ABSTRACT
Klebsiella pneumoniae is an important gram-negative bacterium that causes severe respiratory and healthcare-associated infections. Although antibiotic therapy is applied to treat severe infections caused by K. pneumoniae, drug-resistant isolates pose a huge challenge to clinical practices owing to adverse reactions and the mismanagement of antibiotics. Several studies have attempted to develop vaccines against K. pneumoniae, but there are no licensed vaccines available for the control of K. pneumoniae infection. In the current study, we constructed a novel DNA vaccine, pVAX1-YidR, which encodes a highly conserved virulence factor YidR and a recombinant expression plasmid pVAX1-IL-17 encoding Interleukin-17 (IL-17) as a molecular adjuvant. Adaptive immune responses were assessed in immunized mice to compare the immunogenicity of the different vaccine schemes. The results showed that the targeted antigen gene was expressed in HEK293T cells using an immunofluorescence assay. Mice immunized with pVAX1-YidR elicited a high level of antibodies, induced strong cellular immune responses, and protected mice from K. pneumoniae challenge. Notably, co-immunization with pVAX1-YidR and pVAX1-IL-17 significantly augmented host adaptive immune responses and provided better protection against K. pneumoniae infections in vaccinated mice. Our study demonstrates that combined DNA vaccines and molecular adjuvants is a promising strategy to develop efficacious antibacterial vaccines against K. pneumoniae infections.
Introduction
Klebsiella pneumoniae (K. pneumoniae), an important opportunistic and clinical pathogen, is a serious threat to global public health [Citation1]. It is a gram-negative, encapsulated, and resilient bacterium that causes a wide variety of infectious diseases such as pneumonia, sepsis, and bacteremia [Citation2,Citation3]. With a large genome of virulence genes and plasmids, this bacterium settles in different sites of the host and is divided into three groups: opportunistic, hypervirulent, and multidrug-resistant K. pneumoniae [Citation4,Citation5]. Although antibiotic therapy is an important treatment option for severe cases caused by K. pneumoniae, drug-resistant isolates are considered a major challenge in clinical practices owing to adverse reactions and the mismanagement of antibiotics [Citation6].
Vaccines, are lifesaving prophylactic agents that prevent colonization and infections caused by K. pneumoniae [Citation7]. Previous studies on the development of vaccines targeting K. pneumoniae include whole cell vaccines/cell lysates, capsular polysaccharides vaccines, lipopolysaccharides vaccines, protein-based vaccines, outer membrane vesicle (OMV) vaccines, and a conjugate vaccine [Citation8,Citation9]. However, these vaccines have several shortcomings such as low stability, adverse toxic reactions, and incomplete efficacy in protecting animals and humans [Citation6,Citation8]. K. pneumoniae may be difficult to control by these vaccines owing to strain heterogeneity and none of these vaccine preparations have been licensed due to their high cost [Citation6,Citation10]. Therefore, there is an urgent need to develop effective strategies to prevent K. pneumoniae infections.
DNA vaccines are an efficient strategy to activate the broad immune responses of the host and have been successfully demonstrated in a variety of animal models and humans against many infectious diseases caused by viral, bacterial, and parasitic pathogens [Citation11–13]. Compared to conventional vaccines, DNA vaccines are considered more effective for enhancing both humoral and cellular immunity of the host and are more stable and safe [Citation14]. A DNA vaccine encoding the outer membrane protein A of K. pneumoniae was shown to protect mice from K. pneumoniae infection [Citation15]. A previous study demonstrated that the yidR gene encodes a highly conserved virulence factor among K. pneumoniae strains. YidR is a putative ATP/GTP-binding protein and regulates the hyperadherence phenotype [Citation6,Citation16]. As an important member of interleukin-17 (IL-17) family, the proinflammatory cytokine IL-17 (also known as IL-17A) is produced by T helper 17 cells and IL-17 signaling is mediated by the IL-17 receptor family [Citation17]. Recent studies have demonstrated that IL-17 and IL-17 related signaling are vital for host defense against pulmonary bacterial pathogens such as K. pneumoniae [Citation18,Citation19]. Additionally, IL-17 has been used as a novel molecular adjuvant to boost the immunogenicity of specific antigens and improve the protective efficacy of different vaccines [Citation20,Citation21]. However, whether a DNA vaccine encoding the YidR virulence factor or IL-17 molecular adjuvant could be applied to prevent K. pneumoniae infections remains unknown.
In this study, a combined vaccination scheme was designed to develop a novel DNA vaccine to prevent K. pneumoniae infections. This scheme combines a DNA vaccine named pVAX1-YidR and a molecular adjuvant pVAX1-IL-17 to maximize adaptive immune responses based on a mouse model. Specific humoral or cell-mediated immune responses elicited by the vaccination were detected. In addition, the protective effects of these vaccines were evaluated by infecting with K. pneumoniae. Our work lays the foundation for the development of effective DNA vaccines that induce broad protection against K. pneumoniae.
Materials and methods
Growth conditions of bacterial strains and cells
K. pneumonia WT strain ATCC 43816 (WT) was preserved in this laboratory and were incubated in LB broth medium at 37°C as described in our previous report [Citation2]. Human embryonic kidney cells (HEK293T) were kindly provided by Dr. Xikun Zhou (Sichuan University, China) and were maintained in Dulbecco’s Modified Essential Medium (DMEM, Gibco, USA) with a concentration of 10% fetal bovine serum (FBS, Gibco) at 37°C with 5% CO2 [Citation22].
Construction of plasmids
The vectors pVAX1 and pET28a were preserved in this laboratory [Citation23]. Total DNA of the KPWT strain (GenBank accession number: NZ_KN046818.1) was extracted and used as a template for polymerase chain reaction (PCR) amplification. Specific primers () were applied for the PCR amplification of the yidR gene of KP. The obtained PCR products were purified and treated with restriction endonucleases (), followed by cloning into the empty vector pVAX1 or pET28a, producing the recombinant plasmid pVAX1-YidR (p-YidR) or pET28a-YidR. The IL-17 gene of Mus musculus (GenBank accession number: NM_010552.3) was synthesized by Tsingke Biotechnology Co., Ltd. (Chengdu, China) and incorporated into pVAX1 designated as pVAX1-IL-17 (p-IL17). These recombinant plasmids were confirmed by an endonuclease digestion assay and PCR amplification ().
Table 1. Primers for PCR used in the present study.
Expression and purification of recombinant protein His-YidR
Recombinant proteins were expressed in E. coli BL21 (DE3) strain and purified as previously mentioned with minor modifications [Citation6,Citation23]. Briefly, the recombinant pET28a-YidR plasmid was transformed into chemically competent E. coli BL21 (DE3) in LB medium and the culture was incubated at 37°C with 5% CO2 for 5 h to 6 h until the OD600 reached 0.5. Isopropyl β-D-Thiogalactopyranoside (IPTG) was added to a specified concentration of 0.1 mM, and then the culture was shaken at 37°C for 24 h. Proteins were isolated and identified using 12% (w/v) sodium dodecyl sulfate-polyacrylamide gel electrophoresis. The recombinant His-YidR proteins were further purified using HisTrap affinity column (GE Healthcare, USA) following the manufacturer’s instructions. To further confirm the identity of recombinant His-YidR proteins, separated proteins were detected by western blotting using primary antibody anti-His mouse monoclonal antibody (1 mg/ml, Beyotime, China) at a dilution of 1:1500. The first antibody was then detected with goat anti-mouse antibody conjugated to horseradish peroxidase (HRP) (Abways, China) and detected by using enhanced chemiluminescence (ECL) reagents.
Confocal fluorescence microscopy
The recombinant plasmids p-YidR or p-IL17 were transformed into E. coli DH5a strain and purified to clear endotoxin using FastPure Endo-Free Plasmid Maxi Kit (Vazyme Biotech, China). The plasmids were stored in 0.1 M PBS (pH 7.4) and were adjusted to a concentration of 1000 µg/ml. Subsequently, 1 µg of p-YidR or p-IL17 was transfected into HEK293T cells using Lipofectamine 2000 reagent (Invitrogen, USA). The expression of YidR and IL-17 proteins was detected by immunofluorescence staining 24 h post-transfection as described previously, with minor modifications [Citation24]. Briefly, the cells were gently fixed with 4% methanol and were incubated with the anti-His mouse monoclonal antibody (1:500 dilution in 0.1 M PBS, Beyotime). After being washed 3 times with PBS, the cells were incubated with FITC-labeled goat anti-mouse IgG (Solarbio Life Science, Beijing, China) for 1 h in the dark (1:200 dilution in 0.1 M PBS). Cells were washed with PBS solutions and then were incubated with Hoechst 33,342 dye (Solarbio Life Science) for 10 min in the dark. Fluorescence signals were detected using a confocal microscope (Leica, Germany).
Immunization experiments
All animal protocols were carefully carried out according to the Institutional Animal Care and Use Committee guidelines (Chengdu University). Animals were housed in a room with 12 h light/dark cycle at about 22°C, and were fed with sterile pellet diet and water ad libitum. Six-week-old, out-bred SPF female Kunming (KM) mice were purchased from Dashuo Biotechnology Company (Chengdu, China). The mice were randomly divided into five different groups (18 mice per group) as follows: PBS, p-IL17, p-YidR, p-YidR plus p-IL17 (p-YidR+p-IL17). The normal female KM mice (6 weeks old, n = 12) were without any treatment. After anesthetization with ketamine solution, the mice were primed with plasmids (50 µg/mouse) or PBS solution (50 µL) in the rectus femoris muscle, and were boosted on day 14 after the first vaccination using the same inoculation schemes.
IgG antibody production in serum
The peripheral blood of mice was harvested at regular time points after vaccination (days 0, 14, and 28). After anesthetized intraperitoneally with ketamine solution, the needle connected to a sterile syringe was inserted into the lateral tail vein of mice. Approximately 50 µL-100 µL of blood was collected by the syringe and was moved to a sterile 1.5 mL centrifuge tube from the syringe. After blood collection, the needle was removed and pressed the puncture point to stop bleeding. Blood samples were carefully incubated at 37°C for approximately 1 h and then was centrifuged at 800 rpm for 5 min to collect the serum. The levels of YidR-specific antibodies were detected using an ELISA assay to determine the humoral immune response of the host according to a previous study [Citation6]. In addition, antibody production of IgG1 and IgG2a subtypes in mouse serum was monitored at specific time points (days 0, 14, and 28) by ELISA.
Splenic lymphocyte proliferation assay
The proliferation of lymphocytes from mouse spleens was measured using a cell counting kit-8 (called CCK-8) following the instructions of manufacturer (Mei5 Biotechnology Co., Ltd. Beijing, China). CCK-8 solution contains WST-8, a highly water-soluble tetrazolium salt that is reduced by dehydrogenase activities in cells to produce a soluble orange-color formazan dye. The amount of the formazan dye, produced by the activities of dehydrogenases in cells, is proportional to the quantity of living cells. The spleen lymphocytes were carefully separated by a mouse lymphocyte separation medium kit according to the manufacturer’s instructions (Solarbio Life Science). Subsequently, spleen lymphocytes were added to a 96-well plate at a concentration of 5 × 105 cells/well in 100 µL complete growth medium (DMEM with 10% FBS), and purified His-YidR protein (10 µg/mL) was used as a stimulus. For the negative control group, a total of 100 µL DMEM medium was added to the 96-well plate without any treatment. For the positive control group, spleen lymphocytes from the normal mice were treated with concanavalin A (ConA, 5 µg/mL, Solarbio Life Science). The cells were cultivated for 24 h at 37°C. CCK-8 solution was then added to the 96-well plate (10 µL/well) and incubated for 3–4 h in the incubator. The absorbance of the cell samples was measured at 450 nm using a microplate reader as previously described [Citation25]. All treatments were performed at least thrice.
Flow cytometry immunophenotyping
The percentage of CD4+ and CD8+ T lymphocyte from the mouse spleen was analyzed by flow cytometry, as described in previous studies with minor modifications [Citation26,Citation27]. Briefly, spleen lymphocytes were isolated as described above and fixed with 1% formaldehyde solution. Subsequently, the lymphocytes were treated with FITC-conjugated anti-mouse CD4 antibody, phycoerythrin (PE)-conjugated anti-mouse CD8 antibody, FITC mouse IgG2b κ isotype control antibody, or PE mouse IgG1 κ isotype control antibody (BioLegend, San Diego, CA, USA) at 4°C for approximately 2 h following the instructions of manufacturer. The labeled cells were washed with cold PBS solution three times and analyzed by a flow cytometer (BD CantoII, Franklin Lakes, NJ, USA). All treatments were performed in triplicate.
Cytokine profiling
Spleen lymphocytes were collected as described above. The profile of cytokines including interferon-γ (IFN-γ), IL-2, and IL-4 in the supernatant of the spleen lymphocytes was measured using three different cytokine ELISA kits (Solarbio Life Science) according to the supplier’s manual. The expression levels of these key cytokines were analyzed based on the standard method as illustrated in our previous report [Citation27]. All treatments were performed in triplicate.
Challenge experiments
Challenge experiments were carried out two weeks after the second immunization as previously described with minor modifications [Citation15,Citation28]. Briefly, after anesthetizing the mice with ketamine solution, their trachea was exposed to a vertical cut of the skin. A sterile catheter was inserted into the trachea and 5 × 108 CFU of KPWT strain in 30 µL suspension was gently injected into the lung using a 1 mL syringe and attached to the catheter. The incision was closed using suture clips. The bacterial burden in the lungs and bronchoalveolar lavage fluid (BAL) of the different vaccination groups were collected and determined on day 2 post-infection on an LB agar plate as previously described [Citation29]. Additionally, the survival rate of the immunized mice was assessed for the protective efficacy of the respective DNA vaccine by observing the clinical signs of the mice, including posture, coat quality, ambulation, and body weight, for 14 days as previously described [Citation30].
Histological analysis
The mice were euthanized by isoflurane inhalation and lung samples of mice from different immunized groups were collected aseptically on day 2 post-infection. Subsequently, the lungs were fixed in 4% paraformaldehyde solution using a routine histologic procedure, as previously described [Citation31]. Paraffin-embedded tissue sections were prepared by a rotary microtome and subjected to hematoxylin-eosin staining to observe the tissue lesion using a light microscope. The histology score was determined by using a score varying from 0 (no lesion) to 4 (extended lesions) according to a previous study [Citation32]. In brief, a semi-quantitative analysis was carried out and based on the following criteria: alveolar necrosis, leukocyte infiltration and vascular congestion. The number 0 indicates within normal limits; 1 indicates minimal; 2 indicates slight; 3 indicates moderate; 4 indicates severe. Triplicates were performed for the different vaccination groups (n = 3 mice per group).
Statistical analysis
Statistical analysis was performed by a GraphPad Prism software (Version 5.0) in this work. Data are shown as mean ± SEM and were analyzed using one-way analysis of variance followed by a Tukey – Kramer post hoc test or a chi-square test with Yates’ correction. Statistical value (p < 0.05) was typically considered statistically significant.
Results
Purification and identification of the recombinant protein His-YidR
The yidR amplicon was treated with XhoI and EcoRI enzymes, followed by cloning into the expression vector pET-28a to generate the recombinant plasmid pET28a-YidR (). The expression plasmid pET28a-YidR was transformed into a competent E. coli strain. Subsequently, protein expression in the recombinant strains was induced by different concentration of IPTG and His-YidR protein was detected at about 49.5 kDa (). The recombinant His-YidR protein was purified and was corresponded to its predicted molecular weight (). After purification, the recombinant His-YidR Protein was concentrated () and was identified by western blotting ().
Figure 1. Characterization of recombinant plasmids and recombinant protein His-YidR. (a–c) Schematic representation of pEt28a-YidR (a), pVAX1-YidR (b), and pVAX1-IL-17 (c) plasmids. (d) Expression of His-YidR were detected by coomassie-stained SDS-PAGE gels. M, protein marker; 1, total bacterial proteins in E. coli BL21 (DE3) containing pET-28a plasmid; 2–6, the E. coli BL21 (DE3) containing pEt28a-YidR was induced by IPTG at different concentration (0mM, 0.1mM, 0.5mM, 1.0mM, 2mM). (e) Purification of His-YidR were analyzed with Coomassie-stained SDS-PAGE gels. M, protein marker; 1, supernatant from whole-cell lysates; 2, flow-through solutions; 3–6, the recombinant protein His-YidR was purified by four concentrations of imidazole solutions (100mM, 200mM, 300mM, and 400mM). (f) The purified His-YidR was further concentrated by ultrafiltration device as shown in line 1. The position of the 49.5 kDa His-YidR band is pointed out by the arrow. (g) The separated proteins were transferred to polyvinylidene difluoride and detected by western blotting. 1, purified His-YidR; 2, control, total bacterial proteins in E. coli BL21 (DE3) containing pET-28a plasmid.
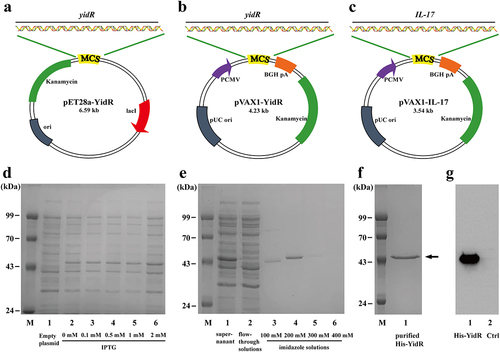
In vitro expression of DNA vaccine in HEK293T cells
The sequence of the yidR or IL-17 genes from the recombinant expression plasmid pVAX1-YidR (p-YidR, ) or pVAX1-IL-17 (p-IL17, ) shared 100% identity with KPWT or M. musculus by DNA sequencing. To confirm the expression of the targeted protein, HEK293T cells were transfected with the plasmids p-yidR or p-IL17 and the targeted protein was detected by an immunofluorescence assay. We found that the green fluorescence signal (, b1–c1) was detected in the cytoplasm by confocal microscopy of HEK293T cells transfected with plasmids p-IL17 or p-YidR. However, these effects were not determined in the control groups transfected with the empty vector pVAX1 (, a1).
Figure 2. Transient expression of YidR and IL-17 in HEK293T cells by a confocal fluorescence microscopy. HEK293T cells were transfected with plasmid pVAX1 (a1–a3), p–IL17 (b1-b3), or p-YidR (c1–c3) for 36 h. Transient expression of proteins was detected with anti-His mouse monoclonal antibody. FITC: FITC-conjugated goat anti-mouse IgG; DAPI: cell nuclei; Merge: the overlay fluorescence images of FITC and DAPI; the scale bar is 20 µm.
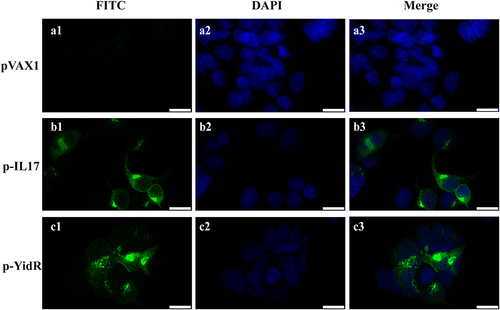
DNA vaccines combined with IL-17 elevated antibody production
To investigate the immunogenicity of a monovalent DNA vaccine group (p-YidR) or the combination group (p-YidR+p-IL17), specific antibody responses were determined using an ELISA assay. The timeline of the in vivo experiment is shown in . As illustrated in , vaccination with the plasmid p-YidR significantly elicited antibody production in mice 14 days post-immunization (dpi). The specific YidR-antibody response was augmented after the second vaccination at 28 dpi. Additionally, antibody production in the p-YidR+p-IL17 group was improved compared to the single DNA vaccine group at 14 and 28 dpi, but the elicited antibody was not significantly different between the PBS group and p-IL17 groups. IgG1 and IgG2a antibodies were detected at the indicated time points as described above by ELISA (). As shown in , the IgG2a antibody was higher than the IgG1 subtype, implying that the DNA vaccine group or combination group mainly activated Th1 type immunity in immunized mice.
Figure 3. Vaccinations and Antibody responses elicited by the DNA vaccines. (a) Schematic illustrating the experimental timeline; KM mice were vaccinated or given PBS buffer, followed by infection with KPWT. Vaccinations are represented by the empty syringe; KPWT challenge is indicated with the black syringe. Mice were immunized with the DNA vaccines and boosted once at day 14 after primary immunization as described in methods. The anti-YidR IgG (b), IgG1 (c) and IgG2a (d) levels were detected at the indicated time point by an ELISA assay. (e) Dynamic changes of sera IgG2a/IgG1 against YidR in immunized mice. PBS was used as a control group. Data represented means ± SEM of three independent experiments. *p < 0.05, **p < 0.01, ***p < 0.001.
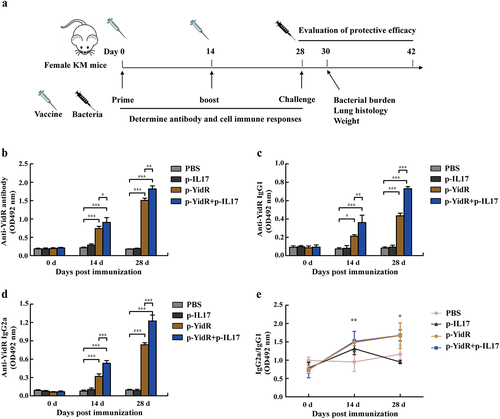
DNA vaccines combined with IL-17 enhanced cellular immune responses
Changes in total lymphocytes/T cell subtype (CD4+ and CD8+ T lymphocytes) and the relative production of cytokines induced by the DNA vaccines were detected to estimate the magnitude of cellular immune responses in the immunized mice. The results of the CCK-8 assay presented that the total lymphocytes in the p-YidR group were significantly increased at 14 dpi (). Notably, the total lymphocytes of the DNA vaccination groups continually increased after the second immunization at 28 dpi, and the p-YidR+p-IL17 group induced more lymphocytes than other groups. However, these effects were not discovered in the PBS group or p-IL17 group (). Next, flow cytometry was carried out to determine the ratios of CD4+ and CD8+ T lymphocytes in the spleens of immunized mice. As shown in , a growing number of CD4+ and CD8+ T lymphocytes were detected after immunization with DNA vaccines between 14 dpi and 28 dpi compared with the control groups. The mice in the combined immunization group showed a significantly higher level of T lymphocytes proliferation than those in the other groups ().
Figure 4. Proliferation of lymphocytes in spleens of mice immunized with the DNA vaccines. (a) The lymphocytes from the spleen of mice were separated and the proliferation of lymphocytes was detected by a CCK-8 assay. ConA indicates concanavalin A. (b–c) The CD4+ and CD8+ T lymphocytes were separated from the spleen and were sorted by flow cytometry at the indicated time points. Data represented means ± SEM of three independent experiments. *p < 0.05, **p < 0.01, ***p < 0.001.
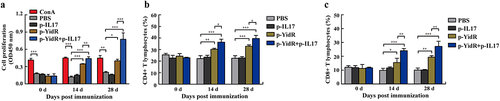
In an ex vivo experiment, the relative production of cytokines (IFN-γ, IL-2, and IL-4) in the separated spleen lymphocytes was determined by an ELISA assay. As shown in , we found that immunization with p-YidR alone promoted the secretion of IL-2, IFN-γ, and IL-4 in spleen lymphocytes. The relative production of cytokines in p-IL17 group was not significant difference in comparison to the PBS group (). Importantly, immunization with p-YidR+p-IL17 triggered higher levels of cytokines from 14 dpi to 28 dpi compared to the other groups (). Collectively, these data demonstrate that vaccination with p-YidR DNA plasmids elicited strong cellular immune responses and that IL-17 could be used as a potent adjuvant in combination with novel DNA antigens to enhance host cellular immune responses.
Figure 5. Assessment of cytokines from the suspension of the spleen lymphocytes. The spleen lymphocytes of immunized mice were harvested and were separated at the indicated time points as described above. The production levels of IL-2 (a), IFN-γ (b), and IL-4 (c) were determined by an ELISA assay. PBS was used as a control group. Data represented means ± SEM of three independent experiments. **p < 0.01, ***p < 0.001.
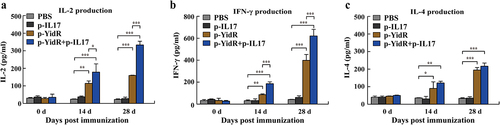
DNA vaccines combined with IL-17 protected mice from K. pneumonia infection
To estimate the protectiveness of DNA vaccine-induced immunity against K. pneumonia infection, the residual CFUs and survivability of immunized mice were examined using a mouse acute pulmonary infection with the KPWT strain. In the mice challenged with KPWT, large bacterial counts from the lung tissues or BAL fluids were detected in the PBS and p-IL17 group (). All mice immunized with DNA vaccines displayed fewer bacteria in the lung tissues () or BAL fluids () than the control group at 2 days post infection. Notably, the group of combined DNA immunization resulted in a remarkable clearance of K. pneumonia compared to the other groups () and represented lower clinical score than the other groups (Figure S1). For the analysis of survival rate, we found that none of the mice survived beyond 5 days or 4 days in the PBS and p-IL17 group (). In contrast, more than half of the mice immunized with DNA vaccines alone survived KPWT challenge at 14 days post challenge (). Moreover, most mice immunized with p-YidR+p-IL17 displayed a stronger protective effect compared to other groups ().
Figure 6. Residual CFUs and survival rate of mice challenged with K. pneumoniae. The mice were challenged at day 28 post immunization with KPWT strain to detect the residual bacteria of lungs (a) and BAL (b). Survival rate of mice was monitored for the subsequent 14 days (c). PBS was used as a control. *p < 0.05 and ***p < 0.001.
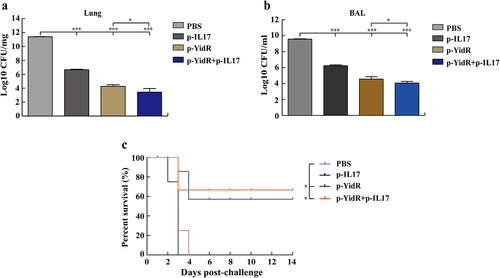
For histological analysis, the mouse lungs of the PBS or p-IL17 group showed necrotic lesions containing destructive alveolar epithelial cells, cellular debris and severe inflammatory cell infiltration compared to the normal group (). Nevertheless, most mice immunized with DNA vaccines significantly eliminated invading bacteria and alleviated tissue injury in the mouse lungs during K. pneumonia infection (). Importantly, we found that fewer histopathological changes were detected in the combined immunization group than in the p-IL17-treated groups (). Altogether, these results indicate that the novel DNA vaccines or their combination with the IL-17 molecular adjuvant effectively protected mice against K. pneumonia challenge.
Figure 7. Histopathologic analysis of mice lungs challenged with K. pneumoniae. The mice were challenged at day 28 with KPWT strain as described above. The lungs of immunized mice were harvested for evaluation of histology alteration (a-e). Abnormal cells of lung and cellular debris in the control group (b) are indicated by the black arrows. (f) Histology score in the lungs. The scale bar is 20 µm. PBS was used as a control. *p < 0.05, **p < 0.01, and ***p < 0.001.
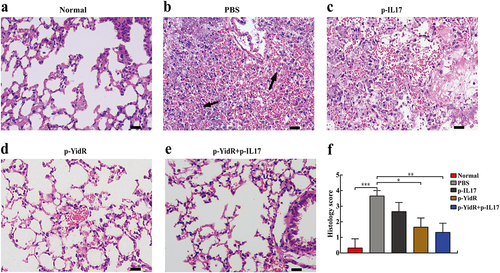
Discussion
As an important clinical bacterium, K. pneumonia is related to nosocomial infections such as severe pneumonia, meningitis, and sepsis [Citation7]. K. pneumonia infections can cause substantial societal burden and healthcare costs worldwide [Citation33]. The emergence and widespread antibiotic resistance of K. pneumoniae limits the therapeutic strategy against this versatile pathogen, especially in hospital environments [Citation8]. In addition, the complex pathogenicity of K. pneumoniae and its diverse strain heterogeneity hinder the development of universal and potent vaccines. Thus, it is essential to design novel and effective vaccines to control K. pneumoniae infections. The gene yidR encodes a presumed and highly conserved ATP/GTP-binding protein, YidR, which mediates the hyperadherence phenotype [Citation6,Citation16]. Because a surface-associated antigen is easily recognized by the host’s immune system, the YidR protein is chosen as a vaccine candidate. In the current study, we designed and evaluated a novel DNA vaccine containing the antigen YidR to prevent K. pneumoniae infection. We found that the DNA vaccine p-YidR induced strong adaptive immune responses and significantly protected the immunized mice against K. pneumoniae. Intriguingly, the p-IL17 plasmids promoted the immunogenicity of p-YidR in a mouse model, implying that IL-17 molecular adjuvant and DNA vaccine p-YidR can act synergistically to provide broad protective immunity to control K. pneumoniae infections.
As mentioned in a published study, YidR-specific antibody production is an important marker of humoral immunity because the antibody response plays a critical role in bacterial clearance and the recovery of host health [Citation6]. Analyses of IgG antibody responses indicated that vaccination with the novel p-YidR DNA vaccines elicited strong antibody responses in the host, which was similar to other findings on the designed DNA vaccines against K. pneumoniae [Citation15]. Surprisingly, the mice immunized with p-YidR+p-IL17 induced higher levels of specific antibodies at 28 dpi than the other groups. Previous studies have demonstrated that IL-17 can improve the immunogenicity of vaccines [Citation20,Citation21]. Thus, our work further validated the adjuvant effect of antibody responses of IL-17 in immunized mice compared to the p-YidR DNA vaccine alone.
Recent findings indicate that cellular immune responses are the predominant protective mechanism against K. pneumoniae infections [Citation8,Citation34]. Using immunized mice to evaluate specific cellular immune responses, we observed that the DNA vaccines p-YidR promoted the proliferation of lymphocytes, such as the T lymphocytes subtypes (CD4+ and CD8+). Meanwhile, the combined p-YidR+p-IL17 promoted higher levels of lymphocyte proliferation in immunized mice than in the other groups. As the production of cytokines acts as the most real-time reflector for the cellular immune regulation of the host [Citation35,Citation36], the secreted levels of IL-2, IFN-γ and IL-4 were subsequently detected by a cytokine profiling assay. We found that the production levels of all tested cytokines were higher in the DNA-vaccinated groups than in the PBS group, implying that the designed DNA vaccines in the current study were able to induce an effective adaptive immune response. Although the level of IL-4 was not significantly different between the groups p-yidR and p-yidR+p-IL-17, the combination p-YidR+p-IL17 markedly increased the production of IL-2 and IFN-γ compared to the other groups. A possible explanation for this observation is that the DNA vaccine group or combination group designed in our study mainly activated Th1 type immunity in immunized mice as mentioned above. Importantly, the essential collaborations contribute to the protective effect of the host defense against K. pneumoniae infections and the development of individual antibacterial cellular immunity. Unlike the protein vaccine that mainly induces a humoral immune response [Citation6], our DNA vaccines induced specific humoral and cytotoxic responses, which provided broad protection against K. pneumoniae challenges. Altogether, this study showed that p-YidR significantly activated the proliferation of T lymphocytes and the production of cytokines in the presence of an adjuvant, suggesting that IL-17 may function as a potent adjuvant in combination with new DNA immunogens to enhance adaptive cellular immunity of the host.
Bacterial distribution and lesions have been investigated in the mouse infection model of K. pneumoniae [Citation6,Citation31]. To further determine the protective effects of p-YidR and the effect of IL-17 as an adjuvant against K. pneumoniae, the lungs and BAL of immunized mice were selected for the detection of residual bacteria. Our findings were similar to those of a previous study in which large amounts of residual bacteria were determined in the lungs and BAL samples of control mice [Citation6,Citation37]. Conversely, mice immunized with DNA vaccines displayed fewer bacteria in the lungs and BAL, suggesting that the majority of invasive K. pneumoniae were eliminated from the vaccinated mice. Intriguingly, immunization with p-IL17 also decreased the bacterial burdens compared to that in the PBS group, although the mice did not survive the challenge at 7 days. A possible explanation for this is that IL-17 or its related signaling pathway plays an important role in host immunity against K. pneumoniae [Citation18,Citation19]. In addition, immunization with DNA vaccines significantly promoted the survival of KPWT-infected mice. Maximum survivability was detected with the combined vaccination of p-YidR+p-IL17. Furthermore, we found that less inflammation of lungs was observed in the combined group p-yidR+p-IL-17 than in the p-IL17-treated groups, because a stronger immune response was activated by the p-yidR+p-IL-17 vaccination and most of invasive bacteria were killed. Although the trend of suggests p-yidR+p-IL-17 is better than p-yidR, the difference is not significant. A possible explanation is that the similar immune responses or immune defenses were activated in the p-yidR+p-IL-17 treated group and p-yidR treated group on day 2 post-infection. However, more comprehensive studies are necessary to further investigate the reasons about the histology score between the p-yidR+p-IL-17 group and p-yidR group is not significantly different. Taken together, our work further confirmed that this novel DNA vaccine in conjunction with molecular adjuvants induced effective immune responses and provided global protection against K. pneumoniae infections.
In conclusion, our study clearly indicated that p-YidR elicited strong adaptive immune responses and protected immunized mice against K. pneumoniae infections. Notably, co-immunization with p-YidR and p-IL17 remarkably improved immunogenicity and increased the survival rate of mice infected with K. pneumoniae. These findings suggest that the combination of molecular adjuvant IL-17 and novel DNA antigens is an efficacious approach for the development of the next-generation K. pneumoniae vaccines. Experiments are planned to evaluate the protective efficacy of the vaccines in a sublethal infection model or to determine the combination vaccination strategy in other mammalian models in the future will extend a deeper understanding of combating K. pneumoniae related diseases.
Author contributions
TH supervised the project; TH and ZL designed the experiments; ZL and XZ performed the experiments; LMD, KLZ, and XRW provided resources; TH and ZL analyzed the data and wrote the manuscript; TH, KLZ, and YWC provided funding. All authors have read and agreed to the final manuscript.
Figure S1.tif
Download TIFF Image (2.5 MB)Acknowledgements
The authors would like to thank Xiting Yang of Chu’s lab for the helpful support.
Disclosure statement
No potential conflict of interest was reported by the author(s).
Data availability statement
The authors confirm that the data supporting the findings of this study are available within the article and its supplementary materials.
Supplementary material
Supplemental data for this article can be accessed online at https://doi.org/10.1080/21505594.2024.2345019
Additional information
Funding
References
- Wyres KL, Lam MMC, Holt KE. Population genomics of Klebsiella pneumoniae. Nat Rev Microbiol. 2020;18(6):344–12. doi: 10.1038/s41579-019-0315-1
- Huang T, Lv Z, Lin J, et al. A potent antibiotic combination of linezolid and polymycin b nonapeptide against Klebsiella pneumoniae infection in vitro and in vivo. Front Pharmacol. 2022;13:887941. doi: 10.3389/fphar.2022.887941
- Paczosa MK, Mecsas J. Klebsiella pneumoniae: going on the offense with a strong defense. Microbiol Mol Biol Rev. 2016;80(3):629–661. doi: 10.1128/MMBR.00078-15
- Martin RM, Colonization BM. Infection, and the accessory genome of Klebsiella pneumoniae. Front Cell Infect Microbiol. 2018;8:4. doi: 10.3389/fcimb.2018.00004
- Tan S, Gao J, Li Q, et al. Synergistic effect of chlorogenic acid and levofloxacin against Klebsiella pneumonia infection in vitro and in vivo. Sci Rep. 2020;10(1):20013. doi: 10.1038/s41598-020-76895-5
- Rodrigues MX, Yang Y, de Souza Meira EB, et al. Development and evaluation of a new recombinant protein vaccine (YidR) against Klebsiella pneumoniae infection. Vaccine. 2020;38(29):4640–4648. doi: 10.1016/j.vaccine.2020.03.057
- Feldman MF, Mayer Bridwell AE, Scott NE, et al. A promising bioconjugate vaccine against hypervirulent Klebsiella pneumoniae. Proc Natl Acad Sci USA. 2019;116(37):18655–18663. doi: 10.1073/pnas.1907833116
- Assoni L, Girardello R, Converso TR, et al. Current stage in the development of Klebsiella pneumoniae vaccines. Infect Dis Ther. 2021;10(4):2157–2175. doi: 10.1007/s40121-021-00533-4
- Choi M, Tennant SM, Simon R, et al. Progress towards the development of klebsiella vaccines. Expert Rev Vaccines. 2019;18(7):681–691. doi: 10.1080/14760584.2019.1635460
- Ahmad TA, El-Sayed LH, Haroun M, et al. El Ashry el SH. Development of immunization trials against Klebsiella pneumoniae. Vaccine. 2012;30(14):2411–2420. doi: 10.1016/j.vaccine.2011.11.027
- Datta D, Bansal GP, Gerloff DL, et al. Immunogenicity and malaria transmission reducing potency of Pfs48/45 and Pfs25 encoded by DNA vaccines administered by intramuscular electroporation. Vaccine. 2017;35(2):264–272. doi: 10.1016/j.vaccine.2016.11.072
- Huang T, Zhao K, Song X, et al. Heterologous prime-boost immunization with DNA vaccine and modified recombinant proteins enhances immune response against Trueperella pyogenes in mice. Vaccines (Basel). 2022;10(6):839. doi: 10.3390/vaccines10060839
- Silveira MM, Moreira G, Mendonça M. DNA vaccines against COVID-19: perspectives and challenges. Life Sci. 2021;267:118919. doi: 10.1016/j.lfs.2020.118919
- Li L, Petrovsky N. Molecular mechanisms for enhanced DNA vaccine immunogenicity. Expert Rev Vaccines. 2016;15(3):313–329. doi: 10.1586/14760584.2016.1124762
- Kurupati P, Ramachandran NP, Poh CL. Protective efficacy of DNA vaccines encoding outer membrane protein a and OmpK36 of Klebsiella pneumoniae in mice. Clin Vaccine Immunol. 2011;18(1):82–88. doi: 10.1128/CVI.00275-10
- Yang Y, Higgins CH, Rehman I, et al. Genomic diversity, virulence, and antimicrobial resistance of klebsiella pneumoniae strains from cows and humans. Appl Environ Microbiol. 2019;85(6):e02654–18. doi: 10.1128/AEM.02654-18
- Lin JS, Kummer LW, Szaba FM, et al. IL-17 contributes to cell-mediated defense against pulmonary yersinia pestis infection. J Immunol. 2011;186(3):1675–1684. doi: 10.4049/jimmunol.1003303
- Chen K, Eddens T, Trevejo-Nunez G, et al. IL-17 receptor signaling in the lung epithelium is required for mucosal chemokine gradients and pulmonary host defense against K. pneumoniae. Cell Host Microbe. 2016;20(5):596–605. doi: 10.1016/j.chom.2016.10.003
- Iwanaga N, Chen K, Yang H, et al. Vaccine-driven lung TRM cells provide immunity against Klebsiella via fibroblast IL-17R signaling. Sci Immunol. 2021;6(63):eabf1198. doi: 10.1126/sciimmunol.abf1198
- Nemattalab M, Shenagari M, Taheri M, et al. Co-expression of Interleukin-17A molecular adjuvant and prophylactic Helicobacter pylori genetic vaccine could cause sterile immunity in Treg suppressed mice. Cytokine. 2020;126:154866. doi: 10.1016/j.cyto.2019.154866
- Gonçalves VS, Santos FDS, Dos Santos Junior AG, et al. Recombinant bovine IL17A acts as an adjuvant for bovine herpesvirus vaccine. Res Vet Sci. 2021;136:185–191. doi: 10.1016/j.rvsc.2021.02.014
- Zhao K, Li W, Li J, et al. TesG is a type I secretion effector of Pseudomonas aeruginosa that suppresses the host immune response during chronic infection. Nat Microbiol. 2019;4(3):459–469. doi: 10.1038/s41564-018-0322-4
- Huang T, Zhao K, Zhang Z, et al. DNA vaccination based on pyolysin co-immunized with IL-1β enhances host antibacterial immunity against Trueperella pyogenes infection. Vaccine. 2016;34(30):3469–3477. doi: 10.1016/j.vaccine.2016.04.025
- Chehelgerdi M, Doosti A. Effect of the cagW-based gene vaccine on the immunologic properties of BALB/c mouse: an efficient candidate for Helicobacter pylori DNA vaccine. J Nanobiotechnology. 2020;18(1):63. doi: 10.1186/s12951-020-00618-1
- Wang Q, Sun X, Huang X, et al. Nanoparticles of chitosan/poly(D,L-Lactide-Co-Glycolide) enhanced the immune responses of Haemonchus contortus HCA59 antigen in model mice. Int J Nanomedicine. 2021;16:3125–3139. doi: 10.2147/IJN.S301851
- Boettler T, Csernalabics B, Salié H, et al. SARS-CoV-2 vaccination can elicit a CD8 T-cell dominant hepatitis. J Hepatol. 2022;77(3):653–659. doi: 10.1016/j.jhep.2022.03.040
- Huang T, Song X, Jing J, et al. Chitosan-DNA nanoparticles enhanced the immunogenicity of multivalent DNA vaccination on mice against Trueperella pyogenes infection. J Nanobiotechnology. 2018;16(1):8. doi: 10.1186/s12951-018-0337-2
- Facchini M, De Fino I, Riva C, et al. Long term chronic Pseudomonas aeruginosa airway infection in mice. J Vis Exp. 2014;2014(85). doi: 10.3791/51019-v
- Huang T, Pu Q, Zhou C, et al. MicroRNA-302/367 Cluster impacts host antimicrobial defense via regulation of mitophagic response against Pseudomonas aeruginosa Infection. Front Immunol. 2020;11:569173. doi: 10.3389/fimmu.2020.569173
- Bergamini G, Perico ME, Di Palma S, et al. Mouse pneumonia model by Acinetobacter baumannii multidrug resistant strains: comparison between intranasal inoculation, intratracheal instillation and oropharyngeal aspiration techniques. PLOS ONE. 2021;16(12):e0260627. doi: 10.1371/journal.pone.0260627
- Li X, Zhou X, Ye Y, et al. Lyn regulates inflammatory responses in Klebsiella pneumoniae infection via the p38/NF-κB pathway. Eur J Immunol. 2014;44(3):763–773. doi: 10.1002/eji.201343972
- Eveillard M, Soltner C, Kempf M, et al. The virulence variability of different acinetobacter baumannii strains in experimental pneumonia. J Infect. 2010;60(2):154–161. doi: 10.1016/j.jinf.2009.09.004
- Lundberg U, Senn BM, Schüler W, et al. Identification and characterization of antigens as vaccine candidates against Klebsiella pneumoniae. Hum Vaccin Immunother. 2013;9(3):497–505. doi: 10.4161/hv.23225
- Lee WH, Choi HI, Hong SW, et al. Vaccination with Klebsiella pneumoniae-derived extracellular vesicles protects against bacteria-induced lethality via both humoral and cellular immunity. Exp Mol Med. 2015;47(9):e183. doi: 10.1038/emm.2015.59
- Berretta F, St-Pierre J, Piccirillo CA, et al. IL-2 contributes to maintaining a balance between CD4+Foxp3+ regulatory T cells and effector CD4+ T cells required for immune control of blood-stage malaria infection. J Immunol. 2011;186(8):4862–4871. doi: 10.4049/jimmunol.1003777
- Soni B, Singh S. Cytokine milieu in infectious disease: a sword or a boon? J Interferon Cytokine Res. 2020;40(1):24–32. doi: 10.1089/jir.2019.0089
- Rangasamy T, Ghimire L, Jin L, et al. Host Defense against Klebsiella pneumoniae pneumonia is augmented by lung-derived mesenchymal stem cells. J Immunol. 2021;207(4):1112–1127. doi: 10.4049/jimmunol.2000688