ABSTRACT
Aim
To compare differences in the disinfection efficacy of calcium hydroxide (CH) and chlorhexidine gluconate (CHD) dressings in pulp revitalization (PR) of traumatized immature necrotic teeth; to investigate the microflora in successful/failed PR and whether bacterial persistence influences the outcomes of PR.
Methods
Microbiological assessment of the average bacterial load (CFU/sample) and bacterial diversity (taxa/sample) was performed on 41 teeth at three timepoints (S2-before, S3-after debridement and S5- after root canal dressing).
Results
The primary microflora was more diverse in successful cases than in failed. Decreases in CFU/sample and taxa/sample occurred S2 - S3, though new increases occurred at S5 in the CHD subgroup (successful and failed) and CFU/sample in the CH subgroup (failed). At S5, the successful cases showed more bacterial decreases. No specific species was associated with the outcomes with no statistical differences between the disinfection efficacy.
Conclusions
There were no statistical differences in CH and CHD efficacy. At S5, microflora persisted in both successful and failed outcomes, but the abundance and diversity increased significantly only in the failed cases. The successful outcomes presented higher diversity and higher decreases of the primary microflora at S5 than the failed outcomes. The abundance and diversity increased significantly at S5 only in failed cases.
Introduction
Despite the raised awareness of the predisposing factors and efforts to reduce traumatic dental injuries (TDI), the global incidence of TDI has not decreased [Citation1]. Studies indicate that TDI tend to peak at young age when the root development of the permanent dentition is still incomplete [Citation2,Citation3]. For all types of TDI, severe injuries to the periodontal ligament, such as avulsion or intrusion, exhibit the highest risk for pulp necrosis [Citation4]. If bacteria invade the internal aspects of the tooth, intra-canal infection might occur. In this situation, root canal procedures must be performed to combat the infection and prevent new infections [Citation5].
Endodontic management of immature necrotic teeth with root canal infection is a difficult task due to their anatomy, which is characterized by wide root canals and open apical foramens [Citation6]. It is not only difficult to manage the technical quality but also to create preconditions for efficient antibacterial treatment. For example, effectively disinfecting immature teeth is difficult because the larger size and depth of the dentinal tubules are subject to bacterial invasion [Citation7,Citation8]. To avoid additional weakening of the tooth, the contemporary recommendations for the treatment of immature necrotic teeth include minimal mechanical instrumentation with hand files [Citation9]. On the other hand, this tactic may create opportunities for persisting root canal infection and treatment failure [Citation10–12]. Studies have shown that intra-radicular microorganisms are organized in biofilms situated both on the dentin walls and at the apical region of the root canals [Citation13]. Moreover, evidence suggests that bacterial species surviving root canal disinfection might hinder local stem cells from facilitating further root maturation, leaving the tooth fragile [Citation14].
The currently acknowledged treatment options for traumatized necrotic immature teeth are apexification with calcium hydroxide or with the MTA-apical plug technique [Citation15]. Notably, endodontic pulp revitalization (PR) has become more frequently used to stimulate continued root maturation through regeneration of the periapical tissues [Citation16–18]. As with apexification treatment in infected teeth, PR requires removing necrotic tissue and disinfecting the intra-radicular space [Citation13,Citation19].
For the treatment of endodontic infections in PR, several intra-canal dressings have been recommended [Citation20]. Calcium hydroxide is still the gold standard with good antibacterial effectiveness on trauma-related root canal infections [Citation21–23]. Calcium hydroxide as an intra-canal disinfectant in mature necrotic teeth is well described in the literature [Citation24–26]. However, in immature necrotic teeth, scientific evidence is available mostly in the context of its ability to produce a matrix of mineralized apical hard tissue [Citation27,Citation28]. The same applies for chlorhexidine gluconate as studies show this treatment provides good antibacterial effectiveness in mature necrotic teeth [Citation29]. However, most of these studies focus particularly on the clinical and radiographical outcomes of calcium hydroxide and chlorhexidine gluconate use in PR of immature necrotic teeth [Citation30].
Effort has been made to assess bacterial reduction during different stages of endodontic revitalization of immature necrotic teeth. Although scarce, studies have evaluated the microbial composition of immature traumatized teeth after intracanal medication with triple antibiotic paste and calcium hydroxide combined with chlorhexidine gluconate [Citation31]. Surprisingly, it was found that the greatest bacterial reduction was achieved when using irrigation solutions. However, no significant differences were found between the intra-canal dressings. This finding was recently confirmed by other researchers who targeted the 16S ribosomal RNA gene using quantitative PCR (qPRC) and next-generation sequencing (NGS) for bacterial DNA detection. The authors found that enhanced antimicrobial protocols with higher concentrations of antimicrobial agents reduced endodontic microflora more effectively [Citation32].
Nevertheless, few studies investigate antimicrobial outcomes of intra-canal dressings with calcium hydroxide and chlorhexidine gluconate in PR. In particular, studies are needed that investigate the composition of the primary microbiota of these teeth and the persisting bacterial species in failed pulp revitalization cases.
Thus, this RCT has three aims:
- To compare differences in the disinfection efficacy after root canal dressings with calcium hydroxide and chlorhexidine gluconate.
- To investigate the microbial flora of immature traumatized necrotic teeth in successful and failed PR cases.
- To evaluate whether bacterial persistence influences the clinical and radiographical outcomes of PR.
Materials and methods
Study population and selection of participants
This study was designed as a randomized clinical trial and was approved by the Stockholm Central Ethical Board, Swedish Ethical Review Authority (Dnr: 2018/692–31; 2022–05715–02). The study was conducted according to ICMJE recommendations for best practice and ethical standards in the conduct and reporting of research [Citation33] and according to the ethical principles of the Declaration of Helsinki [Citation34]. The patient data were coded and stored according to principles of the general data protection regulation. Both spoken and written information were provided to the participants, and the parents of the participants signed informed consent forms before collection of the bacterial samples from the infected root canals.
The participants were referred to the Department of Endodontology at Eastman Institute, Public Dental Services in Stockholm, Sweden. The treatment was performed by one of the authors (AW, senior consultant in endodontics), who has experience treating traumatized immature teeth in young patients.
The study population was comprised of children with one or more traumatized permanent incisors with immature root development with pulp necrosis and/or apical periodontitis. When apical periodontitis was not present radiographically, in addition to a negative response to cold and electric sensibility tests, teeth were included only when clinical signs and symptoms of pulp necrosis were observed (swelling, sinus tract, self-reported pain, and pain to percussion and palpation).
To achieve balance in the allocation of the participants to the two treatment arms according to the intra-canal dressing material, a block randomization protocol was applied. The subjects were assigned to one of the two treatment groups according to a randomization list consisting of blocks of size 4.
The first group received intra-canal dressing with calcium hydroxide paste (CH) (Calasept®, Directa AB, Upplands Väsby, Sweden) and the second group received intra-canal dressing with 2% chlorhexidine digluconate gel (CXD) (Gluco-CHeX 2% gel, PPH Cerkamed, Stalowa Wola, Poland). If a patient was referred for treatment of more than one tooth, those teeth were also allocated for randomization of the dressing material, provided that the inclusion criteria were met. The participants were assigned to the intervention by a person (dental assistant) not involved in the trial management group. After enrolment to the study, the operator, the participants, and their parents were informed which treatment arm was going to be performed. The treatment was performed by one of the authors (AW). Operator blinding was not possible due to differences in how the two dressing materials appeared during the clinical procedure. The results were described in accordance with the CONSORT statement (https://www.consort-statement.org) [Citation35].
A total of 53 incisors in 49 children were included in the study and considered eligible for microbiological analyses. The overall observation period for the treated teeth ranged from 1.19 years to 7.69 years (mean 3.98 years). The inclusion and exclusion criteria are presented in .
Table 1. Inclusion and exclusion criteria for the selection of patients.
Clinical procedures and definition of success and failure of pulp revitalization procedures
Clinical procedures and outcome measures are described in detail in our previous study [Citation35]. We verified pulp necrosis by cold pulp sensibility test (Endo Ice; Roeko, 71 Langenau, Germany; Endo Frost, Coltene Whaledent Roeko, Germany), an electric pulp test (Pulp Tester; Analytic Technology, Redmond, WA), and intraoral radiographs. All treatments were performed with the aid of a dental operating microscope (Leica TM, M320).
The clinical variables were registered at baseline and during the last follow-up (mean survival 46 months after treatment). The clinical variables were self-reported pain, pain to percussion and palpation, pathological tooth mobility, pathological periodontal probing, swelling, sinus tract, and replacement resorption. The radiographical variables were apex closure, root resorption, periapical index (PAI), root development stage, and continued root formation in width and length.
Outcome measures
The primary outcome measure was changes in the counts of colony forming units (CFU/sample) and changes in the number of taxa per sample (taxa/sample) after root canal debridement (S3) and after root canal dressing (S5).
The secondary outcome measure was success or failure. Success was defined by absence of clinical symptoms (no subjective pain, sinus tract, swelling, or sensitivity on palpation or percussion) and radiographical signs of healing of apical periodontitis as well as evidence of continued root development. Failure was defined by the presence of clinical symptoms after the completion of PR, such as subjective pain, sinus tract, swelling, or sensitivity on palpation or percussion. Radiographical signs defined as a failure were no apex closure, persisting apical periodontitis, persisting or progressing resorptions, or the absence of continued root formation in length and width, which were all assessed at the final follow-up.
Clinical and microbiological sampling procedures at the first visit
After conventional application of local anaesthesia, tooth isolation was achieved using a rubber dam and retainer, and an access preparation was initiated only through the enamel using a high-speed burr. Surface disinfection of the tooth crown, retainer, and rubber dam was performed using a sterile foam pellet soaked in H2O2 (30%) and a pellet soaked in chlorhexidine-ethanol solution (5%) followed by a foam pellet soaked in 5% sodium thiosulfate solution.
To assess the sterility of the operation field, bacterial samples of the disinfected tooth crown were taken using two foam pellets (contamination control sample S1) as previously described [Citation36].
One foam pellet was transferred to a tube with Tris-EDTA buffer (10 mmol/L Tris hydrochloride, 1 mmol/L EDTA, TE Sigma) for further DNA analysis. The other pellet was transferred to a tube containing 5 mL of fluid thioglycolate medium supplemented with agar.
Next, the pulp chamber was accessed through the dentine with a sterile burr. A bacterial sample (S2) was collected from the root canal before any instrumentation and irrigation. Sterile saline was applied into the root canal and allowed to fill the root canal to the level of the cementum-enamel junction leaving the pulp chamber dry. Dentin shavings were produced using a sterile hand file (K 20), which was struck towards the root canal wall in order to reach adherent biofilm microbiota. The fluid was absorbed with three sterile paper points that were held in the root canal for 1 min and then immediately transferred to a tube with reduced transport fluid (anaerobic sterilized VMG III, medium, Dept of Oral Microbiology, Umeå).
Then, the root canal was irrigated with 20 mL 0.5% of buffered sodium hypochlorite (Dakin’s solution, APL, Apoteket AB, Stockholm, Sweden). Further irrigation with 5 mL of ethylenediaminetetraacetic acid was applied (EDTA, 15.3% APL, Apoteket AB, Stockholm, Sweden) followed by irrigation with 2 mL of sterile saline solution. After completed debridement, the root canal irrigants were inactivated with 1 mL of sodium thiosulfate for 1 min [Citation37].
The inactivating solution was dried with sterile paper points and irrigated with 2 mL of sterile saline solution, which was left in the root canal during the sampling procedure. Next, a sterile hand file (K 20) was struck towards the root canal wall to produce dentinal shavings, and a bacterial sample (S3) was performed according to the technique described above and transferred to VMG III. Finally, the root canal was dried with sterile paper points, and dressing material was applied with a sterile syringe and distributed with a spiral Lentulo filler. The root canal orifice and the access preparation were temporized with a minimum of 4 mm of temporary filling consisting of zinc oxide eugenol cement (Labservice AB, Sundsvall, Sweden) and glass ionomer (GC Fuji TM IX GP Fast, GC Corporation, Tokyo, Japan). A second appointment was scheduled for approximately 4 weeks after this procedure was performed.
Clinical and microbiological sampling procedures at the second visit
Any clinical symptoms of persisting infection were evaluated at the second appointment. If clinical symptoms were present, the chemo-mechanical disinfection protocol was repeated according to the technique described above. Provided the tooth was asymptomatic and no clinical signs of infection were detected, the revitalization procedure was initiated. Local infiltration anaesthesia was applied without vasoconstrictors (1.8-mL Carbocaine Dental, 30 mg/mL, Dentsply Pharmaceuticals DeTrey GmbH, Surrey, UK). The temporary filling with glass ionomer was removed and a rubber dam was applied and disinfected with the same technique used at the first treatment visit.
A contamination control sample (S4) was taken using the same procedure as S1. The residual temporary material was removed using a sterile burr and the root canal dressing was removed with application of 1 mL of sodium thiosulfate for 1 min; additional irrigation was performed with 2 mL of sterile saline (Natriumklorid Microspol 9 mg/mL, APL, Apoteket AB, Stockholm, Sweden). Next, a sterile hand file (K 20) was struck towards the root canal wall to produce dentinal shavings, and a bacterial sample (S5) was collected from the root canal using the same technique as S3. The root canal was irrigated with 5 mL of 0.5% buffered sodium hypochlorite (Dakin’s solution, APL, Apoteket AB, Stockholm, Sweden) and 15 mL of EDTA for 5 min (15.3% APL, Apoteket AB, Stockholm, Sweden). The final irrigation consisted of 2 mL of sterile saline and the root canal was dried with sterile paper points.
Bleeding from the periapical tissue was induced with a pre-curved file (Kerr 15 or Hedström 30) until the root canal was filled with blood to the level of the cemento-enamel junction. The bleeding was monitored for approximately 15 min to secure blood clot stability. A resorbable collagen scaffold was placed over the blood clot (PARASORB® Cone, Resorba Medical GmbH, Nürnberg, Germany) and a minimum of 2 mm of bioceramic material was placed at the level of cemento-enamel junction. The bioceramic materials were white MTA Pro Root, Dentsply, Maillefer, Switzerland, or Biodentine (Septodont®, Saint-Maur-des-Fossés, France), or Bioceramic Root Repair material condensable putty (EndoSequence® BC RRM™, Brasseler, USA). A glass ionomer was placed over the bioceramic material (GC Fuji TM IX GP Fast, GC Corporation, Tokyo, Japan), and restoration with resin restorative material was made during the same visit. presents a flowchart of the treatment protocol and the time points for the microbiological samplings.
Characterization of isolates and 16S rDNA sequencing
The microbiological analyses were performed at the Laboratory for Oral Microbiology, Umeå University, Sweden between May 2018 and November 2021. The samples were immediately analysed after delivery to the laboratory (24–36 h) as previously described [Citation36].
Briefly, the paper points were transferred to 1-mL TE-buffer, vortexed for 1 min, and then the ten-fold serial dilutions (10–104) were prepared and cultured on Fastidious Anaerobe Agar (FAA) in anaerobic conditions (37°C; 10% H2, 5% CO2, 85% N2) for 1 week. Colony-forming units (CFU) were estimated under a stereomicroscope (SMZ800, Nikon, Japan) using up to x50 magnification. In addition, the number of colonies with different phenotypical patterns were carefully registered from each plate.
Isolates with different phenotypical patterns were selected from each plate and were identified by comparing 16S rRNA gene sequences to databases as previously described [Citation37]. The 16S rRNA gene was PCR-amplified using 16S-LF (AGAGTTTGATCCTGGCTCAG) and 16S-R (GGGCGGTGTGTACAAGGC) primers.
PCR amplifications were prepared in a final reaction volume of 50 µL: 5 µL of total DNA template (bacteria suspended in MilliQ water as described above); 10 µM of each primer; 25 µL of 2× KAPA2G Robust HotStart ReadyMix (KAPA2G Robust HotStart ReadyMix, Merck, USA); and nuclease-free water. An initial denaturation at 95°C (5 min) was followed by 36 cycles of denaturation at 95°C (15 s), annealing at 60°C (20 s), extension at 72°C (45 s), and a final extension at 72°C (90 s). Presence of the PCR product in the PCR reaction was tested by the visualisation of the band of approximately 1420 bp with the gel electrophoresis in a 1% agarose gel. If the PCR reaction resulted in too few PCR products, the PCR was performed as described above with the DNA extracted from the corresponding bacterial isolates using a bacterial Genomic DNA kit (Gene Elute, Sigma, Saint Louis, Missouri, USA) according to the manufacturer’s protocols. Briefly, one bacterial colony was resuspended in nuclease-free water and centrifuged (13000 rpm, 5 min). The pellet was thoroughly resuspended in 200 μL of Lysis Solution containing 45 mg/mL of Lysozyme (Merck, Sweden) and 250 U/mL Mutanolysin (Merck, Sweden) and incubated at 37°C for 30 min. RNase A (20 μL) was added for 2 min of incubation at room temperature, followed by treatment with Proteinase K (20 μL) and Lysis Solution C (200 μL). Ethanol (99.5%) was added after 10 min of incubation at 55°C (10 min). Next, the contents of the tubes were placed on the column to separate the DNA. The column was washed twice and then 50 µL of the elution solution was applied directly onto the centre of the column and incubated for 5 min at room temperature before the DNA was eluted by centrifugation. The PCR products were sent to Eurofins Genomics (https://www.eurofinsgenomics.eu/) for purification and consecutive Sanger sequencing in sense and antisense direction using the aforementioned 16S-LF and 16S-R primers, respectively.
Only the overlapping pair-end reads were used for analysis, and reads with less than 350 nucleotides in length were not considered. Strains were identified by comparing the assembled sequences with Basic Local Alignment Search Tool (BLAST) to the Human Oral Microbiome Database (HOMD; www.homd.org) or to the databases in GenBank at the National Center for Biotechnology Information (NCBI). The threshold for species identification was 98.5%.
Sample size calculation
A minimum potential sample of 56 teeth was shown to have statistical significance (p value of < .05 and considered significant at a statistical power of 95%) with a margin of error 0.08 (population proportion 0.1046, population size 9,307,000, Z = 1.960).
Statistical analysis
Statistical analysis was performed using the commercially available software program IBM SPSS (version 26, IBM Corporations, New York, USA) and the GraphPad Prism 7.0 (GraphPad Software Inc. San Diego, USA) software package. A p-value of <0.05 was considered significant for all tests at a statistical level of 95%. Adjusted to the data properties, the Kruskal–Wallis test, the Mann-Whitney U, and the Z-test for comparison of proportions were performed using GraphPad Prism version 9.0 test to assess the differences between the means for CFU/mL of the calcium hydroxide and the chlorhexidine gluconate groups. Calculations of the effect sizes were performed using Cohen’s d [Citation38,Citation39]. The unpaired student's´ t-test and chi-square tests were used for analyses of the subgroups of failed and successful cases and between the different sampling timepoints (S2; S3; S5).
Calculation of the disinfection efficacy between the two root canal dressings was performed by a method previously described by Sadeghi [Citation40]. The function of logarithm for calculation of the CFU per sample and the limit of detection (LOD) for the both root canal dressings used in the study (CHD and CH) were described in the results [Citation41].
Results
Patients’ demographics and pre-operative characteristics were described in our previous study [Citation42]. Briefly, most patients (80%) were between 6 and 11 years old, 64% of whom were male. The mean age distribution was 10.4 years (range 6–22) for successful PR, and 9.5 years (range 7–11) for unsuccessful PR. Over half (58.7%) of the included teeth were subject to trauma to both hard tissues (enamel and dentine fractures) and soft tissues (involving the periodontal ligament, such as lateral luxations, concussions, subluxations, extrusive luxations, intrusions, and avulsions). The most frequent type of hard tissue trauma was enamel-dentin fracture (73%), and the most frequent type of soft tissue. Trauma was lateral luxation (39%). Severe trauma, such as avulsion and intrusion, was present in 23% of the cases.
Initially, 53 participants were included in the RCT. Before microbiological analyses, the calcium hydroxide subgroup consisted of 29 teeth and the chlorhexidine subgroup of 24 teeth. To control sterility, we cultured samples from tooth surfaces and monitored growth for 7 days after incubation. Teeth showing bacterial growth at contamination control samples S1 and S4 (n = 5) were excluded from further microbiological analyses. In addition, seven teeth were excluded due to logistical issues. As a result, 123 samples from the root canals of 41 participants were considered for further microbiological analyses (). In 68% (n = 28) of the PR cases, we observed successful outcomes with no clinical symptoms or radiographic signs of apical periodontitis as well as evidence of continued root development (in length and width and apex closure). In 32% (n = 13) of the PR cases, we observed failed clinical outcomes with some of the cases presenting with more than one sign of failure: post-operative pain (n = 5), sinus tract (n = 2), presence of periodontal disease with periodontal probings >6 mm (n = 3), abscess (n = 3), and percussion pain (n = 8). Presence of radiographical signs of persisting apical periodontitis and absence of radiographical signs of continued root development was observed in 12 participants. In the calcium hydroxide subgroup, 29 cases were eligible for the final analysis, where endodontic pulp revitalization treatment was successful in 20 cases and failed in 9 cases. In the chlorhexidine gluconate subgroup, 12 cases were eligible for the final analysis, where 8 cases were successful, and 4 cases failed ().
Figure 2. Flow chart of data collection of eligible root canal samples.
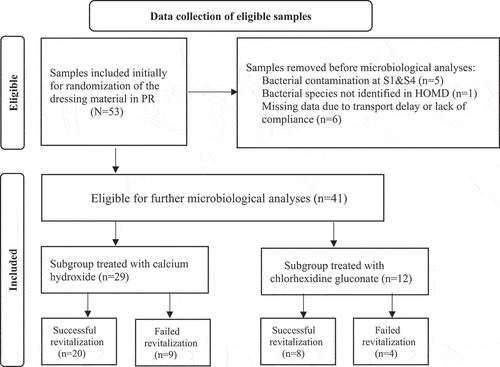
Microbiological analyses at the different stages of the revitalization procedure
The microbiological analyses performed at each sampling time point (S2, S3, and S5) included assessment of the average bacterial abundance (bacterial load per sample, CFU/sample) and analyses of the average bacterial diversity per sample (the number of taxa/sample). Viable bacteria were detected in 80.5% of all traumatized teeth before root canal debridement (S2). Cultivable bacteria were detected in 17.1% of the samples collected after root canal debridement (S3), and 19.5% were detected after root canal dressing (S5).
In the chlorhexidine subgroup, the counts of colony-forming units (CFU/sample) before instrumentation (S2) did not differ statistically significantly in both the successful and the failed PR group (93828.8 ± 187284.1 and 66532.5 ± 74956.2 CFU/sample, respectively). After root canal debridement (S3), both groups showed decreases in CFU; more decrease occurred in the successful PR group (5.0 ± 13.2 CFU/sample) than the failed PR group (12.5 ± 16.4 CFU/sample). Nevertheless, no statistical differences in CFU/sample could be shown between the two groups (i.e. successful and failed outcomes) (p = 0.9354ns at S2 and p = 0.4909ns at S3 by the Mann–Whitney U-test).
After root canal dressing with chlorhexidine gluconate (S5), both groups showed increases in the counts of CFU, but the CFU counts were higher in the failed PR group: 107 ± 225.2 CFU/sample for the successful PR group and 2682.5 ± 4412.7 CFU/sample for the failed PR group. However, no statistically significant differences were shown between the successful and the failed groups (the Mann-Whitney U-test, p = 0.3212ns).
In the calcium hydroxide subgroup, the counts of CFU per sample before root canal debridement (S2) were higher in the successful PR group (651377.5 ± 1542127.6 CFU/sample) than the failed PR group (86788.9 ± 124348.2 CFU/sample). Here, the successful PR group showed more significant decrease in CFU counts after root canal debridement (S3) (2.5 ± 8.9 CFU/sample) than the failed PR group (280 ± 788.4 CFU/sample) and after application of the intracanal dressing with CH (S5) (0.5 ± 2.2 CFU/sample) compared to the failed PR group (5463.3 ± 15265.8 CFU/sample).
Moreover, at S5, only samples from the failed PR group had the CFU/sample values increased (5463.3 ± 15265.8) compared to the corresponding samples at S3 (280 ± 788.4). On the other side, the counts of CFU/sample in successful PR outcome decreased (0.5 ± 2.2) compared with the corresponding samples from S3 (2.5 ± 8.9). The difference between the successful and the failed groups at S5 was statistically significant (p = 0.023) (, Supplementary Tables S1 and S2(a,c)).
Figure 3. Average number of CFU per sample in the successful pulp revitalization cases(n = 29) vs the failed PR cases (n = 12) at each sampling time point (S2, S3, and S5).
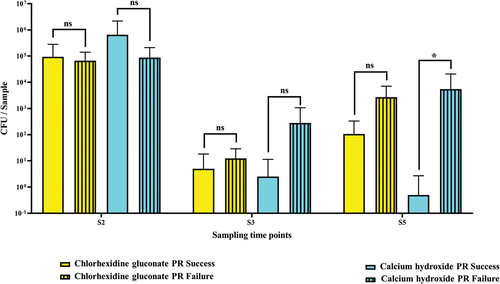
In addition to total bacterial load per sample, the average bacterial diversity per sample (the number of taxa/sample) was estimated. For the chlorhexidine gluconate treated subgroup, the number of taxa per sample before root canal debridement (S2) was higher (2.0 ± 1.5 taxa/sample) in the successful PR cases than in the failed PR cases (3.75 ± 2.5 taxa/sample). Here, a similar trend of decreases at S3 was observed: more decrease in the successful cases (0.1 ± 0.3 taxa) than the failed cases (0.5 ± 0.5) (p > 0.05ns). The same trend of new increases was also observed at S5: the values increased in comparison with the corresponding samples at S3. At this sampling time point (S5), the average number of taxa was higher in the failed PR cases treated with chlorhexidine gluconate (0.5 ± 1.0 taxa/sample in successful and 1.75 ± 2.0 taxa/sample in failed) (p > 0.05 ns) (Mann-Whitney U test).
For the calcium hydroxide treated subgroup, the average number of taxa per sample before root canal debridement (S2) in the successful PR cases was higher (4.7 ± 2.0 taxa/sample) than in the failed PR cases (3.44 ± 3.1 taxa/sample). Both the successful and the failed cases showed decreases in the average number of taxa per sample at S3 (successful cases: 0.2 ± 0.5; failed cases: 0.6 ± 1.3; p > 0.05ns).
At S5, the values increased in comparison with the corresponding samples at S3. The average number of taxa per sample was higher in the failed PR cases: 0.6 ± 0.8 taxa compared to 0.1 ± 0.2 taxa per sample in the successful cases at statistically significant level (p = 0.02**) (Mann-Whitney U test) (, Supplementary Table S1, Supplementary Table S2B and S2D).
Figure 4. Average number of taxa per sample in the successful pulp revitalization cases(n = 29) versus the failed pulp revitalization cases (n = 12) at each sampling time point (S2, S3, and S5).
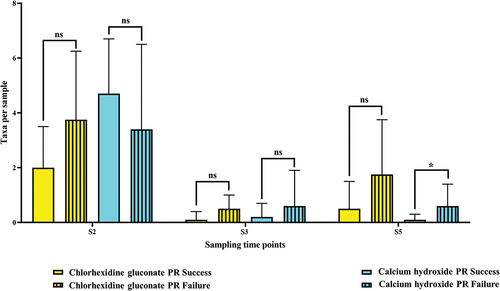
Since statistically significant difference between the failed and the successful outcomes was found only at the S5 sampling point in the CH treated subgroup, for the CFU/sample as well as for the number of the taxa/sample, effect size analysis was performed to calculate the differences between the successful and failed outcomes in both subgroups at different sampling time points [Citation43]. The results showed that the Eta squared effect size appeared at a level higher than 0.6 (i.e. interpreted as intermediate effect) for all variants of comparison in the CH subgroup. The effect size in all compared cases was very similar to the compared pairs of cases with statistically significant differences: for the taxa per sample in the calcium hydroxide subgroup at S5 Eta squared (η2) was 0.62 and Cohen’s d was 2.555; for the CFU per sample, Eta squared (η2) was 0.62 and d Cohen’s d** was 2.557 (Supplementary Table S3).
Disinfection efficacy after intracanal medication with calcium hydroxide and chlorhexidine gluconate
Analysis of the disinfection efficacy of CHD and CH was performed using a logarithm function comparing bacterial log-reductions after root canal disinfection. For this purpose, the limit of detection (LOD) – i.e. the lowest count of colonies detected on the plates – was calculated for each bacterial sample with a cut-off value <10 CFU/sample.
The allocation to the two treatment groups exhibited normal distribution and no skewness with respect to randomization to dressing material. The analysis of the disinfection efficacy of CHD and CH did not show significant differences. The failed pulp revitalization outcomes in the CH-treated subgroup was 31%, and the failed pulp revitalization outcomes in the CHD-treated subgroup was 33%. The successful pulp revitalization outcomes were 69% and 67% for the CH-treated and the CHD-treated subgroups, respectively.
Indication for lower mean value of the disinfection efficacy in the failed outcomes in comparison with the successful outcomes was found in both subgroups, but statistical significance between successful versus failed was not reached (p = 0.13841ns) according to Mann–Whitney U-test ().
Figure 5. Disinfection efficacy in the successful vs. failed pulp revitalization cases, calcium hydroxide and chlorhexidine subgroups.
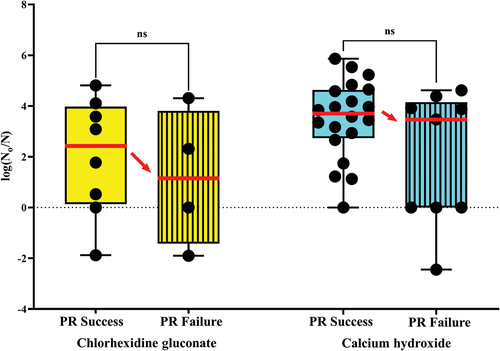
The primary microflora of immature traumatized necrotic teeth associated with the outcome of pulp revitalization
Among all isolates collected from root canals before root canal debridement (S2), 61 bacterial species (represented by 156 strains) were identified by comparing the 16S rRNA gene sequence to the HOMD database. The most prevalent isolated species detected in all samples in S2 were Peptostreptococcaceae [Eubacterium] yurii (8.9%), Fusobacterium naviforme/nucleatum (6.4%), Capnocytophata sp. (5.8%), Granulicatella adiacens (5.1%), Eikenella corrodens/Kingella denitrificans (4.5%), Streptococcus sanguinis (3.8%), Slackia exigua (3.2%), and Actinomyces sp. (2.6%) (Supplementary Table S4). The highest polymicrobial composition of species was detected in the successful cases, where 34 different species were identified. Among the most abundant species were Streptococci species (S. intermedius, S. oralis, S. salivaris, S. sanguinis, and S. infantis), Porphyromonas species (P. catoniae, P. uenoni, and P. pasteri), and Prevotella species (P. denticola, P. nigrencens, and P. oralis). Twenty-one species were common for both the successful and failed PR cases. The bacterial isolates retrieved solely from the failed PR cases showed less phenotypic diversity. Here, only nine isolates were unique for the failed PR outcomes from both the CH and CHD subgroups. Among those, Peptostreptococcus and Streptococcus species were the most prevalent ().
Figure 6. Primary bacterial taxa from all samples before root canal debridement (S2) cultured in both outcomes of successful and failed pulp revitalization (n = 41).
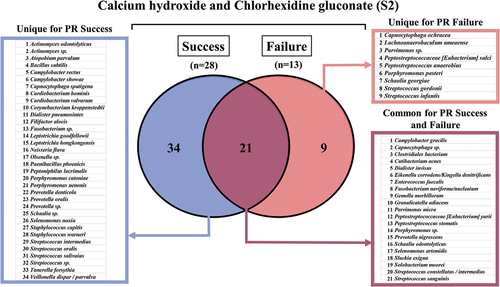
In the calcium hydroxide subgroup, 57 bacterial species were identified: 36 bacterial species for the successful cases; 14 bacterial species for both outcomes; and seven bacterial species for the failed cases (Supplementary Figure S2). In the chlorhexidine gluconate subgroup, 25 bacterial species were identified: 12 bacterial species for the successful cases; 1 bacterial species for both outcomes; and 12 bacterial species for the failed cases (Supplementary Figure S3).
All taxa isolated from both CHD and CH subgroups were compared at the phylum level, representing a higher hierarchical rank of bacterial taxonomy. The sequences of 16S rRNA genes on the phylum level with 75% similarity were distributed into six matching phyla in the CH subgroup and five matching phyla in the CHD subgroup [Citation44]. Five phyla were common for both CHD and CH subgroups, whereas one phylum – Campylobacterota – was only found in the CH subgroup.
The abundance of representatives in a phylum was calculated as the percentage of strains for a given microbial phylum in each subgroup depending on RP outcome. The data were analysed using the Z-test for comparing proportions and did not reveal any statistically significant differences (p > 0.05) between the proportion of taxa belonging to the corresponding phylum in the successful versus the failed RP outcomes in the CH-treatment as well as the CHD-treatment subgroups.
A common tendency (but not at statistically significant level) was shown for both subgroups (CHD and CH) regarding the relative fraction of Bacteroidota phylum, which decreased in the failed outcomes in comparison with the successful outcomes. However, the fraction of Bacillota phylum increased in the failed outcomes in comparison with the successful outcomes. The correlation of the bacterial species/taxa/phyla was compared with the pulp revitalization outcome. The correlation of bacterial load on the phylum level with the pulp revitalization outcome was analysed. The bacterial load of each cultivated taxa in phylum was expressed as a percentage and all fractions in phylum were summarized and corresponding phylum for both outcomes were compared by chi-square test and Fisher’s exact test ().
Figure 7. Phylum diversity between successful and failed pulp revitalization outcomes in relation to the substance used for the root canal disinfection.
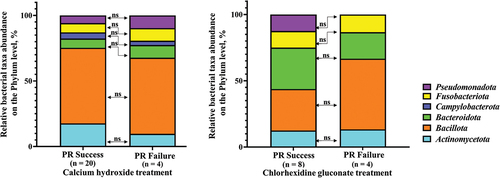
The data analysed for the CH subgroup showed that the bacterial load values were lower in the successful outcomes. Statistically significant differences were found for the phyla Bacteroidota (p<0.0001****) and Actinomycetota (p<0.0001****). No statistical significance was found in the phyla Fusobacteriota (p = 0.2392ns), Pseudomonadota (p = 0.2049ns), and Campylobacterota (p = 0.9999ns).
Only one phylum, Bacillota, had higher numbers of bacterial load in the successful outcomes at a statistically significant level (p<0.0001****) in the CH subgroup ().
Figure 8. Bacterial load on the phyla associated with both outcomes of pulp revitalization in the calcium hydroxide subgroup.
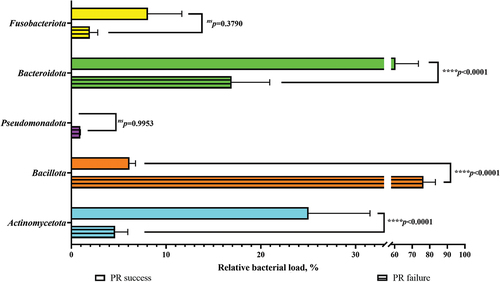
The bacterial load of taxa cultivated from the CHD subgroup (n = 12) was compared in regard to outcome – success versus failure in matching phyla – with the same multiple-comparison method as described above (). The isolates could be matched to the same five phyla as the isolates from the CH subgroup except for the phylum Campylobacterota. Here, the numbers of taxa were higher in the successful pulp revitalization outcomes in the phyla Fusobacteriota (p = 0.3790ns), Bacteroidota (p < 0.0001****), Pseudomonadota (p = 0.9953ns), and Actinomycetota (p<0.0001****).
Figure 9. Bacterial load on the phyla level associated with both outcomes of pulp revitalization in the chlorhexidine subgroup.
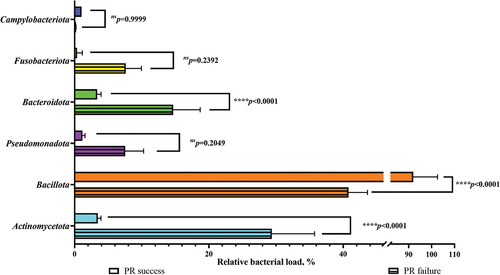
Only the total bacterial load of all cultivated taxa in the phylum Bacillota was lower in the successful outcomes at a statistically significant level (p < 0.0001****) in the CHD subgroup.
Characteristics of the bacterial taxa persisting after root canal debridement and after intra-canal dressings
After root canal debridement (S3), 10 bacterial species (11 strains) were identified and an average of 0.3 species per sample were recovered from each root canal (Supplementary Table S4). The following species were identified in the CH subgroup (S3): Aggregatibacter sp., Clostridiales bacterium, Cutibacterium acnes, Fusobacterium nucleatum, Staphylococcus epidermidis, Prevotella sp., and Staphylococcus capitis, and Streptococcus intermedius. The following species were identified in the CHD subgroup (S3): Cutibacterium acnes, Enterococcus faecalis, and Paenibacillus glucanolyticus ( and ).
Figure 10. Bacterial taxa cultured in all cases from the calcium hydroxide subgroup (pulp revitalization treatment – success and failure at all time points).
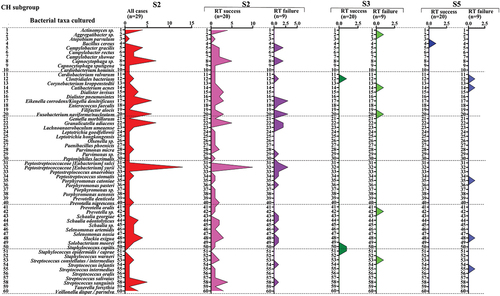
Figure 11. Bacterial taxa cultured in all cases from the chlorhexidine subgroup (pulp revitalization treatment – success and failure at all time points).
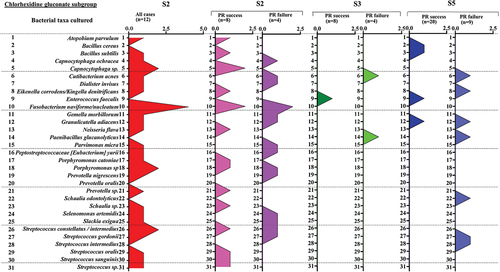
After root canal dressing (S5), 14 persisting bacterial species (17 strains) were recovered and an average of 0.4 species per sample were recovered from each root canal (Supplementary Table S4). The isolates identified in the CH subgroup (S5) were Bacillus cereus and Clostridiales bacterium.
The isolates identified in the CHD subgroup (S5) were Bacillus cereus and B. subtilis, Cutibacterium acnes, Eikenella corrodens, Enterococcus faecalis, Granulicatella adiacens, Paenibacillus glucanolyticus, Schaalia odontolyticus, Streptococcus gordonii, and S. intermedius. The isolates common for both S3 and S5 were Cutibacterium acnes, Enterococcus faecalis, and Paenibacillus glucanolyticus.
Discussion
This randomized clinical study evaluates the antibacterial efficacy of root canal dressings with calcium hydroxide and chlorhexidine gluconate in PR and investigates the primary bacterial composition of traumatized immature permanent teeth with pulp necrosis and apical periodontitis. In addition, we address whether bacterial persistence is correlated to the PR outcomes.
The disinfection efficacy of calcium hydroxide and chlorhexidine gluconate
This study shows that the high bacterial counts in the samples before root canal debridement (S2) decreased after root canal debridement (S3). As expected, the greatest decreases were achieved between S2 and S3 because no antimicrobial disinfection was applied. Comparing both the successful and the failed outcomes in the CH and CHD subgroups, the level of decreases in the CFU/sample and taxa/sample did not show statistically significant differences between S2 and S3. For mature teeth, it has been shown that about 60% of the instrumented root canals were free from cultivable bacteria after one session of instrumentation and irrigation with 0.5% NaOCl [Citation45]. Like other studies, our study shows that the vast majority of the root canals were free from culturable bacteria after CH or CHD treatment (S3). Although decreases were observed in CFU/sample in both the successful and the unsuccessful PR cases between S2 and S3, the successful outcomes had greater decreases in CFU/sample, perhaps because it is more difficult to achieve effective disinfection in immature teeth, which have larger size and depth of the dentinal tubules, without using mechanical instrumentation [Citation7,Citation8]. Thus, it might be difficult to compare disinfection strategies in mature teeth with immature teeth, where chemical disinfection plays a more important role [Citation46].
One month after the application of root canal dressings with calcium hydroxide and chlorhexidine gluconate (S5), 20% of the samples still contained viable bacteria. Moreover, at S5 there was an upward trend of increased counts of CFU/sample and taxa/sample compared to S3. Also, these increases were statistically much higher in the failed outcomes. However, contemporary clinical studies combining molecular-based approaches on the topic of endodontic pulp revitalization have found that the levels of CFU/sample and taxa/sample drastically decreased with the treatment rendered and particularly after root canal dressings [Citation31,Citation32,Citation47]. Although statistical significance could not be tested, to the best of the authors’ knowledge, this is the first study to observe increases in CFU/sample and taxa/sample 1 month after application of root canal dressings among both the successful and failed cases from the CHD subgroup (S5) and in the CFU/sample in the CH subgroup (i.e. failed). In our opinion, this finding could be explained by leakage. Immature teeth traumatized by an impact force not strong enough to cause fracture might cause cracks, the most likely gate for new microbial invasion after endodontic root canal medication [Citation48].
Another possible cause for the increased counts of CFU/sample and taxa/sample after root canal dressing (S5) could be the nature of the revitalization protocol itself. The ESE guidelines recommend minimal or no mechanical instrumentation with hand files to avoid additional weakening of the fragile immature tooth. On the other hand, it has been discussed that the minimal root canal instrumentation of the immature teeth might facilitate regrowth of residual bacterial species causing treatment failure. The presence of untouched bacterial biofilms may significantly alter the root dentin, the apical papilla, and Hertwig’s Epithelial Root Sheath (HERS) [Citation49]. Although the treatment protocol we used is standardized and we applied passive ultrasonic irrigation [Citation50], these efforts might not be enough to eliminate the bacterial biofilm. Furthermore, low concentrations of irrigation solutions have been proposed due to concerns about the potential cytotoxicity of high concentrations of NaOCl solutions on stem cells of the apical papilla [Citation51]: the higher concentrations of NaOCl used, the more cytotoxic effects on survival and differentiation of the stem cells [Citation52,Citation53]. In fact, it has been found that even if bacterial levels are reduced when using 6% NaOCl concentration, a total elimination of the microorganisms might not be guaranteed [Citation47]. In addition, a higher prevalence of post-operative symptoms after irrigation with high concentrations of NaOCl has been reported in adult patients [Citation54]. The same study did not find any statistically significant differences in disinfection efficacy between 0.5% and 3% NaOCl irrigating solutions showing that higher concentrations do not give potentially better antibacterial effect per se.
The irrigation protocol in this study was based on a buffered solution of 0,5% NaOCl (Dakin’s solution) which is a recommendation from the National Board of Health and Welfare established praxis in Swedish dental practice [Citation54]. Due to its high pH, this solution has good antimicrobial and biofilm efficiency [Citation55]. Precaution was taken regarding the risk of postoperative symptoms associated with the concentration of NaOCl, given that this study was performed on school children with immature teeth. Low incidence of postoperative symptoms, due to a low tissue-irritating potential, was reported for patients when concentrations of 0,5–1% NaOCl were used [Citation56].
Chlorhexidine is a potent antimicrobial agent that has been found to provide a significantly higher percentage of bacteria-free root canals in vivo [Citation57]. The good antibacterial efficacy is achieved by binding to the dentine organic matrix and diffusion into dentinal tubules [Citation58]. A possible drawback described in in vitro studies is that chlorhexidine might have negative impact on the survival of the stem cells from the apical papilla. Efforts to reverse its residual effect have been described in the literature [Citation59]. Nevertheless, there is a knowledge gap evaluating the potential cytotoxicity of chlorhexidine versus its antimicrobial efficacy on the clinical outcome of pulp revitalization.
Although we found an indication for lower mean value of the disinfection efficacy in the failed outcomes in comparison with the successful outcomes in both subgroups, these did not reach statistical significance (). To summarize, we found no significant differences between the disinfection efficacy of calcium hydroxide and chlorhexidine gluconate.
Analyses of the primary bacterial composition of the root canal in immature traumatized teeth
Our findings confirmed that the composition of the primary microflora of immature traumatized necrotic teeth was polymicrobial. A considerable diversity among the different cases was found (Supplementary Table S4). This finding agrees with other studies using PCR to investigate the microbial profile of immature traumatized teeth [Citation31,Citation60].
A finding we consider important is that the phenotypic analyses of the primary bacterial isolates with 16 rRNA gene revealed heterogeneity between the successful and the failed outcomes. As shown in Supplementary Figure S1, noticeably higher diversity was observed in the primary microflora in the successful cases than in the failed cases treated with both intra-canal dressings. Therefore, we further estimated the composition of the microflora. The majority of the species were obligate anaerobic and facultative anaerobic bacteria, a finding that has been observed by others [Citation61].
The most diverse isolates before treatment were Streptococci (S. intermedius, S. oralis, S. salivaris, S. sanguinis, and S. infantis), Porphyromonas (P. catoniae, P. uenonis, and P. pasteri), Prevotella (P. denticola, P. nigrencens, and P. oralis). Our findings agree with a recent review listing the most abundant species in preoperative samples from endodontically diseased teeth [Citation62].
We observed that Actinomyces species were present in the initial samples before debridement (S2) only in the subgroup treated with calcium hydroxide; these species presented with low abundance. In fact, the low abundance finding contradicts previously reported results on microbiota in immature necrotic teeth [Citation31]. Although disputable, the small sample size in the chlorhexidine subgroup could explain why Actinomyces species were not detected there.
Previous studies suggest that Porphyromonas species are associated with acute symptomatic endodontic infections [Citation63]. Nevertheless, the Porphyromonas species present in our study showed no association with acute signs and symptoms of endodontic infection. A possible explanation for this finding is that endodontic infections are polymicrobial, although no causation attributable to a single species has been established [Citation64]. In line with the above findings, it has been suggested that Prevotella species, such as P. intermedia and P. nigrescens, might also play an important role in acute endodontic infections [Citation65]. Interestingly, other studies have also tried to correlate the type of acute signs and symptoms of endodontic infection to particular combinations of bacterial taxa, such as Porphyromonas, Prevotella, and Peptostreptococcus species [Citation66]. Nevertheless, no such associations were found in this study. As studies have concluded that there is an elevated risk of acute symptoms if bacteria are pushed outside the apical foramen during treatment, care was taken to minimize that risk [Citation67].
The comparisons of phyla show that the isolates from both outcomes treated with both intra-canal dressings belong to five common phyla: Actinomycetota, Bacillota, Bacteroidota, Fusobacteriota, and Pseudomonadota. Likewise, other studies on endodontic microbial community profiles have identified these phyla as the top five phyla involved in endodontic infections in mature teeth [Citation62].
Furthermore, the primary bacterial composition in the successful outcome from the CH subgroup exhibited higher taxonomic diversity compared to the failed outcomes (Supplementary Figure S2). In addition to the five phyla found in both subgroups, the CH subgroup was populated with Campylobacterota, which is unique to this subgroup. As illustrated in , the successful outcomes were characterized by higher prevalence of this phylum compared to the failed outcomes. We identified C. gracilis, C. rectus, and C. showae as representatives of this phylum. Although it has been confirmed that this gram-negative phylum participates in primary endodontic infections and in periradicular disease, its precise ecologic role in endodontic communities is not understood [Citation68].
An interesting trend in the primary root canal microflora is the fact that the relative abundances of the three phyla – Bacteroidota, Actinomycetota, and Bacillota – showed statistically significant changes in both CH and CHD subgroups. In the CH subgroup, the abundances of the phyla Bacteroidota and Actinomycetota were higher in failed outcomes compared to successful outcomes, whereas in the CHD subgroup, these abundances were lower in the failed outcomes than the successful outcomes. A similar trend was observed for the phylum Bacillota. In the CH subgroup, the abundance of the phylum Bacillota was statistically significantly lower in the failed outcomes than the successful outcomes; however, in the CHD subgroup, the abundance of the phylum Bacillota was higher in the failed outcomes than the successful outcomes. A possible explanation for these shifts in relative phyla abundances is that the intracanal polymicrobial communities adapt to the changing environmental conditions after root canal debridement [Citation62]. That is, when the nutrients in the root canal become scarce, microbiota might survive by inducing a starvation response [Citation69].
Correlation of bacterial presence after root canal dressing (S5) to the clinical and radiographical outcomes of pulp revitalization
We show that bacterial microflora persisted after root canal dressing (S5) in both the successful and failed outcomes. Nevertheless, the abundance and diversity increased significantly only in the failed cases at S5. Twelve bacterial species persisted after application of root canal dressing (S5) in the failed outcomes: Cutibacterium acnes, Schaalia odontolyticus, and Slackia exigua from the phylum Actinomycetota; Eikenella corrodens and Kingella denitrificans from the phylum Pseudomonadota; Granulicatella adiacens, Paenibacillus glucanolyticus, Porphyromonas catoniae, Clostridiales bacterium, Streptococcus constellatus/intermedius, and Streptococcus gordonii from the phylum Bacillota. Our findings partially coincided with studies on the microbiome of post-treatment apical periodontitis [Citation70]. Furthermore, we found that 17 common species persisted after the application of intracanal dressing (S5) in both the successful and failed outcomes (Supplementary Table S4). Consistent with our findings, changes have been reported in abundances and diversities but no total elimination of the microbiota [Citation32].
We found that the taxa persisting after root canal dressing in the successful cases consisted exclusively of gram-positive species, while those identified in the failed cases consisted of a mixture of gram-positive and gram-negative species. This finding has been confirmed by reports that found gram-positive bacteria are significantly more prevalent than gram-negative bacteria in immature necrotic teeth with periapical lesions [Citation71]. Studies conducted on polymicrobial mixtures of gram-positive and gram-negative species highlight that endotoxins might play important roles in both host inflammatory response and clinical significance [Citation72]. Gram-negative bacteria are considered highly virulent due to lipopolysaccharide content (LPS) in their outer membranes [Citation73]. Another bacterial endotoxin, lipoteichoic acid (LTA), is present in gram-positive bacteria. The amplified effect of LPS and LTA activates the inflammatory response and favours bacterial adhesion and resistance to endodontic disinfectants, which causes treatment failure [Citation74,Citation75].
The persistence of low counts of bacterial species after application of root canal dressing (S5) in two of the successful PR cases treated with chlorhexidine gluconate needs further discussion. In ordinary conditions, the oral microbiome is harmless; however, after dental trauma, species in the oral microbiome can appear in direct proximity with the stem cells of the apical papilla (SCAPs). Therefore, microbial correlations with host phenotype might jeopardize the treatment outcomes of PR [Citation76]. In the presence of bacterial species, the immune and inflammatory response of SCAPs is upregulated, but cell division and proliferation are downregulated [Citation51]. Data obtained from animal models confirm that bacteria present even as a low-grade infection have negative effects on bone healing [Citation77,Citation78]. Thus, the role of the residual bacterial species should not be underestimated. PR protocol differs significantly from conventional endodontic treatment. In conventional endodontic treatment, the root canal is obturated after the treatment. In PR, after removal of the necrotic tissues from the root canal and intra-radicular disinfection, the treatment is accomplished by provoking bleeding into the root canal. The idea is that blood clot left inside the root canal serves as a matrix for the stem cells from the apical papilla to attach and proliferate, enabling continued root maturation. Thus, as the root canal is not being obturated with bacteria-tight sealing material in PR, resistant bacteria might repopulate the root canal. This repopulation of bacteria is of particular concern if bacterial species persist in the root canal after treatment.
The existing literature suggests that the presence of Enterococcus faecalis might be associated with treatment failure [Citation79]. However, our results could not confirm predominance or persistence of this species. In fact, we detected E. faecalis in three patients and at three different sampling time points. E. faecalis was detected in one sample before root canal debridement (S2), in another sample after root canal debridement (S3), and in another sample after root canal dressing (S5). Surprisingly, all the three cases were successful and the case where E. faecalis was detected at S5 did not show any presence of this species at S2 or S3. Thus, treatment failure could not be linked to the presence of E. faecalis. A possible explanation of this finding is that the Enterococcus strains are food-borne invaders, where the strains have been strictly selected for use in foods without any virulence genes and should be susceptible to clinically relevant antibiotics [Citation80]. We believe that enterococcal virulence factors are strain specific, suggesting that strains isolated in our study do not carry virulence determinants.
When bacterial persistence is discussed in terms of surviving in the presence of antibacterial agents, it is implied that the particular species are present before the initiation of treatment and the same species are identified in the samples from the same case after the treatment. Remarkably, in our study, none of the samples revealed any specific bacterial species present throughout all the time points or in a specific outcome of PR (S2, S3, and S5). In our opinion, it is not clear whether the identified bacterial species were present before treatment (S2), became predominant during treatment, or entered the root canals later due to failures in the aseptic technique and leakage through temporary fillings or due to cracks in enamel, incomplete fractures, or other trauma-associated pathways of colonization [Citation48]. For example, Paenibacillus glucanolyticus was not identified in the sample taken before root canal debridement (S2) but was present at post-treatment samples (S3 and S5). Although little is known about how Paenibacillus glucanolyticus colonizes human tissues, most likely it is a food-borne colonizer [Citation81]. Similarly, Cutibacterium acne was isolated in S2, S3, and S5 but from different CHD-treated patients. Consequently, it could be concluded that leakage explains the presence of bacterial species not identified before treatment but present at S3 and S5. Another possible explanation is that the bacteria were present in a very low concentration because of the microbiological dilution procedure and might have been missed.
Previous research has found that several species are associated with both periodontal and endodontic infections. These species are Porphyromonas gingivalis, Tannerella forsythia, and Treponema denticola, which form the so-called red complex present in periodontal disease [Citation82]. As the study material represented traumatized teeth, a possible correlation to periodontal pathways of colonization with these species is expected. However, only a Porphyromonas species (P. pasteri) was identified in one sample from the subgroup of failed PR cases treated with chlorhexidine gluconate. Thus, a correlation with periodontal pathways of colonization could not be stated. Interestingly, Porphyromonas sp. and Tannerella forsythia were identified in the samples before treatment and did not persist in the samples taken after root canal debridement (S3) or after root canal dressing (S5). This finding seems to be related to the fact that microbiota thrive in mixed infections and therefore enhancing nutritional niches [Citation83]. Moreover, approximately 50% of the oral microbiota were still uncultivable by culture-dependent methods [Citation84]. Other uncultivable microorganisms might play important roles in persistent endodontic infections [Citation85].
Strengths and limitations
A strength to be considered regarding the internal validity of this study is that we collected 123 samples from 41 root canals, which contribute to the field of endodontic microbiome in immature necrotic teeth. We provided analysis of the composition of the microbiome employing PCR techniques with culture-based approaches. Also, the study material was collected from homogenous patient group with randomization where only traumatized immature necrotic teeth were included. Furthermore, the root canal dressings (calcium hydroxide and chlorhexidine gluconate) were randomly allocated to the intervention groups.
Another strength of this study is that care was taken to minimize the risk for changes in the composition of the primary microflora of the traumatized immature teeth by including cases with no history of endodontic treatment (i.e. no previous access preparation had to be present before performing the samplings) and by taking contamination control samples before sampling from the root canal. This protocol is in accordance with previous research emphasizing the importance of established DNA decontamination control before taking samples from the root canal [Citation86].
The sampling technique should be discussed regarding both advantages and disadvantages.
On the one hand, we tried to counteract that only planktonic bacteria were collected during the root canal samplings. Before each sampling time point, dentin shavings from the root canal were produced using a hand file, which was struck towards the dentin wall of the root canal. This procedure has been established in bacterial samplings in PR and employed by others as the aim is to reach the adherent microbiota in the wide root canals of immature teeth with non-hand instrumentation debridement protocols [Citation32]. On the other hand, selective dentin shavings might contribute to analyses of certain niches in the root canal, causing the more representative microbiota to be overlooked.
A limitation of this study was that the groups were not equal despite the blocked randomization.
The high dropout rate in the chlorhexidine subgroup was due to technical reasons described in , and the challenging factor of limited participant recruitment during the COVID pandemic.
A possible limitation could concern the calculations of the disinfection efficacy for both the disinfection protocols used in the study. Although we noticed that the disinfection efficacy was lower in groups with the failed PR outcomes, no statistical difference could be shown due to our small sample size in the chlorhexidine subgroup, an issue that should be addressed in future research. Future research should concentrate on the second-generation next sequencing approach (Illumina) to identify the presence of non-cultivable bacteria [Citation62]. This approach would provide even deeper coverage and understanding of the ecologic aspects of polymicrobial endodontic communities in immature necrotic teeth.
Conclusions
There were no statistically significant differences in the disinfection efficacy of the two root canal dressing materials used in this study. The successful outcome cases not only presented higher diversity but also higher decreases of the primary microflora than in the failed outcome cases treated with both intracanal dressings. Bacterial microflora persisted after root canal dressing (S5) in both the successful and failed outcomes, but the abundance and diversity increased significantly only in the failed cases at S5.
Authors contribution
AW: Conceptualization; data curation; investigation; project administration; software; writing the original draft preparation and reviewing (Co-first authorship with equal contribution).
NRV: Formal analysis; validation; methodology; writing the original draft preparation and reviewing and editing (Co-first authorship with equal contribution).
OR: Laboratory analyses, statistical analyses and interpretation of the results; methodology; writing; reviewing, and editing.
GT: Conceptualization; formal analysis; supervision; funding acquisition; validation; writing, reviewing, and editing.
MB: Conceptualization; methodology; formal analysis; funding acquisition; supervision; validation; writing, reviewing, and editing.
DLG: Statistical analyses and interpretation of the results, reviewing, and editing.
Supplementary Tables to manus.docx
Download MS Word (208.5 KB)Supplementary Figure 3. Primary bacterial taxa cultured in both outcomes of successful and failed pulp revitalization cases in chlorhexidine gluconate subgrou.tif
Download TIFF Image (922.3 KB)Supplementary Figure 1. Primary bacterial taxa cultured in both outcomes of successful and failed pulp revitalization cases treated with both intra-canal dres.tif
Download TIFF Image (1.2 MB)Supplementary Figure 2. Primary bacterial taxa cultured in both outcomes of successful and failed pulp revitalization cases in calcium hydroxide subgroup (S2).tif
Download TIFF Image (1.2 MB)Disclosure statement
No potential conflict of interest was reported by the author(s).
Supplementary material
Supplemental data for this article can be accessed online at https://doi.org/10.1080/20002297.2024.2343518
Additional information
Funding
References
- Glendor U. Aetiology and risk factors related to traumatic dental injuries–a review of the literature. Dent Traumatol. 2009;25(1):19–20. doi: 10.1111/j.1600-9657.2008.00694.x
- Oldin A, Lundgren J, Nilsson M, et al. Traumatic dental injuries among children aged 0-17 years in the BITA study - a longitudinal Swedish multicenter study. Dent Traumatol. 2015;31(1):9–17. doi: 10.1111/edt.12125
- Lexomboon D, Carlson C, Andersson R, et al. Incidence and causes of dental trauma in children living in the county of Värmland, Sweden. Dent Traumatol. 2016;32(1):58–64. doi: 10.1111/edt.12218
- Borum MK, Andreasen JO. Therapeutic and economic implications of traumatic dental injuries in Denmark: an estimate based on 7549 patients treated at a major trauma centre. Int J Paediatr Dentistry/The British Paedodontic Soc [And] The Int Assoc Dentistry For Child. 2001;11(4):249–258. doi: 10.1046/j.1365-263X.2001.00277.x
- Haapasalo M, Endal U, Zandi H, et al. Eradication of endodontic infection by instrumentation and irrigation solutions.
- Ridell K, Petersson A, Matsson L, et al. Periapical status and technical quality of root-filled teeth in Swedish adolescents and young adults. A retrospective study. Acta Odontol Scand. 2006;64(2):104–110. doi: 10.1080/00016350500367637
- Kakoli P, Nandakumar R, Romberg E, et al. The effect of age on bacterial penetration of radicular dentin. J Endod. 2009;35(1):78–81. doi: 10.1016/j.joen.2008.10.004
- Carrigan PJ, Morse DR, Furst ML, et al. A scanning electron microscopic evaluation of human dentinal tubules according to age and location. J Endod. 1984;10(8):359–363. doi: 10.1016/S0099-2399(84)80155-7
- Hristov K, Gateva N, Stanimirov P, et al. Comparative analysis of root dentin loss when using modern mechanical cleaning instruments in immature permanent teeth. Folia Med (Plovdiv). 2020;62(2):352–357. doi: 10.3897/folmed.62.e39515
- Almutairi W, Yassen GH, Aminoshariae A, et al. Regenerative endodontics: a systematic analysis of the failed cases. J Endod. 2019;45(5):567–577. doi: 10.1016/j.joen.2019.02.004
- Botero T, Tang X, Gardner R, et al. Clinical evidence for regenerative endodontic procedures: immediate versus delayed induction? J Endodontics [Internet]. 2017;43(9s):S75–S81. Available from: https://www.cochranelibrary.com/central/doi/10.1002/central/CN-01618459/full. doi: 10.1016/j.joen.2017.07.009
- Ding RY, Cheung GSP, Chen J, et al. Pulp revascularization of immature teeth with apical periodontitis: a clinical study. J Endod. 2009;35(5):745–749. doi: 10.1016/j.joen.2009.02.009
- Ricucci D, Siqueira JF Jr. Biofilms and apical periodontitis: study of prevalence and association with clinical and histopathologic findings. J Endod. 2010;36(8):1277–1288. doi: 10.1016/j.joen.2010.04.007
- Petridis X, van der Sluis LWM, Dijkstra RJB, et al. Secreted products of oral bacteria and biofilms impede mineralization of apical papilla stem cells in TLR-, species-, and culture-dependent fashion. Sci Rep. 2018;8(1):12529. doi: 10.1038/s41598-018-30658-5
- Lin JC, Lu JX, Zeng Q, et al. Comparison of mineral trioxide aggregate and calcium hydroxide for apexification of immature permanent teeth: a systematic review and meta-analysis. J Formos Med Assoc. 2016;115(7):523–530. doi: 10.1016/j.jfma.2016.01.010
- Bose R, Nummikoski P, Hargreaves K. A retrospective evaluation of radiographic outcomes in immature teeth with necrotic root canal systems treated with regenerative endodontic procedures. J Endod. 2009;35(10):1343–1349. doi: 10.1016/j.joen.2009.06.021
- Torabinejad M, Nosrat A, Verma P, et al. Regenerative endodontic treatment or mineral trioxide aggregate apical plug in teeth with necrotic pulps and open apices: a systematic review and meta-analysis. J Endod. 2017;43(11):1806–1820. doi: 10.1016/j.joen.2017.06.029
- Fouad AF, Abbott PV, Tsilingaridis G, et al. International Association of Dental Traumatology guidelines for the management of traumatic dental injuries: 2. Avulsion of Permanent Teeth. Dental Traumatol. 2020;36(4):331–342. doi: 10.1111/edt.12573
- Peters LB, Wesselink PR, Buijs JF, et al. Viable bacteria in root dentinal tubules of teeth with apical periodontitis. J Endod. 2001;27(2):76–81. doi: 10.1097/00004770-200102000-00002
- Galler KM, Krastl G, Simon S, et al. European Society of Endodontology position statement: Revitalization procedures. Int Endod J. 2016;49(8):717–723. doi: 10.1111/iej.12629
- Orstavik D, Haapasalo M. Disinfection by endodontic irrigants and dressings of experimentally infected dentinal tubules. Endodontics Dent Traumatol. 1990;6(4):142–149. doi: 10.1111/j.1600-9657.1990.tb00409.x
- Andreasen JO, Borum MK, Jacobsen HL, et al. Replantation of 400 avulsed permanent incisors. 4. Factors related to periodontal ligament healing. Endodontics Dent Traumatol. 1995;11(2):76–89. doi: 10.1111/j.1600-9657.1995.tb00464.x
- Bakland LK, Andreasen JO. Will mineral trioxide aggregate replace calcium hydroxide in treating pulpal and periodontal healing complications subsequent to dental trauma? A review. Dental Traumatol. 2012;28(1):- 32. doi: 10.1111/j.1600-9657.2011.01049.x
- Sjogren U, Figdor D, Spangberg L, et al. The antimicrobial effect of calcium hydroxide as a short-term intracanal dressing. Int Endod J. 1991;24(3):119–125. doi: 10.1111/j.1365-2591.1991.tb00117.x
- Molander A, Reit C, Dahlen G. The antimicrobial effect of calcium hydroxide in root canals pretreated with 5% iodine potassium iodide. Endodontics Dent Traumatol. 1999;15(5):205–209. doi: 10.1111/j.1600-9657.1999.tb00775.x
- Molander A, Warfvinge J, Reit C, et al. Clinical and radiographic evaluation of one- and two-visit endodontic treatment of asymptomatic necrotic teeth with apical periodontitis: a randomized clinical trial. J Endod. 2007;33(10):1145–1148. doi: 10.1016/j.joen.2007.07.005
- Sheehy EC, Roberts GJ. Use of calcium hydroxide for apical barrier formation and healing in non-vital immature permanent teeth: a review. Br Dent J. 1997;183(7):241–246. doi: 10.1038/sj.bdj.4809477
- Mackie IC, Worthington HV, Hill FJ. A follow-up study of incisor teeth which had been treated by apical closure and root filling. Br Dent J. 1993;175(3):99–101. doi: 10.1038/sj.bdj.4808243
- Mohammadi Z, Abbott PV. The properties and applications of chlorhexidine in endodontics. Int Endodontic J. 2009;42(4):288–302. doi: 10.1111/j.1365-2591.2008.01540.x
- Pereira AC, Oliveira ML, Cerqueira-Neto A, et al. Treatment outcomes of pulp revascularization in traumatized immature teeth using calcium hydroxide and 2% chlorhexidine gel as intracanal medication. J Appl Oral Sci. 2020;28:e20200217. doi: 10.1590/1678-7757-2020-0217
- Nagata JY, Soares AJ, Souza-Filho FJ, et al. Microbial evaluation of traumatized teeth treated with triple antibiotic paste or calcium hydroxide with 2% chlorhexidine gel in pulp revascularization. J Endod. 2014;40(6):778–783. doi: 10.1016/j.joen.2014.01.038
- Fouad AF, Diogenes AR, Torabinejad M, et al. Microbiome changes during regenerative endodontic treatment using different methods of disinfection. J Endod. 2022;48(10):1273–1284. doi: 10.1016/j.joen.2022.07.004
- ICMJE. Recommendations for the conduct, reporting, editing and publication of scholarly work in medical journals; 2018 Sep 10. Available from: www.icmje.org/journals-following-the-icmje-recommendations/
- Puri KS, Suresh Kr F - Gogtay NJ, Gogtay Nj F - Thatte UM, Thatte UM Declaration of Helsinki, 2008: implications for stakeholders in research. (0972-2823 (Electronic)).
- Moher D, Hopewell S, Fau - Schulz KF, et al. CONSORT 2010 explanation and elaboration: updated guidelines for reporting parallel group randomised trials. (1743–9159 (Electronic)).
- Manoharan L, Brundin M, Rakhimova O, et al. New insights into the microbial profiles of infected root canals in traumatized teeth.LID - LID - 3877. (2077-0383 (Print)). doi: 10.3390/jcm9123877
- Holgerson PL, Claesson R, Ohman C, et al. Oral microbial profile discriminates breast-fed from formula-fed infants.J Paediatr Gastroenterol Nutr. 2013. doi: 10.1097/MPG.0b013e31826f2bc6
- Noordzij M, Dekker FW, Zoccali C, et al. Sample size calculations. Nephron Clin Pract. 2011;118(4):c319–23. doi: 10.1159/000322830
- Lenhard WLA. Computation of effect sizes; 2016. https://www.psychometrica.de/effect_size.html.
- Sadeghi S, Abdollahi S, Tarighi P, et al. Comparative evaluation of hydrogen peroxide sporicidal efficacy by different standard test methods. Iran J Microbiol. 2020;12(2):113–120. doi: 10.18502/ijm.v12i2.2616
- Ltd AE. alsenvironmental.Co.uk2017. Available from: https://www.alsenvironmental.co.uk/media-uk/pdf/datasheets/micro-lp/als_micro_limit-of-detection—microbiology_uk_2017.pdf
- Wikström A, Brundin M, Romani Vestman N, et al. Endodontic pulp revitalization in traumatized necrotic immature permanent incisors: Early failures and long-term outcomes—a longitudinal cohort study. Int Endodontic J. 2022;55(6):630–645. doi: 10.1111/iej.13735
- Sullivan GM, Feinn R. Using effect size-or why the P value is not enough. J Grad Med Educ. 2012;4(3):279–282. doi: 10.4300/JGME-D-12-00156.1
- Yarza P, Yilmaz P, Pruesse E, et al. Uniting the classification of cultured and uncultured bacteria and archaea using 16S rRNA gene sequences. 1740-1534 (Electronic).
- Sjogren U, Figdor D, Persson S, et al. Influence of infection at the time of root filling on the outcome of endodontic treatment of teeth with apical periodontitis. Int Endodontic J. 1997;30(5):297–306. doi: 10.1046/j.1365-2591.1997.00092.x
- Cvek M, Nord CE, Hollender L. Antimicrobial effect of root canal débridement in teeth with immature root. A clinical and microbiologic study. Odontol Revy. 1976;27(1):1–10.
- de-Jesus-Soares A, Prado MC, Nardello LCL, et al. Clinical and molecular microbiological evaluation of regenerative endodontic procedures in immature permanent teeth. J Endod. 2020;46(10):1448–1454. doi: 10.1016/j.joen.2020.07.005
- Fouad AF. Microbiological aspects of traumatic injuries. J Endod. 2019;45(12s):S39–s48. doi: 10.1016/j.joen.2019.05.011
- Ricucci D, Siqueira JF Jr., Loghin S, et al. Pulp and apical tissue response to deep caries in immature teeth: a histologic and histobacteriologic study. 1879–176X (Electronic)).
- Orlowski NB, Schimdt TF, Teixeira CDS, et al. Smear layer removal using passive ultrasonic irrigation and different concentrations of sodium hypochlorite. J Endod. 2020;46(11):1738–1744. doi: 10.1016/j.joen.2020.07.020
- Razghonova Y, Zymovets V, Wadelius P, et al. Transcriptome analysis reveals modulation of human stem cells from the apical papilla by species associated with dental root canal infection. Int J Mol Sci. 2022;23(22):14420. doi: 10.3390/ijms232214420
- Martin DE, De Almeida JF, Henry MA, et al. Concentration-dependent effect of sodium hypochlorite on stem cells of apical papilla survival and differentiation. J Endod. 2014;40(1):51–55. doi: 10.1016/j.joen.2013.07.026
- Trevino EG, Patwardhan AN, Henry MA, et al. Effect of irrigants on the survival of human stem cells of the apical papilla in a platelet-rich plasma scaffold in human root tips. J Endod. 2011;37(8):1109–1115. doi: 10.1016/j.joen.2011.05.013
- Ulin C, Magunacelaya-Barria M, Dahlén G, et al. Immediate clinical and microbiological evaluation of the effectiveness of 0.5% versus 3% sodium hypochlorite in root canal treatment: a quasi-randomized controlled trial. Int Endodontic J. 2020;53(5):591–603. doi: 10.1111/iej.13258
- Chávez de Paz LE, Bergenholtz G, Svensäter G. The effects of antimicrobials on endodontic biofilm bacteria. J Endod. 2010;36(1):70–77. doi: 10.1016/j.joen.2009.09.017
- Rosenfeld Ef F - James GA, James Ga F - Burch BS, Burch BS. Vital pulp tissue response to sodium hypochlorite. 0099–2399 (Print)).
- Zamany A, Safavi K, Spangberg LS. The effect of chlorhexidine as an endodontic disinfectant. Oral Surg Oral Med Oral Pathol Oral Radiol. 2003;96(5):578–581. doi: 10.1016/S1079-2104(03)00168-9
- Mohammadi Z. Chlorhexidine gluconate, its properties and applications in endodontics. Iran Endod J. 2008;2(4):113–125.
- Zamany A, Spångberg LS. An effective method of inactivating chlorhexidine. 1079–2104 (Print)).
- Fouad AF, Diogenes AR, Torabinejad M, et al. Microbiome changes during regenerative endodontic treatment using different methods of disinfection. 1878–3554 (Electronic).
- Möller AJ. Microbiological examination of root canals and periapical tissues of human teeth. Methodological studies. Odontologisk tidskrift. 1966;74(5):1–380.
- Shin JM, Luo T, Lee KH, et al. Deciphering endodontic microbial communities by next-generation sequencing. J Endod. 2018;44(7):1080–1087. doi: 10.1016/j.joen.2018.04.003
- Sundqvist G. Pathogenicity and virulence of black-pigmented gram-negative anaerobes. FEMS Immunol Med Microbiol. 1993;6(2–3):125–137. doi: 10.1111/j.1574-695X.1993.tb00315.x
- Nóbrega LM, Montagner F, Ribeiro AC, et al. Bacterial diversity of symptomatic primary endodontic infection by clonal analysis. Braz Oral Res. 2016;30(1):e103. doi: 10.1590/1807-3107BOR-2016.vol30.0103
- Haapasalo M, Ranta H, Ranta K, et al. Black-pigmented bacteroides spp. In human apical periodontitis. Infect Immun. 1986;53(1):149–153. doi: 10.1128/iai.53.1.149-153.1986
- Gomes BPFA, Lilley JD, Drucker DB. Associations of endodontic symptoms and signs with particular combinations of specific bacteria. Int Endodontic J. 1996;29(2):69–75. doi: 10.1111/j.1365-2591.1996.tb01164.x
- Siqueira JF Jr. Microbial causes of endodontic flare-ups. Int Endodontic J. 2003;36(7):453–463. doi: 10.1046/j.1365-2591.2003.00671.x
- Siqueira JF Jr., RôçRôçAs IN. Campylobacter gracilis and Campylobacter rectus in primary endodontic infections. 0143–2885 (Print)).
- de Paz LE C, Hamilton IR, Svensäter G. Oral bacteria in biofilms exhibit slow reactivation from nutrient deprivation. 1350–0872 (Print)).
- Siqueira JF Jr., Antunes HS, RôçRôçAs IN, et al. Microbiome in the apical root canal system of teeth with post-treatment apical periodontitis. (1932–6203 (Electronic)).
- Sharaf PH, El Backly RM, Sherif RA, et al. Microbial identification from traumatized immature permanent teeth with periapical lesions using matrix-assisted laser desorption/ionization time-of-flight mass spectrometry. (1472-6831 (Electronic)).
- Gabrielli ES, Lima AR, Francisco PA, et al. Comparative analysis of bacterial content, levels of lipopolysaccharides and lipoteichoic acid in symptomatic and asymptomatic endodontic infections at different stages of endodontic treatment. Clin Oral Investig. 2022;26(1):287–302. doi: 10.1007/s00784-021-03998-2
- Gomes BP, Martinho FC, Vianna ME. Comparison of 2.5% sodium hypochlorite and 2% chlorhexidine gel on oral bacterial lipopolysaccharide reduction from primarily infected root canals. J Endod. 2009;35(10):1350–1353. doi: 10.1016/j.joen.2009.06.011
- Ginsburg I. Role of lipoteichoic acid in infection and inflammation. Lancet Infect Dis. 2002;2(3):171–179. doi: 10.1016/S1473-3099(02)00226-8
- Fabretti F, Theilacker C, Baldassarri L, et al. Alanine esters of enterococcal lipoteichoic acid play a role in biofilm formation and resistance to antimicrobial peptides. Infect Immun. 2006;74(7):4164–4171. doi: 10.1128/IAI.00111-06
- Structure, function and diversity of the healthy human microbiome. 1476–4687 (Electronic)).
- Zheng Z, Yin W, Zara JN, et al. The use of BMP-2 coupled - Nanosilver-PLGA composite grafts to induce bone repair in grossly infected segmental defects. Biomaterials. 2010;31(35):9293–9300. doi: 10.1016/j.biomaterials.2010.08.041
- Moojen DJ, van Hellemondt G, Vogely HC, et al. Incidence of low-grade infection in aseptic loosening of total hip arthroplasty. Acta Orthop. 2010;81(6):667–673. doi: 10.3109/17453674.2010.525201
- Molander A, Reit C, Dahlen G, et al. Microbiological status of root-filled teeth with apical periodontitis. Int Endodontic J. 1998;31(1):1–7. doi: 10.1046/j.1365-2591.1998.t01-1-00111.x
- Ferchichi M, Sebei K, Boukerb A-O, et al. Enterococcus spp.: is it a bad choice for a good use-a conundrum to solve? LID - LID - 2222. (2076-2607 (Print)). doi: 10.3390/microorganisms9112222
- Sáez-Nieto JA, Medina-Pascual MJ, Carrasco G, et al. Paenibacillus spp. isolated from human and environmental samples in Spain: detection of 11 new species. New Microbes New Infect. 2017;19:19–27. doi: 10.1016/j.nmni.2017.05.006
- Gomes B, Montagner F, Jacinto RC, et al. Polymerase chain reaction of Porphyromonas gingivalis, Treponema denticola, and Tannerella forsythia in primary endodontic infections. J Endod. 2007;33(9):1049–1052. doi: 10.1016/j.joen.2007.05.017
- Gharbia SE, Shah HN. Interactions between black-pigmented gram-negative anaerobes and other species which may be important in disease development. FEMS Immunol Med Microbiol. 1993;6(2–3):173–177. doi: 10.1111/j.1574-695X.1993.tb00321.x
- Paster BJ, Olsen I, Aas JA, et al. The breadth of bacterial diversity in the human periodontal pocket and other oral sites. Periodontol 2000. 2006;42(1):80–87. doi: 10.1111/j.1600-0757.2006.00174.x
- Dewhirst FE, Chen T, Izard J, et al. The human oral microbiome. J bacteriol. 2010;192(19):5002–5017. doi: 10.1128/JB.00542-10
- Figdor DMFDPF, Brundin MDDSP. Contamination controls for analysis of root canal samples by molecular methods: an overlooked and unsolved problem. J Endod. 2016;42(7):1003–1008. doi: 10.1016/j.joen.2016.04.015