ABSTRACT
The rise in energy consumption and the increase in the use of bio-based materials in the building sector, has led to the need to investigate the possibilities to use wood as a porous support material for phase change materials (PCMs), and thereby creating a thermal regulative wood-based product. This study investigated the influence of Kraft lignin-glyoxal prepolymer on the thermal-energy storage properties of wood modified with paraffin-type of PCM. The implementation of the modified wood involves preparing PCM emulsions, synthesising lignin-glyoxal prepolymer, and modifying wood with the PCM-Kraft lignin-glyoxal emulsion through vacuum-pressure impregnation. The infrared imaging suggested the ability of PCM-modified wood to delay the temperature changes, even with the introduction of Kraft lignin-glyoxal prepolymer. In conclusion, it is feasible to introduce thermal-energy storage property into wood with the addition of Kraft lignin-glyoxal prepolymer. Further studies will focus on the long-term thermal storage performance properties when this PCM system is subjected to repeated heating/cooling cycles.
Introduction
One potential method to reduce energy usage in buildings involves integrating large latent heat capacity materials, such as organic phase-change materials (PCMs) to absorb/release excess heating or cooling energy (Hartig et al. Citation2023). Organic PCMs undergo melting and solidification around their melting points, allowing them to stabilise temperatures in the surroundings by absorbing or releasing environmental heat energy.
PCMs selection often depends on aligning the melting temperature with the operating environment. Integrating PCMs into buildings can occur by incorporating them as independent components or by integrating them into existing construction materials, e.g. wood. However, PCM leakage out of the modified wood during its liquid phase poses challenges and may hinder wider applications. One approach to alleviate leakage involves microencapsulating PCMs within a polymeric matrix, confining their phase change within polymeric shells. Nevertheless, the immiscible character of oily organic-based PCMs with water-borne prepolymer commonly used for the wood modification complicates implementation. Surfactants plays a crucial role in preventing phase separation by stabilising interfaces through reducing interfacial tension. Surfactants with appropriate hydrophilic-lipophilic balance (HLB) values can give stable emulsions with small droplet size by use of a sufficient concentration of dissolved surfactant, known as the critical micellar concentration (CMC), leading to the spontaneous formation of nanometre-scale structured micelles (Guerrero-Hernández et al. Citation2022). Although this method shows promising potential for enhancing mixing compatibility PCM with prepolymers, its implementation has been studied very little for wood modification.
The objective of this study was to evaluate the feasibility of blending oily PCMs with water-borne Kraft lignin-glyoxal prepolymer to create a stable mixture. Subsequently, the study examines how the thermal-energy storage properties of PCMs modified wood, with or without Kraft lignin-glyoxal prepolymer, are affected.
Experimental
Materials
Scots pine (Pinus sylvestris L.) sapwood specimens with the dimensions of 30 × 6 × 20 mm (T × R × L), a density of approx. 500 kg/m3, and a moisture content of approx. 7% was used for the experiment. The following chemicals were used: an organic PCM (RT 18HC, Rubitherm GmbH, Germany); Tween 80, Span 80, and analytical-grade NaOH pellets (Merck KGaA, Germany); Kraft lignin (Borregaard A/S, Norway).
The thermophysical properties of RT 18HC are as follows: melting temperature 18°C, heat storage capacity of 260 kJ/kg, density in solid and liquid states of 880 kg/m3 and 770 kg/m3, respectively, and volume expansion of 12.5% during phase change.
Wood modification
The PCM-lignin-glyoxal modified wood was implemented in four stages as follows, and the illustration is presented in .
Preparation of the PCM emulsion: An emulsion containing 5.0 wt.% of surfactants, 20.0 wt.% PCM, and 75.0 wt.% of deionised (DI) water was prepared. The HLB value of the surfactants, crucial for achieving the minimum droplet size, was controlled by adjusting the mass ratio of Tween 80 (HLB value: 15.0) and Span 80 (HLB value: 4.3) (Liu et al. Citation2006). Initially, the surfactant was dissolved in water, followed by the dropwise addition of PCM to the solution under an agitation speed of 1000 rpm using a magnetic stirrer resulting in a homogenous white emulsion.
Synthesis of Kraft lignin-glyoxal prepolymer: Firstly, Kraft lignin was dissolved in DI water, aided by a few drops of 10M NaOH for better dissolution. Then, the crosslinker glyoxal was added to the solution and the pH value was adjusted to 12.5 using 10M NaOH, resulting in a final concentration of 10.0 wt.% Kraft lignin and 10.0 wt.% glyoxal. The mixture was heated at 40°C for 2 h under stirring with a cooling condenser.
Mixing of Kraft lignin-glyoxal prepolymer with PCM emulsion: The obtained Kraft lignin-glyoxal prepolymer solution was added dropwise to the PCM emulsion while stirring. The mass ratio of the two components was 1:1.
Wood modification: Wood specimens underwent three different modifications using products from stages 1 to 3, following the full-cell vacuum pressure impregnation procedure. This involved subjecting the specimen to 30 min of reduced pressure at 20 mbar, followed by 1 h at high pressure of 15 bar. The impregnated specimens were then oven-dried at 80°C overnight. The specimens were named accordingly: (i) specimen modified with the emulsion from step 1 was denoted as HC18-wood, (ii) specimen modified by the solution from stage 2 was denoted as LG-wood, and (iii) specimen modified by the emulsion from stage 3 was denoted as HC18-LG-wood.
Characterisation
Dynamic light scattering (DLS) was used to characterise the droplet size distribution and Z-potential of both the PCM and PCM-lignin-glyoxal emulsions, using a Zetasizer Nano-ZS (Malvern Instruments Ltd., Malvern, UK) equipped with a He−Ne laser of λ = 632.8 nm. Before the measurement, the suspensions were diluted 600 times in DI water. The measurement was performed at 25°C. The Z-average mean diameter was obtained from the average of the three replicate measurements, and the data were analysed using the cumulant method. Z-potential was determined by three replicates, with 12 runs of analysis in each repetition. Infrared image was recorded using the thermal camera FLIR C5 (FLIR Systems, USA) at a distance of the specimen of approximately 40 cm. The specimens were initially cooled down to a temperature of 5°C and were then allowed to warm to ambient room temperature (20°C) to observe their temperature changes. Liquid uptake was calculated based on the mass before and after impregnation. Weight percentage gain (WPG) was calculated based on the dried mass of the specimen before impregnation and mass of the specimen after oven drying at 80°C. Expected WPG was calculated by multiplying the liquid uptake (Δ%) with the solid content of the emulsion/solution.
Results and discussion
The correlation of the Z-average radius (Zavg.r) of the PCM micelles with its HLB value is presented in . A narrow HLB region between 11.25 and 12.0 exhibited the smallest Zavg.r of 148.6 nm at a HLB value of 11.25. The result indicated that PCM nano-micelle was formed in the oil-in-water emulsion.
Figure 2. DLS analysis of the HC18 micelles size with different hydrophilic-lipophilic balance (HLB) values.
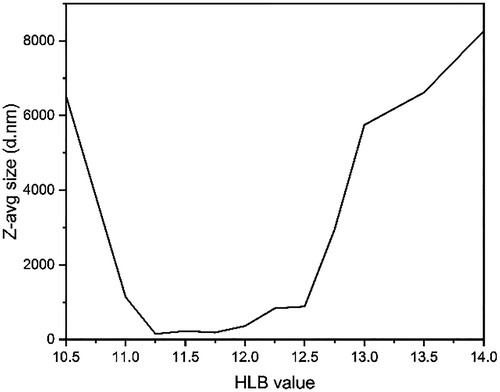
The emulsion with HLB value of 11.25 was selected and further mixed with a lignin-glyoxal prepolymer, resulting in a similar Zavg.r of 138.8 nm. Additionally, the Z-potential of HLB 11.25 of PCM micelles increased from −32.7 mV in the PCM emulsion to −50.0 mV with the introduction of lignin-glyoxal prepolymer. The higher absolute value of Z-potential indicated better stability of the emulsion, attributed to the steric hinderance of the polymeric network of the lignin-glyoxal, enhancing the stability of the PCM micelles (Kontogeorgis and Kiil Citation2016).
The WPG is an indication of the levels of chemicals loaded into the specimen, as presented in . The WPG of HC18-wood and HC18-LG-wood was lower than the expected WPG, likely due to the evaporation of HC18 during drying of the specimens. LG-wood had a WPG close to the expected value, attributed to the low volatility of the lignin-glyoxal prepolymer. Although dehydration during Kraft lignin-glyoxal condensation polymerisation can result in the evaporation of water molecules (Van Nieuwenhove et al. Citation2020), the slightly higher WPG than the expected WPG might be attributed to the addition of NaOH used for the pH adjustment.
Table 1. Liquid uptake, weight percentage gain (WPG), and expected WPG of the specimens.
The effect of PCMs delaying the temperature increase of cooled wood specimens when exposed at room temperature was qualitatively visualised by infrared images, as shown in . Unmodified wood and LG-wood required 4 minutes to reach ambient room temperature, while HC18-wood and HC18-LG-wood slowed down the time to reach this equilibrium. This was attributed to the large latent heat of PCMs, which melted and slowed down temperature change.
Conclusion
This study demonstrated the potential of using wood as a thermal-energy storage material by combining Kraft lignin-glyoxal prepolymer and PCM. The DLS result revealed the compatibility of blending oily PCMs and water-borne Kraft lignin-glyoxal prepolymer, with an improved PCM nano-micellar stability. Infrared imaging showed the delaying of the temperature change due to large latent heat of PCM in the wood, even with the addition of Kraft lignin-glyoxal prepolymer. Further study is needed for a comprehensive understanding of material properties, including thermal behaviour, penetration depth, the effectiveness of alleviating leakage from the wood matrix, melting and solidification enthalpy, and the retention of long-term thermal storage performance over repeated heating/cooling cycles.
Acknowledgements
Support through the project “Luleå University of Technology's initiative ‘Natural Resources for Sustainability transitions’ (SUN), advanced research supporting the forestry and wood-processing sector’s adaptation to global change and the 4th industrial revolution”, CT WOOD – a centre of excellence at Luleå University of Technology supported by the Swedish wood industry, and from the Swedish Research Council for Environment, Agricultural Sciences and Spatial Planning (FORMAS), project “Biobased fire protection of wood panel for exterior conditions by using phosphorylated lignin from wheat straw 2021-00818”, are gratefully acknowledged.
Disclosure statement
No potential conflict of interest was reported by the author(s).
Additional information
Funding
References
- Guerrero-Hernández, L., et al., 2022. Gemini and Bicephalous surfactants: a review on their synthesis, micelle formation, and uses. International Journal of Molecular Sciences, 23(3), 1798.
- Hartig, J.U., et al., 2023. Impregnation of wood with a paraffinic phase change material for increasing heat capacity. Wood Material Science & Engineering, 18 (1), 19–28.
- Kontogeorgis, G.M., and Kiil, S., 2016. Colloid stability – Part I: the major players (van der Waals and Electrical Forces. In: Introduction to applied colloid and surface chemistry. 1st ed. Chichester: John Wiley & Sons, Ltd., pp. 211–242.
- Liu, W., et al., 2006. Formation and stability of paraffin oil-in-water nano-emulsions prepared by the emulsion inversion point method. Journal of Colloid and Interface Science, 303 (2), 557–563.
- Van Nieuwenhove, I., et al., 2020. Biobased resins using lignin and glyoxal. ACS Sustainable Chemistry & Engineering, 8, 18789–18809.