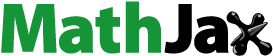
ABSTRACT
During the summer monsoon season, the authors observe a wave train that stretches from the northern Arabian Peninsula and Caspian Sea to the Indo-Gangetic plains along the foothills of the Himalaya and extending further east of the Tibetan Plateau. The trend analysis between 1979 and 2018 with NCEP–NCAR reanalysis data show that the diabatic heating flux (averaged over 1000 to 500 hPa) tends to decrease significantly over the Caspian Sea and its surrounding regions. In addition, the sea level pressure is increasing by ~0.1 hPa yr−1 over the Caspian Sea, forming a high-pressure divergent center over there. The divergent center is collocated with an anticyclonic circulation trend at 850 hPa over the Caspian Sea. This decreasing diabatic heating flux modulates the local atmospheric circulation by increasing the surface pressure around the center of divergence, which further facilitates a wave train to propagate towards South and East Asia. This wave train transports the moisture fluxes at 925 hPa from the Caspian Sea, southeastward towards the South and East Asian monsoon region.
GRAPHICAL ABSTRACT

摘要
本研究发现在夏季风季, 存在从阿拉伯半岛北部和里海至印支恒河平原的一个波列, 该波列进一步沿喜马拉雅山麓伸展到青藏高原东部。利用NCEP–NCAR再分析资料计算的大气非绝热加热通量 (1000 hPa至500 hPa 平均) 在里海及其周围地区表现出从1979至2018年的显著的减少趋势。而海平面气压在里海地区则呈现出大约0.1 hPa yr−1的增加趋势, 并在该区域形成一个高压的辐散中心。这个辐散中心正与里海上空850 hPa的一个反气旋环流趋势相配。减少的大气非绝热通量通过地面气压在辐散中心附近的增加可以调控当地的大气环流, 并进而激发传播向南亚和东亚的波列。该波列可以将925 hPa的水汽从里海向东南输送到南亚季风和东亚季风区。
1. Introduction
The Indian summer monsoon (ISM) circulation is mainly controlled and maintained by two important parameters: the moisture flux convergence and the diabatic heating. During the summer monsoon season, a substantial amount of moisture converges over the monsoon trough through the low-level westerly jet. The precipitation over the South and East Asian monsoon regions intensifies abruptly during the monsoon onset period (Wang and Lin Citation2002). The seasonal changes in moisture transport due to cross-equatorial flow over the West Indian Ocean and a low-level westerly jet from the Arabian Sea to the Bay of Bengal leads to an abrupt increase in the precipitation (Raju, Mohanty, and Bhatla Citation2005; He et al. Citation2007). The strength of the ISM is always influenced by atmospheric moisture transport from oceanic and terrestrial sources. The peak monsoon rainfall occurs during a period when all oceanic and terrestrial moisture sources contribute significantly to the monsoonal rainfall (Pathak et al. Citation2017). The monsoonal rainfall is mainly fed by the strength of the atmospheric transport of oceanic water vapor (significantly from the Indian Ocean); however, the subcontinental land also contributes significantly to the total amount of rainfall (Pathak, Ghosh, and Kumar Citation2014). Therefore, moisture convergence and its advection by the prevailing southwesterly winds are one of the principal sources for the observed rainfall during the monsoon season over the Indian subcontinent.
On the other hand, in the tropics, diabatic heating is the principal source of energy that drives the atmospheric circulation, which in turn influences the atmospheric instabilities. The subseasonal variations of ISM rainfall and associated diabatic heating modulate the zonal and meridional propagation of monsoonal intraseasonal oscillation (Hazra and Krishnamurthy Citation2015). The variations of diabatic heating over the ISM region can lead to low-frequency oscillations in extratropical upper-level westerlies on intraseasonal time scales (Yasunari Citation1986). The latent heat release from organized convection is a key component of diabatic heating over the tropics and monsoon region (Houze Citation1997; Yanai and Tomita Citation1998; Schumacher, Houze, and Kraucunas Citation2004; Houze, Wilton, and Smull Citation2007; Krishnamurti, Chakraborty, and Mishra Citation2010; Choudhury et al. Citation2018). Two-thirds of the global rainfall are received over the tropical region (Tao et al. Citation2006), and the associated latent heat contributes the dominant part of the diabatic heating spatiotemporal distribution. It has also been found that the large-scale tropical circulation is significantly modulated by the vertical distribution of the latent heat (e.g., Lau and Peng Citation1987; Emanuel, Neelin, and Bretherton Citation1994; Schumacher, Houze, and Kraucunas Citation2004). A westward-extending stationary Rossby wave can be generated by the interaction between latent heat release and subtropical westerly mean flow, which in turn leads to summertime descent over the Mediterranean and Sahara regions (Rodwell and Hoskins Citation1996; Liu et al. Citation2001). The excitation of the Rossby wave train in response to diabatic heating over the tropics and monsoon region is associated with the formation of a meridionally extending pattern of large-scale circulation anomalies towards the extratropics and polar latitudes (Sardeshmukh and Hoskins Citation1988; Liu et al. Citation2001; Enomoto, Hoskins, and Matsuda Citation2003; Krishnan et al. Citation2009; Krishnamurti et al. Citation2015).
In this paper, we aim to demonstrate the June–July–August (JJA) trend of near-surface atmospheric moisture transport over the South and East Asian region since 1979. From the aforementioned studies, there is clear occurrence of coupled interplay between the ISM heating and large-scale circulation variations over the Asian monsoon region. This study intends to focus on the large-scale circulation trend during the summer monsoon season and the role of the diabatic heating response to the circulation anomalies. We try to address the impact of midlatitude atmospheric circulation on the variability of near-surface horizontal moisture flux convergence over the South and East Asian region during the ISM season. It has long been known that thermal contrast between land and sea is one of the main driving forces of monsoon (Krishinamurti and Ramanathan Citation1982), which emphasizes the significance of the external forcing. If we take the earth and the atmosphere as a whole into account, both the boundary forcing by the sensible heat flux to the overlying atmosphere and the latent heat release in the air column contribute to the thermally driving atmospheric circulation.
2. Data and methods
This study employs the monthly mean NCEP–NCAR reanalysis data (Kalnay et al. Citation1996) for specific humidity, geopotential height, sea level pressure (SLP), wind fields, and air temperature. All data are at a 2.5° × 2.5° spatial resolution and available from 1979 to 2018.
To identify the spatial distribution of changes, a simple linear regression is computed at each grid point with significance based on the Student’s t-test. During the ISM season, a large amount of cross-equatorial moisture is transported (Findlater Citation1969) from the western Indian Ocean to the Indian landmass (Pathak, Ghosh, and Kumar Citation2014); however, the major evaporative sources for this moisture are not fully understood (Ordóñez et al. Citation2012). In addition, understanding the monsoon circulation can be improved further by studying the atmospheric moisture transport. In order to investigate the moisture transport and its variability, near-surface horizontal moisture flux convergence (925 hPa) is calculated by applying the mass continuity equation and conservation of water vapor in pressure (p) coordinates with the scheme deduced by Banacos and Schultz (Citation2004), which takes the form:
where Vh is horizontal wind component, E is evaporation, ω is the vertical velocity, and P is the precipitation rate. EquationEquation (1)(1)
(1) represents the moisture budget for an air parcel, where the terms represent the local rate of change in specific humidity (q), horizontal moisture flux divergence, vertical moisture flux divergence, evaporation, and precipitation rates (source and sink terms), respectively. The horizontal moisture flux convergence (MFC), which is simply the negative horizontal moisture flux divergence, can be written as
In EquationEquation (2)(2)
(2) , the first two terms are advection terms (where u and v are the zonal and meridional component of wind, respectively), representing the horizontal advection of specific humidity, and the last two terms are convergence terms, which is the product of specific humidity and horizontal mass convergence. The diabatic heating source (Q) is also employed in this study. Q is calculated from the surface to the mid-troposphere (1000 to 500 hPa) with the scheme deduced by Yanai, Esbensen, and Chu (Citation1973), which takes the form
Here, T, Cp, R, and p0 are the air temperature, specific heat of dry air at constant pressure, gas constant for dry air, and standard reference pressure usually 1000 hPa, respectively; denotes the horizontal gradient; and θ is the potential temperature.
3. Results
3.1 Near-surface moisture flux convergence and advection trend
A large amount of atmospheric moisture comes from the Indian Ocean during the summer monsoon season, but its variability from different oceanic and terrestrial sources is also important towards understanding the monsoonal rainfall changes. The western and central Indian Ocean contribute significantly to the atmospheric moisture for the ISM rainfall, with maximum rainfall over the Western Ghats, southern Indian peninsular, and central India (Pathak et al. Citation2017). The continuous supply of moisture from the Indian Ocean remains active during the entire summer monsoon season. In order to sustain the monsoonal circulation, persistent moisture convergence and intense diabatic heating are indispensable features. Therefore, here, we first analyze the trend of near-surface horizontal MFC at 925 hPa. The linear trend of the horizontal moisture flux convergence and advection components with wind (925 hPa) during 1979 to 2018 is depicted in ,b), respectively. Both the convergence and advection term indicate a wave-train-like pattern with alternative positive and negative centers of significant linear trend. The wave train stretches from the northern Arabian Peninsula and the adjacent Caspian Sea to the Indo-Gangetic plains along the foothills of the Himalaya and extending further to the eastern Tibetan Plateau. The pattern exhibits a quasi-zonal wave-like feature, with a prominent anticyclonic feature located over the Caspian Sea. This anticyclonic pattern over the Caspian Sea seems to trigger the wave train, which causes the moisture to advect and converge over the Indian landmass. These results attribute the role of the Caspian Sea as a significant moisture source for the northern part of the Indian subcontinent and East Asia during the JJA monsoon season. Also, it is noted that the equatorial Indian Ocean, especially over the south of the Arabian Sea, remains as another significant moisture convergence region with a cyclonic wind trend. Apart from the wave train, a considerable amount of moisture tends to advect over the north and east of the Arabian Sea. Therefore, near-surface moisture flux analysis over the ISM region reveals the classification of the moisture transport based on their sources. The moisture flux over the land tends to transport from West Asia via the Caspian Sea to the Indian subcontinent in a wave-train form, and the oceanic moisture is primarily originating over the Arabian and equatorial Indian Ocean. However, in order to find the reason behind the anomalous trend of the moisture wave train from West Asia, we investigate the seasonal trends of wind, geopotential height, and SLP.
Figure 1. The near-surface horizontal moisture convergence flux with wind trend (m s−1 yr−1) at 925 hPa: the (a) moisture convergence and (b) moisture advection term (10−6 g kg−1 s−1 yr−1) during the JJA season, 1979–2018. Dots indicate statistical significance at the 0.05 level based on the Student’s t-test. The black lines depict the approximate path of the wave train.

3.2 Variability of wind, SLP, and geopotential height
The JJA mean climatology and interannual linear trend of wind at 925 hPa are shown in ,b), respectively. ) shows that two different kinds of air masses converge over the core monsoon zone (northern part of central India) during the JJA season; one comes from the higher northern latitudes (Eurasian landmass), and the other is the monsoonal cross-equatorial low-level jet from the Indian Ocean. The linear trend exhibits a prominent anticyclonic pattern with wind speed higher than 7 m s−1 over the Caspian Sea ()). The cross-equatorial low-level jet over the Arabian Sea and western Indian Ocean weakens during this period of the study. In ,b), we analyze the linear trend in geopotential height at 850 hPa and SLP, respectively, which also indicate a clear wave-like pattern (alternate positive and negative centers of the trend) propagating eastward from the Caspian Sea and northern Arabian Peninsula to the South and East Asian region. Also, a significant center of the height trend is found over North and Northwest China, which seems like the part of the original wave train diverted due to the high orographic barrier of the Himalaya and Tibetan Plateau. The anticyclone over the Caspian Sea is collocated on the high SLP and positive height anomalies, which in turn probably facilitate the wave train to propagate eastward towards the low-pressure centers over the northern Indian and East Asian landmass. However, the JJA trend in anticyclonic circulation associated with higher surface pressure and positive height anomalies over the Caspian Sea demands considerable attention for further investigation. As the diabatic heating is sustained while there is strong moisture influx coupled with intense rising motion and release of latent heat, thus we also further investigate the wave-train pattern associated with the atmospheric diabatic heating flux.
3.3 Spatial distribution of diabatic heating and its variability
In this section we investigate what role the diabatic heating over the area extending from West to South and East Asia plays in the large-scale atmospheric circulation. The mean state and linear trend of atmospheric diabatic heating flux integrated from 1000 to 500 hPa during 1979 to 2018 are illustrated in ,b), respectively. It can be seen that the region from the northern Arabian Peninsula to the Caspian Sea is the dominant area of the strongest mean seasonal atmospheric diabatic heating ()), which in turn forms the most intense rising motion over the northern Arabian Peninsula. The strongest diabatic heating in these regions might be due to the intense turbulent exchange of sensible heat flux during summer between the surface and upper layers. Around 30°N, an alternating high and low mean diabatic heating source is spread over the northern Arabian Peninsula to East Asia during the JJA season. In the linear trend, the atmospheric diabatic heating flux tends to decrease significantly over the Caspian Sea and its surrounding region during this period ()), and also shows a wave-like pattern from the northern Arabian Peninsula to the Indian subcontinent. Therefore, the anomalous wave train of atmospheric circulation can be perfectly explained in terms of the decreasing trend of the atmospheric diabatic heating flux over the Caspian Sea. The diabatic cooling in the lower and middle troposphere probably inhibits the exchange of moisture between the lower and upper levels. Thus, it is possible that the decreasing trend of atmospheric diabatic heating flux affects the local atmospheric circulation by increasing the surface pressure as lower surface heating forms a relatively higher surface pressure, and thus, as a result, forms a surface divergent circulation from the Caspian Sea region propagating toward the Indian subcontinent during the JJA season. Although Watanabe (Citation2015) earlier demonstrated that the low-level temperature contrast over West Asia plays an important role in the interannual variation of water vapor transportation over the northern and equatorial Indian Ocean and neighboring monsoon area.
4. Summary
In this study, we observe a wave train like pattern with alternate positive and negative significant centers of linear trend in the convergence and advection terms of near-surface horizontal MFC (925 hPa). The wave train stretches from the northern Arabian Peninsula and Caspian Sea to the Indo-Gangetic plains along the foothills of the Himalaya and extends further east of the Tibetan Plateau. The possible mechanism is schematically illustrated in . A decreasing trend in diabatic heating flux during the period 1979–2018 increases the SLP and geopotential height anomaly (850 hPa) trends over the Caspian Sea and its surrounding region. This high-pressure region is collocated with an anticyclonic circulation pattern over the Caspian Sea, which triggers an eastward-propagating wave train from the Caspian Sea to the South and East Asian region. This wave train probably advects the moisture and converges over the North Indian landmass and East Asian regions. This long-range moisture transport may affect the moisture budget over India and the East Asian region during summer monsoon months.
Figure 5. Schematic illustration of the wave-train mechanism. The red oval shows the region of decreasing diabatic heating and divergence. The black oval depicts the area of importance where moisture is converged in association with the wave train.

This work has provided a description of the moisture transport in terms of diabatic heating; however, the variability in other meteorological fields needs to be better understood with further studies. The results attribute an importance of the Caspian Sea as a significant moisture source for the South and East Asian region during the ISM season. The analysis also reveals the classification of the JJA moisture transport over the ISM region based on its sources: the terrestrial moisture tends to be transported from West Asia via the Caspian Sea to the Indian subcontinent in a wave train form, and the oceanic moisture primarily originates over the Arabian and equatorial Indian Ocean. This study may provide a better understanding of the variability of moisture transport over South and East Asia and the mechanism involved in the changes of various atmospheric circulations during the summer monsoon season.
Supplemental Material
Download PDF (585.7 KB)Disclosure statement
The authors declare no conflicts of interest.
SUPPLEMENTAL DATA
Supplemental data for this article can be accessed here.
Correction Statement
This article has been republished with minor changes. These changes do not impact the academic content of the article.
Additional information
Funding
References
- Banacos, P. C., and D. M. Schultz. 2004. “The Use of Moisture Flux Convergence in Forecasting Convective Initiation: Historical and Operational Perspectives.” Weather and Forecasting 20: 351–366. doi:10.1175/WAF858.1.
- Choudhury, A. D., R. Krishnan, M. V. S. Ramarao, R. Vellore, M. Singh, and B. Mapes. 2018. “A Phenomenological Paradigm for Midtropospheric Cyclogenesis in the Indian Summer Monsoon.” Journal of Atmospheric Sciences 75: 2931–2954.
- Emanuel, K. A., J. D. Neelin, and C. S. Bretherton. 1994. “On Large-scale Circulations in Convecting Atmospheres.” Quarterly Journal of Royal Meteorological Society 120: 1111–1143. doi:10.1002/qj.49712051902.
- Enomoto, T., B. J. Hoskins, and Y. Matsuda. 2003. “The Formation Mechanism of the Bonin High in August.” Quarterly Journal of Royal Meteorological Society 129: 157–178.
- Findlater, J. 1969. “A Major Low-level Air Current near the Indian Ocean during the Norther Summer.” Quarterly Journal of Royal Meteorological Society 95: 362–380.
- Hazra, A., and V. Krishnamurthy. 2015. “Space-time Structure of Diabatic Heating in Monsoon Intraseasonal Oscillation.” Journal of Climate 28: 2234–2255.
- He, J., C. Sun, Y. Liu, J. Matsumoto, and W. Li. 2007. “Seasonal Transition Features of Large-scale Moisture Transport in the Asian-Australian Monsoon Region.” Advances in Atmospheric Sciences 24: 1–14. doi:10.1007/s00376-007-000.
- Houze, R. A. 1997. “Stratiform Precipitation in Regions of Convection: A Meteorological Paradox?” Bulletin of American Meteorological Society 78: 2179–2196.
- Houze, R. A., D. C. Wilton, and B. F. Smull. 2007. “Monsoon Convection in the Himalayan Region as Seen by the TRMM Precipitation Radar.” Quarterly Journal of Royal Meteorological Society 133: 1389–1411.
- Kalnay, E., M. Kanamitsu, R. Kistler, W. Collins, D. Deaven, L. Gandin, M. Iredell et al. 1996. “The NCEP/NCAR 40-year Reanalysis Project.” Bulletin of American Meteorological Society 77: 437–472.
- Krishinamurti, T. N., and Y. Ramanathan. 1982. “Sensitivity of the Monsoon Onset to Differential Heating.” Journal of Atmospheric Sciences 39: 1290–1306.
- Krishnamurti, T. N., A. Chakraborty, and A. K. Mishra. 2010. “Improving Multimodel Forecasts of the Vertical Distribution of Heating Using the TRMM Profiles.” Journal of Climate 23: 1079–1094.
- Krishnamurti, T. N., R. Krishnamurti, S. Das, V. Kumar, A. Jayakumar, and A. Simon. 2015. “A Pathway Connecting Monsoonal Heating to Rapid Arctic Ice Melt.” Journal of Atmospheric Sciences 72: 5–34.
- Krishnan, R., V. Kumar, M. Sugi, and J. Yoshimura. 2009. “Internal Feedbacks from Monsoon–mid-latitude Interactions during Droughts in the Indian Summer Monsoon.” Journal of Atmospheric Sciences 66: 553–578.
- Lau, K.-M., and L. Peng. 1987. “Origin of Low-frequency (Intraseasonal) Oscillations in the Tropical Atmosphere. Part I: Basic Theory.” Journal of Atmospheric Sciences 44: 950–972. doi:10.1175/15200469(1987)044,0950:OOLFOI.2.0.CO;2.
- Liu, Y. M., G. X. Wu, H. Liu, and P. Liu. 2001. “Condensation Heating of the Asian Summer Monsoon and the Subtropical Anticyclone in Eastern Hemisphere.” Climate Dynamics 17: 327–338.
- Ordóñez, P., P. Ribera, D. Gallego, and C. Pena-Ortiz. 2012. “Major Moisture Sources for Western and Southern India and Their Role on Synoptic-scale Rainfall Events.” Hydrological Processes 26: 3886–3895. doi:10.1002/hyp.8455.
- Pathak, A., S. Ghosh, and P. Kumar. 2014. “Precipitation Recycling in the Indian Subcontinent during Summer Monsoon.” Journal of Hydrometeorology 15: 2050–2066.
- Pathak, A., S. Ghosh, J. A. Martinez, F. Dominguez, and P. Kumar. 2017. “Role of Oceanic and Land Moisture Sources and Transport in the Seasonal and Interannual Variability of Summer Monsoon in India.” Journal of Climate 30(5): 1839–1859. doi:10.1175/JCLI-D-16-0156.s1.
- Raju, P. V. S., U. C. Mohanty, and R. Bhatla. 2005. “Onset Characteristics of the Southwest Monsoon over India.” International Journal of Climatology 25: 167–182. doi:10.1002/joc.112.
- Rodwell, M. J., and B. J. Hoskins. 1996. “Monsoons and the Dynamics of Deserts.” Quarterly Journal of Royal Meteorological Society 122: 1385–1404.
- Sardeshmukh, P. D., and B. J. Hoskins. 1988. “The Generation of Global Rotational Flow by Steady Idealized Tropical Convergence.” Journal of Atmospheric Sciences 45: 1228–1251.
- Schumacher, C., R. A. Houze, and I. Kraucunas. 2004. “The Tropical Dynamical Response to Latent Heating Estimates Derived from the TRMM Precipitation Radar.” Journal of Atmospheric Sciences 61: 1341–1358. doi:10.1175/15200469(2004)061,1341:TTDRTL.2.0.CO;2.
- Tao, W., E. A. Smith, R. F. Adler, Z. S. Haddad, A. Y. Hou, T. Iguchi, R. Kakar, et al. 2006. “Retrieval of Latent Heating from TRMM Measurements.” Bulletin of American Meteorological Society 87: 1555–1572. doi:10.1175/BAMS-87-11-1555.
- Wang, B., and H. Lin. 2002. “Rainy Season of the Asian–Pacific Summer Monsoon.” Journal of Climate 15: 386–398.
- Watanabe, T. 2015. “Role of Regional Thermal Contrast over West Asia Interannual Variation in Atmospheric Moisture Transportation over the Indian Ocean and Neighboring Areas at Summer Monsoon Onset.” Journal of Geophysical Research Atmosphere 120: 11826–11845. doi:10.1002/2015JD023617.
- Yanai, M., S. Esbensen, and J.-H. Chu. 1973. “Determination of Bulk Properties of Tropical Cloud Clusters from Large-scale Heat and Moisture Budgets.” Journal of Atmospheric Sciences 30: 611–627.
- Yanai, M., and T. Tomita. 1998. “Seasonal and Interannual Variability of Atmospheric Heat Sources and Moisture Sinks as Determined from NCEP-NCAR Reanalysis.” Journal of Climate 11: 463–482.
- Yasunari, T. 1986. “Low-frequency Interactions between the Summer Monsoon and the Northern Hemisphere Westerlies.” Journal of Meteorological Society of Japan 64: 693–708.