ABSTRACT
The combination of low temperatures, oligotrophic conditions, high altitude, and varying salinity in Tibetan Plateau lakes endows them with a wealth of distinctive fungal resources. This study aimed to investigate the diversity of culturable fungi in sediment and water samples collected from six lakes located on the Tibetan Plateau at elevations above 4,500 m. A total of 843 fungal strains were isolated by dilution plate method using four different media. Initial ITS analyses revealed that they belong to 156 species across 83 genera, spanning 50 families, 26 orders, 12 classes, and 6 phyla. Further morphological and multi-locus phylogenetic analyses were conducted, resulting in the formal descriptions of a new genus, Xizangia, and seven new species, including Alternaria xizangensis, Emericellopsis ovoidea, Myceliophthora xizangica, Preussia cylindricalis, Preussia sedimenticola, Pseudeurotium sedimenticola, and Xizangia sedimenticola. This research provides insights into the biodiversity of culturable fungi in the unique and extreme ecosystem, shedding light on the potential discovery of novel species and genera.
1. Introduction
The Qinghai-Tibetan Plateau (QTP) is the largest and highest (average 4,500 m above sea level) plateau on the Earth. It contains thousands of saline/hypersaline lakes, which possess a broad range of environmental gradients such as salinity (from 0.1 to 426.3 g/L) and pH (5.4–10.2) (Zheng Citation1997; Yang et al. Citation2016). Characterised by low temperature, low oxygen, high altitude, and intense UV radiation, lakes on the Tibetan Plateau serve as important repositories of biodiversity, providing insights into the evolutionary history and ecological dynamics of aquatic ecosystems. These extreme and unique ecosystems often harbour undiscovered microbial diversity with the potential to reveal novel species.
To date, investigations into the microbial diversity of Xizang’s lakes have predominantly employed culture-independent methods (e.g. DNA sequencing) (Huang et al. Citation2014; Yang et al. Citation2016; Liu et al. Citation2021; Zhu et al. Citation2023) and focused on topics such as seasonal changes in microbial species composition (Liu et al. Citation2013), the metabolic potential of communities in the carbon and nitrogen cycle (Wei et al. Citation2023), and mechanisms for coping with extremes (Li et al. Citation2020). However, few studies have been performed on the species composition and diversity of culturable fungi in Tibetan Plateau lakes. An examination of culturable filamentous fungi in Nam Co water revealed that the most frequently isolated genera were Lecanicillium, Mucor, and Penicillium, with Alternaria chlamydosporigena and Mucor hiemalis identified as the dominant species (Xue et al. Citation2022a). Notably, these fungi are commonly found in the natural environment. In addition, there has been a paucity of research on the diversity of culturable microbes in the sediments of Xizang’s lakes, with particularly limited investigation into the diversity of fungal taxa.
Impacted by the development of high-throughput sequencing technologies, recent years have increasingly favoured the application of these molecular techniques instead of traditional culture methods for microbial resources and diversity studies (Amend et al. Citation2019). Nonetheless, the isolation of strains remains crucial for understanding microorganisms, serving as the foundational material for subsequent investigations into microbial physiology, genetic evolution, as well as exploitation (Richards et al. Citation2012). This manuscript delves into the fungal diversity of culturable isolates derived from Xizang’s lakes.
2. Material and methods
2.1. Sampling collection and isolation
Samples of water and sediment were collected from six lakes located on the Tibetan Plateau, each with varying salinity levels: Angda’er Co (32.58 g/L), Dagze Co (15.93 g/L), Gomang Co (7.66 g/L), Gyaring Co (0.22 g/L), Nam Co (1.12 g/L) and Siling Co (8.12 g/L). Three sediment samples of different depths from each lake were collected using Grab’s mud collector and then placed in sterile self-sealing bags. Two water samples from the surface and bottom of each lake were collected using the microporous membrane filtration method by filtering 500 mL of water samples through a 0.45-μm polyester fibre microporous membrane (47 mm, Millipore). All the sediment and water samples were transported to the laboratory at 4 °C for fungal isolation.
Fungi were isolated from sediments using either the dilution plate method (Zhang et al. Citation2015) or by directly coating sediment particles onto media plates. For the dilution plate method, one gram of each sediment sample was suspended in 9 mL of sterile water in a 15-mL sterile centrifuge tube. The tubes were shaken using a vortex oscillator. The suspension was then diluted to a series of concentrations, i.e. 10−1, 10−2, 10−3, and 10−4. One hundred microlitres of suspensions from each concentration were spread onto 1/100 PDA (Potato Dextrose Agar), 1/100 PmTG (Peptonised milk-Tryptone-Glucose, 1 g peptonised milk, 1 g tryptone, 5 g glucose, 10 g agar, 1 L H2O), 1/100 CMA (Corn Meal Agar) and 1/100 CDA (Czapek Dox Agar) containing ampicillin (50 µg/mL) and streptomycin (50 µg/mL) along with the corresponding salinity of sea salt with three replicates. For aqueous fungi, the 0.45-μm filter membranes of the aqueous samples were first vortexed with 2 mL of sterile water by oscillation, and then the suspension, as well as dilutions to 10−1 and 10−2, were spread onto 1/100 PDA. All the plates were incubated at 15 °C for 3–4 weeks, from which the single colonies were picked up and inoculated onto new PDA plates every two days. All fungal strains were stored at 4 °C for subsequent studies.
2.2. Molecular analyses
Genomic DNA was extracted from fresh fungal mycelium grown for 7 d on PDA, following the Cetyltrimethylammonium bromide (CTAB) method (Guo et al. Citation2000). The internal transcribed spacer regions and intervening 5.8S nrRNA gene (ITS), the large subunit (LSU) rDNA, the small subunit (SSU) rDNA, the translation elongation factor 1-alpha (tef-1α), RNA polymerase II subunit (rpb2), and β-tubulin (tub2) regions were amplified using primer pairs ITS1/ITS4 (White et al. Citation1990), LR0R/LR5 (Vilgalys and Hester Citation1990), NS1/NS4 (White et al. Citation1990), 983F/2218 R (Rehner and Buckley Citation2005), RPB2-5F2/fRPB2-7cR (Liu et al. Citation1999; Sung et al. Citation2007), and Bt2a/Bt2b (Glass and Donaldson Citation1995), respectively. Amplification reactions were performed in a 25 μL reaction volume including 2.5 μL 10× PCR Buffer (Vazyme, Nanjing, China), 2 mmol/L MgCl2, 50 μmol/L dNTPs, 0.1 μmol/L of each forward and reverse primer, 0.5 U Taq DNA polymerase and 1 ng genomic DNA. PCR parameters were as follows: 94 °C for 10 min, followed by 35 cycles of 94 °C for 30 s, 54 °C for 30 s, 72 °C for 30 s, and a final elongation step at 72 °C for 10 min. The annealing temperature for each gene was 52 °C for LSU, SSU, ITS, and 57 °C for rpb2, tef-1α, and tub2. Sequencing reactions were performed by SinoGenoMax (Beijing, China). All ITS sequences of the obtained strains were subjected to BLASTn searches using UNITE and GenBank databases and assigned to possible genera and species. Furthermore, the ITS phylogenetic tree of all strains was constructed to show their isolation origin and taxonomic status as a whole.
To clarify the taxonomic status of potential new taxonomic units of interest to us in this study, phylogenetic analyses were performed based on ITS, LSU, and genetic markers recommended in recent publications, such as tef1-α, rpb2, and tub2. All the information on reference strains is shown in Tables S2–S7. All sequences of different loci were aligned using MAFFT (http://www.ebi.ac.uk/Tools/msa/mafft/) (Katoh and Toh Citation2010) and edited manually using MEGA v. 7.0 (Kumar et al. Citation2016) separately. Individual alignments were then concatenated and used for phylogenetic analysis. Maximum Likelihood (ML) and Bayesian inference (BI) methods were used to construct the phylogenetic trees. The ML analyses were implemented using RAxML-HPC Blackbox v. 8.2.12 (Stamatakis Citation2014) with 1,000 replicates under the GTR-GAMMA model. The robustness of branches was assessed by bootstrap analysis with 1,000 replicates. For Bayesian analysis, the best model of evolution was estimated using MrModeltest v. 2.4 (Guindon et al. Citation2003). Posterior probabilities (PP) (Rannala and Yang Citation1996; Zhaxybayeva and Gogarten Citation2002) were calculated by Markov Chain Monte Carlo sampling (MCMC) in MrBayes on XSEDE v. 3.2.7a (Huelsenbeck and Ronquist Citation2001), using the estimated evolutionary models. Six simultaneous Markov chains were run for 10,000,000 generations, and trees were sampled every 1,000th generation. The first 2,000 trees, representing the burn-in phase of the analyses, were discarded and the remaining 8,000 trees were used to calculate posterior probabilities (PP) in the majority rule consensus tree. The final trees were visualised in FigTree v 1.4.0. Descriptions and illustrations of taxonomic novelties were deposited in Fungal Names (Wang et al. Citation2023).
2.3. Morphological observation
Strains of potentially new species were transferred to new plates of PDA, OA (Oatmeal Agar), and SNA (Synthetic Nutrient-poor Agar) and were incubated for at least 7 d at 15 °C. Cultures were examined periodically for the development of reproductive structures. Morphological characteristics were examined and photo-documented using a Nikon 80i microscope with differential interference contrast. At least 30 measurements for each structure were made according to methods described by Liu et al. (Citation2012). The dry cultures were deposited in the Fungarium of the Institute of Microbiology, Chinese Academy of Sciences, Beijing, China (HMAS), while living cultures were deposited in the China General Microbiological Culture Collection Center (CGMCC) and LC Culture Collection (personal culture collection held in the Lei Cai’s lab).
3. Results
In this study, a total of 843 fungal strains were isolated and assigned to 6 phyla, 12 classes, 26 orders, 50 families, 83 genera, and 156 species based on the primary analyses by employing a BLASTn search in UNITE or GenBank using ITS sequences (, Table S1). Furthermore, ITS sequence similarity comparisons identified 26 species with less than 98% similarity to sequences in NCBI, and 60 species with less than 99% similarity (see Table S1 and ), indicating their potential novelty.
Figure 1. Culturable fungi in six Tibetan Plateau lakes. (a) Phylogenetic tree of all strains obtained in six lakes based on ITS sequences. (b) The number of fungal genera, species, and strains in different phyla obtained in this study. (c) Number of strains of the top 20 genera with the highest frequency of isolation and their corresponding number of species. (d) The number of species with less than 98% and 99% ITS sequence similarity to known species in the NCBI database.
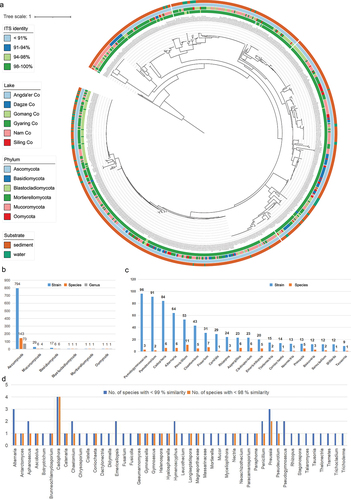
The top 10 most frequently segregated genera were, in order, Pseudogymnoascus, Pseudeurotium, Cadophora, Alternaria, Penicillium, Chaetomium, Fusarium, Candida, Rhizopus, and Aspergillus (Table S1, ). The 10 genera with the highest number of species obtained, in descending order, were Penicillium (11), Fusarium (7), Cadophora (6), Alternaria (6), Aspergillus (6), Cladosporium (6), Chaetomium (5), Emericellopsis (5), Preussia (5), and Pseudogymnoascus (3) (). The top 10 most frequently isolated species were, in order, Pseudogymnoascus roseus (ITS Iden. 99.1%), Cadophora constrictospora (96.5%), Pseudeurotium hygrophilum (99.3%), Alternaria chlamydosporigena (99.4%), Chaetomium globosum (100%), Pseudeurotium desertorum (98.4%), Candida vartiovaarae (100%), Penicillium citrinum (100%), Pseudogymnoascus appendiculatus (98%), and Rhizopus arrhizus (99.7%) (Table S1).
Based on multi-locus phylogenetic analyses and morphological characterisation, a new genus and seven new species were officially described and illustrated in this study, which belonged to Alternaria, Emericellopsis, Myceliophthora, Preussia, Pseudeurotium, and Xizangia.
Taxonomy
Alternaria xizangensis F. Liu, S. Song & L. Cai, sp. nov.,
Figure 2. Alternaria xizangensis (from ex-holotype CGMCC 3.27272). (a–b) Surface and reverse of the colony on PDA. (c–d) Surface and reverse of the colony on OA. (e–f) Surface and reverse of the colony on MEA. (g–j) Conidiophores and conidia. Scale bars: g–j = 10 µm.
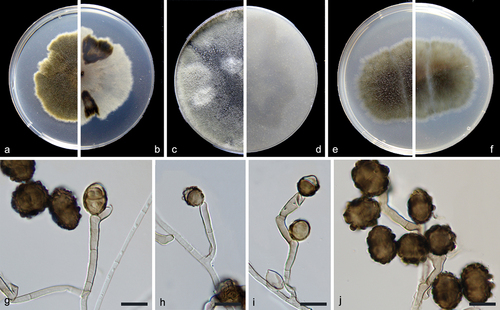
Fungal name: FN571892.
Etymology: Named after the place where the fungus was collected, Xizang.
Description: Conidiophores solitary or clustered, geniculate, frequently proliferating sympodially, pale brown to brown, 9–42 × 2–4 µm. Conidia commonly solitary or several in a chain, varied from subsphaeroid, obovoid, obclavate to ellipsoid, brown to dark brown, verrucose, 9–18 × 7–17 µm (av. ± SD: 14.1 ± 2.1 × 12.2 ± 2.4 µm), mature conidia with 0–1 transverse and 0–1 longitudinal septa.
Material examined: China, Xizang, Siling Co, N 31.7030, E 88.7354, from lake sediment, Aug. 2021, J.E. Huang, HMAS 352877 (holotype), ex-holotype living culture CGMCC 3.27272 = LC19458 = S2.
Notes: A BLASTn search in NCBI using the ITS sequence of the type strain of Alternaria xizangensis indicated its close relationship with species in the Alternaria section Ulocladioides, with the species showing the highest similarity being A. terricola (96%). Multi-locus phylogenetic analysis revealed that A. xizangensis is closely related to A. atra and A. multiformis (), but differs from both by 21 bp in the ITS sequences. Morphologically, A. xizangensis differs from A. atra in the number of transepta (0–1 vs. 1–3) and longisepta (0–1 vs. 1–2) in conidia, and it differs from A. multiformis in producing smaller conidia (9–18 × 7–17 µm vs. 22–24 × 13–18 µm) (Simmons Citation1998).
Figure 3. Maximum likelihood (ML) tree of Alternaria sect. Ulocladioides based on ITS, LSU, SSU, gapdh, rpb2, and tef1-α sequences. The tree is rooted with A. alternariae (CBS 126989). The tree topology of the ML analysis was similar to the BI. ML bootstrap values (≥50%) and Bayesian posterior probability (≥0.9) are indicated along branches (ML/PP). Novel species are in bold font and “T” indicates type derived sequences.
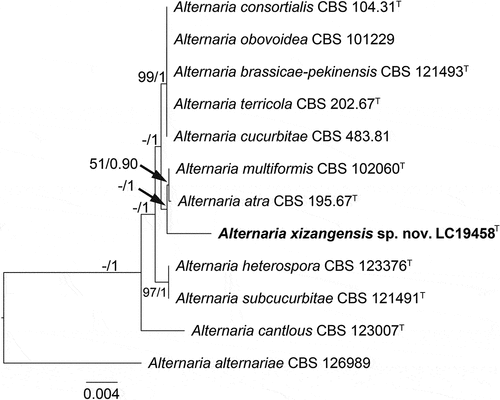
Emericellopsis ovoidea F. Liu, S. Song & L. Cai, sp. nov.,
Figure 4. Emericellopsis ovoidea (from ex-holotype CGMCC 3.27273). (a–b) Surface and reverse of the colony on PDA. (c–d) Surface and reverse of the colony on OA. (e–f) Surface and reverse of the colony on MEA. (g–j) Conidiogenous cells and conidia. (k) Conidia. Scale bars: g–k = 10 µm.
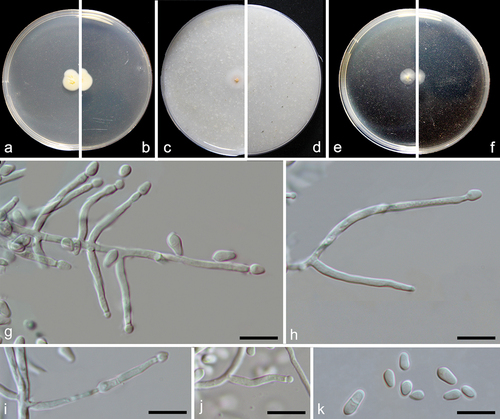
Fungal name: FN571893.
Etymology: Referring to the obovate conidia.
Description: Mycelium consists of branched, septate, hyaline, smooth, and thin-walled hyphae up to 2 μm wide. Conidiophores are produced by immersed or superficial hyphae, erect or slightly curved, single or branched, 2–2.5 μm wide at base, hyaline, smooth wall. Conidiogenous cells erect, cylindrical, 11–20 × 1–2 μm (av. ± SD: 16.3 ± 2.9 × 1.9 ± 0.3 µm). Conidia obovate, sometimes ellipsoidal, aseptate, rarely 1-spetum, hyaline, smooth wall, 3.5–7.5 × 2–3.5 μm (av. ± SD: 5.0 ± 0.9 × 2.7 ± 0.3 µm).
Materials examined: China, Xizang, Siling Co, N 31.8011, E 88.7288, from lake sediment, Aug. 2021, J.E. Huang, HMAS 352878 (holotype), ex-type living culture CGMCC 3.27273 = LC19461 = S58; ibid. LC19490 = SLC1018, LC19498 = SLC2007, LC19504 = SLC2016.
Notes: Based on the blastn searches using GenBank and UNITE databases, the species with the highest similarity to Emericellopsis ovoidea are E. pallida, E. maritima (99.41%), and E. maritima (98.52%), respectively. Through the multi-locus analysis, we noticed that E. ovoidea represents a distinct clade and has a close relationship with E. alkalina, E. pallida, E. minima, and E. maritima (). Morphologically, E. ovoidea differs from E. maritima in producing relatively smaller conidia (3.5–7.5 × 2–3.5 μm vs. 6.5–9 × 2–4 μm) (Belyakova Citation1970), and from E. alkalina and E. minima in the length of conidiogenous cells (11–20 μm in E. ovoidea vs. 20–35 μm in E. alkalina, 20–35 μm in E. minima) (Stolk Citation1955; Grum-Grzhimaylo et al. Citation2013).
Figure 5. Maximum likelihood (ML) tree of Emericellopsis and allied genera based on ITS, LSU, rpb2, and tef1-α sequences. The tree is rooted with Stanjemonium ochroroseum (CBS 656.79). Tree topology of the ML analysis was similar to the BI. ML bootstrap values (≥50%) and Bayesian posterior probability (≥0.9) are indicated along branches (ML/PP). Novel species are in bold font and “T” indicates type derived sequences.
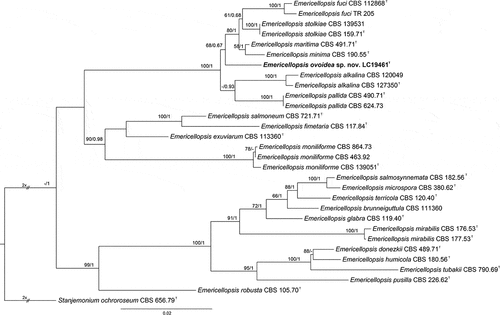
Myceliophthora xizangica F. Liu, S. Song & L. Cai, sp. nov.,
Figure 6. Myceliophthora xizangica (from ex-holotype CGMCC 3.27275). (a–c) Surface of colonies on PDA, OA, and MEA, respectively. (d–g) Conidiophores and conidia. (h) Conidia. Scale bars: d–h = 10 µm.
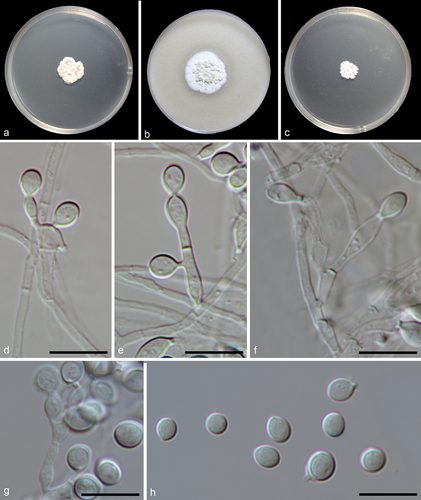
Fungal name: FN571894.
Etymology: Named after the place where the fungus was collected, Xizang.
Description: Vegetative hyphae hyaline, smooth, branched, septate, about 1 μm wide. Conidiophores hayline, branched, septate, 1–28.5 × 1–3 µm, sometimes reduced to conidiogenous cells. Conidiogenous cells originating laterally or terminally from hyphae or conidiophores, subcylindrical, pyriform, subglobose or ampulliform, 1–8.4 × 1.5–5.5 µm. Conidia solitary, or in short chains, aseptate, smooth, hyaline, globose, subglobose, ovoid, or ellipsoidal, with a truncate base, 3.5–5.5 × 3–4 µm (av. ± SD: 4.6 ± 0.6 × 3.7 ± 0.2 µm).
Materials examined: China, Xizang, Gomang Co, N 31.1388, E 89.1987, 4,629 m, from lake sediment, Aug. 2021, J.E. Huang, HMAS 352879 (holotype), ex-type living culture CGMCC 3.27275 = LC20320 = G117; Siling Co, N 31.703, E 88.7354, 4,541.58 m, from lake sediment, Aug. 2021, J.E. Huang, living cultures LC20379 = SLCS3, LC20380 = GMC63, LC20381 = GMC64.
Notes: Myceliophthora xizangica is closely related to M. lutea (), but shares low sequence similarity with the latter at ITS (98%, 443/448), rpb2 (94.66%, 443/468), and tef1-α (93.27%, 679/728). Morphologically, it differs from M. lutea in producing branched conidiophores and subcylindrical conidiogenous cells (Wang et al. Citation2022).
Figure 7. Maximum likelihood (ML) tree of Myceliophthora and allied genera based on ITS, rpb2, and tef1-α sequences. The tree is rooted with Allobotryotrichum blastospora (LC11913). Tree topology of the ML analysis was similar to the BI. ML bootstrap values (≥50%) and Bayesian posterior probability (≥0.9) are indicated along branches (ML/PP). Novel species are in bold font and “T” indicates type derived sequences.
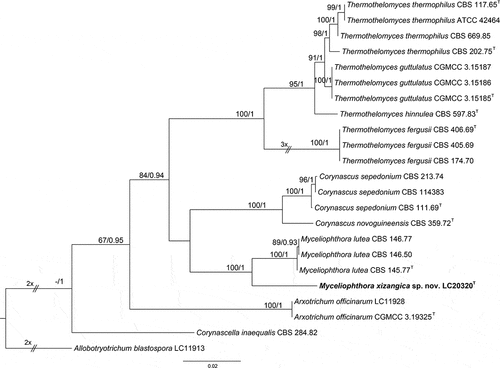
Preussia cylindricalis F. Liu, S. Song & L. Cai, sp. nov.,
Figure 8. Preussia cylindricalis (from ex-holotype CGMCC 3.27271). (a–b) Surface and reverse of the colony on PDA. (c–d) Surface and reverse of the colony on OA. (e–f) Surface and reverse of the colony on MEA. (g) Pycnidium. (h–j) Conidiogenous cells and conidia. (k) Conidia. Scale bars: g = 25 µm; h–k = 10 µm.
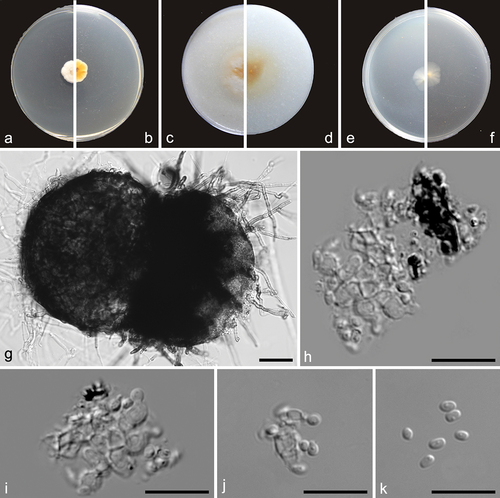
Fungal name: FN571895.
Etymology: Referring to the cylindrical conidia.
Description: Conidiomata pycnidial, black, spherical, scattered or clustered, 117–173 μm diam. Conidiophores reduced to conidiogenous cells lining the inner cavity, hyaline, smooth, phialidic, 2.5–4 × 1.5–3.5 μm (av. ± SD: 3.6 ± 0.5 × 2.79 ± 0.7 µm). Conidia ellipsoid with obtuse ends, hyaline, aseptate, smooth, 1-guttulate, 1.5–2.5 × 1–1.5 μm (av. ± SD: 2.2 ± 0.2 × 1.4 ± 0.1 µm).
Material examined: China, Xizang, Nam Co, N 30.8693, E 91.0386, from lake sediment, Aug. 2021, J.E. Huang, HMAS 352880 (holotype), ex-type living culture CGMCC 3.27271 = LC19384 = NMC1013.
Notes: Although represented by a single strain, Preussia cylindricalis is phylogenetically distinct from all currently accepted species of this genus (). It has a low ITS sequence similarity of 96.77% (510/527) with its closest relative P. typharum. Considering that the asexual morph of P. typharum has not been observed, we could not make a morphological comparison between the two species in this study.
Figure 9. Maximum likelihood (ML) tree of Preussia and allied genera based on ITS and LSU sequences. The tree is rooted with Westerdykella angulata (CBS 610.74). Tree topology of the ML analysis was similar to the BI. ML bootstrap values (≥50%) and Bayesian posterior probability (≥0.9) are indicated along branches (ML/PP). Novel species are in bold font and “T” indicates type derived sequences.
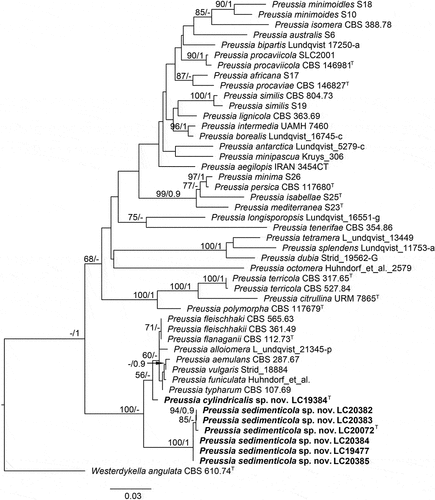
Preussia sedimenticola F. Liu, S. Song & L. Cai, sp. nov.,
Figure 10. Preussia sedimenticola (from ex-holotype CGMCC 3.27276). (a–b) Surface and reverse colony on PDA. (c–g) Conidiogenous cells and conidia. (h) Conidia. Scale bars: c–h = 10 µm.
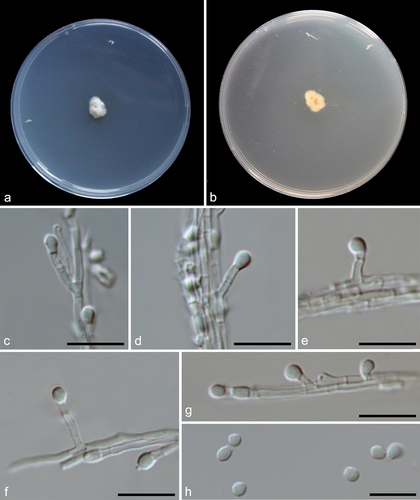
Fungal name: FN571896.
Etymology: Referring to the substrate where the fungus was isolated.
Description: Conidiophores hyaline, branched, or reduced to conidiogenous cells. Conidiogenous cells originating laterally or terminally from hyphae or conidiophores, cylindrical, hyaline, 2–14.5 × 1–2 μm. Conidia hyaline, aseptate, ovoid with truncate base, globose, ellipsoid, solitary or rarely in chains, 2.5–4.5 × 2–3 μm (av. ± SD: 3.5 ± 0.4 × 2.8 ± 0.3 µm).
Materials examined: China, Xizang, Dagze Co, N 31.8237, E 87.4713, from lake sediment, Aug. 2021, J.E. Huang, HMAS 352881 (holotype), ex-type living culture CGMCC 3.27276 = LC20072 = DZC1014; ibid. LC20382 = DZC1035, LC20383 = DZC1043; Siling Co, N 31.8011, E 88.7288, from lake sediment, Aug. 2021, J.E. Huang, living cultures LC19477 = SLC1001, LC20384 = SLC1002, LC20385 = SLC2003.
Notes: Six strains of Preussia sedimenticola formed a distinct clade on the LSU-ITS phylogenetic tree (). Although P. sedimenticola is most closely related to P. cylindricalis, and both were isolated from lakes on the Tibetan Plateau, the two species exhibit distinct morphological differences. The conidiogenous cells of P. sedimenticola mostly form from hyphae and are cylindrical, whereas those of P. cylindricalis are formed in pycnidia and are phialidic in shape. Additionally, P. sedimenticola produces larger conidia than P. cylindricalis (2.5–4.5 × 2–3 μm vs. 1.5–2.5 × 1–1.5 μm).
Pseudeurotium desertorum Mouch., Revue Mycol., Paris 36(2): 123. 1971.
Figure 11. Pseudeurotium desertorum (a, d–g from LC19441; b–c, h–k from LC19712). (a–c) Colonies on PDA, OA, and MEA. (d–f) Conidiogenous cells and conidia. (g) Conidia. (h–j) Asci and ascospores. (k) Ascospores. Scale bars: d–k = 10 µm.
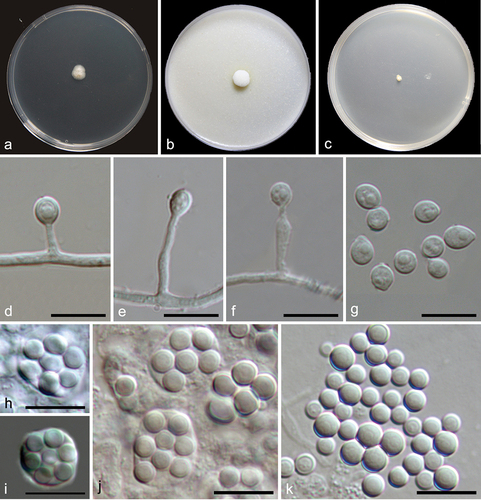
Description: Hyphae hyaline, septate, branched, smooth, 1.5 μm wide. Asci spherical or nearly spherical, pyriform, 8-ascospore, thin-walled, smooth, 8–14 × 6–10 μm. Ascospores globose, hyaline to pale brown, aseptate, smooth, 3–4.5 × 3–4 μm (av. ± SD: 3.42 ± 0.33 × 3.35 ± 0.23 µm). Conidiophores reduced to conidiogenous cells, originating from hyphae, hyaline, and aseptate. Conidiogenou cells cylindrical, clavate, obclavate, straight or curved, 1–15 × 1–2 μm. Conidia aseptate, globose, subglobose to broadly ellipsoidal, 4–7 × 3.5–4.5 μm (av. ± SD: 4.97 ± 0.6 × 4.08 ± 0.27 µm).
Materials examined: China, Xizang, Angda’er Co, N 32.6684, E 89.5275, from lake sediment, Aug. 2021, J.E. Huang, living cultures LC19712 = ADECS193, LC19832 = ADECS495, LC19715 = ADECS198; Nam Co, N 30.8693, E 91.0386, from lake sediment, Aug. 2021, J.E. Huang, living culture LC19441 = NMC4005; Siling Co, N 31.703, E 88.7354, from lake sediment, Aug. 2021, J.E. Huang, living culture LC19502 = SLC2013.
Notes: Many Pseudeurotium desertorum strains were isolated from sediments of lakes on the Tibetan Plateau in this study. In terms of phylogenetic tree topology, intraspecific variation was observed in the species (). However, as the sequences of these isolates obtained in this study differed from the type strain of P. desertorum by only 2–4 base pairs, we identified all these strains as P. desertorum and updated their morphological description herein.
Figure 12. Maximum likelihood (ML) tree of Pseudeurotium based on ITS sequences. The tree topology of the ML analysis was similar to the BI. ML bootstrap values (≥50%) and Bayesian posterior probability (≥0.9) are indicated along branches (ML/PP). “T” indicates type-derived sequences.
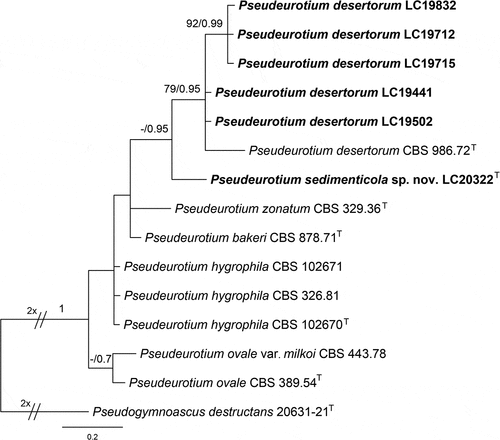
Pseudeurotium sedimenticola F. Liu, S. Song & L. Cai, sp. nov.,
Figure 13. Pseudeurotium sedimenticola (from holotype HMAS 352882). (a–b) Surface and reverse of the colony on PDA. (c–h) Conidiophore and conidia. Scale bars: c–h = 10 µm.
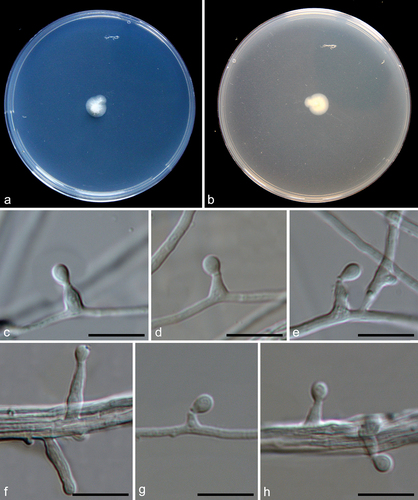
Fungal name: FN571897.
Etymology: Referring to the substrate where the fungus was isolated.
Description: Conidiophores reduced to conidiogenous cells, originating laterally or terminally from hyphae. Terminal conidiogenous cells lageniform, often bent or sinuous, 3–12 µm; intercalary conidiogenous cells similar to terminal cells or ampuliform, or reduced to small denticles on intercalary cells. Conidia aseptate, hyaline, subglobose or obovoid, 3–4 × 2–3 µm (av. ± SD: 3.4 ± 0.4 × 2.6 ± 0.2 µm).
Material examined: China, Xizang, Angda’er Co, N 32.6801, E 89.5327, from lake sediment, Aug. 2021, J.E. Huang, HMAS 352882 (holotype).
Notes: Although represented by single strain, P. sedimenticola forms a distinct clade on the ITS tree () and has 98.6% ITS sequence similarity to the closely related species P. desertorum. Morphologically, P. sedimenticola differs from P. desertorum in producing smaller conidia (3–4 × 2–3 µm vs. 4–7 × 3.5–4.5 μm).
Xizangia F. Liu & L. Cai, gen. nov.
Fungal name: FN571890.
Etymology: Named after the place where we collected the ex-type culture, Xizang.
Description: Conidiogenous cells arising laterally from aerial hyphae, hyaline, phialidic or cylindrical, aseptate or septate. Conidia hyaline, smooth, aseptate, globose or ovoid, guttulate.
Type species: Xizangia sedimenticola F. Liu, S. Song & L. Cai, sp. nov.
Xizangia sedimenticola F. Liu, S. Song & L. Cai, sp. nov.,
Figure 14. Xizangia sedimenticola (from ex-holotype CGMCC 3.27274). (a) Surface of the colony on OA. (b–d) Conidiophores and conidia. (e–g) Conidia. Scale bars: b–g = 10 µm.
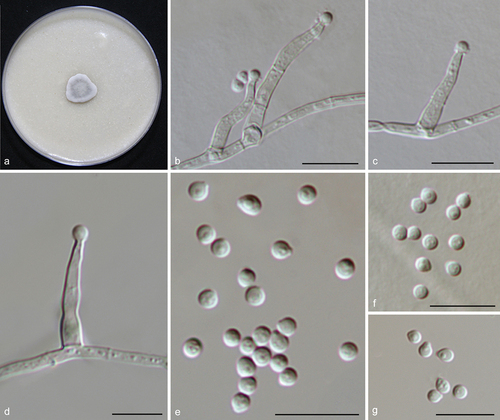
Fungal name: FN571898.
Etymology: Referring to the substrate where the fungus was collected, sediment.
Description: Hyphae smooth, hyaline, septate, branched, 1–1.5 μm wide. Conidiophores arising laterally from aerial hyphae, solitary, erect or curved, hyaline, reduced to conidiogenous cells, 0–2-septa, 8–42 × 1–3 μm. Conidia hyaline, aseptate, smooth, solitary, globose or ovoid, 1–3 oil droplets, 2–2.5 μm diam.
Material examined: China, Xizang, Siling Co, N 31.8011, E 88.7288, from lake sediment, Aug. 2021, J.E. Huang, HMAS 352883 (holotype), ex-type living culture CGMCC 3.27274 = LC19528 = SLCS12.
Notes: Based on the BLASTn searches of NCBI GenBank nucleotide database, the closest hits using the LSU+ITS, rpb2 and tub2 sequences of Xizangia sedimenticola are species in Chaetomiaceae such as Chaetomium spp., Stolonocarpus spp., and Botryoderma spp. (<90%); Stellatospora spp., Staphylotrichum spp., and Madurella spp. (<78%); Acrophialophora spp., Myceliophthora spp., and Colleriella spp. (<80%), respectively. But it is phylogenetically and morphologically distinct from these genera. Based on our phylogenetic analysis, the monotypic species X. sedimenticola is located in the polyphyletic Lasiosphaeriaceae and is distinct from other genera ().
Figure 15. Phylogenetic tree resulting from maximum likelihood (ML) analysis of the concatenated partial rpb2, tub2, ITS, and LSU gene region alignment. Tree topology of the ML analysis was similar to the BI. ML bootstrap values (≥50%) and Bayesian posterior probability (≥0.9) are indicated along branches (ML/PP). Type strains are marked with “T” (type), “ET” (epitype) or “NT” (neotype) after the culture number. The scale bar shows the expected number of changes per site.
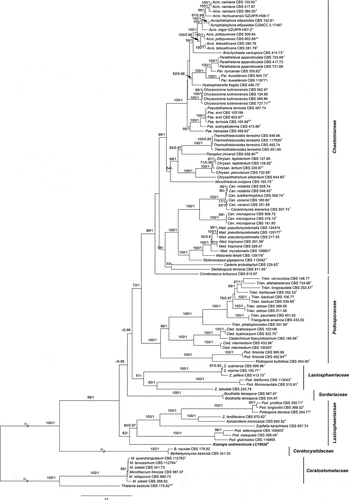
4. Discussion
The lakes on the Tibetan Plateau are characterised by low temperatures, which provide a suitable environment for psychrophilic and psychrotrophic fungi. Both psychrophilic and psychrotrophic fungi demonstrate the capability to thrive at 0 °C. Psychrophilic fungi exhibit an optimal growth temperature of approximately 15 °C or lower, with a maximum growth temperature of 20 °C or below. In contrast, psychrotrophic fungi have a maximum growth temperature that exceeds 20 °C (Morita Citation1975; Gounot Citation1986). To our knowledge, the diversity of cold-adapted fungi in lake sediments has been poorly studied. In an investigation of fungi obtained from soil and lake sediment in the Skarvsnes ice-free area, Antarctica, the cold-adapted fungi were classified into five genera of Ascomycota (Embellisia, Phoma, Geomyces, Tetracladium, Thelebolus) and five genera of Basidiomycota (Mrakia, Cryptococcus, Dioszegia, Rhodotorula, Leucosporidium) (Tsuji et al. Citation2013). Interestingly, our study only obtained one species of Thelebolus from the above taxa, indicating a high degree of variability in fungal species found in different lake sediments.
Until now, research on culturable fungi in Xizang’s lakes has been very limited, with most studies focusing on fungal diversity in Nam Co. The dominant fungal taxa isolated from water and sediments of Nam Co in our study differed from those previously reported by Xue et al. (Citation2022a, Citation2022b). In Xue et al. (Citation2022a, Citation2022b), the genera with the highest frequency of isolation from Nam Co water were Penicillium, Lecanicillium, Mucor, Alternaria, Fusarium, and Cladosporium. However, in our study, in terms of both the number of strains isolated and the frequency of taxa isolation, the prevailing fungi encompass not only ubiquitous environmental genera like Alternaria, Penicillium, and Aspergillus, but also taxa frequently isolated and documented in cold environments, such as glaciers, mangroves, oceans, or the Antarctic, exemplified by Cadophora and Pseudeurotium (Tsuji et al. Citation2013; Zhang et al. Citation2022). This finding suggests that the culturable fungal diversity in Nam Co water is more diverse than previously thought.
It is well known that increased glacier ablation due to global warming and large inputs of glacier meltwater have altered the salinity and nutrients in the Tibetan Plateau lakes (Milner et al. Citation2009), simultaneously, microorganisms enter the lakes with the glacial meltwater. Thus, we suspect that some microorganisms in the lakes are of glacial origin. Combining the results of this study with the literature review, we find that Cadophora is one of the most common genera found in both marine glaciers (Zhang et al. Citation2022) and lakes (Table S1, ) in the Qinghai-Tibetan Plateau. To our knowledge, this genus has been consistently isolated as plant pathogens or root colonisers from northern temperate regions or as decomposers from the cold Arctic and Antarctic locations (Blanchette et al. Citation2004, Citation2010, Citation2016; Gonçalves et al. Citation2012; Furbino et al. Citation2014; Nagano et al. Citation2017; Durán et al. Citation2019), and it possesses a notably elevated number of genes associated with stress tolerance and resource uptake (Egidi et al. Citation2019). In the present study, six Cadophora species were isolated and identified. Remarkably, four of these species exhibited less than 98% sequence similarity to known species in the NCBI database (Table S1), suggesting their potential as new species. This discovery implies that cold environments might harbour a greater diversity of Cadophora species. Additional research is imperative to verify whether these species originated from glaciers or were transported into the lake through glacial meltwater.
Moreover, many fungi obtained in this study are plant-pathogenic. For instance, Fusarium verticillioides, a fungal species most frequently documented for infecting maize (Zea mays) and known for its production of mutagenic chemical compound fusarin C (Satterlee et al. Citation2022), has been isolated at a high frequency from sediment samples collected from Gyaring Co and Nam Co. Some other common plant and human pathogenic fungi isolated in this study include Fusarium fujikuroi (Yilmaz et al. Citation2021), F. oxysporum (Dean et al. Citation2012), and Truncatella angustata (Jagielski et al. Citation2015), etc. Moreover, this study isolated several common phytopathogenic fungal genera, including Alternaria, Didymella, Fusarium, Fusicolla, Neonectria, Paraphoma, Plectosphaerella, and Stagonospora (Table S1), with some species exhibiting less than 99% ITS similarity to the known species. Considering the pronounced diversity within rapidly differentiating phytopathogenic fungal taxa of these genera, even strains with 99% ITS similarity may represent different species (Hou et al. Citation2020; Han et al. Citation2023). In other words, they are likely to be emerging or newly discovered phytopathogenic fungi. For example, Myceliophthora tibetea sp. nov. shares 99.03% ITS sequence similarity with the known species Myceliophthora lutea, but based on multigene phylogenetic analyses and comparisons of morphological characters, we find that they are closely related but separate species (). Hence, it is imperative to accurately distinguish these cryptic species and assess their pathogenicity in upcoming studies, especially concerning economically important local plant species in Xizang. This evaluation is vital for understanding the potential risks they pose and for implementing monitoring and alert systems for these potentially pathogenic fungal species in the ecologically sensitive region of Xizang.
5. Conclusions
In conclusion, our examination of the culturable mycobiota in six lakes revealed a rich fungal diversity and a substantial level of species novelty, with the formal description of eight new taxa in this study. Additional potentially new taxonomic units will be documented in subsequent studies, accompanied by analyses of the evolutionary origins of taxa exhibiting distinctive distributions in this environment. Future research endeavours should integrate culture-independent methods to more comprehensively elucidate the overall landscape of fungal diversity, community compositions, and potential ecological roles of fungi in the lakes of Xizang.
Author contributions
F.L. and D.P. conceived the project. D.P., J.H., and Z.N. contributed to the sample collection. S.S., J.H., F.L., and D.P. performed lab experiments and data analyses. J.H. and S.L. contributed to the deposition of sequences and type specimens. F.L. is mainly responsible for drafting and revising the manuscript, with assistance from D.P., J.H., L.C., and X.Z. in manuscript revision.
Supplemental Material
Download MS Excel (201.8 KB)Supplemental data
Supplemental data for this article can be accessed online at https://doi.org/10.1080/21501203.2024.2333300.
Disclosure statement
No potential conflict of interest was reported by the author(s).
Additional information
Funding
References
- Amend A, Burgaud G, Cunliffe M, Edgcomb VP, Ettinger CL, Gutiérrez MH, Heitman J, Hom EFY, Ianiri G, Jones AC, et al. 2019. Fungi in the marine environment: Open questions and unsolved problems. mBio. 10(2):e01189–18. doi:10.1128/mbio.01189-18.
- Belyakova LA. 1970. Novij vid roda Emericellopsis (Euroticaeae). Mikol Fitopatol. 4:530–531.
- Blanchette RA, Held BW, Arenz BE, Jurgens JA, Baltes NJ, Duncan SM, Farrell RL. 2010. An Antarctic hot spot for fungi at Shackleton’s historic hut on Cape Royds. Microb Ecol. 60(1):29–38. doi: 10.1007/s00248-010-9664-z.
- Blanchette RA, Held BW, Hellmann L, Millman L, Büntgen U. 2016. Arctic driftwood reveals unexpectedly rich fungal diversity. Fungal Ecol. 23:58–65. doi: 10.1016/j.funeco.2016.06.001.
- Blanchette RA, Held BW, Jurgens JA, McNew DL, Harrington TC, Duncan SM, Farrell RL. 2004. Wood-destroying soft rot fungi in the historic expedition huts of Antarctica. Appl Environ Microbiol. 70:1328–1335. doi: 10.1128/AEM.70.3.1328-1335.2004.
- Dean R, Van-Kan JA, Pretorius ZA, Hammond-Kosack KE, Di-Pietro A, Spanu PD, Rudd JJ, Dickman M, Kahmann R, Ellis J, et al. 2012. The top 10 fungal pathogens in molecular plant pathology. Mol Plant Pathol. 13:414–430. doi: 10.1111/j.1364-3703.2011.00783.x.
- Durán P, Barra PJ, Jorquera MA, Viscardi S, Fernandez C, Paz C, Mora ML, Bol R. 2019. Occurrence of soil fungi in Antarctic pristine environments. Front Bioeng Biotechnol. 7:28. doi: 10.3389/fbioe.2019.00028.
- Egidi E, Delgado-Baquerizo M, Plett JM, Wang J, Eldridge DJ, Bardgett RD, Maestre FT, Singh BK. 2019. A few Ascomycota taxa dominate soil fungal communities worldwide. Nat Commun. 10(1):2369. doi: 10.1038/s41467-019-10373-z.
- Furbino LE, Godinho VM, Santiago IF, Pellizari FM, Alves T, Zani CL, Junior P, Romanha A, Carvalho AG, Gil LH, et al. 2014. Diversity patterns, ecology and biological activities of fungal communities associated with the endemic macroalgae across the Antarctic Peninsula. Microb Ecol. 67(4):775–787. doi:10.1007/s00248-014-0374-9.
- Glass NL, Donaldson GC. 1995. Development of primer sets designed for use with the PCR to amplify conserved genes from filamentous ascomycetes. Appl Environ Microbiol. 61:1323–1330. doi: 10.1128/aem.61.4.1323-1330.1995.
- Gonçalves VN, Vaz AM, Rosa CA, Rosa LH. 2012. Diversity and distribution of fungal communities in lakes of Antarctica. FEMS Microbiol Ecol. 82(2):459–471. doi: 10.1111/j.1574-6941.2012.01424.x.
- Gounot AM. 1986. Psychrophilic and psychrotrophic microorganisms. Experientia. 42:1192–1197. doi: 10.1007/BF01946390.
- Grum-Grzhimaylo AA, Georgieva ML, Debets AJ, Bilanenko EN. 2013. Are alkalitolerant fungi of the Emericellopsis lineage (Bionectriaceae) of marine origin? IMA Fungus. 4:213–228. doi: 10.5598/imafungus.2013.04.02.07.
- Guindon S, Gascuel O, Rannala B. 2003. A simple, fast, and accurate algorithm to estimate large phylogenies by maximum likelihood. Syst Biol. 52(5):696–704. doi: 10.1080/10635150390235520.
- Guo LD, Hyde KD, Liew ECY. 2000. Identification of endophytic fungi from Livistona chinensis based on morphology and rDNA sequences. New Phytol. 147:617–630. doi: 10.1046/j.1469-8137.2000.00716.x.
- Han SL, Wang MM, Ma ZY, Raza M, Zhao P, Liang J, Gao M, Li YJ, Wang JW, Hu DM, et al. 2023. Fusarium diversity associated with diseased cereals in China, with an updated phylogenomic assessment of the genus. Stud Mycol. 104:87–148. doi: 10.3114/sim.2022.104.02.
- Hou LW, Groenewald JZ, Pfenning LH, Yarden O, Crous PW, Cai L. 2020. The phoma-like dilemma. Stud Mycol. 96:309–396. doi: 10.1016/j.simyco.2020.05.001.
- Huang S, Liu Y, Hu A, Liu X, Chen F, Yao T, Jiao N, Voordouw G. 2014. Genetic diversity of picocyanobacteria in Tibetan lakes: assessing the endemic and universal distributions. Appl Environ Microbiol. 80(24):7640–7650. doi: 10.1128/AEM.02611-14.
- Huelsenbeck JP, Ronquist F. 2001. MRBAYES: Bayesian inference of phylogenetic trees. Bioinformatics. 17(8):754–755. doi: 10.1093/bioinformatics/17.8.754.
- Jagielski T, Żak I, Tyrak J, Bryk A, Warnock DW. 2015. First probable case of subcutaneous infection due to Truncatella angustata: a new fungal pathogen of humans? J Clin Microbiol. 53(6):1961–1964. doi: 10.1128/JCM.00400-15.
- Katoh K, Toh H. 2010. Parallelization of the MAFFT multiple sequence alignment program. Bioinformatics. 26(15):1899–1900. doi: 10.1093/bioinformatics/btq224.
- Kumar S, Stecher G, Tamura K. 2016. MEGA7: molecular evolutionary genetics analysis version 7.0 for bigger datasets. Mol Biol Evol. 33:1870–1874. doi: 10.1093/molbev/msw054.
- Li D, Davis JE, Wang G, Nabi G, Bishop VR, Sun Y, Meddle SL, Wingfield JC, Lei F. 2020. Coping with extremes: remarkably blunt adrenocortical responses to acute stress in two sympatric snow finches on the Qinghai-Tibet Plateau during winter relative to other seasons. Gen Comp Endocrinol. 291:113434. doi: 10.1016/j.ygcen.2020.113434.
- Liu F, Hu DM, Cai L. 2012. Conlarium duplumascospora gen. et. sp. nov. and Jobellisia guangdongensis sp. nov. from freshwater habitats in China. Mycologia. 104(5):1178–1186. doi: 10.3852/11-379.
- Liu YJ, Whelen S, Hall BD. 1999. Phylogenetic relationships among ascomycetes: evidence from an RNA polymerse II subunit. Mol Biol Evol. 16:1799–1808. doi: 10.1093/oxfordjournals.molbev.a026092.
- Liu Y, Yao T, Jiao N, Liu X, Kang S, Luo T. 2013. Seasonal dynamics of the bacterial community in Lake Namco, the largest Tibetan lake. Geomicrobiol J. 30:17–28. doi: 10.1080/01490451.2011.638700.
- Liu K, Yao T, Pearce DA, Jiao N, Zeng Y, Guo B, Liu Y. 2021. Bacteria in the lakes of the Tibetan Plateau and polar regions. Sci Total Environ. 754:142248. doi: 10.1016/j.scitotenv.2020.142248.
- Milner AM, Brown LE, Hannah DM. 2009. Hydroecological response of river systems to shrinking glaciers. Hydrol Process. 23:62–77. doi: 10.1002/hyp.7197.
- Morita RY. 1975. Psychrophilic bacteria. Biol Rev. 39(2):144–167. doi: 10.1128/br.39.2.144-167.1975.
- Nagano Y, Miura T, Nishi S, Lima AO, Nakayama C, Pellizari VH, Fujikura K. 2017. Fungal diversity in deep-sea sediments associated with asphalt seeps at the Sao Paulo Plateau. Deep Sea Res Part II. 146:59–67. doi: 10.1016/j.dsr2.2017.05.012.
- Rannala B, Yang Z. 1996. Probability distribution of molecular evolutionary trees: a new method of phylogenetic inference. J Mol Evol. 43:304–311. doi: 10.1007/BF02338839.
- Rehner SA, Buckley E. 2005. A beauveria phylogeny inferred from nuclear ITS and EF1- sequences: evidence for cryptic diversification and links to cordyceps teleomorphs. Mycologia. 97(1):84–98. doi: 10.3852/mycologia.97.1.84.
- Richards TA, Jones MD, Leonard G, Bass D. 2012. Marine fungi: their ecology and molecular diversity. Ann Rev Mar Sci. 4:495–522. doi: 10.1146/annurev-marine-120710-100802.
- Satterlee T, Williams FN, Nadal M, Glenn AE, Lofton LW, Duke MV, Scheffler BE, Gold SE. 2022. Transcriptomic response of Fusarium verticillioides to variably inhibitory environmental isolates of Streptomyces. Front Fungal Bio. 3:894590. doi: 10.3389/ffunb.2022.894590.
- Simmons EG. 1998. Multiplex conidium morphology in species of the Ulocladium atrum group. Can J Bot. 76:1533–1539. doi: 10.1139/b98-091.
- Stamatakis A. 2014. RAxML version 8: a tool for phylogenetic analysis and post-analysis of large phylogenies. Bioinformatics. 30:1312–1313. doi: 10.1093/bioinformatics/btu033.
- Stolk AC. 1955. Emericellopsis minima sp. nov. and Westerdykella ornata gen. nov., sp. nov. Trans Br Mycol Soc. 38(4):419–IN2. doi: 10.1016/S0007-1536(55)80046-0.
- Sung GH, Sung JM, Hywel-Jones NL, Spatafora JW. 2007. A multi-gene phylogeny of Clavicipitaceae (Ascomycota, fungi): identification of localized incongruence using a combinational bootstrap approach. Mol Phylogenet Evol. 44:1204–1223. doi: 10.1016/j.ympev.2007.03.011.
- Tsuji M, Fujiu S, Xiao N, Hanada Y, Kudoh S, Kondo H, Tsuda S, Hoshino T. 2013. Cold adaptation of fungi obtained from soil and lake sediment in the skarvsnes ice-free area, Antarctica. FEMS Microbiol Lett. 346:121–130. doi: 10.1111/1574-6968.12217.
- Vilgalys R, Hester M. 1990. Rapid genetic identification and mapping of enzymatically amplified ribosomal DNA from several Cryptococcus species. J Bacteriol. 172:4238–4246. doi: 10.1128/jb.172.8.4238-4246.1990.
- Wang XW, Han PJ, Bai FY, Luo A, Bensch K, Meijer M, Kraak B, Han DY, Sun BD, Crous PW, et al. 2022. Taxonomy, phylogeny and identification of Chaetomiaceae with emphasis on thermophilic species. Stud Mycol. 101:121–243. doi: 10.3114/sim.2022.101.03.
- Wang F, Wang K, Cai L, Zhao M, Kirk PM, Fan G, Sun QL, Li B, Wang S, Yu ZF, et al. 2023. Fungal names: a comprehensive nomenclatural repository and knowledge base for fungal taxonomy. Nucleic Acids Res. 51:D708–D716. doi: 10.1093/nar/gkac926.
- Wei C, Sun D, Yuan WL, Li L, Dai C, Chen Z, Zeng X, Wang S, Zhang Y, Jiang S, et al. 2023. Metagenomics revealing molecular profiles of microbial community structure and metabolic capacity in Bamucuo lake, Tibet. Environ Res. 217:114847. doi: 10.1016/j.envres.2022.114847.
- White TJ, Bruns T, Lee S, Taylor J. 1990. Amplification and direct sequencing of fungal ribosomal RNA genes for phylogenetics. PCR protocols: a guide to methods and applications. 18:315–322. doi:10.1016/B978-0-12-372180-8.50042-1.
- Xue W, Meng H, Wang Y, Zhu P, De J, Guo X. 2022a. Relationship between culturable filamentous fungal diversity and environmental factors in Nam Co Lake. Biodivers Sci. 30:21473. doi: 10.17520/biods.2021473.
- Xue W, Zhu P, De J, Guo X. 2022b. Study on the temporal and spatial characteristics of the dominant species of cultivable filamentous fungi in Nam Co Lake. Ecol Environ Sci. 31:2331–2340.
- Yang J, Ma LA, Jiang H, Wu G, Dong H. 2016. Salinity shapes microbial diversity and community structure in surface sediments of the Qinghai-Tibetan Lakes. Sci Rep. 6:25078. doi: 10.1038/srep25078.
- Yilmaz N, Sandoval-Denis M, Lombard L, Visagie CM, Wingfield BD, Crous PW. 2021. Redefining species limits in the Fusarium fujikuroi species complex. Persoonia. 46:129–162. doi: 10.3767/persoonia.2021.46.05.
- Zhang B, Li X, Li G, Wang QM, Wang M. 2022. Cadophora species from marine glaciers in the Qinghai-Tibet Plateau: an example of unsuspected hidden biodiversity. IMA Fungus. 13:15. doi: 10.1186/s43008-022-00102-5.
- Zhang Y, Liu F, Wu W, Cai L. 2015. A phylogenetic assessment and taxonomic revision of the thermotolerant hyphomycete genera Acrophialophora and Taifanglania. Mycologia. 107:768–779. doi: 10.3852/14-173.
- Zhaxybayeva O, Gogarten JP. 2002. Bootstrap, Bayesian probability and maximum likelihood mapping: exploring new tools for comparative genome analyses. BMC Genomics. 3:1–15. doi: 10.1186/1471-2164-3-4.
- Zheng M. 1997. An introduction to saline lakes on the Qinghai-Tibet plateau Ch. Vol. 1, Kluwer Academic Publisher; p. 1–17. doi:10.1007/978-94-011-5458-1_1
- Zhu X, Deng Y, Huang T, Han C, Chen L, Zhang Z, Liu K, Liu Y, Huang C. 2023. Vertical variations in microbial diversity, composition, and interactions in freshwater lake sediments on the Tibetan plateau. Front Microbiol. 14:1118892. doi: 10.3389/fmicb.2023.1118892.