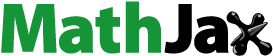
ABSTRACT
Sequestration of blue carbon (C) in mangrove plantations depends on site characteristics and plantation management. This study evaluated the effects of plantation management on C sequestration at a C-neutral site in Xiamen, China. A field study was conducted on 10-year-old Sonneratia apetala and Kandelia obovata plantations (mono-Sa, mono-Ko) and a 15-year-old mixed plantation-. We found that mono-Ko had a significantly higher ecosystem C sequestration rate (3.32 ± 0.62 kg C m−2 yr−1) than others when planted in the indirect shade of pioneer species. As a non-native species, Sonneratia performed better (0.57 ± 0.01 kg C m−2 yr−1) when planted with Kandelia than in monotypic plantations. The temporary and long-term certified emission reduction (tCER and lCER) of the 12.98 ha mono-Ko was 4103.89 and 3693.50 net CO2-e within 10 years, respectively. A literature review was conducted to show the compatibility of this study with other natural and plantation sites of China and Bangladesh (the native habitat for Sonneratia). The C sequestration of Sonneratia plantation sites in China is comparable with those in Bangladesh. However, the soil C accumulation rate for a Sonneratia monotypic plantation in Bangladesh decreased with age, yet the same did not occur in the plantations of China.
Introduction
Coastal wetlands are the most active biological and geochemical ecosystems (Gastusso, Frankignoulle, and Wollast Citation1998). Despite their very small spatial distribution (0.1% of all continental surface) (Kristensen et al. Citation2008; Donato et al. Citation2011), mangroves have the potential to cycle and trap more carbon (C) than any other ecosystem. Their C sequestration ability is a result of regular tidal inundation, muddy anaerobic soil, and their unique complex root system that efficiently traps suspended materials (Mcleod et al. Citation2011; Alongi Citation2012; Atwood et al. Citation2017). According to Keuskamp et al. (Citation2013), the mangrove ecosystem can bury triple to tenfold more C than other terrestrial ecosystems. The C sequestration rate of mangroves is on average 168 ± 36 g C m−2 y−1 (Breithaupt et al. Citation2012; Alongi Citation2012). As a result, mangroves draw the attention of researchers for their potential for C sequestration and as C sinks. Increasing human interference and global climate change are now causing drastic degradation of existing mangroves (Mcleod et al. Citation2011). However, afforestation and reforestation of mangrove species can be an effective method to increase the area of mangroves and restore degraded mangrove communities globally (Barbier Citation2006; Chang et al. Citation2006).
In 1990, the natural mangroves of China were designated national/regional natural reserves. Several mangrove reforestation projects have been initiated to achieve goals such as site restoration, biodiversity, C sequestration, and natural ecological services and functions (Ren et al. Citation2009; Feng et al. Citation2019). For rapid and successful restoration, China introduced Sonneratia apetala from Bangladesh in 1985 for its fast growth rate and high environmental suitability (Chen et al. Citation2009). S. apetala has been widely used as a pioneer species in restoration projects along the southeastern coasts of China throughout the last three decades (Lu, Yang, and Chen et al. Citation2014). As a pioneer afforestation species, S. apetala has accounted for more than 50% of the mangrove restoration area of China with a coverage of 3800 ha (Chen et al. Citation2009). S. apetala has the ability to increase stand biomass and area stability within a short period of time. It can also enhance organic C and nitrogen (N) content with its succession (Ren et al. Citation2008, Citation2010). In addition, S. apetala can transform a habitat and make it suitable for further colonization by the native species (Yu et al. Citation2020). Several studies have been conducted to estimate the growth and C content of mangrove plantations reforested with S. apetala in which researchers discuss the suitability of S. apetala plantation for restoration projects and the effect of plantation structure on total ecosystem C (Yu et al. Citation2020; Chen et al. Citation2012; Lu, Yang, and Chen et al. Citation2014; Feng et al. Citation2019; Ren et al. Citation2008, Citation2010). Before 2010, the studies were focused on the biomass and C sequestration of monocultural stands of S. apetala (Ren et al. Citation2008, Citation2010). After that, the study of S. apetala mixed plantation with other native species has been a point of focus.
Feng et al. (Citation2019) compared native mixed plantations with exotic S. apetala and found no significant advantages of S. apetala on the stocks of organic C and nutrients except for high biomass. The output and turnover of the mangrove net primary production may have been accelerated owing to low C/N ratio for high C accumulation capacity and high litterfall production. According to Yu et al. (Citation2020), the increase of biomass stopped after 15 years of planting with native mangroves. Eventually, the vegetation C density decreased over time. However, the soil C of the stand increased even after its above-ground growth stopped, owing to the high litterfall and dead roots entering into the soil and decomposing with time. According to Gao et al. (Citation2017), in Hainan the soil C density at a soil depth of 0–40 cm increased even after S apetala growth stopped at 13 years (Yu et al. Citation2020). The pioneer S. apetala also influences the regeneration and colonization of other associated mangroves. However, some studies have suggested that the native mangroves are adversely affected by the exotic species over a longer time scale (Ren et al. Citation2008, Citation2010). Since the beginning of restoration projects with S. apetala, a debate has raged about whether it is an invasive species or a restoration species. In the southern part of China, S. apetala has proved to be an invasive species threatening native mangroves (Ren et al. Citation2009). In the other areas, this species performed superbly in terms of site restoration, land stabilization, aboveground C accumulation, and had a positive influence on the regeneration and colonization of native mangrove species (Ren et al. Citation2009; Chen et al. Citation2012). Several studies of plantations with S. apetala and other species in different coastal regions of China gave a clear view that plantation strategy (mixture or monotypic) influences the afforested ecosystem in terms of functions and services (Lunstrum and Chen Citation2014; Yu et al. Citation2015; Feng et al. Citation2017; Yu et al. Citation2020). Studies of S. apetala in various regions have shown that the performance of mangroves depends on age and location (Feng et al. Citation2019).
As a coastal city, Xiamen has the opportunity to develop mangroves to increase the region’s biodiversity, purify seawater, and improve the scenic view. To offset C emissions, Xiamen started planting mangroves around Yundang Lagoon in 1990. Following this, the local government constructed a 38.7 ha mangrove plantation in Xiatanwei with the goal of producing a C-neutral BRICS summit (Liu Citation2017). Through this plantation, 3,095 metric tons of summit-generated C will be neutralized over 20 years and other values will be added to the environment, society, and economy (UNEP Citation2019). The plantation site is occupied by Kandelia obovata with the distinctive shape of “I love China” in Sonneratia apetala. This study was conducted in adjacent plantations of relatively young 10-year-old S. apetala and K. obovata at a similar tidal level and a mixed 15-year-old plantation in Xiatanwei, Xiamen, China. The goals of the study were: 1) to evaluate the blue C sequestration of Xiatanwei mangroves, which were planted to achieve C neutrality; 2) to assess the effect of plantation strategy on the C sequestration performance of Xiatanwei mangroves; and 3) to assess and compare the effects of plantation strategy and age on the C sequestration and annual soil C concentration rate of other Sonneratia restoration programs in China and Bangladesh, which were found through the literature review.
Materials and method
Study site
The Xiatanwei Mangrove Wetland Park is in Xiamen city, Fujian Province, China. It is a mangrove plantation site with a total planning area of 13,375 m2 of S. apetala (mono-Sa), 129,805 m2 of K. obovata (mono-Ko), and a total area of mixed plantation (containing S. apetala and K. obovata) (mix-SK) of 115,289 m2 (118°11′38″–118°11′32″E, 24°39′15″–24°38′16″N) (). A total of 4.05 ha area was planted with K. obovata and S. apetala as a mixed plantation in 2006. After the successful establishment of the mixed plantation, new plantations were established after 2011. K. obovata is the dominant species in this plantation with S. apetala, Avicennia marina, and Aegiceras corniculatam as the second dominant species. Low tidal flats are occupied by the primary dominant species and the high tidal flats are dominated by the secondary species. The park is situated at the transition zone of the north subtropical zone and warm temperate zone. The annual average precipitation is 1000 mm and the annual average temperature ranges from 13.7°C to 14.6°C (Yin and Yan Citation2020).
Field investigation and biomass estimation
The field survey for this study began in August 2020. A systematic sampling method was conducted in the Xiatanwei because of the homogenous distribution of the plant species in small sub-strata. A total of four plots for the 10-year-old mono-Sa, four plots for the 10-year-old mono-Ko, and three plots for the 15-year-old mixed plantation containing mix-SK were selected as a reference (). Eleven standard sampling plots of 5 m × 5 m, 2 m × 2 m, and 5 m × 5 m were selected for mono-Sa, mono-Ko, and mix-SK, respectively, covering an area of 416 m2. For S. apetala, the height and diameter at breast height (D) were measured. The tree height and base diameter (D0) of K. obovata were measured within the plots of both monotypic and mixed plantations. The biomass was calculated using species-species allometric equations as suggested by Ren et al. (Citation2010) and Tam et al. (Citation1995) for S. apetala and K. obovata, respectively. We also sited up a 1 m *1 m square with gauze under each canopy to get the litterfall. We collected the the litterfall twice monthly and brough it back to the laboratory. The litterfall samples were oven-dried at 60°C for 72 hours and the dry weight was measured using an electric balance.
The biomass of different mangroves was estimated by different allometric regression models (Appendix A). According to the corresponding C content in the reference, the carbon density was calculated for both species where the C conversion factors for K. obovata and S. apetala were 42.3% and 43.02% respectively (Yu et al. Citation2020). The total vegetative biomass was assessed by multiplying total biomass with C content for both monospecific and mixed plantations.
Soil sampling
For each plantation, three plots were chosen for soil sampling. Soil samples down to 100 cm depth were collected from each plot using a 50 cm steel soil corer. The soil was collected into 100 cm depth with the help of a 50 cm long extension arm. Each soil core was divided into 10 samples with a 10 cm interval, collected in a sealed bag, and transported to the laboratory. With a soil cutting ring, a fixed volume soil core was collected from every section to measure the bulk density. The fixed volume samples were oven-dried at 60°C for 72 hours and the dry weight was measured using an electric balance. The soil bulk density of each section was calculated as the ratio of soil dry weight and volume. The dry soil samples were ground by hand with a mortar and pestle and the shells, roots, and other impurities were removed. The ground soil was then screened in a 60 mesh sieve and placed in clean and fresh PP tubes. A total of 120 subsamples (3 replicates* 4 plantations *10 layers) were taken for C and N analysis (% of dry soil mass) in a Vario EL CHN auto-analyzer (Germany).
where C is total soil C content (kg C cm−3), VDn is soil bulk density (g cm−3) in n depth, and Cn is soil C concentration (%) for n depth.
The inorganic soil C was very low and the difference between total C and organic C was insignificant, so it was assumed that the total C was equivalent to organic C in this study. The total ecosystem C stock was calculated as the sum of above-ground C stock, below-ground C stock, and soil organic C stock.
Calculation of net GHG removal by the sink and temporary certified emission reductions
Following the guidelines in AR-AM0014-var 03 “Afforestation and reforestation of degraded mangrove habitats” (2013), the actual net GHG removal by the sink was calculated to understand the C-neutral potential of the plantation site. However, the plantation site does not fit the criteria as a degradation site. Therefore, the following equation was used to calculate net GHG removal by the sink:
where ΔCACTUAL,t is the actual net GHG removal by the sink in the year t (t CO2-e) and ΔCP,t is the change in C stock in the project occurring in the selected C pools in year t (t CO2-e). GHGE,t increases in non-CO2 GHG emissions within the project boundary as a result of the implementation of the A/R CDM project activity in year t, as estimated in the tool (t CO2-e). GHG emissions resulting from removal of herbaceous vegetation, combustion of fossil fuel, fertilizer application, use of wood, decomposition of litterfall and fine roots of N-fixing trees, construction of access roads within the project boundary, and transportation attributable to the project activity were considered insignificant and therefore counted as zero.
The net anthropogenic GHG removal by the sink was the same as the net GHG removal because the other two factors in the calculation of baseline GHG removal and leakage were 0 for the site. The number of units of temporary certified emission reductions (tCERt) and long-term certified emission reductions (lCERt) were calculated issuably in the year of this reporting according to the CDM manual (AR-AM0014 Citation2014). The number of tCERs earned at each verification is equal to the amount of sequestered CO2 at that moment, taking into account the baseline scenario. Within a verification period T = t2-t1 (where t1 and t2 are the beginning and end years of the verification period respectively), tCERt and lCERt can be verified as follows:
Where, is net estimated greenhouse gas removal in year t, t1 and t2 are the beginning and end years of the verification period respectively. Here, we choose t = 10 years.
Literature review
A literature review was conducted to support the field study data according to the method described by Lunstrum and Chen (Citation2014). They presented all of the available information on relative change in soil C up to 2010, so our review focused on articles published after 2010. Web-based literature search and citation indexes, including Web of Science, Google Scholar, and Research Gate, were searched for articles published between 2010 and 2020 that discussed mangrove forests in China and Bangladesh and met the following criteria: i) the forest contained a baseline young aged forest or ii) a baseline mudflat of known age like the previous study. A total of 15 studies and 1 unpublished data set were considered for this study.
The selected works of literature were divided into three groups: 1) S. apetala plantation in China, 2) native plantation in China, and 3) S. apetala plantation or natural in its native environment of Bangladesh and India. Ecosystem C was estimated for these three groups based on the age of the forest. A 30-year-old forest was considered to be the average natural forest in Bangladesh and India. However, the actual age of S. apetala in natural forests is indeterminate owing to the lack of information in the literature. Furthermore, ecosystem C data were lacking for both Bangladesh and India. In these situations, the DBH, tree height, and a common allometric equation were used to calculate the above-ground biomass (AGB), below-ground biomass (BGB), and C stock. As soil information was more available for S. apetala plantations, comparisons were also made of the annual change in soil C concentration from baseline for forests in China and Bangladesh with different age classes.
Due to the lack of soil bulk density or soil layer for C density from the searched papers, only soil C concentration (%) was considered in this study. The soil concentration data was divided into < 40 cm soil layers in four age groups (<5, 5–10, 10–30, and 40 years). The studies conducted on afforested or restored mangroves with S. apetala were compared to one study with K. obovata. Four studies conducted on afforested mangroves and native Sundarbans of Bangladesh were also considered. If an unknown aged natural forest was found, then the data were classified as a > 40-year-old stand. When multiple data sets (repeated measurements) were found, only the most recent published data were considered. For each observation, the calculation of annual change in soil C concentration was conducted using the following equation:
where ΔC is the amount of annual soil C accumulation average change from baseline to year t; Ct and C0 are the soil C concentration in site and baseline; and Δt is the age difference between baseline and site. To illustrate the magnitude of the change, the relative annual change of soil C concentration with baseline was calculated as ΔC/C0.
Data analysis
One-way ANOVA and Tukey HSD post-hoc tests were performed to evaluate the differences in soil C stocks, diameter at breast height, tree height, plant density, above/below-ground biomass and biomass C sequestration among all of the mangrove forests. Linear regressions were tested to determine the function that provided the optimal fit for the relationship between: (i) forest age and soil C stocks and (ii) biomass and soil C concentration. These statistical tests were conducted using SPSS 23.0 software (IBM SPSS Statistics for Windows, IBM Corp., Armonk, NY, USA) and plotted by Sigmaplot 13.
Results
Vegetation structure and biomass carbon for monotypic and mixed plantation
Ten-year-old stands of both species had homogenous patches but the 15-year-old stand was planted in a heterogeneous way without any distinctive pattern. The morphological parameters of the monotypic and mixed plantation are presented in . The tree density of K. obovata differs significantly between monotypic and mixed forest types: the density of K. obovata in mixed plantations was nearly three times lower than in monotypic plantations. No significant difference in S. apetala density was found for the monotypic and mixed plantations, but tree density of mono-Ko differs significantly from other plantations. There were no dead trees at the mono-Sa and mono-Ko sites indicating that the existing plant density was the same as the initial planting. For mixed vegetation, the existing plant density was 2.28 ± 0.67 tree m−2 and the number of dead trees indicated that the initial tree density in age was 2.53 ± 0.31 tree m−2. However, the mono-Ko had higher AGB and BGB (22.55 ± 4.19 and 20.15 ± 3.73 kg m−2, respectively) than S. apetala even through the tree size was significantly lower. Both AGB and BGB per area for S. apetala increased by 45.03% and 63.2% from monotypic to mixed plantation, respectively, showing a positive relationship with plant age. In contrast, the AGB and BGB for K. obovata decreased 61% from monotypic to mixed plantation ().
Table 1. Morphology parameters of each mangrove community (mean ± SE, n = 4 for mono and 3 for mix) in Xiatanwei, Xiamen, China.
The one-way ANOVA showed that the plantation strategy (i.e., monotypic or mixed) has a significant effect on biomass and soil C sequestration (). The average stored C in AGB for mono-Sa was 4.6 (kg C m−2) while the biomass C increase for mix-SK and mono-Ko (16.01 and 24.68 kg C m−2, respectively). BGB also increased with a similar pattern similar to AGB for both species of the two types of plantation. For S. apetala, most of the biomass C was stored in their stem while K. obovata stored a high amount of C in their branches. The other tree components of mixed and monotypic plantations of K. obovata had almost similar C contents. The ratio of leaf biomass C to total tree biomass C (21%) did not show any difference between monotypic and mixed plantations. The total tree biomass C of mono-Ko was significantly higher (33.2 ± 12.61 kg C m−2) than others. K. obovata also had higher biomass than S. apetala in both sites. The annual biomass C accumulation rate for K. obovata in monotypic and mixed stands were 3.32 ± 0.62 and 0.85 ± 0.33 kg C m−2 yr−1, respectively. The annual biomass C accumulation rates for S. apetala in monotypic and mixed stands were 0.57 ± 0.01 and 0.54 ± 0.21 kg C m−2 yr−1, respectively (). The annual biomass C accumulation rates of K. obovata indicate that monotypic plantations had higher C accumulation than mixed plantations.
Table 2. One-way ANOVA of the site condition effect on different part of biomass and soil C properties. Plantation variation means monotypic plantation or mixed plantation.
Soil parameters
The soil bulk density varied for every site but in general, mudflats had a significantly lower bulk density than others. The average bulk density ranged from 0.98 g cm−3 to 1.15 g cm−3 for mudflat (MF) and mono-Ko (). At every site, bulk density increased with depth. In bare mudflats, gravels and sands were found below 60 cm depth. The soil bulk density changed for every site, whereas the C concentration decreased in all sites with depth (). The three plantation sites had average soil C concentration and soil C content significantly higher than the average value of mudflats. Soil in the mono-Sa at 0–10 cm depth had the highest C concentration (2.47%) while soil in the mono-Ko at 30–40 cm depth had the lowest C concentration (0.47%) (considering only the sites having vegetation cover).
Soil carbon accumulation
The soil C content of vegetated sites had a significantly higher value than mudflats (). A significant relationship was found between forest age and total C content for both species (r2 = 0.735; p < 0.05). The soil C accumulation rate of mono-Sa and mono-Ko was 0.5 ± 0.25 MgCha−1 yr−1 and 0.44 ± 0.12 MgCha−1 yr−1, respectively. The soil C accumulation rate of mix-SK was 0.45 ± 0.065 MgCha−1 yr−1. Despite having higher soil bulk density, mono-Ko had a slightly lower soil C accumulation rate. It was not possible to differentiate the soil C content in the mixed plantation because this site was planted heterogeneously with two species. The slope of the regression line displayed that the average annual C accumulation rate for the sites of the plantation was 0.45 MgCha−1 yr−1.
Table 3. Biomass carbon sequestration, soil carbon accumulation, and CO2 emission reduction potential (mean ± SE, n = 3) in Xiatanwei, Xiamen, China.
Ecosystem blue carbon density and sequestration
Ecosystem blue C sequestration for all the sites is shown in where the total ecosystem C stock (sum of soil organic C and vegetation C) was 13.54, 17.62, 40.40, 22.43, and 2.84 kg C m−2 for monotypic and mixed plantation of S. apetala and K. obovata and mudflat, respectively. The total ecosystem C stock was significantly higher for mono-Ko (considering individual species for mixed plantation) and significantly lower for open mudflats (p < 0.05). The C stock relative to total C was higher in mixed plantations (58%) than in monotypic S. apetala plantations (54%). The soil C was also higher in mixed plantations (82%) than in monotypic plantations (18%). However, these results indicate that this ecosystem C depends on vegetation C rather than soil C stock, except for mono-Sa. The C sequestration rate of the three plantation types had significant differences from each other in terms of biomass C (). However, the soil C sequestration rates did not show any significant differences. The vegetation C seque-stration rate of mono-Ko was 3.32 ± 0.62 kg C m−2 yr−1; for mixed plantation, it was 1.4 ± 0.54 kg C m−2 yr−1; and for mono-Sa, it was 0.57 ± 0.10 kg C m−2 yr−1. These results indicate that the ecosystem C sequestration rate depends on the plantation management. Most importantly, the total C sequestration of the mangrove plantation depends on the vegetation biomass and age. Over a certain period of time, the soil C sequestration rate becomes slower depending on monospecific or mixed plantation formation. However, the results indicate the potential of mangrove plantations in Xiatanwei, China as a C-neutral site.
Figure 3. Effect of plantation strategy on total ecosystem carbon density. Uppercase letters indicate significant difference from vegetation and litterfall biomass carbon density; Lowercase letters indicate significant difference from soil organic carbon density, P < 0.05. Error bars represent SE. n = 3.

Emission reduction potential as carbon-neutral site and its services
The net actual GHG removal in the sink was quite high after 10 and 15 years of plantation. Currently, the ΔCACTUAL,t value of the plantation site was 2622.19 Mt C, which is quite close to the emissions caused by the Summit. shows the total C stock development (i.e., C in above-ground biomass, below-ground biomass, and soil organic C) of the project scenarios. These results showed that the mono-Ko gained the largest C stock during the time period, followed by the mix-SK. However, the tCER and lCER values of mono-Sa were found to be significantly lower than the mono-Ko and mix-SK. Despite this, united mono-Ko, mono-Sa, and mix-SK plantations of Xiatanwei successfully reduce the net emissions for both the long term and temporary patterns, which are reflected by the tCER and lCER values. The site does not provide any release of GHGs into the atmosphere because there is no biomass collection, no fuel burning, and no agricultural conversion. There is transportation and other construction work on the site, but the emissions from these sources are considered insignificant according to the manual (AR-AM0014 Citation2014). The site also shows potential for planting shade-tolerant species alongside the pioneer species to develop a C-neutral plantation site. However, further assessment is needed for this site and other sites in China with similar or different plantation strategy to estimate their emission reduction rate.
Literature review
Change of C stock for different sites depending on age
The ecosystem C under three different plantation criteria is presented in . It was observed that the C sequestration of native species in China and Bangladesh showed a positive relationship with age, except for the 70-year-old natural forest. As an introduced species in China, S. apetala does not show a positive relationship with age in the young stage. It was observed that S. apetala plantations in China for 2, 5, 10, and 12 years had lower C sequestration than the other lower-aged forest sites with mixed native species (Appendix B). However, the introduction of S. apetala in the restoration of Chinese mangroves has had a positive effect over time in terms of C sequestration when planted in monotypic strips. The S. apetala plantation in China had higher C sequestration potential than native plantations. S. apetala in its native environment had lower C sequestration than plantations in China, but there are complicating factors in this comparison. One is that most of the studies in Bangladesh or India did not measure both biomass and soil C. Other important reasons are rotational cutting and human intervention in the plantation sites in Bangladesh and India. According to Borua and Rahman (Citation2019), people who live near the S. apetala plantation in the southeastern region of Bangladesh collect fuelwood, fodder, aquaculture products, and products for medicinal purposes. In contrast, most of the plantations in China are free from biomass harvesting. Data for all S. apetala plantation areas in Bangladesh with different management systems and plantation criteria are limited. Therefore, this study creates further opportunities to study these sites for better understanding of the effect of management, site conditions, and plantation pattern (i.e., homogeneity or heterogeneity) on the C sequestration potential of S. apetala plantations.
Annual soil C concentration rate
All of the studies showed positive soil C concentrations from baseline. Two studies of < 5 years and one study of 5–10-year-old stands showed negative values because of either previous vegetation presence or a baseline site situated near an old mature forest. In China and Bangladesh, among all of the forest age groups, the youngest-aged forest (< 5 years) had high soil C concentration relative to baseline. Comparing the two countries, young mangroves in Bangladesh had higher soil C accumulation and soil C percentage relative to baseline. In the 0–10 cm forest layer, the average annual soil C concentration relative to baseline was 14.12% (). The average annual soil C concentration in < 5-year-old sites in China was 14.51% annual change relative to the baseline compared with the 16.12% annual change relative to the baseline for natural and plantation sites in Bangladesh. A previous study showed that the average annual soil C concentration change from baseline was 8% at this age (Lunstrum and Chen Citation2014).
Figure 5. Literature review of the annual change in soil C at <40 cm soil depth level binned by forest age in terms relative change of soil C concentration from baseline for China and Bangladesh. Error bars represent SE. n = 15.

At < 40 cm soil depth, the average annual soil C concentration change over time ranged from 0.17% to 0.05% and 0.266% to 0.01% in China and Bangladesh, respectively. Therefore, the average annual C concentration in Bangladesh was found to be slightly higher (0.089%) than China (0.086%). Having been planted or grown its natural habitat, the S. apetala had a lower soil C accumulation rate in Bangladesh than China between the ages of 5–40 years.
Discussion
Effect of plantation strategy on the growth and biomass
Most of the mangrove forests in China are afforested or reforested with the native mangrove species 3–5 m in height. The non-native S. apetala can reach heights up to 15–20 m and diameters up to 20–30 cm in the same region (Ren et al. Citation2010). Two different planting patterns of S. apetala had a higher growth rate than K. obovata. Despite having higher growth, monotypic and mixed Sa had a positive relationship between total vegetative biomass and plantation strategy (13.73 kg m−2 and 20.37 kg m−2, respectively). This value is similar to the 15-year-old S. apetala stand in Qi’ao Island, China (Yu et al. Citation2020) but lower than the 17-year-old plantation in Leizhou, China (Feng et al. Citation2019). Compared with trees growing in their native environment, the biomass of S. apetala in China was significantly inferior to the native habitat in Sundarbans (Mitra, Sengupta, and Banerjee Citation2012). However, the afforested S. apetala in Teknaf, Bangladesh had plant biomass per unit area (17.98 kg m−2) similar to that of this study (Miah and Hossain Citation2020).
Tidal level, salinity, temperature, humidity, and precipitation can be major factors affecting biomass and distribution variation at different sites. Furthermore, the biomass per area of a site depends on the density of the tree. In this study, the density decreased from 0.71 to 0.29 tree m−2 for S. apetala depending on plantation strategy and age. The self-thinning mechanism of S. apetala over time through interspecies competition may be a reason that the biomass per area is limited by its density in comparison with natural habitat (Feng et al. Citation2019). The K. obovata forests significantly decrease in biomass from 42.7 kg m−2 to 16.59 kg m−2 for monotypic to mixed stands. The biomass of mix-Ko is substantially lower than the biomass (23 kg m−2) of 6-year-old K. obovata in Shenzhen, China (Lunstrum and Chen Citation2014). However, mono-Ko had higher biomass than a 23-year-old monotypic plantation of K. obovata in Shenzhen, China, and a 17-year-old K. obovata monotypic plantation in Leizhou, China (Peng et al. Citation2016; Feng et al. Citation2019). This shows that the mono-Ko plantation was homogenous and contained more than double the tree density (5 tree m−2) than the mix-SK stands (2.28 tree m−2) where the initial tree density of the plantation was 2.53 tree m−2. The relatively small tree density of the mixed plantation stand could be the cause of the high mortality of K. obovata when planted under the shade of pioneer S. apetala. The previous study conducted in the Futian Reserve showed that an approximately 20-year-old mix stand can experience a decrease in tree density over time (Liao et al. Citation2004). This contradicts with other studies such as Chen et al. (Citation2004), which demonstrated that the K. obovata species can co-exist with an understory of pioneer S. apetala species to ensure more diversity.
Effect of mangrove plantation pattern on blue carbon
The vegetation C had a positive relationship with plant biomass distribution depending on plantation strategy where the stem of S. apetala in mix plantation stored higher biomass C than mono-Sa stand. In this study, S. apetala in mixed plantations stored 8.06 kg C m−2 with an annual accumulation rate of 0.54 kg C m−2 yr−1. This value is lower than the 15-year-old monotypic S. apetala stand on Qi’ao island (11.33 kg C m−2) (Yu et al. Citation2020) but higher than the 13-year-old monotypic S. apetala (6.5 kg C m−2) in Leizhou Bay, China (Gao et al. Citation2017). The biomass C of a S. apetala monotypic plantation in Bangladesh was 8.99 kg C m−2, which was similar to what was found in this study but significantly lower than the average biomass C in its natural habitat in Sundarbans (25.67 kg C m−2) (Ahmed and Iqbal Citation2011). The previously described self-thinning effect of S. apetala is the reason for the low biomass C in this study. Despite this decreased biomass, the biomass C of this study site comply with its 23-year-old native plantation site in Bangladesh. The C in the biomass of K. obovata does not depend on age, but is instead driven by plantation strategy and initial plantation density. Homogeneously planted mono-Ko stores 61% more than the K. obovata of the mixed (understory of pioneer species) stand in biomass C sequestration. This indicates that C sequestration of mono-Ko has been affected by the plantation strategy and initial plant density where the initial plant density was almost double than at the mixed site. The mono-Ko stored even more biomass C (33.2 kg C m−2) than mature K. obovata (11.52 kg C m−2) on Qi’ao Island, China (Yu et al. Citation2020) and another natural habitat (7.12 kg C m−2), the Manko wetland in Japan (Hoque, Sharma, and Hagihara Citation2011).
The average soil C concentration of this study changed positively over time and the patterns of plantations (0.65%–0.95%). Both monotypic S. apetala and K. obovata had low C concentrations. However, the result of 15-year-old mixed plantation (mix-SK) was similar to those of other plantations (0.94%) of China (Feng et al. Citation2019) but was lower than those of other young forests of China that have both pioneer and native species (Lunstrum and Chen Citation2014; Yu et al. Citation2015; Feng et al. Citation2017; Yu et al. Citation2020). A S. apetala plantation in this study had the same soil C concentration as a 7-year-old S. apetala plantation in Bangladesh (Shaifullah et al. Citation2008). All of the sites in this study had a lower value than the estimated global average of soil organic C concentration (Alongi Citation2014; Duarte, Middelburg, and Caraco Citation2005; Kristensen et al. Citation2008). The low soil C concentration in the 15-year-old mixed plantation may be attributed to the lower tree density (1.4 tree m−2) of the area. Additionally, spatial variation and restoration period may be a factor in this discrepancy in soil C. Lunstrum and Chen (Citation2014) observed the dissimilarity of global average C concentration and suggested that to assess global C, relatively young mangrove forests with low soil C should be considered in the future.
By taking into consideration the large differences in root biomass, the young (10 years) monotypic S. apetala and K. obovata species had similar C content (1–100 cm) at identical depths. This result does not support the soil development model proposed by Chen and Twilley (Citation1999). However, the soil C content increase for these two species is low now but could increase in the future. The previous study conducted by Lunstrum and Chen (Citation2014) found a similar result for a comparative study between 6-year-old K. obovata and S. apetala stands where S. apetala had similar soil C content despite having higher root biomass and growth. The lower C/N ratio of Sonneratia litterfall (Lu, Yang, and Chen et al. Citation2014) may expedite the microbial decomposition and reduce the C burial into the soil. Our literature review supports this and found a positive trends in soil C accumulation in young forests () by observing a consistent increase in the data set. Since most of the studies are not designed to address soil C, the data does not represent the actual average. A similar literature review conducted by Lunstrum and Chen (Citation2014) properly described this discrepancy and the importance of accurate assessment of soil C change in the approved project to ensure proper estimation of soil C accumulation.
In this study, both species have the potential to increase soil C and N content. This may occur due to autochthonous root tissue or litterfall and allochthonous materials such as phytoplankton (Xiong, Liao, and Wang Citation2018). The abundant litterfall can transfer the biomass into the soil and accelerate the accumulation of soil C content. For soil N, mangroves tissue as well as the high microbial activity in the rhizosphere can increase the soil N content (Inoue et al. Citation2011a). The N content of the litterfall also increases by the nitrogen fixation activities of the microorganisms during decomposition (Inoue et al. Citation2011b). The C/N ratio of the soil can identify the source of organic matter (Xiong, Liao, and Wang Citation2018). In this study, the C content at 0–30 cm soil depth with the roots mainly distributed and litterfall collected, was high for every plantation type other than mudflats, indicating the presence and contribution of the rhizosphere and litterfall of mangroves ().
Comparison of carbon density and accumulation rate among different ecosystem
Compared with the vegetation and soil C density of 60–100-year-old subtropical evergreen broad-leaved forest (11.95 kg C m−2 and 8.77 kg C m−2), the mixed S. apetala plantation of Xiatanwei had lower vegetation C density but higher soil C density. These differences are due to the continuous biomass contribution to the soil and root decomposition (Sun and Guan Citation2014; Cui et al. Citation2018). At the global scale, the salt marsh has lower soil C density and can store less C than the mangroves with a long-term turnover rate (Bianchi et al. Citation2013). In this study, the S. apetala and K. obovata had lower C density than the 80-year-old natural mangroves in Yangluo Bay (13.52 and 15.04 kg C m−2 in vegetation C and soil C, respectively). Only mono-Ko had higher vegetation C accmulation (33.21 kg C m−2) (Yu et al. Citation2020). Since the 15-year-old stands are mixed plantations, it is unclear which species contribute more to soil C accumulation. According to Gao et al. (Citation2017), the soil C accumulation of mangroves can be enhanced by high litterfall and the decomposition of dead S. apetala roots even after its growth stops. Therefore, S. apetala could be the species contributing soil C to the mixed plantation with the same plant density. Comparing plantation structure, it was clear that mono-Sa stored slightly more soil C in Xiatanwei. Furthermore, continuous monitoring is necessary to determine the pattern of soil C change.
The C stock of Xiatanwei was lower than the global average C stock of coastal wetlands (47.1 kg C m−2 for mangroves and 34 kg C m−2 for saltmarshes) (Nahlik and Fennessy Citation2016). Generally in mature ecosystems, the proportion of soil C is much higher than the vegetation C relative to the ecosystem C (Yu et al. Citation2020). Studies have shown that soil C of the mangrove ecosystem accounts for >90% of total C stock, indicating the important role of soil in C sequestration (Donato et al. Citation2011). In this study, mono-Sa behaved consistently with this statement but mono-Ko did not. However, the soil C accumulation rate in this study was higher than soil C in other Chinese artificial mangrove forests (Yin and Yan Citation2020). The soil C accumulation rate of restored mangroves in Viet Nam (0.51–0.81 kg C m−2 y−1) (Ha et al. Citation2018) and Malaysia (0.28–0.95 kg C m−2 y−1) (Adame et al. Citation2018) had a higher accumulation rate than a similar plantation in China. Thus, the results indicate the influence of geographic location on C stock and accumulation rate in mangroves.
The data from Bangladesh and India show that S. apetala had a lower soil C accumulation rate in these countries than in China (Appendix B). This may be due to a lack of detailed information of sites in the literature. Most of the literature did not state the actual age of the mangroves (in Sundarbans) or have any baseline data for the plantations and Sundarbans. Owing to the lack of this data, standard baseline data were selected for calculating the soil C concentration relative to baseline. Another possible reason could be the fact that almost all of the coastal plantations of Bangladesh are participatory where the local people can collect the brunches and litterfall including fruits from the plantation and natural sites for their daily household needs (Sheikh et al. Citation2021; Haque and Datta Citation2005). This can reduce the C transfer from vegetation into the soil and cause a reduction in soil C accumulation rate at plantation sites of Bangladesh. These data suggest a further scope of study in Bangladesh Sundarbans and plantation sites to calculate the annual soil C accumulation rate accurately.
As with other afforested sites, the Xiatanwei mangrove plantation can provide many services to the environment and society. The presence of egrets, crabs, and fish indicates that this plantation has increased the biodiversity of the area. New development of the site could provide a leisure area and scenic beauty for the local urban people. Furthermore, the plantation site can also support economic enterprises such as boat rentals and fishing activity. This type of multi-disciplinary service is essential for the modern urbanization era (UNEP Citation2019). Further study is needed about the multiple ecosystem services of this area to find its capacity to operationalize ecosystem service agendas.
Conclusion
The study demonstrated that the biomass C sequestration of mono-Ko increased more significantly than at two other sites. There was no significant difference between soil C sequestration in each plantation in Xiatanwei. However, the plantation strategy (i.e., homogeneous and heterogeneous) had a positive effect in both biomass and soil C sequestration. The plantation site with no anthropogenic degradation performed well in terms of GHG reduction as the site. From the literature review, it was clear that the site had an identical blue C accumulation rate as other plantation sites in China containing Sonneratia. Compared to trees growing in the native habitat of Sonneratia in Bangladesh, the soil C accumulation rate of China’s restored mangroves showed a similar effect for the native plantations in Bangladesh but quite lower than the natural Sundarbans. This may be due to the lack of specific data on monotypic and mature Sonneratia stands in Bangladesh Sundarbans. This comparison also indicates that the morphological and physiological site variation affects the blue C sequestration of mangroves. Long-term study of the Xiatanwei mangrove plantation is needed to monitor its blue C sequestration change over time. This will give a clear view of this plantation as a C-neutral site that also provides multiple services. Because the mangrove management strategy of Bangladesh is different than in China, more study is also needed in different plantation sites of Sonneratia in Bangladesh to identify the effects of management on blue C sequestration.
Acknowledgments
The authors thank Qiulian Lin, Yun Zhang, Xiaofang Jiang, Yan Chen, for their assistance on the field survey and data analysis.
Disclosure statement
No potential conflict of interest was reported by the author(s).
Additional information
Funding
References
- Adame, M. F., R. M. Zakaria, B. Fry, V. C. Chong, Y. Then, C. J. Brown, and S. Y. Lee. 2018. “Loss and Recovery of Carbon and Nitrogen after Mangrove Clearing.” Ocean Coast Manage 161: 117–15. doi:10.1016/j.ocecoaman.2018.04.019.
- Ahmed, I., and M. Z. Iqbal. 2011. “Sundarbans Carbon Inventory 2010, a Comparison with 1997 Inventory.” SAARC For Journal 1: 59–72.
- Alongi, D. M. 2012. “Carbon Sequestration in Mangrove Forests.” Carbon Manage 3 (3): 313–322. doi:10.4155/cmt.12.20.
- Alongi, D. M. 2014. “Carbon Cycling and Storage in Mangrove Forests.” Annual Review Marine Science 6 (1): 195–219. doi:10.1146/annurev-marine-010213-135020.
- AR-AM0014. 2014. ““Afforestation and Reforestation of Degraded Mangrove Habitats.”” A/R Large-scale Methodology 03. http://cdm.unfccc.int/Reference/index.html.
- Atwood, T. B., R. M. Connolly, A. Hanan, P. E. Carnell, C. M. Duarte, C. J. Ewers Lewis, X. Irigoien, et al. 2017. “Global Patterns in Mangrove Soil Carbon Stock and Losses.” Nature Climate Change 7 (7): 523–528. doi:10.1038/nclimate3326.
- Aysha, A., M. H. K. Abu, M. Mishra, Nesarul, M. H., Padhi, B. K., Mishra S. K., S. K., Islam, M. S., Idris, M. H., and Masum, M. B. 2015. “Sediment and Carbon Accumulation in sub-tropical Saltmarsh and Mangrove Habitats of north-eastern Coast of Bay of Bengal, Indian Ocean.” International Journa Fisheries Aqua Studies 2 (4): 184–189.
- Barbier, E. B. 2006. “Natural Barriers to Natural Disasters: Replanting Mangroves after the Tsunami.” Frontiers in Ecology and the Environment 4 (3): 124–131. doi:10.1890/1540-9295(2006)004.
- Bianchi, T. S., M. A. Allison, J. Zhao, X. Li, R. S. Comeaux, R. A. Feagin, and R. W. Kulawardhana. 2013. “Historical Reconstruction of Mangrove Expansion in the Gulf of Mexico: Linking Climate Change with Carbon Sequestration in Coastal Wetlands.” Estuarine, Coastal and Shelf Science 119: 7–16. doi:10.1016/j.ecss.2012.12.007.
- Borua, P., and S. H. Rahman. 2019. “Sustainable Livelihood of Vulnerable Communities in Southern Coast of Bangladesh through the Utilization of Mangroves.” Asian Journal of Water Environmental Pollution 16 (1): 59–67. doi:10.3233/AJW190007.
- Breithaupt, J. L., J. M. Smoak, C. J. S. T. J. Smith III, A. Hoare, and A. Hoare. 2012. “Organic Carbon Burial Rates in Mangrove Sediments: Strengthening the Global Budget.” Global Biogeochemical Cycles 26 (3): 1–11 GB3011. doi:10.1029/2012GB004375.
- Chang, S. E., B. J. Adams, J. Alder, P. R. Berke, R. Chuenpagdee, S. Ghosh, C. Wabnitz, et al. 2006. “Coastal Ecosystems and Tsunami Protection after the December 2004 Indian Ocean Tsunami.” Earthquake Spectra 22 (3): 863–887. doi:10.1193/2F1.2201971.
- Chen, R., and R. Twilley. 1999. “A Simulation Model of Organic Matter and Nutrient Accumulation in Mangrove Wetland Soils.” Biogeochemistry 44 (1): 93–118. doi:10.1007/BF00993000.
- Chen, Y., B. Liao, S. Zheng, M. Li, and X. Song. 2004. “Dynamics and species-diversities of Artificial Sonneratia Apetala, Sonneratia Caseolaris and Kandelia Candel Communities.” Chinese Journal Applied Ecologica 15 (6): 924–928.
- Chen, L., W. Wang, Y. Zhang, and G. Lin. 2009. “Recent Progresses in Mangrove Conservation, Restoration and Research in China.” Journal Plant Ecol-Uk 2 (2): 45–54. doi:10.1093/jpe/rtp009.
- Chen, L., X. Zeng, N. F. Y, W. Lu, Z. Luo, X. Du, J. Wang, et al. 2012. “Comprising Carbon Sequestration and Stand Structure of Monoculture and Mixed Mangrove Plantation of Sonneratia Caseolaris and S. Apetala in Southern China”. Forest ecology and Management 284: 222–229. 10.1016/j.foreco.2012.06.058.
- Cui, X., J. Liang, W. Lu, H. Chen, F. Liu, G. Lin, F. Xu, Y. Luo, and G. Lin. 2018. “Stronger Ecosystem Carbon Sequestration Potential of Mangrove Wetlands with respect to Terrestrial Forests in Subtropical China.” Agricultural Meteorology 249: 71–80. doi:10.1016/j.agrformet.2017.11.019.
- Donato, D. C., J. B. Kauffman, D. Murdiyarso, S. Kurnianto, M. Stidham, and Kanninen, M. 2011. “Mangroves among the Most carbon-rich Forests in the Tropics.” Nature Geoscience 4 (5): 293–297. doi:10.1038/ngeo1123.
- Duarte, C., J. Middelburg, and N. Caraco. 2005. “Major Role of Marine Vegetation on the Oceanic Carbon Cycle.” Biogeosciences. 10.5194/bg-2-1-2005, 2005 2 1: 1–8. 10.5194/bg-2-1-2005.
- Feng, J., J. Zhou, L. Wang, X. Cui, C. Ning, H. Wu, X. Zhu, and G. Lin. 2017. “Effects of Short Term Invasion of Spartina Alterniflora and the Subsequent Restoration of Native Mangroves on the Soil Organic Carbon, Nitrogen and Phosphorus Stock.” Chemosphere 184: 774–783. doi:10.1016/j.chemosphere.2017.06.060.
- Feng, J., X. Chui, J. Zhou, L. Wang, X. Zhu, and G. Lin. 2019. “Effect of Exotic and Native Mangrove Forest Plantations on Soil Organic Carbon, Nitrogen and Phosphorus Contents and Pools in Leizhou, China.” Catena 180: 1–7. doi:10.1016/j.catena.2019.04.018.
- Gao, T., W. Guan, J. Mao, Z. Jiang, and B. Liao. 2017. “ Carbon Storage and Influence Factors of Major Mangrove Communities in Fucheng, Leizhou Peninsula, Guangdong Province.“.” Ecology and Environmental Sciences, 26, 985–990.
- Gastusso, J. P., M. Frankignoulle, and R. Wollast. 1998. “Carbon and Carbonate Metabolism in Coastal Aquatic Ecosystems.” Annual Review of Ecologica Science 29 (1): 405–434. doi:10.1146/annurev.ecolsys.29.1.405.
- Ha, T. H., C. Marchand, J. Aime, H. N. Dang, N. H. Phan, X. T. Nguyen, and T. K. C. Nguyen. 2018. “Belowground Carbon Sequestration in a Mature Planted Mangroves (Northern Viet Nam).” Forest Ecology and Management 407: 191–199. doi:10.1016/j.foreco.2017.06.057.
- Haque, A. K. F., and D. K. Datta. 2005. “The Mangroves of Bangladesh.” International Journal Ecologica Environmental Science 31 (3): 245–253.
- Hoque, A., S. Sharma, and A. Hagihara. 2011. “Above and Belowground Carbon Acquisition of Mangrove Kandelia Obovata Trees in Manko Wetland, Okinawa, Japan.” International Journal Env 1 (1): 7–13. doi:10.1016/j.foreco.2012.06.058.
- Inoue, T., S. Nohara, K. Matsumoto, and Y. Anzai. 2011a. “What Happens to Soil Chemical Properties after Mangrove Plants Colonize”?” Plant and Soil 346 (1–2): 259–273. doi:10.1007/s11104-011-0816-9.
- Inoue, T., S. Nohara, H. Takagi, and Y. Anzai. 2011b. “Contrast of Nitrogen Contents around Roots of Mangrove Plants.” Plant and Soil 339 (1–2): 471–483. doi:10.1007/s11104-010-0604-y.
- Kamruzzaman, M., S. Ahmed, S. Paul, M. M. Rahman, and A. Osawa. 2018. “Stand Structure and Carbon Storage in the Oligohaline Zone of the Sundarbans Mangrove Forest, Bangladesh.” For Science Technik 14 (1): 23–28. doi:10.1080/21580103.2017.1417920.
- Keuskamp, J. A., H. Schmitt, H. J. Laanbroek, J. T. A. Verhoeven, and M. M. Hefting. 2013. “Nutrient Amendment Does Not Increase Mineralisation of Sequestered Carbon during Incubation of a Nitrogen Limited Mangrove Soil.” Soil Biology and Biochemistry 57: 822–829. doi:10.1016/j.soilbio.2012.08.007.
- Kristensen, E., S. Bouillon, T. Dittmar, and C. Marchand. 2008. “Organic Carbon Dynamics in Mangrove Ecosystems: A Review.” Aquatic Botanica 89 (2): 201–219. doi:10.1016/j.aquabot.2007.12.005.
- Liao, W., C. Lan, Q. Zan, Y. Wong, and N. F. Tam. 2004. “Growth Dynamics and Selfthinning of the Dominant Populations in the Mangrove Community.” Acta Bontanica Sinica 46 (5): 522–532.
- Liu, August 24. 2017. “Xiamen Promises a carbon-neutral BRICS Summit. China Climate Change info-net ”. http://en.ccchina.org.cn/Detail.aspx?newsId=68853&TId=96%22%20title=%22Xiamen%20promises%20a%20carbon-neutral%20BRICS%20Summit
- Lu, W., S. Yang, L. Chen, Wang, W., and Lin, G. 2014. ““Changes in Carbon Pool and Stand Structure of Native Subtropical Mangrove Forest after inner-planting with Exotic Species Sonneratia Apetala.”” PLoS ONE 9 (3): 1–8. doi:10.1371/journal.pone.0091238.
- Lunstrum, A., and L. Chen. 2014. “Soil Carbon Stocks and Accumulation in Young Mangrove Forests.” Soil Biology & Biochemistry 75: 223–232. doi:10.1016/j.soilbio.2014.04.008.
- Mamun, A. A., M. K. Hossain, M. H. Kabir, and M. A. Kader. 2021. “Coastal Afforestation Effect on Soil Physiochemical Properties at Sitakunda Coast of Chittagong, Bangladesh.” Journal of Forest and Environmental Science 37 (1): 25–34. doi:10.7747/JFES.2021.37.1.25.
- Mcleod, E., G. L. Chmura, S. Bouillon, R. Salm, M. Bjork, C. M. Duarte, C. E. Lovelock, W. H. Schlesiner, and B. R. Silliman. 2011. “A Blueprint for Blue Carbon: Toward an Improved Understanding of the Role of Vegetated Coastal Habitats in Sequestering CO 2.” Frontiers Ecologica Environmental 9 (10): 552–560. doi:10.1890/110004.
- Miah, M. D., and A. Hossain. 2020. “Carbon Concentration in the Coastal Afforested Sites of Cox’s Bazar, Bangladesh.” Forestist. doi:10.5152/forestist.2020.20012.
- Mitra, A., K. Sengupta, and K. Banerjee. 2012. “Spatial and Temporal Trends in Biomass and Carbon Sequestration Potential of Sonneratia Apetala Buch.-Ham in Indian Sundarbans.” Proceedings National Academic Science India Section B Biology Science 82 (2): 317–323. doi:10.1007/s40011-012-0021-5.
- Nahlik, A. M., and M. S. Fennessy. 2016. “Carbon Storage in US Wetlands.” Nature Communications 7 (1): 1–9. doi:10.1038/ncomms13835.
- Peng, C., J. Qian, X. Guo, H. Zhao, N. Hu, Q. Yang, C. Chen, and L. Chen. 2016. “Vegetation Carbon Stocks and Net Primary Productivity of the Mangrove Forests in Shenzhen China.” Chinese Journal of Applied Ecology 27 (7): 2059–2065. doi:10.13287/j.1001-9332.201607.029.
- Ren, H., S. Jian, H. Lu, Q. Zhang, W. Shen, W. Han, Z. Yin, and Q. Guo. 2008. “Restoration of Mangrove Plantations and Colonisation by Native Species in Leizhou Bay, South China.” Ecologica Research 23: 401–407. doi:10.1007/s11284-007-0393-9.
- Ren, H., H. Lu, W. Shen, C. Huang, Q. Guo, Z. Li, and S. Jian. 2009. ““Sonneratia Apetala Buch.Ham in the Mangrove Ecosystems of China: An Invasive Species or Restoration Species”?” Ecological Engineering 35 (8): 1243–1248. doi:10.1016/j.ecoleng.2009.05.008.
- Ren, H., H. Chen, Z. Li, and W. Han. 2010. “Biomass Accumulation and Carbon Storage of Four Different Aged Sonneratia Apetala Plantations in Southern China.” Plant and Soil 327 (1–2): 279–291. doi:10.1007/s11104-009-0053-7.
- Shaifullah, K. M., M. Mezbahuddin, M. Sujauddin, and S. M. S. Haque. 2008. “Effects of Coastal Afforestation on Some Soil Properties in Lakshmipur Coast of Bangladesh.” Journal For Research 19 (1): 32–36. doi:10.1007/s11676-008-0005-8.
- Sheikh, A. A., M. Halder, M. S. Talukder, and S. Saha. 2021. “The Dependency of Coastal Livelihood of Forest Resources, and Alternative Options in the Periphery of the Sundarbans Reserve Forest, Patharghata, Bangladesh.” Open Journal Forestry 11: 398–414. doi:10.4236/ojf.2021.114024.
- Sun, L., and D. S. Guan. 2014. “Carbon Stock of the Ecosystem of Lower Subtropical Broadleaved Evergreen Forests of Different Ages in Pearl River Delta, China.” Journal Tropica Science 26 (2): 249–258.
- Tam, N. F. Y., Y. S. Wong, C. Y. Lan, and G. Z. Chen. 1995. “Community Structure and Standing Crop Biomass of a Mangrove Forest in Futian Nature Reserve, Shenzhen, China.” Hydrobiologia 295 (1–3): 193–201. doi:10.1007/BF00029126.
- UNEP. May 2019. Carbon Neutral Project for BRICS Leaders Xiamen Meeting. NBS Good Practices from Chinese government. https://wedocs.unep.org/bitstream/handle/20.500.11822/29510/CarbonNeutral.pdf?sequence=1&isAllowed=y
- Wang, G., D. Guan, M. R. Peart, Y. Chen, and Y. Peng. 2013. “Ecosystem Carbon Stocks of Mangrove Forest in Yingluo Bay, Guangdong Province of South China.” Forest Ecology and Management 310: 539–546. doi:10.1016/j.foreco.2013.08.045.
- Wang, G., D. Guan, L. Xiao, and M. R. Peart. 2019. “Ecosystem Carbon Storage Affected by Intertidal Locations and Climatic Factors in Three Estuarine Mangrove Forests of South China.” Reg Environmental Change 19 (6): 1701–1712. doi:10.1007/s10113-019-01515-6.
- Xiong, Y., B. Liao, and F. Wang. 2018. “Mangrove Vegetation Enhances Soil Carbon Storage Primarily through in Situ Inputs Rather than Increasing Allochthonous Sediments.” Marine Pollution Bulletin 131: 378–385. doi:10.1016/j.marpolbul.2018.04.043.
- Yin, Y., and Z. Yan. 2020. “Variation of Soil Bacterial Diversity and Metabolic Function with Tidal Flat Elevation Gradient in an Artificial Mangrove Wetland.” The Science of the Total Environment 718: 137385. doi:10.1016/j.scitotenv.2020.137385.
- Yu, X. Q., Yang, J., Liu, L. M., Tian, Y. and Yu, Z. 2015. “Effects of Spartina alterniflora invasion on biogenic elements in a subtropical coastal mangrove wetland.” Environ Sci Pollut Res 22: 3107–3115. 10.1007/s11356-014-3568-2
- Yu, X. Q., J. Yang, L. M. Liu, Y. Tian, and Z. Yu. 2015. “Effects of Spartina Alterniflora Invasion on Biogenic Elements in a Subtropical Coastal Mangrove Wetland.” Environmental Science Pollution Research 22 (4): 3107–3115. doi:10.1007/s11356-014-3568-2.
- Yu, C., J. Feng, K. Liu, G. Wang, Y. Zhu, H. Chen, and D. Guan. 2020. “Changes in Ecosystem Carbon Stock following the Plantation of Exotic Sonneratia Apetala in Qi’ao Island, China.” The Science of the Total Environment 707: 137142. doi:10.1016/j.scitotenv.2020.137142.