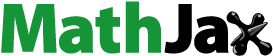
Abstract
The forest distribution in relation to mountain slope position and the relationships between forest canopy area and soil physical and chemical properties in Mongolia attract the attention of scientists. In this study, we aimed to determine (1) how the slope aspect affects the forest soil physical and chemical properties, (2) how the soil variables correlate with projected canopy area. For comparative study, we established a total of 18 (20 × 25 m2 sized) sample plots in upper forest edge (UFE), upper slope of the forest (USF), lower slope of the forest (LSF), lower forest edge (LFE), sparse forest (SF), and degraded forest area (DFA) stand types with three replications. We measured the tree height, diameter and crown projection area of each growing tree in the sample plots, and collected soil samples for further soil physical and chemical analyses in the laboratory. Our results showed a high variation in growing stock (p < 0.0001), stand density and projected canopy area (p < 0.0001) in relation to mountain slope position and land-use management. We found that the slight changes in stand canopy area projection cause a high variation in soil physical and chemical properties. Among studied stand types higher means of soil temperature and bulk density and lower moisture content were observed on SF and DFA stand types. Contrary, relatively higher content of soil moisture and nutrient elements were recorded in USF, LSF, LFE. We conclude that tree canopy area becomes key determinant factor that positively influences forest soil moisture and fertility by increasing organic matter content in Mongolia.
1. Introduction
Soils play an important role in forest formation, productivity and ecosystem sustainability (Bennett and Barton Citation2018; FAO Citation2018; Sukhbaatar et al. Citation2019). Scientists highlighted that soil properties are a consequence of past land-use management and can determine the site quality in different ecosystems (Kim et al. Citation2005; Kang et al. Citation2023). Several studies (Sefidi et al. Citation2020; Ehbrecht et al. Citation2021) have emphasized that water availability in forest soils is one of the main determinant factors of forest productivity and stand structure. In forested areas, tree canopies reduce soil moisture loss (Zhang et al. Citation2018) and improve soil nutrient status (Vale et al. Citation2013; Dinca et al. Citation2018). Several studies have reported that forest conservation and successful forest rehabilitation are the main tools for mitigating the effects of accelerated global warming and deforestation worldwide (Brockerhoff et al. Citation2017; Bennett and Barton Citation2018; Oral Citation2020). Continuous forest degradation and deforestation caused by overexploitation, fires, pests, and climate change have resulted in soil degradation and reduction in soil water availability not only in arid regions (Gichuho et al. Citation2013; Akbulut Citation2014; Zhang et al. Citation2018), but also in humid regions (Exbrayat et al. Citation2017). Moreover, high-intensity logging and clearcutting in Mongolia trigger rapid soil water loss and lead to soil compaction, especially in the surface layer (Nemček-Korenkova and Urík Citation2012; Sukhbaatar et al. Citation2018, Citation2019). However, Ehbrecht et al. (Citation2021) highlighted that the measures of stand structural complexity are strong predictors of net primary productivity because the important drivers of forest growth, such as occupied canopy space connectedness and light absorption, are accounted for in structural complexity metrics. Recent studies (Missanjo and Kamanga-Thole Citation2014; Zhou et al. Citation2015; Behjou and Mollabashi Citation2016) have noted that the removal of a number of trees and their canopies from a forest most likely caused high fluctuations in air and soil temperature as a consequence of increased direct sunlight and soil water evaporation.
Forests must be managed in ways that maintain their productive capacity, as well as the nutrient reserves in the soils, which facilitate biomass accumulation in forest ecosystems (Venterea et al. Citation2003; Tsui et al. Citation2004; Hansson et al. Citation2020). In temperate forests, compaction due to timber harvesting has significant consequences on tree regeneration and forest productivity (Saarsalmi et al. Citation2010). Soil physical and chemical properties are important for tree growth and forest biomass production. Soil bulk density, porosity, and temperature reflect the soil ability to provide structural support and facilitate the flow of water, solutes, and air (Tan et al. Citation2009; Missanjo and Kamanga-Thole Citation2014). As a result, water deficit and raised soil temperature eventually lead to the formation of compact and nutrient-poor soils (Zhou et al. Citation2015). In semi-arid environments, changes in forest canopy and microclimate could create preconditions for forest succession and shift of the forest into a non-forest area.
The accelerated forest degradation and deforestation in Central Asia are closely linked to low water availability and frequent droughts (Gradel et al. Citation2019; Sukhbaatar et al. Citation2021). Mongolia has a relatively low amount of forest resources, with forests accounting for only 11.8% of the country’s territory. Boreal forests in Mongolia mainly grow on north-facing mountain slopes between 700 and 2500 m a.s.l., coinciding with the seasonal permafrost distribution in the country. Geographically, these forests belong to the transition zone between the Great Siberian Taiga forests and the Central Asian dry steppe and grow under harsh continental semi-humid and semi-arid climate conditions.
Reason for this study (e.g. the lack of studies on the effect of canopy area on soil properties, necessity for determining key factor of soil physicochemical properties among many variables).
Despite the importance of boreal forests in soil conservation and their fertility status in Mongolia, few information is available on determining the effect of stand tree canopy projection area and mountain slope position on soil physical and chemical properties for consideration of further forest restoration, soil conservation and water resource management in the study region.
The main objectives of this study were to determine the following: (1) how slope location affects the physical and chemical qualities of forest soil; and (2) how soil factors correspond with projected canopy area.
2. Materials and methods
2.1. Study area
The present study was carried out in natural Betula platyphylla dominated forests in the Hustai Mountains (47°43′–47°60′E, 105°51′–106°51′N) of Tuv Province, Mongolia (). These forests are part of the southernmost limit of boreal forest distribution in Mongolia and directly border the dry steppe. According to the Köppen-Geiger World Climate Classification Map, in the study area exists a cold semiarid climate (Bsk). According to climate observations over the last 10 years, the average air temperature and precipitation are 0.2 °C and 222 mm, respectively, with precipitation peaking between July and August (). The dry season extends from March to June in spring, and from August to October in autumn. The soils in the study area are Mollic umbrisols and Kastanozems. Forests growing in the study area are characterized by high sensitivity to drought and logging regimes. A total of 451 vascular plants have been registered in Khustai.
2.2. Sampling design and field measurements
The study was conducted in July 2020. Sample plots were selected considering the canopy area and their location on the mountain elevation within the forest distribution area (1550–1646 m a.s.l.). The sampling design included the upper forest edge (UFE), upper slope of the forest (USF), lower slope of the forest (LSF), lower forest edge (LFE), sparse forest (SF), and degraded forest area (DFA), with the aim of detecting the different effects of stand density and slope on the soil physical and chemical properties in the forested area. A total of 18 sample plots (20 × 25 m2 sized) were established using a completely randomized selection method. The growing conditions of the sampled stands were similar, and all sample plots were established on the north-facing slopes of the mountain at the elevation range between 1550 and 1620 m a.s.l.
In these plots, stem diameter at breast height (DBH), height, height up to the lowest live branch, and distance between trees were measured using a hypsometer, digital caliper, Vertex IV, and measuring tape, respectively. To visualize the spatial distribution of each tree and estimate their canopy projection area, the crown diameter was measured in four directions (north, east, south, and west) with measuring tape. The growing stock was determined based on tree stem volume estimation for standing trees in the sample plots (Altrell Citation2019). Soil samples were taken using soil auger (Air-Met Scientific) in each of the sample plots from different soil depths (depending on varying thickness) of each soil horizon in the profiles with five replicates. Soil temperature, moisture were measured every 4 h for 3 days using a soil Checktemp® digital thermometer (Hanna Instruments, Australia), HT5204 moisture meter. Soil pressure was measured once at the moment of soil sampling using DIK5553 soil hardness meter. The soil samples were placed in labeled polyethylene bags and stored in a refrigerator at 3 °C during the field survey.
2.3. Laboratory measurements and analyses
The soil samples were oven-dried at 65 °C for 24 h. They were then sieved through a 2 mm sieve prior to wet-sieving for homogenization and removal of large roots and stones (Ma et al. Citation2023). In the laboratory, the following chemical properties were analyzed: soil pH, electrical conductivity, organic matter content, loss of ignition (LOI), nitrogen dioxide (NO2) concentration, nitrate (NO3) concentration, exchangeable cation (CaO and MgO) concentrations, available potassium (K2O) concentration, and available phosphorus (P2O5) concentration (Zalamea et al. Citation2016; Oyuntsetseg et al. Citation2019). Soil humus and organic matter contents were determined according to Ivanov et al. (Citation2017) and loss on ignition method (LOI) (Oyuntsetseg et al. Citation2019; Hoogsteen et al. Citation2015), respectively.
2.4. Statistical analysis
The canopy area (CPA) for each tree was estimated according to Sukhbaatar et al. (Citation2019)
(1)
(1)
where: CPA is the tree canopy area, CD is the crown diameter of the individual tree,
is the mathematical constant pi (equaling 3.14).
Soil moisture content was determined using EquationEquation (2)(2)
(2) :
(2)
(2)
XLSTAT (Addinsoft, France) was used for statistical analyses. One-way analysis of variance (ANOVA) was used to evaluate the significant differences among studied variables for the comparison. The degrees of freedom (DF), mean squares of error (MSE), sum of squares, mean squares, F-statistic, and P-value of the F statistics (Pr > F) were analyzed using one-way ANOVA. The Duncan’s multiple range test was used for multiple comparisons of means. In addition, Pearson’s correlation coefficients were applied to assess relationships between variables, and principal component analysis (PCA) was used for exploratory data analysis and predictive modeling of crown projection areas and soil properties.
3. Results
3.1. Comparison of the main stand characteristics in different stand types
The main characteristics of the sampled stands are listed in . Our results showed a high variation in stand characteristics among stand types (). In terms of stand age, all Betula platyphilla stands belonged to mature classes, ranging from 53 (DFA) to 71 (SF) years of age. Site quality/bonitet class was determined based on tree age and height. There are five main stands of quality classes from I to V, where stands of quality class I are more productive than following classes. The poorest stands belong to class V. However, stands of quality class Va are more productive than those of class Vb ().
Table 1. Stand characteristics of the inventoried birch forests. For each stand variable, the mean and standard deviation are shown in the same column.
Table 2. Results of one-way ANOVA for some stand variables among the selected stands. DF is the degrees of freedom; MSE is the mean squared error.
The results showe relatively low growing stocks which did not exceed 65.2 m3 ha−1, as well as a very high dead wood stock in the sampled stands. The growing stock varied greatly among the stand types (p < 0.0001), and the lowest stock was observed in SF (9.2 m3 ha−1). In comparison, the greater means of canopy area and crown diameter, tree height and DBH were observed in LSF and LEE stand types that were established lower slope positions of the mountain (). Therefore, we found relatively high stand density (1420 stems ha−1 in UFE) and growing stock (61.3 m3 ha−1 in USF) in upper positions of the forest distribution. Contrary, the lowest mean of growing stock (9.2 m3 ha−1) and canopy area (41.8 m2 ha−1) were recorded in SF and DFA which caused by high intensity harvesting. We found better growth and crown development of trees only in middle and lower stand types. According to the site quality index, all studied stands belonged to the poorest quality class (Va and Vb) () owing to their geographical location and limited growing conditions.
3.2. Changes in soil physical properties
The correlations between tree canopy area and some of soil physical and chemical properties are shown in . Among the main tested stand variables, tree canopy area was the key determinant factor on soil properties. Increase in canopy area was positively correlated with soil moisture content (r = 0.37) and organic matter (r = 0.58), whereas it was negatively correlated with soil temperature (r = −0.72), pressure (r = −0.85), humus (r = −0.38), and EC (r = −0.31) (). In addition, growing stock was also positively correlated with soil moisture content (r = 0.73) which is influenced to tree growth.
Figure 2. Relationships between stand canopy area and soil physical properties. The vertical axis indicates the mean of the canopy area, and the horizontal axis indicates the means of (a) soil moisture, (b) soil temperature, (c) soil pressure, (d) organic matter content, (e) humus content, and (f) Electrical conductivity (EC).

Relatively higher means of soil temperature and pressure were recorded in DFA and SF, where canopy area was less than 145.8 m2 ha−1. However, compared to those in the other stand types, higher soil moisture and lower temperature values were observed in the LFE and LSF stand types at lower positions on the mountain slope ().
In contrast, the lowest canopy area in the DFA (41.8 m2 ha−1) resulted in the lowest moisture content as well as the highest temperature and pressure in the soils among the selected stands (). The most of soil properties were found to be positively correlated with soil moisture content (). Thus, it can be concluded that decrease in stand canopy area triggered a rapid soil compaction in the study area owing to insufficient moisture and increased temperature in the soils.
Table 3. Pearson’s correlations between soil properties.
3.3. Comparison of soil chemical properties in different stand types
Results of the chemical analysis are shown in . Clay mineral and organic matter components in the soil have negatively charged sites on their surfaces, which adsorb and hold positively charged ions (cations) by electrostatic forces. This electrical charge is critical for the nutrient supply. The main ions in the soil are exchangeable cations of calcium (Ca2+) and magnesium (Mg2+), and they are generally referred to as the base cations (Lavelle and Spain Citation2007; Rayment and Lyons Citation2010).
Furthermore, we found a gradual deterioration in soil chemical properties with the decrease in stand density. In comparison, soil nutrient content was often higher in the lower (LSF and LFE) positions of the mountain slope than in the higher ones (). The stands with relatively low stand densities had a low soil nutrient content. Noticeable negative effects of low stand density on soil chemical properties were observed in the SF and DFA stand types, where the contents of humus, organic matter, nitrate, and available potassium were lower than those in the denser stand types. Overall, most of these chemical properties were positively correlated with stand canopy area, and the correlation coefficient ranged between 0.75 and 0.83 (with p < 0.05). Consequently, our results indicated that forest degradation and reduction in stand canopy area resulted in a lower soil nutrient content.
Table 4. Soil chemical properties in different stand types.
We examined relationship between stand canopy area and some macro nutrient elements of soil. Our results carried out canopy area is one of the critical factor of soil chemical properties. Canopy area was positively correlated with soil nitrate (r = 0.78), phosphorus (r = 0.42), potassium (r = 0.62) and negatively correlated with magnesium (r= −0.37) ().
Figure 3. Relationships between stand canopy area and soil macronutrient elements. The vertical axis indicates the mean of the canopy area, and the horizontal axis indicates the means of (a) nitrate (NO3-), b) phosphorus (P), (c) potassium (K), (d) calcium (Ca), (e) magnesium (Mg), and (f) sodium (Na).

The eigenvalues and cumulative percentage of variance for each component are described (). The results showed that there were significant relationships between the factors, with F1 and F2 being the most prolific, with eigenvalues greater than 1. These principal components accounted for 69.14% of the total variation. The percentages of eigenvalues for the first and second axis were 45.33 and 23.81%, respectively. Specifically, the first principal axis was positively correlated with canopy area, basal area, growing stock, tree height, and tree diameter, and the second principal axis was positively correlated with soil moisture and soil EC, and negatively correlated with soil humus and soil pressure ().
Figure 4. Distributions of soil properties and forest cover parameters according to PCA. (a) A scree plot showing the relative eigenvalues for the principal components for the measured variables. (b) The diagram of PCA ordination biplot was scaled by 1 with a focus on soil moisture (%), soil electrical conductivity (EC) (µS cm−1), soil pH, soil humus (%), soil pressure (kPa), crown projection area (CPA, m2), tree height (H, m), growing stock (GS, m3), tree diameter (D, cm), and basal area (m2).

4. Discussion
4.1. Soil nutrients and water availability are sensitive to stand density
The six study sites are situated in different topographical conditions, which could be major factors influencing soil properties. Our comparative study suggests that forest soil properties are likely to be influenced by forest density and crown area. We detected a high sensitivity of forest soil properties to small changes in stand density and canopy area. Several studies have reported that ground-based logging and the removal of tree biomass can cause serious disturbances to soil properties as a result of soil compaction and nutrient loss (Missanjo and Kamanga-Thole Citation2014; Hosseini et al. Citation2015; Zhou et al. Citation2015). For instance, the physical (soil texture), chemical (soil reactions), and biological (microbial activity) characteristics of the soil can significantly affect the status, turnover, and subsequent nutrient uptake by plants (Bünemann and Condron Citation2007; Alamgir and Marschner Citation2013). Our results supported this idea and showed that the highly intensive removal of tree biomass in the DFA and SF stand types significantly affected soil bulk density, temperature, and moisture content (). Our findings are similar to those of previous studies (Hashimoto and Suzuki Citation2004; Ares et al. Citation2005; Twedt and Daniel Citation2012; Sukhbaatar et al. Citation2019), who reported that the absence of shading or reduction in shading in intensively logged areas generally leads to increases in soil temperature and compaction.
Moreover, Zhou et al. (Citation2015) obtained similar results in mixed forests of northeastern China, where a reduction in forest stand density and tree biomass resulted in a decrease in soil organic matter, porosity, and water-holding capacity. In our study, critically low soil moisture content was observed only in the soils of the DFA (<9.5%) and SF (<7%) stand types, and this was apparent up to 80 cm of depth. However, relatively higher means of soil moisture content (>12.3%) were recorded in all other stand types ().
4.2. Decrease in the forest canopy area causes soil degradation in Mongolia
Mongolia is currently one of the ten countries most affected by global warming and climate change in the world. According to the Desertification Map of Mongolia (2020), 76.9% (120.3 million ha) of the country’s territory is affected by land degradation and desertification. This pattern shows the importance of soil protection management strategies for conserving forest ecosystems against land degradation and desertification in the region. Moreover, several studies have emphasized that intensive logging and the removal of woody biomass from forests have a serious negative impact not only on the restoration of forest ecosystems (Allen et al. Citation2010; Akbulut Citation2014; Sukhbaatar et al. Citation2019), but also on soil properties (Hajabbasi et al. Citation1997; Jang et al. Citation2016).
Our findings demonstrated that forest soils in the study area are quite sensitive to slight changes in stand canopy areas, which is the main determining factor of changes in soil physical and chemical properties. For this reason, the absence and insufficient crown area in the DFA and SF stand types led to soil compaction and nutrient loss. In comparison, in denser stands with a greater canopy area, we observed relatively lower temperatures and higher soil moisture and nutrient content compared to those in less dense stands with lower canopy areas ( and ). The increased evaporation and loss of nutrients in the soils because of weak shading from the stand and relatively small amount of rainfall create ideal conditions for land degradation in the study area.
The soil microbial community is critical for maintaining forest ecosystem functioning (Wall et al. Citation2015; Fierer Citation2017), including carbon storage in the soil (Liang et al. Citation2017) and humification (Mitchell et al. Citation2010), and is sensitive to environmental changes (He et al. Citation2019). As a result, the stand types with higher canopy areas (UFE, USF, LSF, and LFE) led to relatively higher soil nutrient concentrations and better physical properties than those of the SF and DFA stand types. Such a suitable environment for the microbial community in forested areas can lead to intensified decomposition of dead organic matter and humification.
In general, the threat of forest degradation and deforestation caused by frequent fires, overexploitation, and overgrazing may ultimately cause large-scale land degradation and expansion of desertified areas in Mongolia. Effective forest protection and afforestation measures are necessary to avoid the potential risks of deforestation and land degradation at the southern boundary of boreal forests in Mongolia.
5. Conclusion
In this study, we examined the correlation between the canopy area and soil moisture, temperature, and pressure. Our results showed that canopy area was one of the factors that induced changes in soil physical properties. Significantly low moisture content was observed in soils up to 80 cm depth DFA (<9.5%) and SF (<7%). It can be assumed that soil moisture loss contributes to land degradation. Furthermore, we determined the relationship between forest measurements and soil parameters using PCA. The key components accounted for 69.14% of the total variation. Our results also showed that the forest soils of the study area were highly sensitive to small changes in canopy area, which was a key factor determining the changes in the soil physical and chemical properties. These results contribute to our understanding for the management and restoration of forest ecosystems as well as how soil has changed under climate change and forest degradation.
Author contributions
Otgontuya Tsogbadrakh was responsible for research design and conceptualization of the study. Bolormaa Oyuntsetseg and Kyoung-Woong Kim were responsible for funding acquisition and project administration. Otgontuya Tsogbadrakh, Bolormaa Oyuntsetseg, Gerelbaatar Sukhbaatar, Burmaa Batchuluun, Delgermaa Altanjin, and Kah Yee Seah collected and processed the data. Otgontuya Tsogbadrakh, Bolormaa Oyuntsetseg, and Gerelbaatar Sukhbaatar drafted the manuscript.
Acknowledgments
The authors are grateful to the Ministry of Science and Technology in South Korea through the International Environmental Research Institute (IERI) of Gwangju Institute of Science and Technology (GIST) for providing us with the funds in 2020 that facilitated the delivery of the program. We also thank the Administration of the Khustai National Park and the students who participated in field data collection and laboratory measurements.
Disclosure statement
No potential conflict of interest was reported by the authors.
Additional information
Funding
References
- Akbulut A. 2014. Environmental degradation as a security threat: the challenge for developing countries. IJHS. 11(1):1227–1237. doi: 10.14687/ijhs.v11i1.2894.
- Alamgir M, Marschner P. 2013. Changes in phosphorus pools in three soils upon addition of legume residues differing in carbon/phosphorus ratio. Soil Res. 51(6):484–493. doi: 10.1071/SR12378.
- Allen CD, Macalady AK, Chenchouni H, Bachelet D, McDowell N, Vennetier M, Kitzberger T, Rigling A, Breshears DD, Hogg E, et al. 2010. A global overview of drought and heat-induced tree mortality reveals emerging climate change risks for forests. Forest Ecol Manage. 259(4):660–684. doi: 10.1016/j.foreco.2009.09.001.
- Altrell D. 2019. Multipurpose National Forest Inventory in Mongolia, 2014-2017: a tool to support sustainable forest management. GES. 12(3):167–183. doi: 10.24057/2071-9388-2019-36.
- Ares A, Terry T, Miller R, Anderson H, Flaming B. 2005. Ground-based forest harvesting effects on soil physical properties and Douglas-fir growth. Soil Science Soc of Amer J. 69(6):1822–1832. doi: 10.2136/sssaj2004.0331.
- Behjou F, Mollabashi O. 2016. Impact of logging intensity on stem density, basal area and biodiversity indices five years after logging in a Caspian hardwood forest. J Forest Sci. 63:167–172. doi: 10.17221/103/2016-JFS.
- Bennett B, Barton G. 2018. The enduring link between forest cover and rainfall: a historical perspective on science and policy discussions. For Ecosyst. 5(1-9). doi: 10.1186/s40663-017-0124-9.
- Brockerhoff EG, Barbaro L, Castagneyrol B, Forrester DI, Gardiner B, González-Olabarria JR, Lyver PO, Meurisse N, Oxbrough A, Taki H, et al. 2017. Forest biodiversity, ecosystem functioning and the provision of ecosystem services. Biodivers Conserv. 26(13):3005–3035. doi: 10.1007/s10531-017-1453-2.
- Bünemann EK, Condron LM. 2007. Phosphorus and sulphur cycling in terrestrial ecosystems. In Petra Marschner and Zdenko Rengel, editors. Nutrient cycling in terrestrial ecosystems. Soil Biology, vol. 10. Berlin, Heidelberg: Springer Berlin Heidelberg; p. 65–92. doi: 10.1007/978-3-540-68027-7_3.
- Dinca L, Badea O, Guiman G, Braga C, Crisan V, Greavu V, Murariu G, Georgescu L. 2018. Monitoring of soil moisture in long-term ecological research (LTER) sites of Romanian Carpathians. Ann Res. 61(2):171–188. doi: 10.15287/afr.2018.1188.
- Ehbrecht M, Seidel D, Annighöfer P, Kreft H, Köhler M, Zemp DC, Puettmann K, Nilus R, Babweteera F, Willim K, et al. 2021. Global patterns and climatic controls of forest structural complexity. Nat Commun. 12(1):519. doi: 10.1038/s41467-020-20767-z.
- Exbrayat J-F, Liu Y, Williams M. 2017. Impact of deforestation and climate on the Amazon basin’s above-ground biomass during 1993–2012. Sci Rep. 7(1):15615. doi: 10.1038/s41598-017-15788-6.
- FAO. 2018. The State of the World’s Forests 2018: forest Pathways to Sustainable Development. The State of the World’s Forests (SOFO) 2018. Rome, Italy: FAO. http://www.fao.org/documents/card/en/c/I9535EN/.
- Fierer N. 2017. Embracing the unknown: disentangling the complexities of the soil microbiome. Nat Rev Microbiol. 15(10):579–590. doi: 10.1038/nrmicro.2017.87.
- Gichuho C, Njoroge S, Wambui W. 2013. Land cover change and deforestation in Gazetted Maji Mazuri Forest, Kenya. Int J Sci Res (IJSR). 2(April):563–566.
- Gradel A, Sukhbaatar G, Karthe D, Kang H. 2019. Forest management In Mongolia – a review of challenges and lessons learned with special reference to degradation and deforestation. GES. 12(3):133–166. doi: 10.24057/2071-9388-2019-102.
- Hajabbasi M, Jalalian A, Karimzadeh H. 1997. Deforestation effects on soil physical and chemical properties, Lordegan, Iran. Plant Soil. 190(2):301–308. doi: 10.1023/A:1004243702208.
- Hansson K, Laclau J-P, Saint-André L, Mareschal L, van der Heijden G, Nys C, Nicolas M, Ranger J, Legout A. 2020. Chemical fertility of forest ecosystems. Part 1: common soil chemical analyses were poor predictors of stand productivity across a wide range of acidic forest soils. Forest Ecol Manag. 461(April):117843. doi: 10.1016/j.foreco.2019.117843.
- Hashimoto S, Suzuki M. 2004. The impact of forest clear-cutting on soil temperature: a comparison between before and after cutting, and between clear-cut and control sites. J Forest Res. 9(2):125–132. doi: 10.1007/s10310-003-0063-x.
- He X, Hou E, Veen G, Ellwood F, Dijkstra P, Sui X, Zhang S, Wen D, Chu C. 2019. Soil microbial biomass increases along elevational gradients in the tropics and subtropics but not elsewhere. Global Ecol Biogeogr. 29(2):345–354. doi: 10.1111/geb.13017.
- Hoogsteen MJJ, Lantinga EA, Bakker EJ, Groot JCJ, Tittonell PA. 2015. Estimating soil organic carbon through loss on ignition: effects of ignition conditions and structural water loss. Eur J Soil Sci. 66(2):320–328. doi: 10.1111/ejss.12224.
- Hosseini S, Akbarimehr M, Emadian SF, Agh A. 2015. Considering the soil compaction status on logging areas in a Hyrcanian forest. J for Sci. 61(5):210–215. doi: 10.17221/48/2014-JFS.
- Ivanov L, Kogut B, Semenov V, Turina I, Waksman N. 2017. Development of theory on humus and soil organic matter: from Turin and Waksman to Present Days. Byull Pochvennogo Inst Im. V.V. Dokuch. 90(Dec):3–38.
- Jang W, Page-Dumroese D, Keyes C. 2016. Long-term soil changes from forest harvesting and residue management in the Northern Rocky Mountains. Soil Sci Soc Am J. 80(3):727–741. doi: 10.2136/sssaj2015.11.0413.
- Kang J, Deng Z, Zhang Z, Chen S, Huang J, Ding X. 2023. Relative importance of soil properties and functional diversity to the spatial pattern of the forest soil nitrogen. Ecol Indicat. 146(Feb):109806. doi: 10.1016/j.ecolind.2022.109806.
- Kim C, Koo K, Byun J, Jeong J. 2005. Post-fire effects on soil properties in red pine (Pinus densiflora) stands. Forest Sci Technol. 1(1):1–7. doi: 10.1080/21580103.2005.9656261.
- Lavelle P, Spain A. 2007. Soil ecology. Spain: Springer Science & Business Media. 654 p
- Liang C, Schimel J, Jastrow J. 2017. The importance of anabolism in microbial control over soil carbon storage. Nat Microbiol. 2(8):17105. doi: 10.1038/nmicrobiol.2017.105.
- Ma S, Song Y, Liu J, Kang X, Yue ZQ. 2023. Extended wet sieving method for determination of complete particle size distribution of general soils. J Rock Mech Geotech Eng. 5(1-16). doi: 10.1016/j.jrmge.2023.03.006.
- Missanjo E, Kamanga-Thole G. 2014. Impact of site disturbances from harvesting and logging on soil physical properties and Pinus Kesiya tree growth. Int Sch Res Notices. 2014(July):323626–323627. doi: 10.1155/2014/323626.
- Mitchell R, Hester A, Campbell C, Chapman S, Cameron C, Hewison R, Potts J. 2010. Is vegetation composition or soil chemistry the best predictor of the soil microbial community? Plant Soil. 333(1-2):417–430. doi: 10.1007/s11104-010-0357-7.
- Nemček-Korenkova L, Urík M. 2012. Soil moisture and its effect on bulk density and porosity of intact aggregates of three mollic soils. Indian J Agri Sci. 82(Feb):172–176.
- Oral HV. 2020. Deforestation. doi: 10.1007/978-3-319-74336-3_390-1.
- Oyuntsetseg B, Yondonjamts J, Bayanjargal O, Prathumratana L, Watanabe M, Kim K-W. 2019. Geochemical source and dispersion of copper, arsenic, lead, and zinc in the topsoil from the vicinity of Erdenet mining area, Mongolia. GEEA. 19(2):110–120. doi: 10.1144/geochem2018-025.
- Rayment GE, Lyons DJ. 2010. Soil chemical methods – Australasia. Australian Soil and Land Survey Handbook Series, Australia: Csiro Publishing.
- Saarsalmi A, Tamminen P, Kukkola M, Hautajärvi R. 2010. Whole-tree harvesting at clear-felling: impact on soil chemistry, needle nutrient concentrations and growth of scots pine. Scand J Forest Res. 25(2):148–156. doi: 10.1080/02827581003667314.
- Sefidi K, Ghavidel A, Esmaeilpour M, Mohammadi S. 2020. Effect of soil physical and chemical properties on tree cover diversity and structure in Marivan Qamyshlh Forests. Forest Wood Prod. 73(2):225–233.
- Sukhbaatar G, Ganbaatar B, Jamsran T, Purevragchaa B, Nachin B, Gradel A. 2019. Assessment of early survival and growth of planted scots pine (Pinus sylvestris) seedlings under extreme continental climate conditions of Northern Mongolia. J For Res. 31(1):13–26. doi: 10.1007/s11676-019-00935-8.
- Sukhbaatar G, Kyoung-Woong K, Lobanov A, Ganbaatar B, Purevragchaa B, Batchuluun T. 2021. Deforestation and degradation of forests in the Khustai Mountains of Northern Mongolia. Sibirskij Lesnoj Žurnal. 2:53–63. doi: 10.15372/SJFS20210205.
- Sukhbaatar G, Nachin B, Purevragchaa B, Ganbaatar B, Mookhor K, Tseveen B, Gradel A. 2019. Which selective logging intensity is most suitable for the maintenance of soil properties and the promotion of natural regeneration in highly continental scots pine forests?–Results 19 years after harvest operations in Mongolia. Forests 10(2):141. doi: 10.3390/f10020141.
- Sukhbaatar G, Suran B, Baatarbileg N, Chultem D. 2018. Effects of scots pine (Pinus sylvestris L.) plantations on plant diversity in Northern Mongolia. Mong. J. Biol. Sci.16:59–70.
- Tan X, Curran M, Chang S, Maynard D. 2009. Early growth responses of Lodgepole pine and Douglas-fir to soil compaction, organic matter removal, and rehabilitation stand types in Southeastern British Columbia. Forest Sci. 55(June):210–220.
- Tsui C-C, Chen Z-S, Hsieh C-F. 2004. Relationships between soil properties and slope position in a lowland rain forest of Southern Taiwan. Geoderma. 123(1-2):131–142. doi: 10.1016/j.geoderma.2004.01.031.
- Twedt D, Daniel J. 2012. Wildlife forestry, Chapter 10. In C. A. Okia, editor, Global perspectives on sustainable forest management, Rijeka, Croatia: INTECH; pp 161–190. ISBN: 978-953-51-0569-5, http://www.Intechopen.Com/Books/Global-Perspectives-on-Sustainable-Forest-Management/Wildlife-Forestry.
- Vale Vagner I, Schiavini G, Araújo A, Gusson S, Lopes A, Paula de Oliveira J, Prado Júnior C, Arantes, O, Neto. 2013. Fast changes in seasonal forest communities due to soil moisture increase after damming. Rev Biol Trop. 61(Dec):1901–1917.
- Venterea R, Lovett G, Groffman P, Schwarz P. 2003. Landscape patterns of net nitrification in a northern hardwood-conifer forest. Soil Sci Soc Am J. 67(2):527–539. doi: 10.2136/sssaj2003.0527.
- Wall D, Nielsen U, Six J. 2015. Soil biodiversity and human health. Nature. 528(7580):69–76. doi: 10.1038/nature15744.
- Zalamea M, González G, Lodge D. 2016. Physical, chemical, and biological properties of soil under decaying wood in a tropical wet forest in Puerto Rico. Forests. 7(12):168. doi: 10.3390/f7080168.
- Zhang J, Wang L, Su J. 2018. The soil water condition of a typical agroforestry system under the policy of Northwest China. Forests. 9(12):730. doi: 10.3390/f9120730.
- Zhou X, Zhou Y, Zhou C, Wu Z, Zheng L, Hu X, Chen H, Gan J. 2015. Effects of cutting intensity on soil physical and chemical properties in a mixed natural forest in Southeastern China. Forests. 6(12):4495–4509. doi: 10.3390/f6124383.