences in the upper-level troposphere jet and western North Pacific subtropical high around Korea (not known).
5.2. Development of ascending flows around TCs
In WEC, ascending flows also developed strongly in a wide area around the TC due to the wide and strong divergence region around the TC (). On the other hand, in WTD, week ascending flows were observed in a narrow area due to the large-scale environments surrounding the TC that were responsible for continuous weakening of the TC ().
Fig. 10. Vertical cross section over the dashed vertical line in for (a) WEC and (b) WTD. Shaded area indicates ascending flow (hPa h−1). Thick lines and thin lines indicate divergence (10−6 s−1) and convergence (10−6 s−1), respectively. Square box indicates a latitude region where Korea is located.

These results suggest that in WTD, with the northbound movement of the TC, the precipitation area around the TC became narrower. With the influence of the surrounding large-scale environments causing weakening of the TC, more precipitation was observed in Region 2 also in Korea, which was closer to the TC, while a considerably small amount of precipitation was observed in Region 1, which was far from the TC. On the other hand, in WEC, with the northbound movement of the TC, a wide and strong precipitation area was formed around the TC, and with the influence of the surrounding large-scale environments around the TC, a large amount of precipitation was observed not only in Region 2 but also in Region 1 also in Korea. The actual precipitation areas around the TC while the TC was heading north is revealed in the next section.
6. Precipitation areas around the TC over time
Through the case studies of WEC and WTD, changes in the specific precipitation intensity area around the TC over time were analysed. The exemplary cases were chosen from TCs that occurred after 1998, of which data were provided by the TRMM satellite rainfall dataset. Typhoon Mindulle (T0407) and Typhoon Neil (T9905) were selected for WEC and WTD, respectively.
6.1. Distribution of precipitation areas
Looking at the precipitation areas around the typhoons at intervals of 3 h from the typhoon approaching time point, it is apparent that the precipitation area gradually decreased in Typhoon Neil (WTD; ). On the other hand, a wide precipitation area persisted around the TC in Typhoon Mindulle (WEC; ).
6.2. Ratio of specific precipitation intensities
shows a time-course illustration of the ratio of areas with specific precipitation intensities within an 800-km north radius from the centre of the typhoon to numerically determine the precipitation areas around each typhoon seen in and . In Typhoon Neil (WTD), all areas with precipitation intensities of 5 and 1 mm h−1 steadily decreased as the typhoon moved further north. In Typhoon Mindulle (WEC), the areas with precipitation intensities of 5 and 1 mm h−1 gradually increased as the typhoon moved further north, then decreased after +18 h. In both cases, the temporal distribution of precipitation in Korea over time also showed a similar pattern as the average temporal distribution of precipitation ( and ).
Fig. 13. Time evolution of area proportion of detected rainfall intensity above (a) 5 mm h−1 or (b) 1 mm h−1 within the northern 800 km radius of the center of Typhoon MINDULLE and Typhoon NEIL. Solid and dashed vertical lines indicate the extratropical transition time point and weakening time point of each TC, respectively.

Fig. 14. Time evolution of 3-h interval rainfall (bars) from the approaching time point (–12 h) of each typhoon (MINDULLE and NEIL) to 48 h later for (a) Region 1 and (b) Region 2. Lines with an open circle indicate distances between the TC center and Seoul or Gwangju (star-marked point in embedded map). Solid vertical line indicates the time (+6 h) that Typhoon MINDULLE was weakened to EC and dashed vertical line indicates the time (+24 h) that Typhoon NEIL was weakened to TD.

7. Summary and conclusion
In this study, we analysed the spatiotemporal characteristics of precipitation in Korea in connection with weakening of tropical cyclones (TCs) around the Korean Peninsula (32°–36° N, 122°–132° E) over the past 30 years (1979–2015). Weakened TCs were classified into TCs weakened to extratropical cyclones (WEC) and TCs weakened to tropical depressions (WTD). In WEC, precipitation was evenly distributed over all of the Korean Peninsula, with the greatest precipitation recorded on the southern coast. In WTD, the amount of precipitation was the greatest on the southern coast, while low precipitation was recorded in the central and inland areas of Korea. In Region 1 (Central Province, Jeollabuk-do, and inland region of Gyeongsangbuk-do), greater precipitation was observed in WEC than in WTD, while in Region 2 (Jeollanam-do, Gyeongsangnam-do, southeast Gyeongsangbuk-do, and Jeju-do), little difference in precipitation was found between WEC and WTD.
WEC was mainly distributed in June and September, while WTD was distributed only in July and August. Analysis of the surrounding large-scale environments at the TC-approaching time point revealed that in WEC, there were potential vorticity developed in the upper-level troposphere and thermal trough in the lower-level troposphere to the northwest of the TCs. Meanwhile, an upper-level troposphere jet and a strong upper-level troposphere divergence region were observed to the northeast of the TCs. As a result, baroclinic disturbances and nondiabatic processes were developed around the TC, and a wide precipitation area was created, affecting all of Korea. However, in WTD, none of the intense potential vorticity, thermal trough, and upper-level troposphere jets was observed around TCs, and the upper-level troposphere divergence region was relatively small. As a result, no wide precipitation area was created around the TC and precipitation areas were created mainly in the southern regions.
A case study was conducted to compare the ratio of areas with specific precipitation intensities (5 and 1 mm h−1) around the TC using the TRMM satellite rainfall dataset. It was found that in WTD, the precipitation area decreased continuously even before the TC weakened to a TD, while in WEC, a wide precipitation area lasted for a certain period of time even after the TC weakened to an EC.
It was evident that when a TC weakened to an EC and a TD, there were differences in the large-scale environments, but this study mainly focused on comparing the relationship between the background of these large-scale environments and the spatiotemporal distribution of precipitation in Korea. Further studies are necessary to investigate the relationship between intense TCs and precipitation, as well as the relationship between weakened TCs and precipitation, and their characteristics, and various exemplary cases.
Disclosure statement
No potential conflict of interest was reported by the authors.
Additional information
Funding
References
- Browning, K. A., Vaughan, G. and Panagi, P. 1998. Analysis of an extratropical cyclone after its reintensification as a warm core extratropical cyclone. Q. J. Royal Met. Soc. 124, 2329–2356. doi:https://doi.org/10.1002/qj.49712455108
- DiMego, G. J. and Bosart, L. F. 1982a. The transformation of tropical storm Agnes into an extratropical cyclone. Part I: The observed fields and vertical motion computations. Mon. Weather Rev. 110, 385–411. doi:https://doi.org/10.1175/1520-0493(1982)110<0385:TTOTSA>2.0.CO;2
- DiMego, G. J. and Bosart, L. F. 1982b. The transformation of tropical storm Agnes into an extratropical cyclone. Part II: Moisture, vorticity, and kinetic energy budgets. Mon. Weather Rev. 110, 412–433. doi:https://doi.org/10.1175/1520-0493(1982)110<0412:TTOTSA>2.0.CO;2
- Foley, G. R. and Hanstrum, B. N. 1994. The capture of tropical cyclones by cold fronts off of the west coast of Australia. Weather Forecast. 9, 577–592. doi:https://doi.org/10.1175/1520-0434(1994)009<0577:TCOTCB>2.0.CO;2
- Harr, P. A. and Elsberry, R. L. 2000. Extratropical transition of tropical cyclones over the western North Pacific. Part I: Evolution of structural characteristics during the transition process. Mon. Weather Rev. 128, 2613–2633. doi:https://doi.org/10.1175/1520-0493(2000)128<2613:ETOTCO>2.0.CO;2
- Harr, P. A., Elsberry, R. L. and Hogan, T. F. 2000. Extratropical transition of tropical cyclones over the western North Pacific. Part II: The impact of midlatitude circulation characteristics. Mon. Weather Rev. 128, 2634–2653. doi:https://doi.org/10.1175/1520-0493(2000)128<2634:ETOTCO>2.0.CO;2
- Holton, J. R. 1992. An Introduction to Dynamic Meteorology. 2nd ed. Academic Press, San Diego, CA, 511 pp.
- Jang, Y. H. 2002. Diagnosis of heavy rainfall in east coast of Korea by lower-level circulations around typhoon. Atmosphere 12, 456–459.
- Kalnay, E., Kanamitsu, M., Kistler, R., Collins, W., Deaven, D. and co-authors. 1996. The NCEP/NCAR 40-year reanalysis project. Bull. Am. Meteor. Soc. 77, 437–471. doi:https://doi.org/10.1175/1520-0477(1996)077<0437:TNYRP>2.0.CO;2
- Kim, J.-H., Ho, C.-H., Lee, M.-H., Jeong, J.-H. and Chen, D. 2006. Large increase in heavy rainfall associated with tropical cyclone landfalls in Korea after the late 1970s. Geophys. Res. Lett. 33. https://doi.org/10.1029/2006GL027430
- Kim, J. S. and Yoo, J. H. 2002. Study on heavy rainfall in front of typhoon in the eastern region of Kangwon Province. Atmosphere 12, 140–143.
- Kistler, R., Kalnay, E., Collins, W., Saha, S., White, G. and co-authors. 2001. The NCEP/NCAR 50-year reanalysis. Bull. Am. Meteor. Soc. 82, 247–267. doi:https://doi.org/10.1175/1520-0477(2001)082<0247:TNNYRM>2.3.CO;2
- Klein, P. M., Harr, P. A. and Elsberry, R. L. 2000. Extratropical transition of western North Pacific tropical cyclones: An overview and conceptual model of the transformation stage. Weather Forecast. 15, 373–396. doi:https://doi.org/10.1175/1520-0434(2000)015<0373:ETOWNP>2.0.CO;2
- Korea Meteorological Administration. 1996. Typhoon White Book. Korea Meteorological Administration, Soul Korea, 261 pp.
- Kwon, H. J. and Kim, J. Y. 2005. On extratropical transition of tropical cyclone MINDULLE. Atmosphere 15, 17–25.
- Matano, H. and Sekioka, M. 1971. Some aspects of the extratropical transformation of a tropical cyclone. Journal of the Meteorological Society of Japan 49A, 736–743. doi:https://doi.org/10.2151/jmsj1965.49A.0_736
- Newton, C. W. and Trevisan, A. 1984. Clinogenesis and frontogenesis in jet-stream waves. Part I: Analytical relations to wave structure. J. Atmos. Sci. 41, 2717–2734. doi:https://doi.org/10.1175/1520-0469(1984)041<2717:CAFIJS>2.0.CO;2
- Rantanen, M., Räisänen, J., Sinclair, V. A., Lento, J. and Järvinen, H. 2020. The extratropicla transition of hurricane Ophelia (2017) as diagnosed with a genenalized omega equation and vorticity equation. Tellus A 72, 1–26. doi:https://doi.org/10.1080/16000870.2020.1721215
- Ritchie, E. A. 2005. Simulated precipitation structure of the extratropical transition of tropical cyclones. In: 11th Conference on Mesoscale Processes, Albuquerque, NM.
- Ritchie, E. A. and Elsberry, R. L. 2007. Simulations of the extratropical transition of tropical cyclones: Phasing between the upper-level trough and tropical cyclones. Mon. Weather Rev. 135, 862–876. doi:https://doi.org/10.1175/MWR3303.1
- Sekioka, M. 1956a. A hypothesis on complex of tropical and extratropical cyclones for typhoon in the middle latitudes. I. Synoptic structure of Typhoon Marie passing over the Japan Sea. J. Meteorol. Soc. Jpn. 34, 276–287. doi:https://doi.org/10.2151/jmsj1923.34.5_276
- Sekioka, M. 1956b. A hypothesis on complex of tropical and extratropical cyclones for typhoon in the middle latitudes. II. Synoptic structure of Typhoons Ouise, Kezia, and Jane passing over the Japan Sea. J. Meteorol. Soc. Jpn. 34, 336–345. doi:https://doi.org/10.2151/jmsj1923.34.6_336
- Sekioka, M. 1957. A hypothesis on complex of tropical and extratropical cyclones for typhoon in the middle latitudes. III. Examples of typhoon not accompanied by extratropical cyclone in the middle latitudes. J. Meteorol. Soc. Jpn. 35, 170–173. doi:https://doi.org/10.2151/jmsj1923.35.3_170
- Shapiro, M. A. and Kennedy, P. J. 1981. Research aircraft instruments of jet stream geostrophic and ageostrophic winds. J. Atmos. Sci. 38, 2642–2652. doi:https://doi.org/10.1175/1520-0469(1981)038<2642:RAMOJS>2.0.CO;2
- Shi, J. J., Chang, S. and Raman, S. 1997. Interaction between Hurricane Florence(1988) and an upper-tropospheric westerly trough. J. Atmos. Sci. 54, 1231–1247. doi:https://doi.org/10.1175/1520-0469(1997)054<1231:IBHFAA>2.0.CO;2
- Sinclair, M. R. 1993. Synoptic-scale diagnosis of the extratropical transition of a southwest Pacific tropical cyclone. Mon. Weather Rev. 121, 941–960. doi:https://doi.org/10.1175/1520-0493(1993)121<0941:SSDOTE>2.0.CO;2
- Skok, G., Bacmeister, J. and Tribbia, J. 2013. Analysis of tropical cyclone precipitation using an object-based algorithm. J. Clim. 26, 2563–2579. doi:https://doi.org/10.1175/JCLI-D-12-00135.1
- Yang, J. K. and Oh, J. D. 2001. Analysis on convergence zone in front of typhoon causes heavy rainfall. Atmosphere 11, 165–168.
- Yuan, J. and Wang, D. 2014. Potential vorticity diagnosis of tropical cyclone Usagi (2001) genesis induced by mid-level vortex over the South China Sea. Meteorol. Atmos. Phys. 125, 75–87. doi:https://doi.org/10.1007/s00703-014-0316-6
- Ziv, B. and Paldor, N. 1999. The divergence fields associated with time-dependent jet streams. J. Atmos. Sci. 56, 1843–2734. doi:https://doi.org/10.1175/1520-0469(1999)056<1843:TDFAWT>2.0.CO;2