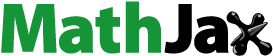
ABSTRACT
Landscape alterations and ecosystem services (ES) are crucial elements that affect the socio-ecological development of ecologically fragile regions. To provide scientific support for the land-use planning and regional ecological restoration to achieve sustainable development goals (SDGs) in the Loess Plateau from 1992 to 2015, this study proposes an integrated assessment framework for assessing the supply – demand match and equilibrium of ES combining potential relationships between landscape alterations, ES, and sustainable development at the county and regional scales. Results show that more than 85% of local counties have a supply – demand mismatch in terms of ecosystem services. Supply – demand distributions for provisioning and supporting services were relatively balanced compared to cultural services, which were imbalanced. Although the overall supply – demand relationship is relatively balanced because of the significant influence of ecological restoration, it shows an increasing trend toward imbalance as human – land conflict is prominent in some regions. SDGs emphasize on specific ES information such as ecosystem conservation, sustainable agriculture, and urban construction. Furtherly, positive impacts from landscape dynamics can improve the supply capacity of the ES and contribute to regional sustainability.
Introduction
Supply – demand equilibrium of ecosystem services (ES) is a central issue in socio-ecological management, which effectively indicates that ES dynamics are affected by landscape patterns, decision-making, and human well-being (Dai et al. Citation2015; Wei et al. Citation2017). ES involve various human benefits obtained from ecosystems, thereby reflecting environmental conditions and utility (Ramel et al. Citation2020). The Millennium Ecosystem Assessment (MEA) classifies ES into provisioning, regulating, cultural, and supporting services, and is one of the most influential classifications and evaluation frameworks for ES (Marques et al. Citation2013). The term supply refers to the capacity of ecosystems to provide various products and services, whereas the term demand refers to the willingness to acquire or consume multiple products and services (Castillo-Eguskitza, Martín-López, and Onaindia Citation2018). Generally, the match between supply and demand of ES is analyzed in terms of quantity, quality, time, space, and stakeholders (Wang and Wang Citation2021). Due to mismatches between supply and demand of ES, there are quantitative differences and spatial misalignments (Geijzendorffer, Martin-Lopez, and Roche Citation2015), indicating changes in profit and loss between the provision of ecosystem functions and social needs (Lin et al. Citation2022). The imbalance or mismatches in the supply and demand of ES severely constrains the sustainability of the socio-ecological system at global and regional scales, indicating that the supply – demand assessment of ES is becoming a key challenge for interdisciplinary research and landscape management decision-making (Schirpke et al. Citation2019).
Supply – demand studies on ES are rapidly evolving from conceptual and integrated assessment methods to quantitative analysis (Tang et al. Citation2020), and mainstream methods include valuation based on ecological economics (e.g., monetary estimation and ecological footprint) (Costanza et al. Citation2014; Mancini et al. Citation2018) and processes-based models (Marques et al. Citation2013; Othoniel et al. Citation2019). However, these methods mainly focus on the supply side of the service, while the theoretical and assessment methods for ES demand are not yet fully developed. Assessment methods for ES demand mainly reflect two aspects. First, for a certain ES, supply services mostly use various forms of consumption as demand indicators, such as per capita water and food consumptions in each administrative unit (Yu et al. Citation2021). For regulation and support services, they use emissions (Xia et al. Citation2022), importance or vulnerability (Larondelle and Lauf Citation2016; Feurer et al. Citation2021), and accessibility (Ala-Hulkko et al. Citation2019) as demand indicators. For cultural services, they mostly use public preference and willingness as demand indicators. Second, for ES as a whole, socioeconomic indicators (e.g., land use intensity, population density, and GDP) are usually chosen to characterize ES demand (Sun et al. Citation2022). The above methods have been frequently applied in supply – demand assessment studies of ES at global and regional scales (Torres, Tiwari, and Atkinson Citation2021). For ecological economics and processes-based models, complex multisource data are required from biophysical and socioeconomic fields. Many studies related to supply and demand of ES have been conducted, including assessing supply and demand ratios of ES for water supply in Mediterranean basins (Boithias et al. Citation2014) and spatial patterns of supply and demand of ES in the Jing-Jin-Ji region in China (Chen et al. Citation2022). Most of these studies used methods such as supply and demand ratios of ES and coupled coordination models to determine supply and demand imbalances of ES (Cai et al. Citation2021; Tao et al. Citation2022). Previous studies tend to aggregate to a single ecosystem type at the administrative unit or watershed scale, with less attention to vulnerable areas (Cao et al. Citation2021; Wei et al. Citation2021). Usually, these studies spatially cover one or many typical ES indicators, and an integrated supply – demand relationship assessment of ES is lacking (Deng, Li, and Gibson Citation2016). The coupling coordination model is still inadequate as it only involves ecological variables without exploring the coupling between land use/cover changes (LUCC) and supply and demand of ES. The matrix approach is becoming a spatially explicit tool to evaluate supply – demand changes of ES by integrating LUCC data (Burkhard et al. Citation2009; Ramel et al. Citation2020), which has obvious advantages and high efficiency in regional scenarios (Lee et al. Citation2016; Luiza Petroni, Siqueira-Gay, and Lucia Casteli Figueiredo Gallardo Citation2022). The matrix method considers the changes in supply and demand of ES caused by the dynamics of LUCC. Datasets of LUCC are used as measurement proxies to facilitate the estimation process of ES and their changes in relation to changes in LUCC, with obvious advantages and high efficiency in regional scenarios (Arowolo et al. Citation2018). Preliminary applications of the matrix assessment focused on the identification of spatiotemporal dynamics of supply – demand of ES under land use scenarios (Sun et al. Citation2019). The Gini coefficient is an emerging measure of the degree of equilibrium in the distribution of evaluation indicators (Cai et al. Citation2017; Benra and Nahuelhual Citation2019), such as inequalities between urban green space area and ES (Chen et al. Citation2022) and inequalities between ES and well-being (Yang et al. Citation2021). Overall, a comprehensive assessment of supply – demand relationship of ES is still scarce due to the limitation of its data availability and suitable methods (Sun et al. Citation2020).
Sustainable ES management is an emerging issue (Yuan and Lo Citation2020). The Intergovernmental Science – Policy Platform on Biodiversity and Ecosystem Services focuses on the sustainable assessment of ES and biodiversity at the global scale. The United Nations has proposed 17 Sustainable Development Goals (SDGs) with 169 nested targets as an inclusive solution (Yang et al. Citation2020). Among them, SDG 13 (climate action), SDG 14 (life underwater), and SDG 15 (life on land) emphasize improving the value of ES and livelihoods to increase the resilience of socio-ecological systems (Lee et al. Citation2016). The abovementioned studies show that changes in the supply – demand relationships of ES due to anthropogenic disturbances could have cascading effects and affect the implementation of SDGs and human well-being (Linders et al. Citation2020). Furthermore, LUCC processes under anthropogenic stress can also affect the implementation of SDGs by changing the supply – demand relationship of ES. Thus, a quantitative assessment of the association between ES and SDGs is imperative (Yang et al. Citation2020). Therefore, the analysis of the LUCC – ES–SDGs nexus can lead decision-makers to develop rational ecological restoration projects and socio-ecological regulation schemes, and thus it is an emerging hotspot in sustainable socio-ecological systems management (Peng et al. Citation2021). However, the level of ES changes required to cause impacts that could be used to monitor SDGs and reflect LUCC processes remains unclear (Yang et al. Citation2020).
The Chinese Loess Plateau (LP) is an ecologically fragile area with various ecological goods and services that are spatially imbalanced. For example, the regional supply of clean water cannot meet the demand due to shortages (Feng et al. Citation2020), and there is an imbalance in the supply – demand of carbon sequestration in forests and grasslands (Yang et al. Citation2021). China has implemented a series of socio-ecological system management measures in the LP, such as the Grain for Green (GfG) program (Fu et al. Citation2011, Citation2017) and the Loess Plateau Comprehensive Management Outline (2010–2030) in 2011. The “greenness” of the LP is recovering due to these efforts (Feng et al. Citation2017); however, rapid vegetation expansion may lead to unsustainable water demand by the ecosystem (Wu, Liu et al. Citation2019) and also affect related ES (e.g., food security) (Chen et al. Citation2015). To address the scientific issues and policy needs mentioned above, we (1) analyzed the LUCC spatiotemporal dynamics during 1992–2015 using the spatial mapping method; (2) assessed the multi-scale spatiotemporal variation characteristics, matching patterns, and equilibrium of supply and demand of ES integrating LUCC, matrix – index assessment, and Gini methods; (3) identified the potential impact of supply and demand of ES driven by the LUCC processes on the SDGs using a semi-quantitative approach. The comprehensive assessment of the nexus between LUCC, ES, and SDGs under the supply – demand dynamics of ES will provide crucial scientific support for the management of socio-ecological systems and optimization of decision-making in the LP.
Methods
Study area
The LP (33.68° N–41.82° N, 100.85° E–114.55° E) accounts for 6.76% (6.4 × 105 km2) of the total area of China (). It covers seven provinces/autonomous regions (including 341 counties). The elevation in this region is 75–5,149 m, the annual mean temperature is 3.6–14.3°C, the annual precipitation is 150–700 mm, and the annual evaporation is 1,400–2,000 mm (Fu et al. Citation2017). From 1999 to 2015, 88.20% of the vegetation in the LP was significantly restored, and the ecological management was effective (Lü et al. Citation2021). Socioeconomically, this region is a crucial part of the national policy for the large-scale development of western China (LSDWC). In 2015, the gross domestic product reached 5.38 trillion yuan, and the total and urban populations in the study area reached approximately 114 and 62.06 million, respectively. Regional landscape alterations have led to complex spatiotemporal heterogeneity in the supply and demand of ES (Yurui et al. Citation2021). For example, the increase in grain production in some regions has led to water shortages for supporting human activities, whereas 21.3% of counties in the LP showed a severe decrease in grain output (Feng et al. Citation2016; Wu, Liu et al. Citation2019), which seriously affects the implementation of SDGs in the region.
Data
LUCC maps were obtained from the Climate Change Initiative (CCI) provided by the European Space Agency (1992–2015, 300 m spatial resolution, https://www.esa–landcover–cci.org/). The dataset contains 22 initial LUCC types; this data is known to be highly reliable for environmental monitoring and climate change applications, with an overall accuracy of 75.4% (Fuchs, Prestele, and Verburg Citation2018; Li et al. Citation2018; Mousivand and Arsanjani Citation2019). The original LUCC classes with coding 10–210 in this region were reclassified into six types (cultivated land: 10–40; forests: 50–122, 160, and 170; grassland: 110 and 130; built-up land: 190; bare land: 140–153 and 200–202; waterbodies: 180 and 210) following the land monitoring classification of the Intergovernmental Panel on Climate Change (IPCC). Land-use forms and the 2030 SDGs Agenda (https://sdgs.un.org/2030agenda) were also collected for modeling.
LUCC analysis
The spatial change of landscape in the LP was analyzed using LUCC maps following the kernel density method using the following equation:
The kernel density function k() is a non-parametric spatial analysis method to calculate element densities in their neighborhood. The closer the value is to 1, the greater the amount of change in the element area (Xu et al. Citation2020). We converted the yearly changes in each land type into point data and calculated the kernel density values k() using ArcGIS 10.5, h is the bandwidth, x-xi is the distance from the estimated point x to the sample xi, and n is the number of samples.
Supply – demand matrices of ES
The ES indicators were collected from different landscape patterns based on the environmental and socioeconomic characteristics of the LP (Liu, Liang, and Hashimoto Citation2020). Following the high salience principle of supply and demand of ES, we selected services of high regional interest, including eight provisioning services, six regulating services, four cultural services, and three supporting services. We used the modified LUCC-based score matrix, which follows a three-step assessment procedure to assess the potential supply – demand relationships in ES (Burkhard et al. Citation2009; Ramel et al. Citation2020). First, the 44 land types in Burkhard’s matrices were merged into six based on similar land classes. Second, we searched in Web of Science™ for literature on Burkhard’s matrix to calibrate the supply and demand matrix based on the matrix values of case studies (Tao et al. Citation2018; Wu, Liu et al. Citation2019; Sun et al. Citation2020; Li et al. Citation2022). Third, the final scores were discussed and determined by four professors who worked together. Therefore, a threshold scoring (0–2) was used to develop the supply – demand matrix of ES integrating 21 ES indicators with six landscape types (). Specifically, the supply matrix reflected the capacity of the landscape to supply ecological products or services and divided it into low (<1), medium (1–2), and high ( = 2) supply. The supply capacity of forests and waterbodies in provisioning services was 1.25, and for others it was ≤1. Forests, grasslands, and waterbodies had higher supply capacities in regulating services compared to other land types. The supply capacities of major land cover types were >1 in cultural and supporting services (). The demand matrix assessed the level of human demand for ecological products or services from a specific landscape, including low (<1), medium (1–2), and high ( = 2) demand. The demand level was higher in the human-dominated built-up landscape than in the other landscapes. Natural ecosystem types have low demand levels because of their low population distribution and low product consumption. Cultivated land has high demand values (e.g., pollination and photosynthesis) in regulating and supporting services ().
Figure 2. Supply (a) and demand (b) matrix of ecosystem services inferred from six land-cover types in the Loess Plateau.

Second, an integrated index IAESC was used to indicate the supply – demand levels of the LUCC system at a specific spatial scale (Vihervaara et al. Citation2010; Liu, Liang, and Hashimoto Citation2020).
where yj is the average supply capacity or demand level of ES, Aj and A are the area of LUCC type j and the total area of this region (unit: km2), respectively. After using EquationEquation 2(2)
(2) , all the values of IAESC were normalized using Z-scores using EquationEquations 3
(3)
(3) –Equation5
(5)
(5) (Cao et al. Citation2021). Z is the normalized supply – demand levels of ES at a specific administrative unit, μf is the supply values or demand values of the f–th unit,
and S are the mean and standard deviation of μf in all the units, respectively. To break down the characteristics of the changes in the overall supply – demand patterns of ES in the LP and to identify the mismatch between the supply and demand of ES at spatiotemporal scales, the supply (x–axis) and demand (y–axis) values were plotted to generate four quadrant diagrams and obtain high supply – high demand (H – H), low supply – high demand (L – H), low supply – low demand (L – L), and high supply – low demand (H – L) patterns.
Equilibrium assessment of ecosystem services
An integrated Gini coefficient, which allows for more targeted ecological management, was used to reflect the equilibrium of supply and demand of ES in the LP (Wu, Liu et al. Citation2020). First, the normalized values of IAESC were arranged in an ascending order, and the Lorenz curve was plotted. A single Gini coefficient was derived using the cumulative percentage of IAESC from demand (x–axis) and supply (y–axis) in a specific ES type. Finally, the Gini coefficients were aggregated to obtain the integrated Gini coefficient, and its value reflected the equilibrium of supply – demand distribution of ES in the LP (Cai et al. Citation2017).
where B1 is the area between the 45° diagonal and the Lorenz curve, and B2 is the area under the curve. Based on previous studies (Teng et al. Citation2011; Wu, Liu et al. Citation2020), the types of Gini values are divided as: high equilibrium (<0.2), medium equilibrium (0.2–0.3), basic equilibrium (0.3–0.4), disequilibrium (0.4–0.6), and extreme disequilibrium (>0.6) of supply – demand distribution of ES.
In addition, LUCC – ES–SDGs nexus was analyzed using frequency statistics (Geijzendorffer et al. Citation2017; Wood et al. Citation2018), including the influencing intensity of LUCC – ES and the frequency of ES mentioned in the 2030 Agenda for Sustainable Development policy document (United Nations Citation2015). Thus, a multi-step and integrated assessment framework was developed to analyze the supply – demand equilibrium of ES at the county and regional scales in the LP during 1992–2015 ().
Results
LUCC analysis
Area analysis of land-use showed that the major landscapes in LP in 2015 were grassland (43.21%), cultivated land (41.8%), and forests (10.74%) (). Except for 2003, the area of cultivated and bare land from 1992 to 2015 showed a decrease of 2.45 and 2.3%, respectively. The area of built-up land, grasslands, and forests increased by 1.95, 1.74, and 1.01%, respectively, whereas the area of waterbodies increased slightly (0.05%). Affected by the national ecological restoration policies (e.g., GfG), the decreased cultivated land area was transferred to new grassland and forests. The policies (e.g., LSDWC) also drove the rapid economic development and urbanization, resulting in a significantly increased area of built-up land (2.05% in 2015).
The values of kernel density (>0.5) showed that the major changing types are built-up land, grassland, and cultivated land during the period. Cultivated land decreased significantly in the central LP, for example, a decrease of 496.83 km2 in Yan’an city (especially in the Wuqi, Zhidan, and Ansai counties). Increase in grassland was mainly observed at the borders of Shaanxi, Shanxi, and Inner Mongolia provinces (e.g., a 1792.92 km2 increase in Yulin City). In Jinzhong and Changzhi cities, forests had the most significant increase of 1164.94 and 1417.14 km2, respectively. The expansion of built-up land was primarily centered around Xi’an, Yuncheng, Taiyuan, and Luoyang cities, where the area of built-up land increased by 1090.23, 893.79, 460.49, and 670.97 km2, respectively. The most significant reduction in bare land area (3644.34 km2) was observed in Baotou city, as well as in the other regions of the Inner Mongolia province ().
Supply – demand patterns of ecosystem services
The supply – demand patterns of ES were divided into four types using the quadrant diagrams (). The overall trend in the pattern of matching supply and demand for ES at the county scale was the same from 2000 to 2015. In 1992, the four patterns of supply and demand of ES in all counties accounted for a balanced proportion of 20%; among them, the H – L pattern accounted for the lowest proportion of 22.97% and the L – H pattern had the largest proportion (27.7%) of the counties. The proportion of H – H and L – L patterns declined rapidly from 1992 to 2000, accounting for 7.72 and 6.04% of all the counties, respectively, and more than 85% of the counties showed L – H and H – L patterns. The H – H and L – L patterns showed significant transformations from a relatively small decrease to gradual stability from 2000–2008, and the H – L and L – H patterns showed a slightly decreasing trend. By 2015, the numbers of L – H (129) and H – L (138) patterns were significantly greater than the L – L (13) and H – H (18) patterns.
Figure 5. Quadrant diagram and proportion of supply (S)–demand (D) patterns of ecosystem services in the Loess Plateau during 1992–2015 (H–H: high supply–high demand; L–H: low supply–high demand; L–L: low supply–low demand; H–L: high supply–low demand).

The spatial ES distribution showed that the supply capacity was generally low in the southwestern LP, and the demand level was high in the central region. A coexistent pattern with the H – L type was detected in the northeastern regions of the LP in 1992. After 1992, the distribution of supply – demand showed a similar pattern, but with significant differences in the proportions (). For example, the H – L pattern accounted for 58.24% in 2015, mainly located in the forests and grassland landscapes, indicating that the ecological environment had improved significantly through long-term water – soil conservation and eco-restoration. The region proposes to adopt an ecological priority strategy to facilitate the movement of typical ES across the region while safeguarding the stability of the socioeconomic system. The L – H pattern was mainly concentrated in the provincial capital cities (e.g., Xi’an, Zhengzhou) and their surrounding areas, with high population density, human-dominated land use, and excessive ES consumption. It is necessary to improve the land-use efficiency and vegetation coverage in these area to mitigate the potential conflicts between low supply and high demand of ES. The L – L pattern was mainly distributed in the northern and southern parts of the LP, including 13 counties (50,274 km2), a dominant landscape in the desert area with weak service supply capacity and slow economic development. The area with the H – H pattern was the smallest (39,410 km2), and it has typical historical and cultural cities in the LP, such as Lintong, Helan, Zichang, Fugu, Shouyang, and Yuanping.
Supply – demand equilibrium of ecosystem services
The Gini coefficient of provisioning service showed a high equilibrium (<0.2) of supply – demand in the LP from 1992 to 2000. Further, it changed into a basic equilibrium (0.3246) affected by a decrease in provisioning of crops and timber for the implementation of the GfG policy from 2000 to 2015 (). The Gini coefficient of regulating services showed a disequilibrium during 1992–2015, indicating a large gap between the supply and demand distribution of regulating services. Particularly, the Gini coefficient decreased from 0.5683 in 2008 to 0.5516 in 2015, showing a slight decrease in the mismatch between the supply and demand of regulating services affected by the improvement in vegetation restoration and soil conservation capacity (). The Gini coefficient of cultural services showed an extreme disequilibrium (>0.6) during 1992–2015. There are many historical, cultural, and natural heritage sites in the study area. However, the development of recreation and ecotourism was inadequate, resulting in a significant imbalance between the supply and demand of cultural services (). The Gini coefficient of supporting services increased from 0.2139 in 1992 to 0.241 in 2015, indicating a medium equilibrium of supply – demand in supporting services. For example, a synergistic interaction between carbon and nitrogen cycles that was affected by the restoration of forest and grassland occurred during the period (). The synthesized Gini values (0.3–0.4) showed that the total supply – demand of ES in the LP exhibited equilibrium during 1992–2015. However, the trend toward a balanced supply and demand for synthesized ES has weakened (). Demand control and targeted ecological management program enhancements must be implemented to ensure ecological integrity and consistency.
Assessment of potential nexus of LUCC – ES–SDGs
The term ecosystem services has only been mentioned explicitly in SDG 15.1 (sustainable use of terrestrial ecosystem and services) in the document Transforming our World: The 2030 Agenda for Sustainable Development (https://sdgs.un.org/2030agenda), but all the SDG categories have described ES. The frequencies of four ES types in the description of 17 SDGs was provisioning (40), regulating (54), cultural (21), and supporting services (18) (). Among these SDGs, the most legible description for the nexus between LUCC, ES, and SDGs are Goal 15 (life on land), Goal 11 (sustainable cities and communities), and Goal 2 (zero hunger), which were mentioned 58, 18, and 17 times, respectively. For example, the LP region is a vital crop producing area in China, where long-term severe land degradation has caused a decline in soil fertility. The LUCC management of suitable cultivated land can improve the ecological environment of local crop production and create a sustainable food production system (SDG 2) (). In recent years, China has promoted water-efficient agricultural techniques for dryland farming, watercourse management projects in the valley plains, and the construction of check-dams in the LP, which have improved agricultural industrial structure, water and soil conservation, and farmers’ income simultaneously, as well as promoted SDG 2 and SDG 6. In addition, the typical land use forms such as the restoration of vegetation and optimization of livestock structure (e.g., adjusting the grass planting area and developing forage farming and grass-livestock industry) resulted in the rapid restoration of degraded land, which promoted the achievement of SDG 15. Overall, the restoration of degraded land improved the supply of ES, such as soil conservation, flood protection, nutrient cycling, and climate regulation. The diverse LUCC processes described above contributed positively to the supply – demand equilibrium of ES and they can also promote the achievement of key SDGs (e.g., SDG 15) ().
Figure 8. Frequency of ES types mentioned in the document transforming our world: The 2030 agenda for sustainable development. (P- provisioning services, R-regulating services, C-cultural services, and S-supporting services).

Figure 9. Potential nexus between LUCC–ES–SDGs (LUCC–ES: the wider the line, the stronger the relationship, and the node size of LUCC represents the influencing intensity of the landscape on ES, the larger the node, the stronger the relationship. The line width and node size were determined using the average supply capacity of the expert-based ES matrix. ES–ES indicators: The flow directions of the lines indicate ownership. ES indicators–sdgs: The node size and line represent the connection between ES and SDGs, which were determined according to the policy document).

Discussion
Applicability of LUCC matrices in the Loess Plateau
The integrated matrix method used in this study can assess spatial changes in the supply and demand of ES. The advantage of this method is that the expert scores can be corrected quickly to obtain a higher accuracy based on local ecological features. The coefficient of sensitivity (CS) was calculated to validate the reasonableness of the assessment method and the validity of the results (Wu, Liu et al. Citation2019). If CS < 1, ES are insensitive to the quantified value, indicating that the quantified method is reasonable. From the calculations, we found that the values for cultivated land and grassland were slightly greater than 1 while the CS values for all other land use types were <1, indicating that the results of the matrix model based on land use types were generally plausible (). However, accurate measurement of the realistic supply situation and demand level of ES still needs an in-depth exploration. It is essential to pay a great deal of attention to the connotation of supply and demand of ES to ensure the consistency of analysis and accuracies of quantitative methods.
Table 1. Changes in ES and sensitivity coefficient.
Link age of LUCC – ES–SDGs under ecological restoration
The implementation of ecological restoration projects has led to significant landscape alterations of the LP. For example, we found that 12.57 million km2 of cultivated land was converted to other land types during the research period, which is consistent with the previous LUCC studies in the LP region (Zhang et al. Citation2020; Liang, Hashimoto, and Liu Citation2021). LUCC affects ES mainly through different vegetation cover types. Factors such as topography and meteorology depend largely on physical geographic conditions, which do not change quickly (Wang et al. Citation2022). Therefore, spatial differences in the supply and demand of ES in different areas were closely related to the local spatial landscape patterns and socioeconomic conditions. Particularly, counties containing higher proportions of forests and grassland tended to be stronger in the provision of ES, while those with higher built-up land had lower supply capacities of ES or deficit in terms of supply – demand. The assessment also indicated that the enhancement of ecological projects and improvement of ecosystem management would alleviate the supply – demand imbalance of ES in the LP, which is generally consistent with conclusions in earlier studies (Wu, Liu et al. Citation2019; Jiang et al. Citation2021). In addition, a series of large-scale ecological measures since 1999 have led to profound changes in the ecological environment of the LP. For example, the vegetation cover in the LP increased by 17.06% and soil conservation efficacy increased by an average of 22% from 2000 to 2019 (Chinese Academy of Sciences Citation2020), and the desertification process in the northern LP began to reverse during the same period. These land use patterns have significantly improved the supply capacity of ES and promoted the achievement of local SDG15.
Supply – demand relationships in provisioning services were balanced in the study area; for example, food services can meet the local market demand in accordance with SDG2 (zero hunger) that focuses on sustainable food production systems (Chinese Academy of Sciences Citation2020). The supply process of ES is not immune to spillover; the shift in the center of gravity of food was toward the northwest in China in recent years (Zhong, Liu, and Wang Citation2022). The cultivated land area was reduced, regional vegetation recovery was accompanied by soil drying (Lü et al. Citation2021), and agricultural cultivation is highly dependent on regulating services (e.g., climate regulation and pollination). The imbalance between the supply and demand of LP regulation services is not conducive to SDG realization. Natural and resource-rich habitats should be created or protected by developing multifunctional forested landscapes combined with diverse crop rotations. Promotion of multifunctional sustainable crop production is required to ensure the sustainable development of the LP.
Implications of the assessment of sustainable development in the Loess Plateau
The LP occupies an important position in ensuring the overall ecological security and resource supply of China. We found that the high supply area of LP, which accounts for more than half of its total area, mainly belongs to the economically underdeveloped regions, and the urbanization level of this region lags behind in terms of ecological and environmental improvement. Therefore, achieving a win – win situation of socioeconomic development and environmental protection is still a key challenge for this region. In addition, the ecological barrier of LP, which mainly consists of forest, grassland, and cultivated land, is mainly distributed in the Guanzhong Plain and the eastern part of the Yellow River Basin (Zhang et al., Citation2020), and it plays an important role in maintaining regional ecological security. However, most areas in this region have low supply and high demand pattern of ES, and the growth rate of construction land is more significant than that of forest and grassland. Demand and consumption by human populations in these areas are increasing, and therefore, these are key areas in terms of ecological protection along with the strengthening of the supply and demand flow of ES with neighboring areas. In conclusion, good land use management is needed to enhance the synergy between multiple ES (Wu, Wang et al. Citation2019), balance between supply and demand of ES, and realization of SDGs.
Research limitations and prospects
The CCI LUCC dataset is the first global-scale land cover map with a high spatiotemporal resolution, and our study showed that this data could reveal changing trends of land use types at a regional scale over a long time series, which is consistent with the study by Yang et al. (Yang et al. Citation2017). However, the spatial resolution of the CCI product (300 m) does not imply that all image cells are pure, and there is uncertainty in identifying features such as small and non-contiguous cultivated land and narrow rivers in the study area. Improving the LUCC data resolution to solve the mixed image element problem is necessary in the future. Although the 2030 Agenda for Sustainable Development provides relevant suggestions for eliminating social inequalities and protecting the ecosystem, it neither explains how to use natural resources equitably nor establishes indicators to measure progress on achieving ecological goals. Currently, provisioning and regulating services are the most studied, and cultural and supporting services are much less represented; however, in spite of this lacuna, more importance is provided for global sustainability policies. Therefore, research on theories and assessment methods must be increased for cultural and supporting services, particularly on methodology-related studies that can develop indicators.
In this study, the integrated assessment framework was used to assess the mismatch and equilibrium phenomena of the supply and demand of ES at multiple scales, and the results are useful to improve the understanding of socio-ecological conflict mechanisms. It is recommended that results of the assessment of supply and demand of ES should be integrated into landscape management and environmental decision-making to reduce the loss of socio-ecological capital in the LP. Data monitoring of socio-ecological systems and nexus assessment of LUCC – ES–SDGs should be the key domains in the future.
Conclusions
Our study assessed ecosystem services and regional implementation of SDGs using an integrated assessment framework. We identified spatiotemporal changes in landscape patterns, matrices, and composite indices of supply and demand of ES in the LP from 1992 to 2015 and analyzed the patterns of change and equilibrium characteristics of supply – demand patterns of ES at the county and regional scales. The potential impacts of LUCC – ES processes on SDGs were also analyzed. We found that land use strategies such as intensive land use and ecological restoration have driven landscape alterations in the LP. The reduction in cultivated land and bare land resulted in an increase in ecological land, indicating that the regional ecological environment has been restored. Although local provisioning and supporting services were generally in a more balanced state, there was a severe imbalance between supply and demand for regulating and cultural services. In addition, corresponding land use strategies and socioeconomic and ecological management measures should be rationally developed and implemented for different supply – demand matching patterns of ES to mitigate environmental deficit and promote sustainable socio-ecological system development. Finally, the LUCC – ES–SDGs nexus is complex, and the SDGs related to ES mainly emphasize ecosystem conservation, sustainable agriculture, and urban development. It is suggested that information on LUCC – ES should be integrated into the monitoring and evaluation programs of SDGs to provide scientific support for decision-making of sustainable development in the LP.
Acknowledgment
The authors acknowledge the editors and anonymous reviewers for their valuable inputs, which undoubtedly led to a substantial improvement in the quality of this article.
Disclosure statement
No potential conflict of interest was reported by the author(s).
Additional information
Funding
References
- Ala-Hulkko, T., O. Kotavaara, J. Alahuhta, and J. Hjort. 2019. “Mapping Supply and Demand of a Provisioning Ecosystem Service Across Europe.” Ecological Indicators 103: 520–14. doi:10.1016/j.ecolind.2019.04.049.
- Arowolo, A. O., X. Deng, O. A. Olatunji, and A. E. Obayelu. 2018. “Assessing Changes in the Value of Ecosystem Services in Response to Land-Use/land-Cover Dynamics in Nigeria.” The Science of the Total Environment 636: 597–609. doi:10.1016/j.scitotenv.2018.04.277.
- Benra, F., and L. Nahuelhual. 2019. “A Trilogy of Inequalities: Land Ownership, Forest Cover and Ecosystem Services Distribution.” Land Use Policy 82: 247–257. doi:10.1016/j.landusepol.2018.12.020.
- Boithias, L., V. Acuña, L. Vergoñós, G. Ziv, R. Marcé, and S. Sabater. 2014. “Assessment of the Water Supply:Demand Ratios in a Mediterranean Basin Under Different Global Change Scenarios and Mitigation Alternatives.” The Science of the Total Environment 470-471: 567–577. doi:10.1016/j.scitotenv.2013.10.003.
- Burkhard, B., F. Kroll, F. Müller, and W. Windhorst. 2009. “Landscapes’ Capacities to Provide Ecosystem Services – a Concept for Land-Cover Based Assessments.” Landscape Online 15: 1–12. doi:10.3097/LO.200915.
- Cai, W. B., D. Gibbs, L. Zhang, G. Ferrier, and Y. L. Cai. 2017. “Identifying Hotspots and Management of Critical Ecosystem Services in Rapidly Urbanizing Yangtze River Delta Region, China.” Journal of Environmental Management 191: 258–267. doi:10.1016/j.jenvman.2017.01.003.
- Cai, J., X. Li, L. Liu, Y. Chen, X. Wang, and S. Lu. 2021. “Coupling and Coordinated Development of New Urbanization and Agro-Ecological Environment in China.” The Science of the Total Environment 776: 145837. doi:10.1016/j.scitotenv.2021.145837.
- Cao, T. G., Y. J. Yi, H. X. Liu, Q. Xu, and Z. F. Yang. 2021. “The Relationship Between Ecosystem Service Supply and Demand in Plain Areas Undergoing Urbanization: A Case Study of China’s Baiyangdian Basin.” Journal of Environmental Management 289: 112492. doi:10.1016/j.jenvman.2021.112492.
- Castillo-Eguskitza, N., B. Martín-López, and M. Onaindia. 2018. “A Comprehensive Assessment of Ecosystem Services: Integrating Supply, Demand and Interest in the Urdaibai Biosphere Reserve.” Ecological Indicators 93: 1176–1189. doi:10.1016/j.ecolind.2018.06.004.
- Chen, Y., Y. Ge, G. Yang, Z. Wu, Y. Du, F. Mao, S. Liu, et al. 2022. “Inequalities of Urban Green Space Area and Ecosystem Services Along Urban Center-Edge Gradients.” Landscape and Urban Planning 217: 217. doi:10.1016/j.landurbplan.2021.104266.
- Chen, Y. P., K. B. Wang, Y. S. Lin, W. Y. Shi, Y. Song, and X. H. He. 2015. “Balancing Green and Grain Trade.” Nature Geoscience 108 (10): 739–741. doi:10.1038/ngeo2544.
- Chinese Academy of Sciences, 2020. Big Earth Data in Support of the Sustainable Development Goals. Accessed November 2020. sdgs.casearth.cn
- Costanza, R., R. de Groot, P. Sutton, S. van der Ploeg, S. J. Anderson, I. Kubiszewski, S. Farber, and R. K. Turner. 2014. “Changes in the Global Value of Ecosystem Services.” Global Environmental Change 26: 152–158. doi:10.1016/j.gloenvcha.2014.04.002.
- Dai, E. F., X. L. Wang, J. J. Zhu, and J. B. Gao. 2015. “Progress and Perspective on Ecosystem Services Trade-Offs.” Advances in Earth Science 30: 1250–1259. doi:10.1109/ISDPE.2010.14.
- Deng, X., Z. Li, and J. Gibson. 2016. “A Review on Trade-Off Analysis of Ecosystem Services for Sustainable Land-Use Management.” Journal of Geographical Sciences 26 (7): 953–968. doi:10.1007/s11442-016-1309-9.
- Feng, X. M., B. J. Fu, S. L. Piao, S. Wang, P. Ciais, Z. Z. Zeng, Y. H. Lü, et al. 2016. “Revegetation in China’s Loess Plateau is Approaching Sustainable Water Resource Limits.” Nature Climate Change 6 (11): 1019–1022. doi:10.1038/nclimate3092.
- Feng, Q., W. W. Zhao, B. J. Fu, J. Y. Ding, and S. Wang. 2017. “Ecosystem Service Trade-Offs and Their Influencing Factors: A Case Study in the Loess Plateau of China.” The Science of the Total Environment 607-608: 1250–1263. doi:10.1016/j.scitotenv.2017.07.079.
- Feng, Q., W. Zhao, X. Hu, Y. Liu, S. Daryanto, and F. Cherubini. 2020. “Trading-Off Ecosystem Services for Better Ecological Restoration: A Case Study in the Loess Plateau of China.” Journal of Cleaner Production 257: 257. doi:10.1016/j.jclepro.2020.120469.
- Feurer, M., H. Rueff, E. Celio, A. Heinimann, J. Blaser, A. M. Htun, and J. G. Zaehringer. 2021. “Regional Scale Mapping of Ecosystem Services Supply, Demand, Flow and Mismatches in Southern Myanmar.” Ecosystem Services 52: 101363. doi:10.1016/j.ecoser.2021.101363.
- Fuchs, R., R. Prestele, and P. H. Verburg. 2018. “A Global Assessment of Gross and Net Land Change Dynamics for Current Conditions and Future Scenarios.” Earth System Dynamics 9 (2): 441–458. doi:10.5194/esd-9-441-2018.
- Fu, B. J., Y. Liu, Y. H. Lu, C. S. He, Y. Zeng, and B. F. Wu. 2011. “Assessing the Soil Erosion Control Service of Ecosystems Change in the Loess Plateau of China.” Ecological Complexity 8: 284–293. doi:10.1016/j.ecocom.2011.07.003.
- Fu, B. J., S. Wang, Y. Liu, J. B. Liu, W. Liang, and C. Y. Miao. 2017. “Hydrogeomorphic Ecosystem Responses to Natural and Anthropogenic Changes in the Loess Plateau of China.” Annual Review of Earth and Planetary Sciences 45: 223–243. doi:10.1146/annurev-earth-063016-020552.
- Geijzendorffer, I. R., E. Cohen-Shacham, A. F. Cord, W. Cramer, C. Guerra, and B. Martin-Lopez. 2017. “Ecosystem Services in Global Sustainability Policies.” Environmental Science & Policy 74: 40–48. doi:10.1016/j.envsci.2017.04.017.
- Geijzendorffer, I. R., B. Martin-Lopez, and P. K. Roche. 2015. “Improving the Identification of Mismatches in Ecosystem Services Assessments.” Ecological Indicators 52: 320–331. doi:10.1016/j.ecolind.2014.12.016.
- Jiang, C., Z. Yang, M. Wen, L. Huang, H. Liu, J. Wang, W. Chen, and C. Zhuang. 2021. “Identifying the Spatial Disparities and Determinants of Ecosystem Service Balance and Their Implications on Land Use Optimization.” The Science of the Total Environment 793: 793. doi:10.1016/j.scitotenv.2021.148472.
- Larondelle, N., and S. Lauf. 2016. “Balancing Demand and Supply of Multiple Urban Ecosystem Services on Different Spatial Scales.” Ecosystem Services 22: 18–31. doi:10.1016/j.ecoser.2016.09.008.
- Lee, B. X., F. Kjaerulf, S. Turner, L. Cohen, P. D. Donnelly, R. Muggah, R. Da Vis, A. Realini, B. Kieselbach, and L. S. Macgregor. 2016. “Transforming Our World: Implementing the 2030 Agenda Through Sustainable Development Goal Indicators.” Journal of Public Health Policy 37: 13–31. doi:10.1057/s41271-016-0002-7.
- Liang, Y., S. Hashimoto, and L. Liu. 2021. “Integrated Assessment of Land-Use/land-Cover Dynamics on Carbon Storage Services in the Loess Plateau of China from 1995 to 2050.” Ecological Indicators 120: 106939. doi:10.1016/j.ecolind.2020.106939.
- Li, W., N. MacBean, P. Ciais, P. Defourny, C. Lamarche, S. Bontemps, R. A. Houghton, and S. Peng. 2018. “Gross and Net Land Cover Changes in the Main Plant Functional Types Derived from the Annual ESA CCI Land Cover Maps (1992–2015).” Earth System Science Data 10 (1): 219–234. doi:10.5194/essd-10-219-2018.
- Linders, T. E. W., K. Bekele, U. Schaffner, E. Allan, T. Alamirew, S. K. Choge, S. Eckert, et al. 2020. “The Impact of Invasive Species on Social-Ecological Systems: Relating Supply and Use of Selected Provisioning Ecosystem Services.” Ecosystem Services 41: 101055. doi:10.1016/j.ecoser.2019.101055.
- Lin, Y., M. Zhang, M. Gan, L. Huang, C. Zhu, Q. Zheng, S. You, et al. 2022. “Fine Identification of the Supply–demand Mismatches and Matches of Urban Green Space Ecosystem Services with a Spatial Filtering Tool.” Journal of Cleaner Production 336: 130404. doi:10.1016/j.jclepro.2022.130404.
- Liu, L., Y. Liang, and S. Hashimoto. 2020. “Integrated Assessment of Land-Use/coverage Changes and Their Impacts on Ecosystem Services in Gansu Province, Northwest China: Implications for Sustainable Development Goals.” Sustainability Science 15 (1): 297–314. doi:10.1007/s11625-019-00758-w.
- Li, W., Y. Wang, S. Xie, and X. Cheng. 2022. “Spatiotemporal Evolution Scenarios and the Coupling Analysis of Ecosystem Health with Land Use Change in Southwest China.” Ecological Engineering 179: 106607. doi:10.1016/j.ecoleng.2022.106607.
- Luiza Petroni, M., J. Siqueira-Gay, and A. Lucia Casteli Figueiredo Gallardo. 2022. “Understanding Land Use Change Impacts on Ecosystem Services Within Urban Protected Areas.” Landscape and Urban Planning 223: 104404. doi:10.1016/j.landurbplan.2022.104404.
- Lü, Y., D. Lü, X. Feng, and B. Fu. 2021. “Multi-Scale Analyses on the Ecosystem Services in the Chinese Loess Plateau and Implications for Dryland Sustainability.” Current Opinion in Environmental Sustainability 48: 1–9. doi:10.1016/j.cosust.2020.08.001.
- Mancini, M. S., A. Galli, L. Coscieme, V. Niccolucci, D. Lin, F. M. Pulselli, S. Bastianoni, and N. Marchettini. 2018. “Exploring Ecosystem Services Assessment Through Ecological Footprint Accounting.” Ecosystem Services 30: 228–235. doi:10.1016/j.ecoser.2018.01.010.
- Marques, M., R. F. Bangash, V. Kumar, R. Sharp, and M. Schuhmacher. 2013. “The Impact of Climate Change on Water Provision Under a Low Flow Regime: A Case Study of the Ecosystems Services in the Francoli River Basin.” Journal of Hazardous Materials 263: 224–232. doi:10.1016/j.jhazmat.2013.07.049.
- Mousivand, A., and J. J. Arsanjani. 2019. “Insights on the Historical and Emerging Global Land Cover Changes: The Case of ESA-CCI-LC Datasets.” Applied Geography 106: 82–92. doi:10.1016/j.apgeog.2019.03.010.
- Othoniel, B., B. Rugani, R. Heijungs, M. Beyer, M. Machwitz, and P. Post. 2019. “An Improved Life Cycle Impact Assessment Principle for Assessing the Impact of Land Use on Ecosystem Services.” The Science of the Total Environment 693. doi:10.1016/j.scitotenv.2019.07.180.
- Peng, K. F., W. G. Jiang, Z. Y. Ling, P. Hou, and Y. W. Deng. 2021. “Evaluating the Potential Impacts of Land Use Changes on Ecosystem Service Value Under Multiple Scenarios in Support of SDG Reporting: A Case Study of the Wuhan Urban Agglomeration.” Journal of Cleaner Production 307: 307. doi:10.1016/j.jclepro.2021.127321.
- Ramel, C., P.-L. Rey, R. Fernandes, C. Vincent, A. R. Cardoso, O. Broennimann, L. Pellissier, et al. 2020. “Integrating Ecosystem Services Within Spatial Biodiversity Conservation Prioritization in the Alps.” Ecosystem Services 45: 101186. doi:10.1016/j.ecoser.2020.101186.
- Schirpke, U., L. Egarter Vigl, E. Tasser, and U. Tappeiner. 2019. “Analyzing Spatial Congruencies and Mismatches Between Supply, Demand and Flow of Ecosystem Services and Sustainable Development.” Sustainability 11 (8): 11. doi:10.3390/su11082227.
- Sun, R., X. Jin, B. Han, X. Liang, X. Zhang, and Y. Zhou. 2022. “Does Scale Matter? Analysis and Measurement of Ecosystem Service Supply and Demand Status Based on Ecological Unit.” Environmental Impact Assessment Review 95: 106785. doi:10.1016/j.eiar.2022.106785.
- Sun, Y. X., S. L. Liu, Y. H. Dong, Y. An, F. N. Shi, S. K. Dong, and G. H. Liu. 2019. “Spatio-Temporal Evolution Scenarios and the Coupling Analysis of Ecosystem Services with Land Use Change in China.” The Science of the Total Environment 681: 211–225. doi:10.1016/j.scitotenv.2019.05.136.
- Sun, X., H. Tang, P. Yang, G. Hu, Z. Liu, and J. Wu. 2020. “Spatiotemporal Patterns and Drivers of Ecosystem Service Supply and Demand Across the Conterminous United States: A Multiscale Analysis.” The Science of the Total Environment 703: 135005. doi:10.1016/j.scitotenv.2019.135005.
- Tang, J., Y. Li, S. Cui, L. Xu, S. Ding, and W. Nie. 2020. “Linking Land-Use Change, Landscape Patterns, and Ecosystem Services in a Coastal Watershed of Southeastern China.” Global Ecology and Conservation 23: e01177. doi:10.1016/j.gecco.2020.e01177.
- Tao, Y., Q. Tao, X. Sun, J. Qiu, S. G. Pueppke, W. Ou, J. Guo, and J. Qi. 2022. “Mapping Ecosystem Service Supply and Demand Dynamics Under Rapid Urban Expansion: A Case Study in the Yangtze River Delta of China.” Ecosystem Services 56: 101448. doi:10.1016/j.ecoser.2022.101448.
- Tao, Y., H. Wang, W. Ou, and J. Guo. 2018. “A Land-Cover-Based Approach to Assessing Ecosystem Services Supply and Demand Dynamics in the Rapidly Urbanizing Yangtze River Delta Region.” Land Use Policy 72: 250–258. doi:10.1016/j.landusepol.2017.12.051.
- Teng, F., J. K. He, X. Z. Pan, and C. Zhang. 2011. “Metric of Carbon Equity: Carbon Gini Index Based on Historical Cumulative Emission per Capita.” Advances in Climate Change Research 2 (3): 134–140. doi:10.3724/sp.J.1248.2011.00134.
- Torres, A. V., C. Tiwari, and S. F. Atkinson. 2021. “Progress in Ecosystem Services Research: A Guide for Scholars and Practitioners.” Ecosystem Services 49: 49. doi:10.1016/j.ecoser.2021.101267.
- United Nations, 2015. Transforming Our World: The 2030 Agenda for Sustainable Development. https://sdgs.un.org
- Vihervaara, P., T. Kumpula, A. Tanskanen, and B. Burkhard. 2010. “Ecosystem Services–a Tool for Sustainable Management of Human–environment Systems. Case Study Finnish Forest Lapland.” Ecological Complexity 7 (3): 410–420. doi:10.1016/j.ecocom.2009.12.002.
- Wang, M. M., and Y. C. Wang. 2021. “Balance or Match?multi-Scenario Analysis and Optimization of Supply and Demand of Park Green Space with Ecological Wisdom.” Chinese Landscape Architecture 37: 37–42. doi:10.19775/j.cla.2021.07.0037.
- Wang, X., J. Wu, Y. Liu, X. Hai, Z. Shanguan, and L. Deng. 2022. “Driving Factors of Ecosystem Services and Their Spatiotemporal Change Assessment Based on Land Use Types in the Loess Plateau.” Journal of Environmental Management 311: 114835. doi:10.1016/j.jenvman.2022.114835.
- Wei, H. J., W. G. Fan, X. C. Wang, N. C. Lu, X. B. Dong, Y. N. Zhao, X. J. Ya, and Y. F. Zhao. 2017. “Integrating Supply and Social Demand in Ecosystem Services Assessment: A Review.” Ecosystem Services 25: 15–27. doi:10.1016/j.ecoser.2017.03.017.
- Wei, Y. P., S. L. Wu, C. Jiang, and X. M. Feng. 2021. “Managing Supply and Demand of Ecosystem Services in Dryland Catchments.” Current Opinion in Environmental Sustainability 48: 10–16. doi:10.1016/j.cosust.2020.08.007.
- Wood, S. L. R., S. K. Jones, J. A. Johnson, K. A. Brauman, R. Chaplin-Kramer, A. Fremier, E. Girvetz, et al. 2018. “Distilling the Role of Ecosystem Services in the Sustainable Development Goals.” Ecosystem Services 29: 70–82. doi:10.1016/j.ecoser.2017.10.010.
- Wu, X., S. L. Liu, S. Zhao, X. Y. Hou, J. W. Xu, S. K. Dong, and G. H. Liu. 2019. “Quantification and Driving Force Analysis of Ecosystem Services Supply, Demand and Balance in China.” The Science of the Total Environment 652: 1375–1386. doi:10.1016/j.scitotenv.2018.10.329.
- Wu, J. S., X. N. Men, J. T. Liang, and Y. H. Zhao. 2020. “Research on Supply and Demand Equilibrium of Ecosystem Services in Guangdong Province Based on the Gini Coefficient.” Acta Ecologica Sinica 40: 6812–6820. doi:10.5846/stxb201903280602.
- Wu, X. T., S. Wang, B. J. Fu, X. M. Feng, and Y. Z. Chen. 2019. “Socio-Ecological Changes on the Loess Plateau of China After Grain to Green Program.” The Science of the Total Environment 678: 565–573. doi:10.1016/j.scitotenv.2019.05.022.
- Xia, F., Y. Yang, S. Zhang, Y. Yang, D. Li, W. Sun, and Y. Xie. 2022. “Influencing Factors of the Supply-Demand Relationships of Carbon Sequestration and Grain Provision in China: Does Land Use Matter the Most?” The Science of the Total Environment 832: 154979. doi:10.1016/j.scitotenv.2022.154979.
- Xu, L., S. Zhao, S. S. Chen, C. Yu, and B. Lei. 2020. “Analysis of Arable Land Distribution Around Human Settlements in the Riparian Area of Lake Tanganyika in Africa.” Applied Geography 125: 102344. doi:10.1016/j.apgeog.2020.102344.
- Yang, M. H., X. D. Gao, X. N. Zhao, and P. T. Wu. 2021. “Scale Effect and Spatially Explicit Drivers of Interactions Between Ecosystem Services—a Case Study from the Loess Plateau.” The Science of the Total Environment 785: 147389. doi:10.1016/j.scitotenv.2021.147389.
- Yang, Y. K., P. F. Xiao, X. Z. Feng, and H. X. Li. 2017. “Accuracy Assessment of Seven Global Land Cover Datasets Over China.” ISPRS Journal of Photogrammetry and Remote Sensing 125: 156–173. doi:10.1016/j.isprsjprs.2017.01.016.
- Yang, S. Q., W. W. Zhao, Y. X. Liu, F. Cherubini, B. J. Fu, and P. Pereira. 2020. “Prioritizing Sustainable Development Goals and Linking Them to Ecosystem Services: A Global Expert’s Knowledge Evaluation.” Geography and Sustainability 1 (4): 321–330. doi:10.1016/j.geosus.2020.09.004.
- Yuan, M.-H., and S.-L. Lo. 2020. “Ecosystem Services and Sustainable Development: Perspectives from the Food-Energy-Water Nexus.” Ecosystem Services 46: 46. doi:10.1016/j.ecoser.2020.101217.
- Yurui, L., Z. Xuanchang, C. Zhi, L. Zhengjia, L. Zhi, and L. Yansui. 2021. “Towards the Progress of Ecological Restoration and Economic Development in China’s Loess Plateau and Strategy for More Sustainable Development.” The Science of the Total Environment 756: 143676. doi:10.1016/j.scitotenv.2020.143676.
- Yu, H., W. Xie, L. Sun, and Y. Wang. 2021. “Identifying the Regional Disparities of Ecosystem Services from a Supply-Demand Perspective.” Resources, Conservation and Recycling 169: 105557. doi:10.1016/j.resconrec.2021.105557.
- Zhang, J., M. Qu, C. Wang, J. Zhao, and Y. Cao. 2020. “Quantifying Landscape Pattern and Ecosystem Service Value Changes: A Case Study at the County Level in the Chinese Loess Plateau.” Global Ecology and Conservation 23: e01110. doi:10.1016/j.gecco.2020.e01110.
- Zhong, H., Z. Liu, and J. Wang. 2022. “Understanding impacts of cropland pattern dynamics on grain production in China: A integrated analysis by fusing statistical data and satellite-observed data.” Journal of Environmental Management 313: 114988. doi:10.1016/j.jenvman.2022.114988.