ABSTRACT
The gut microbiota has coevolved with the host for hundreds of millions of years, playing a beneficial role in host health. Human parasitic helminths are widespread and pose a pervasive global public health issue. Although Type 2 immunity provides partial resistance to helminth infections, the composition of the gut microbiota can change correspondingly. Therefore, it raises the question of what role the gut microbiota plays during helminth infection. Akkermansia muciniphila has emerged as a notable representative of beneficial microorganisms in the gut microbiota. Recent studies indicate that A. muciniphila is not merely associated with helminth infection but is also causally linked to infection. Here, we provide an overview of the crosstalk between A. muciniphila and enteric helminth infection. Our goal is to enhance our understanding of the interplay among A. muciniphila, helminths, and their hosts while also exploring the potential underlying mechanisms.
1. Introduction
Approximately 1.5 billion individuals are infected with helminth parasites, making helminths a pervasive global public health issue.Citation1,Citation2 In addition to helminths, commensal bacteria have coevolved with their hosts. The gut microbiome, comprising around 10 million bacteria, plays a critical role in maintaining the healthy metabolic status of an individual.Citation3,Citation4 The interaction between helminths and gut bacteria is crucial for determining the development of helminth infections.Citation5,Citation6
Among these commensal bacteria, Akkermansia muciniphila has emerged as a critical bacterium. A recent review demonstrated that the absence or reduction of A. muciniphila is associated with inflammatory disorders.Citation7 A. muciniphila is vital for maintaining a healthy intestinal barrier and has been extensively studied in mice, as well as in an initial trial involving humans,Citation8–13 Gaps in existing knowledge regarding the crosstalk between A. muciniphila and enteric helminths are being filled. In this review, we discuss the effect of enteric helminth infections on A. muciniphila and the role of A. muciniphila in enteric helminth infections. We aim to improve our understanding of A. muciniphila-helminth – host interactions and identify the possible underlying mechanisms.
2. Helminth-induced immune responses and the gut microbiota
Helminth parasites typically trigger robust type 2 immune responses that play a crucial role in eliminating these parasites.Citation14 These responses become crucial as multicellular helminths migrate through host tissues.Citation15 The key characteristics of helminth infections include goblet cell hyperplasia and mucin secretion in the intestines. This immune response is critical for the expulsion of helminths through the maintenance of the epithelial barrier.Citation16 Goblet cells respond to type 2 cytokines by generating mucus, with well-established roles for IL-13 and IL-4.Citation16,Citation17 In the absence of IL-4 and IL-13, efficient expulsion of helminths from the mouse intestine is hindered ,Citation18 , .Citation19,Citation20 Additionally, IL-5 deficiency in mice results in a higher worm load during both acute and chronic infections.Citation21,Citation22 These findings underscore the importance of type 2 cytokines and their signaling pathways in limiting helminth infections. Accumulating evidence suggests that the microbiota plays a pivotal role in maintaining host homeostasis, exerting significant effects on numerous disease mechanisms.Citation23 Research on host – helminth interactions has focused on host-associated gut microbiomes.Citation24 Notably, Trichuris muris larvae are unable to develop and colonize germ-free mice, underscoring the essential role of bacterial microbiota in establishing infection.Citation25 The gut microbiome undergoes alterations during T. muris infection associated with Th2 responses.Citation26 Specifically, T. muris infection-associated goblet cell activation restricts the colonization of Bacteroides vulgatus, a common member of the gut microbiota. This restriction is attributed to the activation of a robust Th2 immune response. Deworming enhances the abundance of Bacteroidales, suggesting a causal relationship between helminth infections and microbial diversity. Trichinella spiralis-induced strong type 2 immunity still occurs independently of changes in the microbiota in germ-free mice.Citation27 However, fecal bacterial transplantation from healthy mice to T. spiralis-infected mice can increase the number of goblet cells but not the expression of IL-4 and IL-13 at day 3 post infection.Citation28 Along these lines, the gut microbiota may contribute to mucus-producing goblet cell function in deworming, independent of type 2 immunity, which necessitates further investigation.
3. The impact of helminth infections on A. muciniphila abundance
The gastrointestinal tract relies on a crucial defense mechanism known as the mucus barrier. The gut microbiota, considered a vital factor influencing host health, plays a pivotal role in altering the properties of the mucus layer.Citation29 Researchers at the Wageningen Laboratory of Microbiology have made groundbreaking discoveries, identifying A. muciniphila as a novel species within the genus Akkermansia, phylum Verrucomicrobiota, commonly inhabiting the gut mucus layer.Citation30 A. muciniphila has been found in the human intestine since early life, without causing harm to the host.Citation31,Citation32 Remarkably, decreased abundance of A. muciniphila is associated with the occurrence of various diseases and physiological changes, such as obesity, diabetes mellitus, and inflammatory bowel disease,Citation33–35 Researchers are particularly intrigued by the interaction between A. muciniphila and infectious diseases, especially helminth infections. A thorough qualitative systematic review of human studies revealed a strong association between Akkermansia spp. and helminth infection.Citation36 For instance, individuals with gastrointestinal helminths in Sri Lanka exhibit a significant increase in A. muciniphila compared to uninfected individuals.Citation37 Similar observations have been reported in several helminth-infected mouse models. In mice, Heligmosomoides polygyrus infection increases the relative abundance of A. muciniphila.Citation38,Citation39 Helminth-induced type 2 immunity plays a role in modulating the gut microbiota, including A. muciniphila.Citation39 Another helminth that colonizes the intestine, Trichuris muris, can significantly increase the abundance of Verrucomicrobiales.Citation26 During infection with T. spiralis, which inhabits the small intestine for approximately two weeks, the abundance of Akkermansia increases in helminth-infected mice,Citation40–42 Interestingly, Schistosoma japonicum, which exhibits tropism for the liver, leads to an increase in Akkermansia abundance during S. japonicum-induced liver cirrhosis.Citation43 Furthermore, in a mouse model of percutaneous infection with Schistosoma mansoni, the infected mice exhibited a greater abundance of Akkermansia.Citation44,Citation45
Collectively, these findings suggest that the abundance of A. muciniphila increases during helminth infection, potentially resulting from interactions between the helminths and their hosts. Helminth Heligmosomoides polygyrus infection promotes the induction of alternatively activated (M2) macrophages and helminth-induced M2 cells can increase the abundance of A. muciniphila. An enhanced type 2 immune response leading to increased intestinal mucus secretion is a distinctive feature of helminth infections.Citation46 allows A. muciniphila to reach a more favorable growth environment.
4. The impact of A. muciniphila on helminth infections
A positive relationship between A. muciniphila colonization and helminth infection restrain has been determined (). Our previous study demonstrated that A. muciniphila exerts anthelmintic effects against T. spiralis infection.Citation47 A. muciniphila improved intestinal mucus secretion, and pasteurized A. muciniphila was more effective than live A. muciniphila. Another study showed that these compounds were retained even when inactivated by pasteurization.Citation11 However, there is insufficient evidence to explain why pasteurized A. muciniphila often exhibits better efficacy. One possible explanation is that pasteurization not only has no effect on mucus-promoting bacterial components but also eliminates intestinal invasion caused by bacteria. This phenomenon has paved the way for making bacteria more suitable for various applications. To date, no studies have systematically explored the role of A. muciniphila in helminth infections.
Figure 1. Akkermansia muciniphila is involved in mucin production by goblet cells independent on type 2 immunity.
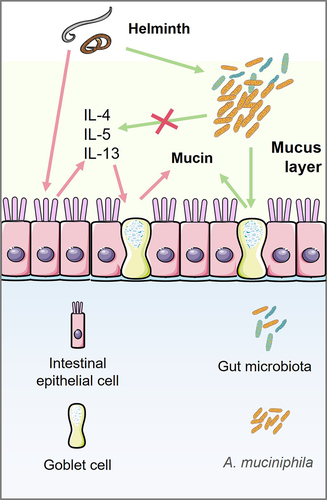
Furthermore, infection with helminth T. spiralis causes cardiac fibrosis (). Recent metagenomics analysis revealed a higher presence of A. muciniphila in the small intestine of infected mice. Interestingly, antibiotic treatment exacerbated helminth-induced cardiac fibrosis, and the dominant species, A. muciniphila, during T. spiralis infection appeared to positively influence cardiac fibrosis outcomes.Citation40 Schistosoma is also one of the major pathogens that causes fibrosis in the liver.Citation48,Citation49 As mentioned in above section, the expansion of A. muciniphila in humans and mice is induced by infection with different genera of Schistosoma, such as S. mansoni and S. japonicum,Citation43–45 Treatment with A. muciniphila protects against liver fibrosis induced by a high-fat diet and carbon tetrachloride administration.Citation50 A. muciniphila has shown potential for ameliorating liver inflammation and fibrosis in NEMO∆hepa mice prone to liver carcinogenesis.Citation51 However, the role of A. muciniphila in the development of Schistosoma-induced liver fibrosis has not been determined. Additionally, it has been observed that compounds present in the outer membrane of A. muciniphila (Amuc_1100) mechanically bind to the toll-like receptor (TLR) 2.Citation11 Our previous studies have confirmed the involvement of TLR2 in the effects of pasteurized A. muciniphila on helminth infection.Citation40,Citation47 We conducted an experiment involving TLR2 knockout mice and found that treatment with pasteurized A. muciniphila failed to alleviate the helminth burden and pathology associated with T. spiralis infection.Citation40,Citation47
Figure 2. Akkermansia muciniphila plays a role in protection against Trichinella spiralis infection.
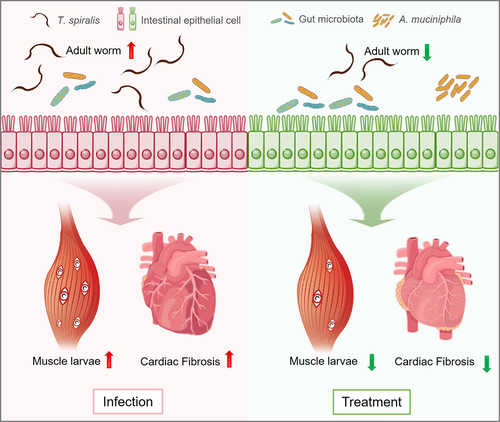
While A. muciniphila has a deworming effect, it does not enhance the type 2 immune response, as evidenced by no increase in IL-4 concentration.Citation52 No evidence supports a correlation between A. muciniphila and other type 2 cytokines. Although A. muciniphila cannot induce Th2 cell response,Citation53 indicating the presence of an alternative type 2 immunity-independent mechanism for the mucin-promoting function of A. muciniphila, more thorough mechanistic studies using a type 2 immunity-deficient helminth-infected model should be conducted in future. A recent study indicated that 15:0-i15:0 phosphatidylethanolamine, a lipid found in the cell membrane of A. muciniphila, increases the release of tumor necrosis factor (TNF)-α in a TLR2-dependent manner.Citation54 Moreover, TNF-α plays a crucial role in the expulsion of T. spiralis,Citation55 suggesting its putative involvement in the capacity of A. muciniphila to expel T. spiralis. A. muciniphila promotes the differentiation of secretory intestinal epithelial cell lineages, accelerates intestinal epithelial regeneration and increases the number of mucin-producing goblet cells in the intestines, consequently enhancing mucus production during gut damage caused by radiation and methotrexate.Citation56 Supplementation with A. muciniphila prevents the decrease in thickness of the colonic mucus layer that occurs with aging.Citation57 A. muciniphila promotes the development of goblet cells in the intestine, resulting in increased mucin productionCitation58 and enhancing the integrity of the gut barrier.Citation8 A. muciniphila also upregulates the genes responsible for maintaining intestinal barrier function (e.g., MUC2, BIRC3, and TNFAIP3) and improves intestinal homeostasis by activating the ALPK1/TIFA/TRAF6 axis.Citation59 Furthermore, A. muciniphila stimulates NLRP6 expression and enhances autophagy in goblet cells, thereby promoting the production of mucin in the context of inflammatory bowel disease.Citation58 These findings provide mechanistic and novel insights into A. muciniphila-induced mucus production. However, the mechanisms underlying helminth infection have not yet been fully elucidated.
5 Conclusions
A. muciniphila-induced regulation of gut barrier function, including mucus production, has been identified by various research teams. Although the relevance of A. muciniphila in helminth infections has been described in many publications, the studies about relationship between A. muciniphila colonization and helminth infection restrain have just begun. Indeed, although some studies have argued that mucin-degrading bacteria have a risk of reducing the mucus layer due to their capacity for mucus consumption,Citation60,Citation61 improved mucus production by A. muciniphila during helminth T. spiralis infection is crucial for its anthelmintic effects. Given that increasing goblet cell mucus secretion constitutes part of the “weep and sweep” response that develops to promote helminth expulsion,Citation14 helminth infection could be considered a model for investigating the crosstalk between A. muciniphila and mucus. The protective role of A. muciniphila and its associated molecules against infection with other helminths should be explored further. Moreover, the detailed mechanism of the type 2 immune independent mechanism by which A. muciniphila regulates mucin function in helminth infections is worth exploring in the future.
Author contributions
JW, XZ, XL and XJ performed the literature review and wrote the manuscript. XL and XJ revised the manuscript. The authors read and approved the final manuscript.
Acknowledgments
We thank Dr. Mingyuan Liu and Dr. Xiaolei Liu at the College of Veterinary Medicine, Jilin University for preparing the manuscript.
Disclosure statement
No potential conflict of interest was reported by the author(s).
Data availability statement
All data are included in the manuscript.
Additional information
Funding
References
- Santano R, Rubio R, Grau-Pujol B, Escola V, Muchisse O, Cuamba I, Vidal M, Cisteró P, Ruiz-Olalla G, Aguilar R. et al. Plasmodium falciparum and helminth coinfections increase ige and parasite-specific IgG responses. Microbiol Spectr. 2021;9(3):e0110921. doi:10.1128/Spectrum.01109-21.
- Disease GBD, Injury I, Prevalence C, Abay SM, Abbafati C, Abbasi N, Abbastabar H, Abd-Allah F, Abdela J, Abdelalim A. Global, regional, and national incidence, prevalence, and years lived with disability for 354 diseases and injuries for 195 countries and territories, 1990–2017: a systematic analysis for the global burden of disease study 2017. Lancet. 2018;392(10159):1789–8. doi:10.1016/S0140-6736(18)32279-7.
- Cani PD. Human gut microbiome: hopes, threats and promises. Gut. 2018;67(9):1716–1725. doi:10.1136/gutjnl-2018-316723.
- El Aidy S, Hooiveld G, Tremaroli V, Backhed F, Kleerebezem M. The gut microbiota and mucosal homeostasis: colonized at birth or at adulthood, does it matter? Gut Microbes. 2013;4(2):118–124. doi:10.4161/gmic.23362.
- Mutapi F. The gut microbiome in the helminth infected host. Trends Parasitol. 2015;31(9):405–406. doi:10.1016/j.pt.2015.06.003.
- Rapin A, Harris NL. Helminth–bacterial interactions: cause and consequence. Trends Immunol. 2018;39(9):724–733. doi:10.1016/j.it.2018.06.002.
- Cani PD, Depommier C, Derrien M, Everard A, de Vos WM. Akkermansia muciniphila: paradigm for next-generation beneficial microorganisms. Nat Rev Gastroenterol Hepatol. 2022;19(10):625–637. doi:10.1038/s41575-022-00631-9.
- Everard A, Belzer C, Geurts L, Ouwerkerk JP, Druart C, Bindels LB, Guiot Y, Derrien M, Muccioli GG, Delzenne NM. et al. Cross-talk between Akkermansia muciniphila and intestinal epithelium controls diet-induced obesity. Proc Natl Acad Sci U S A. 2013;110(22):9066–9071. doi:10.1073/pnas.1219451110.
- Li J, Lin S, Vanhoutte PM, Woo CW, Xu A. Akkermansia muciniphila protects against atherosclerosis by preventing metabolic endotoxemia-induced inflammation in Apoe −/− Mice. Circulation. 2016;133(24):2434–2446. doi:10.1161/CIRCULATIONAHA.115.019645.
- Hanninen A, Toivonen R, Poysti S, Belzer C, Plovier H, Ouwerkerk JP, Emani R, Cani PD, De Vos WM. Akkermansia muciniphila induces gut microbiota remodelling and controls islet autoimmunity in NOD mice. Gut. 2018;67(8):1445–1453. doi:10.1136/gutjnl-2017-314508.
- Plovier H, Everard A, Druart C, Depommier C, Van Hul M, Geurts L, Chilloux J, Ottman N, Duparc T, Lichtenstein L. et al. A purified membrane protein from Akkermansia muciniphila or the pasteurized bacterium improves metabolism in obese and diabetic mice. Nat Med. 2017;23(1):107–113. doi:10.1038/nm.4236.
- Depommier C, Van Hul M, Everard A, Delzenne NM, De Vos WM, Cani PD. Pasteurized Akkermansia muciniphila increases whole-body energy expenditure and fecal energy excretion in diet-induced obese mice. Gut Microbes. 2020;11(5):1231–1245. doi:10.1080/19490976.2020.1737307.
- Dao MC, Everard A, Aron-Wisnewsky J, Sokolovska N, Prifti E, Verger EO, Kayser BD, Levenez F, Chilloux J, Hoyles L. et al. Akkermansia muciniphila and improved metabolic health during a dietary intervention in obesity: relationship with gut microbiome richness and ecology. Gut. 2016;65(3):426–436. doi:10.1136/gutjnl-2014-308778.
- Anthony RM, Rutitzky LI, Urban JF Jr., Stadecker MJ, Gause WC. Protective immune mechanisms in helminth infection. Nat Rev Immunol. 2007;7(12):975–987. doi:10.1038/nri2199.
- Chen F, Liu Z, Wu W, Rozo C, Bowdridge S, Millman A, Van Rooijen N, Urban JF, Wynn TA, Gause WC. et al. An essential role for TH2-type responses in limiting acute tissue damage during experimental helminth infection. Nat Med. 2012;18(2):260–266. doi:10.1038/nm.2628.
- Grencis RK. Immunity to helminths: resistance, regulation, and susceptibility to gastrointestinal nematodes. Annu Rev Immunol. 2015;33(1):201–225. doi:10.1146/annurev-immunol-032713-120218.
- Medzhitov R. Recognition of microorganisms and activation of the immune response. Nature. 2007;449(7164):819–826. doi:10.1038/nature06246.
- Finkelman FD, Shea-Donohue T, Goldhill J, Sullivan CA, Morris SC, Madden KB, Gause WC, Urban JF. Cytokine regulation of host defense against parasitic gastrointestinal nematodes: lessons from studies with rodent models. Annu Rev Immunol. 1997;15(1):505–533. doi:10.1146/annurev.immunol.15.1.505.
- Urban JF Jr., Noben-Trauth N, Donaldson DD, Madden KB, Morris SC, Collins M, Finkelman FD. IL-13, IL-4Rα, and Stat6 are required for the expulsion of the gastrointestinal nematode parasite nippostrongylus brasiliensis. Immunity. 1998;8(2):255–264. doi:10.1016/S1074-7613(00)80477-X.
- Voehringer D, Reese TA, Huang X, Shinkai K, Locksley RM. Type 2 immunity is controlled by IL-4/IL-13 expression in hematopoietic non-eosinophil cells of the innate immune system. J Exp Med. 2006;203(6):1435–1446. doi:10.1084/jem.20052448.
- Volkmann L, Bain O, Saeftel M, Specht S, Fischer K, Brombacher F, Matthaei KI, Hoerauf A. Murine filariasis: interleukin 4 and interleukin 5 lead to containment of different worm developmental stages. Med Microbiol Immunol. 2003;192(1):23–31. doi:10.1007/s00430-002-0155-9.
- Licona-Limon P, Henao-Mejia J, Temann AU, Gagliani N, Licona-Limon I, Ishigame H, Hao L, Herbert D, Flavell R. Th9 cells drive host immunity against gastrointestinal worm infection. Immunity. 2013;39(4):744–757. doi:10.1016/j.immuni.2013.07.020.
- Cho I, Blaser MJ. The human microbiome: at the interface of health and disease. Nat Rev Genet. 2012;13(4):260–270. doi:10.1038/nrg3182.
- Loke P, Harris NL. Networking between helminths, microbes, and mammals. Cell Host Microbe. 2023;31(4):464–471. doi:10.1016/j.chom.2023.02.008.
- White EC, Houlden A, Bancroft AJ, Hayes KS, Goldrick M, Grencis RK, Roberts IS. Manipulation of host and parasite microbiotas: survival strategies during chronic nematode infection. Sci Adv. 2018;4(3):eaap7399. doi:10.1126/sciadv.aap7399.
- Ramanan D, Bowcutt R, Lee SC, Tang MS, Kurtz ZD, Ding Y, Honda K, Gause WC, Blaser MJ, Bonneau RA. et al. Helminth infection promotes colonization resistance via type 2 immunity. Science. 2016;352(6285):608–612. doi:10.1126/science.aaf3229.
- Osborne LC, Monticelli LA, Nice TJ, Sutherland TE, Siracusa MC, Hepworth MR, Tomov VT, Kobuley D, Tran SV, Bittinger K. et al. Coinfection. Virus-helminth coinfection reveals a microbiota-independent mechanism of immunomodulation. Science. 2014;345(6196):578–582. doi:10.1126/science.1256942.
- Li C, Liu Y, Liu X, Bai X, Jin X, Xu F, Chen H, Zhang Y, Vallee I, Liu M. et al. The gut microbiota contributes to changes in the host immune response induced by Trichinella spiralis. PLoS Negl Trop Dis. 2023;17(8):e0011479. doi:10.1371/journal.pntd.0011479.
- Paone P, Cani PD. Mucus barrier, mucins and gut microbiota: the expected slimy partners? Gut. 2020;69(12):2232–2243. doi:10.1136/gutjnl-2020-322260.
- Derrien M, Vaughan EE, Plugge CM, de Vos WM. Akkermansia muciniphila gen. nov., sp. nov., a human intestinal mucin-degrading bacterium. Int J Syst Evol Microbiol. 2004;54(5):1469–1476. doi:10.1099/ijs.0.02873-0.
- Derrien M, Collado MC, Ben-Amor K, Salminen S, de Vos WM. The mucin degrader Akkermansia muciniphila is an abundant resident of the human intestinal tract. Appl Environ Microbiol. 2008;74(5):1646–1648. doi:10.1128/AEM.01226-07.
- Derrien M, Van Baarlen P, Hooiveld G, Norin E, Muller M, de Vos WM. Modulation of mucosal immune response, tolerance, and proliferation in mice colonized by the mucin-degrader Akkermansia muciniphila. Front Microbiol. 2011;2:166. doi:10.3389/fmicb.2011.00166.
- Everard A, Lazarevic V, Gaia N, Johansson M, Stahlman M, Backhed F, Delzenne NM, Schrenzel J, François P, Cani PD. et al. Microbiome of prebiotic-treated mice reveals novel targets involved in host response during obesity. Isme J. 2014;8(10):2116–2130. doi:10.1038/ismej.2014.45.
- Macchione IG, Lopetuso LR, Ianiro G, Napoli M, Gibiino G, Rizzatti G. et al. Key player in metabolic and gastrointestinal disorders. Eur Rev Med Pharmaco. 2019;23:8075–8083.
- Zhang X, Shen D, Fang Z, Jie Z, Qiu X, Zhang C, Chen Y, Ji L. Human gut microbiota changes reveal the progression of glucose intolerance. PLoS One. 2013;8(8):e71108. doi:10.1371/journal.pone.0071108.
- Kupritz J, Angelova A, Nutman TB, Gazzinelli-Guimaraes PH, Sibley LD. Helminth-induced human gastrointestinal dysbiosis: a systematic review and meta-analysis reveals insights into altered taxon diversity and microbial gradient collapse. mBio. 2021;12(6):e0289021. doi:10.1128/mBio.02890-21.
- Jenkins TP, Rathnayaka Y, Perera PK, Peachey LE, Nolan MJ, Krause L, Rajakaruna RS, Cantacessi C. Infections by human gastrointestinal helminths are associated with changes in faecal microbiota diversity and composition. PLoS One. 2017;12(9):e0184719. doi:10.1371/journal.pone.0184719.
- Su C, Su L, Li Y, Long SR, Chang J, Zhang W, Walker WA, Xavier RJ, Cherayil BJ, Shi HN. et al. Helminth-induced alterations of the gut microbiota exacerbate bacterial colitis. Mucosal Immunol. 2018;11(1):144–157. doi:10.1038/mi.2017.20.
- Su CW, Chen CY, Jiao L, Long SR, Mao T, Ji Q, O’Donnell S, Stanton C, Zheng S, Walker WA. et al. Helminth-induced and Th2-dependent alterations of the gut microbiota attenuate obesity caused by high-fat diet. Cell Mol Gastroenterol Hepatol. 2020;10(4):763–778. doi:10.1016/j.jcmgh.2020.06.010.
- Wang J, Liu X, Sun R, Mao H, Liu M, Jin X, Gause WC. Akkermansia muciniphila participates in the host protection against helminth-induced cardiac fibrosis via TLR2. PLoS Pathog. 2023;19(10):e1011683. doi:10.1371/journal.ppat.1011683.
- Liu S, Pan J, Meng X, Zhu J, Zhou J, Zhu X. Trichinella spiralis infection decreases the diversity of the intestinal flora in the infected mouse. J Microbiol Immunol Infect. 2021;54(3):490–500. doi:10.1016/j.jmii.2019.09.009.
- Xie Y, Xu D, Yan S, Hu X, Chen S, Guo K, Wang J, Chen Q, Guan W. The impact of MIF deficiency on alterations of fecal microbiota in C57BL/6 mice induced by Trichinella spiralis infection. FASEB J. 2023;37(10):e23202. doi:10.1096/fj.202300179RR.
- Gui QF, Jin HL, Zhu F, Lu HF, Zhang Q, Xu J, Yang Y-M, Xiao C. Gut microbiota signatures in Schistosoma japonicum infection-induced liver cirrhosis patients: a case–control study. Infect Dis Poverty. 2021;10(1):10. doi:10.1186/s40249-021-00821-8.
- Floudas A, Aviello G, Schwartz C, Jeffery IB, O’Toole PW, Fallon PG, Herbert DR. Schistosoma mansoni worm infection regulates the intestinal microbiota and susceptibility to colitis. Infect Immun. 2019;87(8). doi:10.1128/IAI.00275-19.
- Jenkins TP, Peachey LE, Ajami NJ, MacDonald AS, Hsieh MH, Brindley PJ, Cantacessi C, Rinaldi G. Schistosoma mansoni infection is associated with quantitative and qualitative modifications of the mammalian intestinal microbiota. Sci Rep. 2018;8(1):12072. doi:10.1038/s41598-018-30412-x.
- Sharpe C, Thornton DJ, Grencis RK. A sticky end for gastrointestinal helminths; the role of the mucus barrier. Parasite Immunol. 2018;40(4):e12517. doi:10.1111/pim.12517.
- Jin X, Liu Y, Wang J, Wang X, Tang B, Liu M, Liu X. β-glucan-triggered Akkermansia muciniphila expansion facilitates the expulsion of intestinal helminth via TLR2 in mice. Carbohydr Polym. 2022;275:118719. doi:10.1016/j.carbpol.2021.118719.
- Colley DG, Bustinduy AL, Secor WE, King CH. Human schistosomiasis. Lancet. 2014;383(9936):2253–2264. doi:10.1016/S0140-6736(13)61949-2.
- McManus DP, Bergquist R, Cai P, Ranasinghe S, Tebeje BM, You H. Schistosomiasis—from immunopathology to vaccines. Semin Immunopathol. 2020;42(3):355–371. doi:10.1007/s00281-020-00789-x.
- Keshavarz Azizi Raftar S, Ashrafian F, Yadegar A, Lari A, Moradi HR, Shahriary A, Azimirad M, Alavifard H, Mohsenifar Z, Davari M. et al. The protective effects of live and pasteurized Akkermansia muciniphila and its extracellular vesicles against HFD/CCl4-Induced Liver Injury. Microbiol Spectr. 2021;9(2):e0048421. doi:10.1128/Spectrum.00484-21.
- Schneider KM, Mohs A, Gui W, Galvez EJC, Candels LS, Hoenicke L, Muthukumarasamy U, Holland CH, Elfers C, Kilic K. et al. Imbalanced gut microbiota fuels hepatocellular carcinoma development by shaping the hepatic inflammatory microenvironment. Nat Commun. 2022;13(1):3964. doi:10.1038/s41467-022-31312-5.
- Wang B, Chen X, Chen Z, Xiao H, Dong J, Li Y, Zeng X, Liu J, Wan G, Fan S. et al. Stable colonization of Akkermansia muciniphila educates host intestinal microecology and immunity to battle against inflammatory intestinal diseases. Experimental & Molecular Medicine. 2023;55(1):55–68. doi:10.1038/s12276-022-00911-z.
- Ansaldo E, Slayden LC, Ching KL, Koch MA, Wolf NK, Plichta DR, Brown EM, Graham DB, Xavier RJ, Moon JJ. et al. Akkermansia muciniphila induces intestinal adaptive immune responses during homeostasis. Science. 2019;364(6446):1179–1184. doi:10.1126/science.aaw7479.
- Bae M, Cassilly CD, Liu X, Park SM, Tusi BK, Chen X, Kwon J, Filipčík P, Bolze AS, Liu Z. et al. Akkermansia muciniphila phospholipid induces homeostatic immune responses. Nature. 2022;608(7921):168–173. doi:10.1038/s41586-022-04985-7.
- Ierna MX, Scales HE, Mueller C, Lawrence CE. Transmembrane tumor necrosis factor alpha is required for enteropathy and is sufficient to promote parasite expulsion in gastrointestinal helminth infection. Infect Immun. 2009;77(9):3879–3885. doi:10.1128/IAI.01461-08.
- Kim S, Shin YC, Kim TY, Kim Y, Lee YS, Lee SH, Kim M-N, O E, Kim KS, Kweon M-N. et al. Mucin degrader Akkermansia muciniphila accelerates intestinal stem cell-mediated epithelial development. Gut Microbes. 2021;13(1):1–20. doi:10.1080/19490976.2021.1892441.
- van der Lugt B, van Beek AA, Aalvink S, Meijer B, Sovran B, Vermeij WP, Brandt RMC, de Vos WM, Savelkoul HFJ, Steegenga WT. et al. Akkermansia muciniphila ameliorates the age-related decline in colonic mucus thickness and attenuates immune activation in accelerated aging Ercc1−/Δ7 mice. Immun Ageing. 2019;16(1):6. doi:10.1186/s12979-019-0145-z.
- Yu J, Liu T, Guo Q, Wang Z, Chen Y, Dong Y, Fu Y. Disruption of the intestinal mucosal barrier induced by high fructose and restraint stress is regulated by the intestinal microbiota and microbiota metabolites. Microbiol Spectr. 2023;11(2):e0469822. doi:10.1128/spectrum.04698-22.
- Martin-Gallausiaux C, Garcia-Weber D, Lashermes A, Larraufie P, Marinelli L, Teixeira V, Rolland A, Béguet-Crespel F, Brochard V, Quatremare T. et al. Akkermansia muciniphila upregulates genes involved in maintaining the intestinal barrier function via ADP-heptose-dependent activation of the ALPK1/TIFA pathway. Gut Microbes. 2022;14(1):2110639. doi:10.1080/19490976.2022.2110639.
- Yoshihara T, Oikawa Y, Kato T, Kessoku T, Kobayashi T, Kato S, Misawa N, Ashikari K, Fuyuki A, Ohkubo H. et al. The protective effect of Bifidobacterium bifidum G9-1 against mucus degradation by Akkermansia muciniphila following small intestine injury caused by a proton pump inhibitor and aspirin. Gut Microbes. 2020;11(5):1385–1404. doi:10.1080/19490976.2020.1758290.
- Desai MS, Seekatz AM, Koropatkin NM, Kamada N, Hickey CA, Wolter M, Pudlo NA, Kitamoto S, Terrapon N, Muller A. et al. A dietary fiber-deprived gut microbiota degrades the colonic mucus barrier and enhances pathogen susceptibility. Cell. 2016;167(5):1339–53 e21. doi:10.1016/j.cell.2016.10.043.