ABSTRACT
This study tested whether an alloimmune response can occur in the marrow donor when infused or injected with leukocytes from their mixed chimeric transplant recipient. Two mixed chimeras were produced after conditioning with three Gray total body irradiation, donor marrow infusion, and post-grafting immunosuppression. The marrow donors were then repeatedly infused and injected with leukocytes from their respective chimeric recipient. A donor lymphocyte infusion (DLI) into their mixed chimeras had no effect, even after the experiments were repeated. The presence of blood dendritic cells (DCs) of recipient origin was confirmed in chimeric recipients, as well as the presence of microchimerism in the marrow donors. Donor sensitization did occur following placement of a recipient skin graft that was confirmed following DLI into recipients that changed the mixed chimeras into full donor chimeras. These observations suggest that mixed chimerism renders recipient peripheral blood DCs incapable of inducing a donor T cell response.
Introduction
Alloimmunity refers to various cellular and humoral immune responses against genotypic differences between individuals, whereas immunological tolerance refers to the ability to prevent these immune responses.Citation1 For major histocompatibility matched (MHC) allogeneic haematopoietic cell transplantation (HCT), the majority of alloimmunity refers to adaptive T cell-mediated responses against minor histocompatibility antigens (miHAs). MiHAs are MHC presented peptides derived from endogenous polymorphic gene differences between the transplant donor and host.Citation2 T cell responses against miHAs are implicated in the non-relapse mortality caused from host versus graft rejectionCitation3 and graft-vs.-host disease (GVHD),Citation4 as well as in the curative graft-vs.-tumor (GVT) responses for patients with hematologic malignancies.Citation5 Therefore, improving outcomes following allogeneic HCT is directly dependent on refining the ability to control alloimmunity and immunological tolerance.
Boosting miHA-mediated immune responses depends on understanding the initiating events. The 1960 Nobel Prize in Physiology or Medicine highlighted the work of Owen,Citation6 Burnet,Citation7 MedawarCitation8 and colleagues who formulated a “nonself” model to explain alloimmunity. Building on that foundation, a more nuanced “danger” model emerged supported by the discovery of pattern recognition receptors,Citation9,10 that provide evidence of alarm signals that link the innate activity of dendritic cells (DCs)Citation11 with the adaptive responses of T cells.Citation12 According to the danger model, inducing a T cell response to miHAs depends on the presence of alarm signals, and an immune response may not occur even when exposed to miHAs if the tissue, or more aptly the DCs in the tissue, lack these alarm signals. It is now apparent that alloimmunity or tolerance depends upon a series of receptor-ligand interactions between various T cell and DC subsets under the influence of the local cytokine milieu.Citation13
MiHA-mediated immune responses can occur when the transplant recipient's DCs present antigens through direct antigen presentation to donor T cells or when DCs present recipient miHAs through indirect antigen presentation to donor T cells. With this biological understanding, the canine model of allogeneic HCT, specifically mixed chimerism, was used as a platform to further characterize donor T cell responses to miHAs. Stable mixed haematopoietic chimerism can be achieved following low dose total body irradiation (TBI), dog leukocyte antigen (DLA)-identical marrow infusion, and a short course of post-grafting immunosuppression with mycophenolate mofetil and cyclosporine.Citation14 T regulatory cells induce an active cellular immunological tolerance allowing donor and host T cells to co-exist,Citation15,16 despite numerous miHA differences. “Unsensitized” donor lymphocyte infusions (DLI) do not overcome the T regulatory-mediated tolerance of mixed chimerism, producing no significant change in mixed chimerism.Citation17,18 However, if the marrow donor is first “sensitized” to miHAs of the recipient via recipient skin grafts,Citation17 recipient organ grafts,Citation19 or infusion of leukocytes from DLA-identical littermates,Citation20 then a “sensitized” DLI breaks tolerance changing the mixed chimera to 100% donor chimerism. Thus, extrapolating from these earlier studies, it was hypothesized that intravenous and subcutaneous infusions of viable recipient cells from mixed chimeras into their respective marrow donors would sensitize the donors to the miHAs of the recipients. In this setting, a DLI converting the mixed chimera to full donor chimera would provide an in vivo readout and confirm the hypothesis. However, the marrow donors did not become sensitized to recipient miHAs despite multiple exposures to viable recipient leukocytes present within the blood of the mixed chimeras. The studies were repeated and the ability of the marrow donor to respond to recipient miHAs was verified by skin grafting.
Results
First attempt at alloimmunization of the donor
To test the hypothesis whether remaining recipient leukocytes in a stable mixed chimera could successfully alloimmunize the marrow donor, two mixed chimeric recipients, H550 and H552, were produced with three Gray TBI, marrow donor infusion, and post grafting immunosuppression with cyclosporine and mycophenolate mofetil. At the time of the first DLI, both recipients had been off all immunosuppression for 12 months. The percentage of donor-recipient mixed chimerism for the eight months preceding the first DLI is shown as a baseline for H550 and H552, with the eight-month time point occurring two weeks prior to the first DLI (black lines, ). For H550, granulocytes ranged from 90 to 97% donor and PBMC ranged from 62 to 86% donor, while H552 granulocytes ranged from 26 to 68% donor and PBMC ranged from 45 to 64% donor for the eight months preceding the first DLI (black lines, ).
Figure 1. Summary of the chimerism results before and after three DLIs into two stable mixed chimeric recipients. The y-axis shows the percentage of donor chimerism in the granulocyte fraction for H550 (A) and H552 (B), and PBMC fraction for H550 (C) and H552 (D). The x-axis shows times in months. Baseline reported chimerism values for each recipient for the 8 months preceding the first DLI with the last time point taken two weeks prior to the first DLI. The rest of the chimerism values are reported following each DLI with zero representing the time of the DLI.
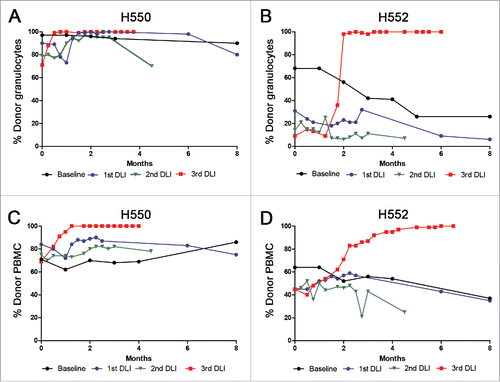
For the first attempt, marrow donors were infused intravenously and injected subcutaneously with leukocytes harvested from 60 mL of blood from their respective mixed chimeric recipient on day −30, day −20, and day −10, followed by a DLI into the recipient on day 0. The donor for H550 received a total dose of 1.64 × 108 viable mixed chimeric leukocytes, while the donor for H552 received a total dose of 2.51 × 108 viable mixed chimeric leukocytes in the first attempt (). Taking into account a mixed chimera with 80 to 90% donor chimerism resulting in only 10 to 20% of the cells of recipient origin, the number of recipient cells injected into the donor reached at least 1–2 × 107 cells per attempt.
Table 1. Summary of the donor sensitization attempts followed by DLI into the mixed chimeric recipient.
To minimize the effects of route of administration on the interpretation of the results, half the cell dose was infused intravenously and the other half was injected subcutaneously.Citation21,22 A DLI was then performed into H550 and H552 and the level of donor chimerism was assessed over time (). There was a transient increase in the percent donor granulocyte levels for H550 ranging from 73 to 100% donor (blue line, ). However, there was no change in the percent donor PBMC for H550, and by eight months after the first DLI, the percent donor chimerism for the granulocyte and PBMC fractions was essentially unchanged from pre-DLI levels in both chimeras.
Second attempt at alloimmunization of the donor
The second attempt at marrow donor sensitization was performed to verify the results obtained following the initial attempt. The antigen source and the timing of three separate infusions of mixed chimeric recipient leukocytes were kept the same as the initial attempt. To further eliminate route of administration effects on the interpretation of these results the cells were again split, half were infused IV, and the other half injected via an intradermal route instead of a subcutaneous route in order to deliver recipient antigens directly into dermal DCs of the donor. Using trypan blue exclusion, the donor for H550 received a total dose of 1.15 × 108 viable mixed chimeric leukocytes, while the donor for H552 received a total dose of 1.97 × 108 viable mixed chimeric leukocytes ().
To further confirm the viability of the cell injections, cell dosages were also counted using acridine orange/ethidium bromide (AO/EB) double staining.Citation23 The viable cell counts using AO/EB were on average 80%, range 57–106%, of the value obtained using trypan blue exclusion (data not shown). The difference in viability was consistent with the underestimation of the cell death assessment of trypan blue staining by light microscopy as compared with fluorescent exclusion dyes detected by fluorescence microscopy. A DLI was then performed into H550 and H552 and the level of donor chimerism was assessed over time (). Again there was a transient increase in the percent donor granulocyte levels for H550 ranging from 80 to 97% donor (green line, ). However, there was no change in the percent donor PBMC for H550, and by eight months after the second DLI, the percent donor chimerism for the granulocyte and PBMC fractions was essentially unchanged from pre-DLI levels in both chimeras.
Donor microchimerism studies and third attempt at alloimmunization
The two marrow donors were evaluated for the presence of recipient cells. Microchimerism, or the presence of small amounts of cells from another individual, is a well-recognized phenomenon of pregnancy, as well as increasingly appreciated following blood transfusions.Citation24 A PCR signal of recipient DNA was detected from the peripheral blood of both marrow donors one month after the last injection of mixed chimeric cells. This signal was not present for marrow donor H555 (), and was only half the signal strength for marrow donor H554 () prior to the infusion of mixed chimeric cells. A standard curve was created with mixtures of marrow donor and recipient DNA collected prior to transplant. The standard curve was accurate up to a mixture of 97% donor DNA for H555 () and 99.7% donor DNA for H554 ().
Figure 2. Variable number tandem repeat (VNTR) assay. Genomic DNA of H555 and H552 (A) H554 and H550 (C) were isolated and amplified by PCR for VNTR. The data are represented by peaks with the shaded areas representing area under the curve (AUC). The top panel shows the marrow donor VNTR prior to any cell injections from the mixed chimera. The middle panel shows marrow donor VNTR one month after the last set of cell injections from the mixed chimera. The bottom panel shows the VNTR of the transplanted recipient prior to haematopoietic cell transplant as a comparison. Titrated mixtures of pre-transplant donor and recipient DNA shown for H555 and H552 (B) and H554 and H550 (D) were shown in a logarithmic scale to determine the limits of detection.
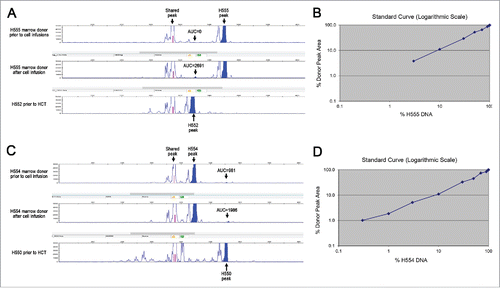
The third attempt at marrow donor sensitization was performed to confirm that both marrow donors remained capable of responding to miHAs. Past studies have already shown that miHA sensitization of the marrow donor occurs following mixed chimeric recipient skin graft placement.Citation17 Each donor underwent placement of a two by two centimeter full thickness skin graft from their respective mixed chimeric recipients. The skin grafts were viewed every other day to confirm adequate surgical technique and assess for signs of rejection. The recipient skin graft on the marrow donor for H550 became erythematous on day +16 after placement; the skin graft on the marrow donor for H552 became erythematous on day +13 after placement. Both skin grafts then blistered and were obliterated over the next seven days. Six weeks after the full thickness skin graft, the donors were further sensitized with a “modified” skin graft placement consisting of a subcutaneous placement of a minced square centimeter of recipient skin. DLIs were performed 10 days after the modified skin graft. H550 rapidly converted to 100% donor granulocytes and PBMC within four weeks (red lines, ). H552 converted more slowly, but ultimately achieving 100% donor granulocytes and PBMC (red lines, ). Neither recipient demonstrated any signs of GVHD at necropsy.
Evaluating dendritic cell and T regulatory cell populations in mixed chimeras
The active cellular tolerance that prevents marrow donor sensitization by mixed chimeric leukocytes likely depends on regulatory T cells and tolerogenic DCs, and these cell populations were further evaluated. Consistent with previous reports,Citation25 the level of T regulatory cells in the peripheral blood of H550 and H552 prior to donor conversions were within the range of normal controls, as assessed by CD4+, CD25+, and FoxP3+ expression by flow cytometry. Percent CD4+, CD25+, and FoxP3+ cells from within the lymphocyte gate ranged between 0.26 to 0.8 for H550 and H552, which was comparable to five normal controls that ranged from 0.06 to 0.83.
Next, studies were performed to eliminate the possibility that the results from the first two sensitization attempts were due to the lack of recipient-derived blood DCs in the mixed chimera capable of sensitizing the marrow donor. Functional, peripheral blood DC population in canines were previously defined as cells that express MHC class II and canine CD11c that were not granulocytes or monocytes ().Citation26 At the time of this analysis, no cells were available from H550 and H552 prior to conversion to full donor; instead, blood DCs were flow sorted from frozen PBMC samples from three mixed chimeras H382, H519, and H597, previously banked from past studies. The purity of the sorted cells ranged from 91 to 92%, representing approximately 100-fold enrichment considering blood DCs consist of less than 1% of the PBMC population.Citation26 Donor chimerism percentages of the sorted DC as compared with the PBMCs populations were 76% vs. 62% for H382, 31% vs. 47% for H519, and 63% vs. 65% for H597, confirming that the sorted blood DCs contained both recipient and donor cells in nearly the same ratio as the PBMC fraction.
Figure 3. Fluorescence-activated cell sorting of peripheral blood dendritic cells. The first gate utilized forward scatter and side scatter properties to eliminate cell debris (left plot). The second gate was then used to exclude CD14+, DM5- monocytes and the DM5+, CD14- granulocytes from the sorted population (middle plot). MHC class II+ cells determined using an antibody against DLA-DR, and canine CD11c+ cells were then sorted for use in chimerism analysis or in MLRs (right plot).
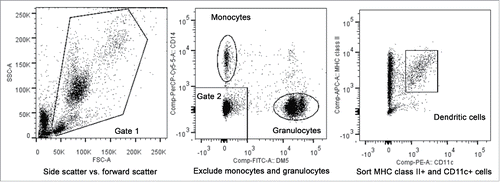
Functional analysis of the sorted DCs from these mixed chimeras was then pursued to eliminate the possibility that the lack of sensitization was due to non-functional recipient-derived DCs. However, an in vitro test to determine the responsiveness of recipient-derived DCs to miHAs was not possible, given that miHAs await molecular characterization in the dog model. Instead, the functional characterization of the sorted DCs was limited to testing their ability to respond to major MHC mismatches in a mixed leukocyte reaction (MLR). The sorted DCs from all three mixed chimeras were capable of stimulating third party responders, demonstrating that these DCs were functional (). However, there was no way to further narrow the contribution of this response to either the donor- or recipient-derived DCs.
Figure 4. Functional characterization of sorted cell populations assayed in MLR. The monocyte, granulocyte, and peripheral blood DCs populations were sorted as shown for and used as stimulator cells. Constant numbers of third party responding PBMC (1×105 cells per well) were mixed with varying numbers of irradiated stimulator cells (5–20×103). The responders and stimulators were MHC-mismatched. The stimulation index was calculated as the average counts per minute of the responder cells with stimulator cells added divided by the average counts per minute of responder cells only plus or minus the standard deviation (SD). The solid line and filled in data points were for the normal control, while the three samples from mixed chimeric recipients H382, H597, and H519 were shown with dotted lines and open data points.
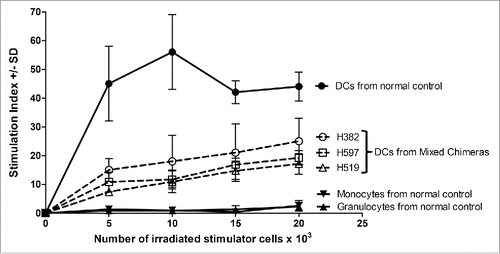
Discussion
Conclusions
This report represents the first study in the dog HCT model to ask the question whether an alloimmune response can develop when a marrow donor is infused or injected with leukocytes from their respective mixed chimeric transplant recipients. The small numbers of transplant pairs used in these studies prevent definitive conclusions. However, the sensitization experiments were repeated and miHA sensitization of the marrow donor did not occur despite repeated exposures to recipient blood cells from their respective mixed chimera. These experiments disprove the initial hypothesis that miHA sensitization of the marrow donor was going to occur following exposure to recipient leukocytes from a mixed chimera. The results of these experiments suggest that direct miHA presentation from the mixed chimeric recipient's DCs and indirect miHA presentation from the marrow donor DCs did not occur in spite of the presence of the genetically disparate cells.
The lack of miHA sensitization following the infusion and injection of mixed chimeric leukocytes into the marrow donor was not due to insufficient numbers of viable recipient cells. It is known that leukocyte infusions between MHC-matched sibling transplant pairs in outbred populations leads to rapid miHA sensitization.Citation27 For example, a single 100 mL, non-irradiated marrow donor blood transfusion into an MHC-matched sibling recipient 24 hours prior to transplant resulted in six of seven graft rejections despite ablative 9.2 Gy TBI conditioning and DLA-identical marrow infusion.Citation28 MiHA sensitization occurs due to a small number of blood DCs present within the infused leukocytes that sensitize the transplant recipient's T cells.Citation29 It takes only a small number of infused leukocytes, and hence an exceedingly small number of blood DCs, between DLA-identical siblings to induce miHA sensitizationCitation27-39 From past experiments, it was shown that infusing the transplant recipient with as few as 5 × 104 donor leukocytesCitation33,40,41 or PBMCCitation29 on day −24, −17, and day −10 prior to transplant for a total dose of 1.5 × 105 cells resulted in 40% graft rejection despite myeloablative 9.2 Gy TBI conditioning. Compared to these past studies and taking into account that a percentage of the mixed chimeric cells were donor, each marrow donor in this study received at least 1–2 × 107 total recipient leukocytes per sensitization attempt. Therefore, each marrow donor was exposed to at least 100-fold more recipient leukocytes than what can induce miHA sensitization, and each marrow donor then underwent a second sensitization attempt.
Future experiments will need to test whether infusion of mixed chimeric cells into the marrow donor could be used as a platform to study the still poorly understood danger signals that govern alloimmunization vs. tolerance. These studies can then further test the hypothesis that mixed chimerism renders recipient blood DCs tolerant and incapable of sensitizing the marrow donor. A hypothesis consistent with the underlying notion that mixed chimerism is maintained by T regulatory cells,Citation15,16 and it is increasingly recognized that the presence of T regulatory cells is synonymous with the presence of tolerogenic DCs.Citation42 If future studies confirm the hypothesis, then it remains a testable question whether infusion of mixed chimeric cells into the marrow donor can be used to achieve microchimerism or even mixed chimerism with the associated potential of a transfer of tolerance. With respect to this concept, this study did report some preliminary evidence of microchimerism in the marrow donors following the infusion of mixed chimeric cells. However, a larger cohort is needed to confirm the presence and persistence of microchimerism, and determine if this can be increased or even converted to mixed chimerism with infusions of mixed chimeric cells that contain haematopoietic stem cells. As for this study, the marrow donors did not demonstrate any evidence of tolerance.
Materials and methods
Experimental animals and DLA typing
Random-bred beagles and mini-mongrel cross breeds were raised at the Fred Hutchinson Cancer Research Center (FHCRC), Seattle, WA or purchased commercially. Animals were housed in kennels certified by the American Association for Accreditation of Laboratory Animal Care. All study designs were approved by the Institutional Animal Care and Use Committee. Dog leukocyte antigen (DLA)-typing used highly polymorphic microsatellite markers within DLA class I (DLA 88)Citation43 and class II regions (DLA-DRB1).Citation44,45 The DLA typing for donor-recipient transplant pair H554-H550 were DRB1 6/15 and DLA-88 00402/03201, and for donor-recipient transplant pair H555-H552 were DRB1 6/17 and DLA-88 00402/04901.
Minimal intensity DLA-identical HCT and chimerism analysis
HCT recipients were treated with 300 centigray (cGy) TBI at a dose rate of 7 cGy/minute from a high-energy linear accelerator (6 MV Elekta Precise; Stockholm, Sweden). Within four hours of TBI, bone marrow was collected and infused from their respective DLA-identical siblings as described,Citation46 H550 received 5 × 108 nucleated cells/kg and H552 received 4.8 × 108 nucleated cells/kg. Post-grafting immunosuppression consisting of oral cyclosporine at 15 mg/kg orally twice daily started the day before the marrow infusion and continued for 36 days, and mycophenolate mofetil at 10 mg/kg twice daily injected subcutaneously started on the day of marrow infusion for 28 days, as decribed.Citation47 Chimerism analyses were done on PBMCs and granulocytes following separation of blood on Ficoll (density = 1.074), and quantified by fluorescent variable number of tandem repeat PCR analysis, as described.Citation48 Microchimerism analysis required pre-transplant samples from the donor in order to compare the PCR signal for the recipient, and the robustness of the PCR reaction was further validated using a standard curve created through mixture of donor and recipient DNA.
Marrow donor sensitization
Buffy coat cells from their respective mixed chimera were isolated from 60 mL of heparinized blood. The buffy coat was lysed with ammonium chloride lysing solution (155 mM ammonium chloride, 10 mM sodium bicarbonate, 0.1 mM EDTA), washed twice with phosphate-buffered saline (PBS). Viable infused cells were determined using a hemocytometer and Trypan Blue 0.4% staining (Thermo Fisher Scientific, Waltham, MA). For the first sensitization attempt, half of the cells were suspended in 10 mL of PBS and infused intravenously. The other half was suspended in 200 microliters of PBS and injected subcutaneously into the flank of their respective donors. This was repeated every 10 days for a total of three injections. For the second attempt the same experimental protocol was followed with the exception that half of the cells were injected into the dermis instead of subcutaneous tissue of the flank. Viable cells were determined by trypan blue and acridine orange/ethidium bromide per Cold Spring Harbor Protocols (cshprotocols.cshlp.org). For the third attempt at donor sensitization, subcutaneous placement of a full-thickness 3 × 3 cm recipient skin graft onto the marrow donor was performed under general anesthesia, as described.Citation49,50 Six weeks after the full thickness skin graft, a “modified” skin graft was placed via a small incision in the donor to create a subcutaneous pocket following conscious sedation and local anesthetic as follows: several 8 mm punch biopsies that did not exceed one square centimeter were obtained from the recipient following conscious sedation and local anesthetic;the biopsies were minced and placed into a subcutaneous pocket in the marrow donor that was then stapled closed, as described.Citation51
Donor lymphocyte infusion
Ten days after the last cell infusion or skin graft, marrow donor dogs underwent general anesthesia, central venous catheter placement in the external jugular vein, and collection of 200 mL leukapheresis product, as described (COBE 2997; Blood Component Technology, Lakewood, CO).Citation52,53 Cell counts with differentials were obtained using an ADVIA 2120i (Siemens, Deerfield, IL). Recipients were pre-treated with diphenhydramine and the entire leukapheresis product was slowly infused. Cell counts with differentials were obtained using an ADVIA 2120i (Siemens, Deerfield, IL).
Flow cytometry, cell sorting, and mixed lymphocyte reaction
CD3 cell counts were determined using anti-canine CD3 (17.6F9), as described,Citation17 and reported in . T regulatory cells were evaluated using anti-canine CD4-FITC (CA13.1E4) (Bio-Rad, Hercules, CA), anti-canine CD25-PE (P4A10) (Thermo Fisher, Waltham, MA), and intracellular stained using anti-FOXP3-AF647 (150D) Fix/Perm buffer set (Biolegend, San Diego, CA). For the T regulatory cell analysis, the flow cytometry gates were set based on isotype controls for mouse IgG1-PE and mouse IgG1-AF647 (Southern Biotech, Birmingham, AL). Peripheral blood DCs were defined using anti-DM5-FITC,Citation54 anti-human CD14-PerCP (TuK4) (Thermo Fisher, Waltham, MA), anti-canine CD11c-PE (6A1),Citation55 and anti-canine DLA-DR-AF647 (H81.9).Citation56 Flow cytometry was performed using a FACS Canto II and cell sorting with a FACS ARIAII (BD Biosciences, San Jose, CA). Data was analyzed using FlowJo Software (Treestar Ashland, OR). MLRs were performed with MHC-mismatched stimulator and responder cells as previously described.Citation57,58 The DLA typing for the stimulator H382 was DRB1 1/1 and DLA-88 50801/50801, H597 DRB1 9/15 and DLA-88 01101/01201, H519 DRB1 9/22 and DLA-88 03801/50101, and the normal control stimulator H810 DRB1 1/3 DLA-88 03202/03401. The DLA typing for the normal responder H750 was DRB1 17/19 and DLA-88 04501/04901.
Disclosure of potential conflicts of interest
No potential conflicts of interest were disclosed.
Author contributions
SLR designed experiments, performed the data analysis, and drafted the article. SSG and RS designed the experiments and drafted the article. DAH reviewed the article and assisted in data acquisition.
Funding
The authors are grateful for research funding from the National Institutes of Health, Bethesda, MD, grants P01 CA078902, P30 CA015704, K12 CA076930, P30 DK056465, support from Gabrielle's Angel Foundation for Cancer Research (SLR), and by awards from the Joseph Steiner Krebsstiftung, Bern, Switzerland and Lupin Foundation, Metairie, LA (RS).
References
- Lafferty KJ, Woolnough J. The origin and mechanism of the allograft reaction. Immunol Rev 1977; 35:231-62; PMID:330389; http://dx.doi.org/10.1111/j.1600-065X.1977.tb00241.x
- Roopenian DC. What are minor histocompatibility loci? A new look at an old question (Review). Immunol Today 1992; 13:10; http://dx.doi.org/10.1016/0167-5699(92)90197-F
- Goulmy E, Termijtelen A, Bradley BA, van Rood JJ. Y-antigen killing by T cells of women is restricted by HLA. Nature 1977; 266:544-5; PMID:300847; http://dx.doi.org/10.1038/266544a0
- Storb R, Rudolph RH, Kolb HJ, Graham TC, Mickelson E, Erickson V, Lerner KG, Kolb H, Thomas ED. Marrow grafts between DL-A-matched canine littermates. Transplantation 1973; 15:92-100; PMID:4268054; http://dx.doi.org/10.1097/00007890-197301000-00014
- Weiden PL, Flournoy N, Thomas ED, Prentice R, Fefer A, Buckner CD, Storb R. Antileukemic effect of graft-versus-host disease in human recipients of allogeneic-marrow grafts. N Engl J Med 1979; 300:1068-73; PMID:34792; http://dx.doi.org/10.1056/NEJM197905103001902
- Owen RD. Immunogenetic consequences between vascular anastomoses between bovine twins. Science 1945; 102:400-2; PMID:17755278; http://dx.doi.org/10.1126/science.102.2651.400
- Burnet FM. Sir Frank Macfarlane Burnet - Nobel Lecture (1960): Immunological Recognition of Self. Nobelprizeorg 1960; http://www.nobelprize.org/nobel_prizes/medicine/laureates/1960/burnet-lecture.html
- Medawar P. Peter Medawar - Nobel Lecture (1960): Immunological Tolerance. Nobelprizeorg 1960; http://www.nobelprize.org/nobel_prizes/medicine/laureates/1960/medawar-lecture.html
- Beutler BA. Bruce A. Beutler - Nobel Lecture (2011): How Mammals Sense Infection: From Endotoxin to the Toll-like Receptors. Nobelprizeorg 2011; http://www.nobelprize.org/nobel_prizes/medicine/laureates/2011/beutler-lecture.html
- Hoffmann JA. Jules A. Hoffmann - Nobel Lecture (2011): The Hosts Defense of Insects: A Paradigm for Innate Immunity. Nobelprizeorg 2011; http://www.nobelprize.org/nobel_prizes/medicine/laureates/2011/hoffmann-lecture.html
- Nussenzweig MC. Ralph M. Steinman - Nobel Lecture (2011): Ralph Steinman and the Discovery of Dendritic Cells. Nobelprizeorg 2011; http://www.nobelprize.org/nobel_prizes/medicine/laureates/2011/steinman-lecture.html
- Matzinger P. The danger model: a renewed sense of self. Science 2002; 296:301-5; PMID:11951032; http://dx.doi.org/10.1126/science.1071059
- Gutcher I, Becher B. APC-derived cytokines and T cell polarization in autoimmune inflammation. J Clin Invest 2007; 117:1119-27; PMID:17476341; http://dx.doi.org/10.1172/JCI31720
- Storb R, Yu C, Wagner JL, Deeg HJ, Nash RA, Kiem HP, Leisenring W, Shulman H. Stable mixed hematopoietic chimerism in DLA-identical littermate dogs given sublethal total body irradiation before and pharmacological immunosuppression after marrow transplantation. Blood 1997; 89:3048-54; PMID:9108426
- Lesnikova M, Nikitine A, Pogosov L, Nash RA, Georges GE. Peripheral CD4 + CD25 + regulatory T cells (Treg) block alloreactive host anti-donor T cells in canine mixed hematopoietic chimeras. Blood (ASH Annual Meeting Abstacts) 2005; 106:3101.
- Lesnikova M, Nikitine A, Mason N, Nash RA, Georges GE. Ex vivo expanded T regulatory (Treg) cells block conversion of mixed chimeras to complete donor chimerism. Blood (ASH Annual Meeting Abstracts) 2006; 108:5168.
- Georges GE, Storb R, Thompson JD, Yu C, Gooley T, Bruno B, Nash RA. Adoptive immunotherapy in canine mixed chimeras after nonmyeloablative hematopoietic cell transplantation. Blood 2000; 95:3262-9; PMID:10807798
- Georges GE, Storb R, Zaucha JM, Taranova AG, Gooley T, Nash RA. IL-2 does not enhance the conversion to complete donor chimerism following nonmyeloablative hematopoietic cell transplantation in dogs. Bone Marrow Transplant 2003; 31:1027-31; PMID:12774055; http://dx.doi.org/10.1038/sj.bmt.1704046
- Junghanss C, Takatu A, Little MT, Zaucha JM, Zellmer E, Yunusov M, Sale G, Georges GE, Storb R. Adoptive immunotherapy against kidney targets in dog-leukocyte antigen-identical mixed hematopoietic canine chimeras. Transplantation 2003; 75:268-74; PMID:12589144; http://dx.doi.org/10.1097/01.TP.0000045224.52516.FC
- Graves SS, Hogan WJ, Kuhr C, Diaconescu R, Harkey M, Sale GE, Stone B, Georges GE, Storb R. Adoptive immunotherapy against allogeneic kidney grafts in dogs with stable hematopoietic trichimerism. Biol Blood Marrow Transplant 2008; 14:1201-8; PMID:18940673; http://dx.doi.org/10.1016/j.bbmt.2008.08.005
- van Twuyver E, Kast WM, Mooijaart RJ, Melief CJ, De Waal LP. Induction of transplantation tolerance by intravenous injection of allogeneic lymphocytes across an H-2 class II mismatch. Different mechanisms operate in tolerization across an H-2 class I vs. H-2 class II disparity. Eur J Immunol 1990; 20:441-4; PMID:2311650; http://dx.doi.org/10.1002/eji.1830200232
- Huck SP, Tang SC, Andrew KA, Yang J, Harper JL, Ronchese F. Activation and route of administration both determine the ability of bone marrow-derived dendritic cells to accumulate in secondary lymphoid organs and prime CD8+ T cells against tumors. Cancer Immunol Immunother 2008; 57:63-71; PMID:17609951; http://dx.doi.org/10.1007/s00262-007-0350-z
- Baskic D, Popovic S, Ristic P, Arsenijevic NN. Analysis of cycloheximide-induced apoptosis in human leukocytes: fluorescence microscopy using annexin V/propidium iodide versus acridin orange/ethidium bromide. Cell Biol Int 2006; 30:924-32; PMID:16895761; http://dx.doi.org/10.1016/j.cellbi.2006.06.016
- Bloch EM, Jackman RP, Lee TH, Busch MP. Transfusion-associated microchimerism: the hybrid within. Transfus Med Rev 2013; 27:10-20; PMID:23102759; http://dx.doi.org/10.1016/j.tmrv.2012.08.002
- Nash RA, Yunusov M, Abrams K, Hwang B, Castilla-Llorente C, Chen P, Farivar AS, Georges GE, Hackman RC, Lamm WJ, et al. Immunomodulatory effects of mixed hematopoietic chimerism: immune tolerance in canine model of lung transplantation. Am J Transplant 2009; 9:1037-47; PMID:19422333; http://dx.doi.org/10.1111/j.1600-6143.2009.02619.x
- Mielcarek M, Kucera KA, Nash RA, Torok-Storb B, McKenna HJ. Identification and characterization of canine dendritic cells generated in vivo. Biol Blood Marrow Transplant 2007; 13:1286-93; PMID:17950915; http://dx.doi.org/10.1016/j.bbmt.2007.07.010
- Storb R, Thomas ED, Buckner CD, Appelbaum FR, Clift RA, Deeg HJ, Doney K, Hansen JA, Prentice RL, Sanders JE, et al. Marrow transplantation for aplastic anemia. Semin Hematol 1984; 21:27-35; PMID:6367054
- Weiden PL, Storb R, Kolb HJ, Graham TC, Kao G, Thomas ED. Effect of time on sensitization to hemopoietic grafts by preceding blood transfusions. Transplantation 1975; 19:240-4; PMID:1094638; http://dx.doi.org/10.1097/00007890-197503000-00007
- Deeg HJ, Aprile J, Storb R, Graham TC, Hackman R, Appelbaum FR, Schuening F. Functional dendritic cells are required for transfusion-induced sensitization in canine marrow graft recipients. Blood 1988; 71:1138-40.
- Storb R, Epstein RB, Rudolph RH, Thomas ED. The effect of prior transfusion on marrow grafts between histocompatible canine siblings. J Immunol 1970; 105:627-33.
- Storb R, Kolb HJ, Graham TC, Kane PJ, Thomas ED. The effect of prior blood transfusions on hemopoietic grafts from histoincompatible canine littermates. Transplantation 1972; 14:248-52; PMID:4558907; http://dx.doi.org/10.1097/00007890-197208000-00017
- Weiden PL, Storb R, Thomas ED, Graham TC, Lerner KG, Buckner CD, Fefer A, Neiman PE, Clift RA. Preceding transfusions and marrow graft rejection in dogs and man. Transplant Proc 1976; 8:551-4; PMID:793113
- Storb R, Weiden PL, Deeg HJ, Graham TC, Atkinson K, Slichter SJ, Thomas ED. Rejection of marrow from DLA-identical canine littermates given transfusions before grafting: antigens involved are expressed on leukocytes and skin epithelial cells but not on platelets and red blood cells. Blood 1979; 54:477-84; PMID:378293
- Storb R, Deeg HJ, Atkinson K, Weiden PL, Sale G, Colby R, Thomas ED. Cyclosporin-A abrogates transfusion-induced sensitization and prevents marrow graft rejection in DLA-identical canine littermates. Blood 1982; 60:524-6; PMID:7046849
- Storb R, Deeg HJ. Failure of allogeneic canine marrow grafts after total body irradiation: Allogeneic resistance vs transfusion induced sensitization. Transplantation 1986; 42:571-80; PMID:3538545; http://dx.doi.org/10.1097/00007890-198612000-00001
- Bean MA, Storb R, Graham T, Raff R, Sale GE, Schuening F, Appelbaum FR. Prevention of transfusion-induced sensitization to minor histocompatibility antigens on DLA-identical canine marrow grafts by gamma irradiation of marrow donor blood. Transplantation 1991; 52:956-60; PMID:1836286; http://dx.doi.org/10.1097/00007890-199112000-00004
- Storb R, Bean M, Appelbaum F, Schuening F, Graham T, Raff R. Treatment of marrow donor blood products with gamma-irradiation prevents transfusion-induced sensitization to DLA-identical marrow grafts. Transplant Proc 1991; 23:1697-8; PMID:1824901
- Bean MA, Graham T, Appelbaum FR, Deeg HJ, Schuening F, Sale GE, Storb R. Gamma-irradiation of pretransplant blood transfusions from unrelated donors prevents sensitization to minor histocompatibility antigens on dog leukocyte antigen-identical canine marrow grafts. Transplantation 1994; 57:423-6; PMID:8108879; http://dx.doi.org/10.1097/00007890-199402150-00019
- Bean MA, Graham T, Appelbaum FR, Deeg HJ, Schuening F, Sale GE, Leisenring W, Pepe M, Storb R. Gamma radiation of blood products prevents rejection of subsequent DLA-identical marrow grafts: Tolerance vs. abrogation of sensitization to non-DLA antigens. Transplantation 1996; 61:334-5; PMID:8600648; http://dx.doi.org/10.1097/00007890-199601270-00031
- Deeg HJ, Slichter SJ, Storb R. Transfusion-induced allosensitization in marrow transplant recipients: Mechanisms and preventive measures. In: S. J. Baum, GWSa FT, ed. Recent Advances and Future Directions in Bone Marrow Transplantation, (Experimental Hematology Today–1987). New York: Springer Verlag, 1988:18-23.
- Ladiges WC, Keast M, Appelbaum F, Storb R. Phenotypic characterization of canine lymphoma, using monoclonal antibodies and a microlymphocytotoxicity assay. Am J Vet Res 1988; 49:870-2; PMID:3400923
- Maldonado RA, von Andrian UH. How tolerogenic dendritic cells induce regulatory T cells. Adv Immunol 2010; 108:111-65; PMID:21056730; http://dx.doi.org/10.1016/B978-0-12-380995-7.00004-5
- Venkataraman GM, Stroup P, Graves SS, Storb R. An improved method for dog leukocyte antigen 88 typing and two new major histocompatibility complex class I alleles, DLA-88*01101 and DLA-88*01201. Tissue Antigens 2007; 70:53-7; PMID:17559581; http://dx.doi.org/10.1111/j.1399-0039.2007.00839.x
- Wagner JL, Burnett RC, DeRose SA, Francisco LV, Storb R, Ostrander EA. Histocompatibility testing of dog families with highly polymorphic microsatellite markers. Transplantation 1996; 62:876-7; PMID:8824495; http://dx.doi.org/10.1097/00007890-199609270-00032
- Wagner JL, Works JD, Storb R. DLA-DRB1 and DLA-DQB1 histocompatibility typing by PCR-SSCP and sequencing (Brief Communication). Tissue Antigens 1998; 52:397-401; PMID:9820606; http://dx.doi.org/10.1111/j.1399-0039.1998.tb03063.x
- Ladiges WC, Storb R, Thomas ED. Canine models of bone marrow transplantation. Lab Anim Sci 1990; 40:15-Nov.
- Sorror ML, Leisenring W, Mielcarek M, Baron F, Diaconescu R, Hogan WJ, Graves SS, Storb R. Intensified postgrafting immunosuppression failed to assure long-term engraftment of dog leukocyte antigen-identical canine marrow grafts after 1 gray total body irradiation. Transplantation 2008; 85:1023-9; PMID:18408584; http://dx.doi.org/10.1097/TP.0b013e318169be24
- Yu C, Ostrander E, Bryant E, Burnett R, Storb R. Use of (CA) n polymorphisms to determine the origin of blood cells after allogeneic canine marrow grafting. Transplantation 1994; 58:701-6; PMID:7940690; http://dx.doi.org/10.1097/00007890-199409270-00011
- Weiden PL, Storb R, Tsoi MS, Graham TC, Lerner KG, Thomas ED. Infusion of donor lymphocytes into stable canine radiation chimeras: Implications for mechanism of transplantation tolerance. J Immunol 1976; 116:1212-9.
- Yunusov MY, Kuhr CS, Georges GE, Hogan WJ, Taranova AG, Lesnikova M, Kim YS, Abrams K, Hwang B, Sale GE, et al. Partial donor-specific tolerance to delayed skin grafts after rejection of hematopoietic cell graft. Transplantation 2006; 82:629-37; PMID:16969285; http://dx.doi.org/10.1097/01.tp.0000229449.09622.28
- Buckner CD, Clift RA, Sanders JE, Williams B, Gray M, Storb R, Thomas ED. ABO-incompatible marrow transplants. Transplantation 1978; 26:233-8; PMID:30194; http://dx.doi.org/10.1097/00007890-197810000-00006
- Sandmaier BM, Storb R, Santos EB, Krizanac-Bengez L, Lian T, McSweeney PA, Yu C, Schuening FG, Deeg HJ, Graham T. Allogeneic transplants of canine peripheral blood stem cells mobilized by recombinant canine hematopoietic growth factors. Blood 1996; 87:3508-13; PMID:8605371
- Lupu M, Gooley T, Zellmer E, Graves SS, Storb R. Principles of peripheral blood mononuclear cell apheresis in a preclinical canine model of hematopoietic cell transplantation. J Vet Intern Med 2008; 22:74-82; PMID:18289292; http://dx.doi.org/10.1111/j.1939-1676.2007.0016.x
- Sandmaier BM, Schuening FG, Bianco JA, Rosenman SJ, Bernstein I, Goehle S, Storb R, Appelbaum FR. Biochemical characterization of a unique canine myeloid antigen. Leukemia 1991; 5:125-30; PMID:2020194
- Danilenko DM, Moore PF, Rossitto PV. Canine leukocyte cell adhesion molecules (LeuCAMs): characterization of the CD11/CD18 family. Tissue Antigens 1992; 40:13-21; PMID:1359668; http://dx.doi.org/10.1111/j.1399-0039.1992.tb01952.x
- Ladiges WC, Pesando JM, Severns E, Longton G, Deeg HJ, Storb R. Selective inhibition of the canine mixed lymphocyte response by HLA-DR and DP-reactive monoclonal antibodies. Transplantation 1988; 45:484-8; PMID:2964111; http://dx.doi.org/10.1097/00007890-198802000-00048
- Lee WS, Suzuki Y, Graves SS, Iwata M, Venkataraman GM, Mielcarek M, Peterson LJ, Ikehara S, Torok-Storb B, Storb R. Canine bone marrow-derived mesenchymal stromal cells suppress alloreactive lymphocyte proliferation in vitro but fail to enhance engraftment in canine bone marrow transplantation. Biol Blood Marrow Transplant 2011; 17:465-75; PMID:20457265; http://dx.doi.org/10.1016/j.bbmt.2010.04.016
- Graves SS, Stone DM, Loretz C, Peterson LJ, Lesnikova M, Hwang B, Georges GE, Nash R, Storb R. Antagonistic and agonistic anti-canine CD28 monoclonal antibodies: tools for allogeneic transplantation. Transplantation 2011; 91:833-40; PMID:21343872; http://dx.doi.org/10.1097/TP.0b013e31820f07ff