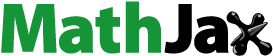
ABSTRACT
Vaccination active, promising alternative immunological strategy to treat of CUD. Various models of cocaine vaccines have been evaluated in animals and humans with relative success. In this sense, it is necessary to improve or optimize the cocaine vaccines already evaluated. Our laboratory previously reported the efficacy of the tetanus toxoid-conjugated morphine vaccine (M6-TT). The M6-TT vaccine can generate high titers of antibodies and reduce heroin-induced behavioral effects in rodents. So, it would be plausible to assume that if we modify the M6-TT vaccine by changing the hapten and maintaining the rest of the structural elements of the vaccine, we will maintain the properties of the M6-TT vaccine (high antibody titers). The objective of this study was to determine whether the antibodies generated by a tetanus toxoid-conjugated cocaine vaccine (COC-TT) can recognize and capture cocaine and decrease the cocaine-induced reinforcing effects. Male Wistar rats were immunized with the COC-TT. A solid-phase antibody-capture ELISA was used to monitor antibody titer responses after each booster dose in vaccinated animals. The study used cocaine self-administration and place-preference testing to evaluate the cocaine-reinforcing effects. The COC-TT vaccine could generate high levels of anti-cocaine antibodies. The antibodies reduced the cocaine self-administration and cocaine place preference. In addition, they decreased the cocaine-induced Fos protein expression. These findings suggest that the COC-TT vaccine generates a robust immunogenic response capable of reducing the reinforcing effects of cocaine, which supports its possible future use in clinical trials in patients with CUD.
Introduction
Cocaine is a psychostimulant, powerful, addictive drug and is considered one of the most consumed and popular illicit drugs and is responsible for important medical, psychiatric, and social problems throughout the world.Citation1,Citation2 Cocaine use disorder (CUD) is a psychiatric disorder characterized by compulsive and repetitive use of cocaine, a high rate of relapse, a significant rate of psychiatric and somatic comorbidity, and limited treatment options available approved by the FDA, making CUD a serious global public health problem.Citation3–5
Despite significant advances in the development of new pharmacological treatments against CUD; these treatments have shown limited effectiveness and severe side effects, which is evidenced by an increase in desertion, relapse, morbidity, and mortality.Citation6–8 Thus, given the lack of effective pharmacological agents, there is a need to identify and develop new effective long-term therapeutic approaches to reduce the cocaine effects.Citation9,Citation10
Cocaine is a low molecular weight lipophilic drug that readily crosses the blood-brain barrier (BB) and carries out its effects primarily through blocking dopamine transporters (DAT) in the central nervous system (SNC).Citation11 Given these cocaine pharmacological properties, it has been suggested for several years that an effective alternative treatment approach could include a pharmacological agent capable of blocking the pharmacokinetic and pharmacodynamic effects of cocaine.Citation12,Citation13
In this sense, immunotherapy is a promising alternative immunological therapeutic strategy.Citation9,Citation10 This therapeutic strategy is based on the development of a vaccine, which, when dosed in specific active vaccination programs, can generate specific serum antibodies, capable of recognizing and sequestering cocaine circulating in the bloodstream; In this way, these antibodies prevent the permeability of cocaine through the BB and its subsequent binding to the DAT. This alteration in the pharmacodynamics of cocaine results in a decrease in the cocaine-induced reinforcing and psychomotor effects.Citation13–15
Currently, there are various models of cocaine vaccines,Citation16–26 which have shown relative success in preventing cocaine from crossing the BBCitation27–30 and in reducing self-administration,Citation24,Citation27–29,Citation31–34 place-preference,Citation35 and locomotor activity,Citation18,Citation19,Citation21,Citation25,Citation29,Citation36–40 induced by cocaine, in rodents. However, the efficacy of anti-cocaine vaccines has been largely limited by the modest levels of vaccine-induced antibodies and/or by a variable production of anti-cocaine antibodies, when evaluated in primates.Citation22,Citation41–46 Therefore, new tactics and technical strategies should be explored to increase the immunogenicity of a cocaine vaccine.
Various studies have shown that the decrease of the cocaine-induced reinforcing effects depends on the vaccine’s ability to generate a large number of specific antibodies to cocaine.Citation14,Citation22,Citation27,Citation28,Citation43 Additionally, other authors have mentioned that the hapten, the carrier protein, the position of attachment of the hapten (cocaine) to the carrier protein, and even the adjuvant with which the vaccine is formulated greatly influence the magnitude of immunogenic responses and the specificity of antibodies.Citation14,Citation18,Citation20,Citation37,Citation47–50
In this sense, our laboratory previously reported the efficacy of the tetanus toxoid-conjugated morphine vaccine.Citation51–57 The M6-TT vaccine was capable of generating high titers of antibodies capable of capturing morphine, heroin, and their metabolitesCitation51,Citation52 and reducing heroin- or morphine-induced antinociception,Citation53,Citation55,Citation56 self-administration,Citation51,Citation52 and locomotor activity,Citation54,Citation57 in Wistar rats and BALB/c, C57Bl/6 and DBA/2 mice.Citation54
As mentioned above, vaccines may be an attractive therapeutic option for the treatment of CUD.Citation9,Citation10 However, preclinical and clinical studies agree that the results of active vaccination against cocaine-induced reinforcing and psychoactive effects have not been very encouraging, mainly when its effectiveness has been evaluated in clinical trials.Citation22,Citation41–46 Which suggests that anti-cocaine vaccines must be optimized even further to be able to transfer their use to clinical trials. Thus, given that the tetanus toxoid-conjugated morphine vaccine (M6-TT) demonstrated to be effective in generating high titers of specific antibodies to heroin, morphine and their metabolites,Citation51,Citation52 and given that various studies suggest that the magnitude of immunogenicity responses and the specificity of antibodies depends on the hapten, the carrier protein, the position of attachment of the hapten to the carrier protein and even the adjuvant with which the vaccine is formulated,Citation14,Citation18,Citation20,Citation37,Citation47–50 So it would be plausible to assume that if we modify the M6-TT vaccine by changing only the hapten (cocaine for morphine) and maintain the rest of the structural elements of the vaccine (spacer arm, carrier protein and adjuvant) we would maintain the properties of the M6-TT vaccine (high antibody titers); therefore, the objective of this study was to determine whether the antibodies generated by a tetanus toxoid-conjugated cocaine vaccine (COC-TT) are capable of recognizing and capturing cocaine and decreasing the cocaine-induced reinforcing effects.
Methods
Subjects
The study used male Wistar rats weighing 250–280 g at the onset of the experiments. They were housed four per cage in standard plastic rodent cages (57 cm × 35 cm × 20 cm) in a colony room at 21 ± 2°C and 40–50% humidity, under a 12-h light/dark cycle (lights on at 7:00 AM). The animals had free access to water during the experiments. Rats trained to food self-administration were restricted to 20 g per day of rat chow after daily operant sessions. The rats were acclimated to the experimental chambers for 2-h over 5 days, before the lever-press training sessions. All the experiments took place during the light phase of the light/dark cycle (9:00 a.m. to 5:00 p.m.). All the animal studies and standards followed the Principles of Laboratory Animal Care, as outlined by the National Institutes of Health in the USA, and were approved by the Animal Care and Bioethics Committee of the National Institute of Psychiatry in Mexico City.
Drugs
Cocaine hydrochloride was kindly donated by the Mexican government under strict regulatory controls. All the drugs used in experimental animals were placed under official surveillance (COFEPRIS- LC-0004-2003). Cocaine hydrochloride was dissolved in sterile saline solutions (0.9% NaCl, Sigma Aldrich) freshly prepared and filtered through a 25 µm syringe filter (Fisher Scientific, Pittsburgh, PA) before administration.
Synthesis of the COC-TT vaccine
The synthesis of the COC-TT vaccine begins with the production of benzoylecgonine (BE). Briefly, cocaine hydrochloride is dissolved at PH 9.5 in 0.1 M phosphate buffered saline (PBS) at a temperature of 70°C. The benzoylecgonine was activated for coupling with water-soluble 1-(3-dimethyl aminopropyl)-3-ethyl carbodiimide (EDC, Pierce, Rockford, IL, USA).
To prepare the TT-TFCS conjugate (tetanus toxoid+N-(-trifluoroacetyl caproyloxy) succinimide ester), the TFCS (Pierce, Rockford, IL, USA) was dissolved in a freshly prepared solution of 10–20% DMSO (Sigma – Aldrich, St. Louis, MO, USA)/80% distilled H2O. This solution was mixed with the tetanus toxoid (TT) at a volume of 4 ml of PBS, pH 7.2, and incubated at room temperature overnight. To remove the TFCS trifluoroacetyl protecting group, which is required to couple the TFCS to the
-amino groups of the side chain of lysine residues of the TT, it was incubated at pH 8.1 in PBS at room temperature for up to 3 hours. The final purification of the TT-TFCS derivative was carried out by exhaustive dialysis against PBS, pH 7.2.
To prepare the TT-COC conjugate, the activated EDC-COC was added to TT-TFCS in a volume of 100 ml of PBS, pH 7.5, and the reaction mixture was incubated under gentle stirring at room temperature overnight. After exhaustive dialysis against PBS, pH 7.4, the purified conjugate was concentrated by pressure dialysis, aliquoted (unit dose = 1 mg TT/ml), and stored in sealed sterile glass vials. The COC BSA conjugate was synthesized using the same method as for TT.
Immunization schedule
In total, 40 rats were immunized with the COC-TT vaccine adsorbed to an aluminum hydroxide gel adjuvant (Pierce, Rockford, IL, USA), and 40 rats were immunized with TT vaccine adsorbed to an aluminum hydroxide gel adjuvant. Each animal was injected subcutaneously (s.c.) at four sites over the shoulders bilaterally (two inoculations/side), for a total dose of COC-TT vaccine/adjuvant of 100 μg/1 mg aluminum hydroxide (≈100 μg/inoculation/animal/booster). Subsequently, 6 booster injections were administered using the same unit dose and adjuvant, over 14–16 weeks (once every 14 days). Control rats received TT injections at the same time as vaccinated rats. Fourteen days after each booster, the rats were invariably bled and sera were collected and frozen at –20°C until use.
Determination of serum antibody titers via ELISA
A solid-phase antibody capture ELISA was used to monitor antibody titer responses after each booster in vaccinated animals. ELISA plates were coated with COC-BSA conjugate, and a range of serial dilutions of antisera was added in triplicate. Biotin-labeled anti-mouse secondary antibody (Jackson Immunoresearch, West Grove, PA, USA), coupled to an OPD system (Sigma – Aldrich, St. Louis, MO, USA) as chromogenic substrate, was used to detect immunopositive signals. Antibody titers were initially defined as the inverse of the serum dilution that gave 50% of the maximal response.
Behavioral procedure
Self-administration procedure
The cocaine or food self-administration procedures were performed according to a method described elsewhere.Citation58,Citation59 Briefly, the rats were trained to self-administer cocaine using a 1 mg/kg cocaine unit dose (30 μl/infusion/30 sec), in daily 2-h sessions (FR5 with 2-min TO) for 6 days per week (Complete methods in Supplementary Material).
Place preference procedure
A standard protocol was used to perform the CPP procedure (Supplementary material).Citation60,Citation61
Histological procedure
A standard protocol was used to perform the histological procedure (Supplementary material).
Experimental procedures
The study used 80 male Wistar rats, which were assigned to three experimental groups. For Experiments 1 and 2, we used 40 animals that were further divided into two experimental groups (n = 20); for Experiment 3, we used 40 animals in two groups (n = 20). Each experimental group received a different pharmacological treatment.
Experiment 1
This experiment focused on determining whether the COCT-TT vaccine alters the re-acquisition of cocaine seeking in animals that self-administered cocaine. This experiment was divided into three experimental phases. Phase I, or the cocaine-acquisition phase, lasted 10 days. Phase II, or the cocaine-extinction phase, lasted 85 days. This phase includes the immunization phase which consists of 6 immunizations every 14 days. Phase III, or the cocaine-re-acquisition phase, lasted 10 days. At the end of this phase, the animals will be sacrificed (the sacrifice phase, lasted 1 day).
Four groups of animals were tested – TT + SAL (n = 10); TT + COC (n = 10); COC-TT + SAL (n = 10); and COC-TT + COC (n = 10).
The TT + SAL and COC-TT + SAL groups received daily saline self-administration during the acquisition, extinction, and re-acquisition phases. During the extinction phase, rats in the TT group received immunization with the TT vaccine, and animals in the COC-TT group received vaccination with the COC-TT vaccine.
In contrast, the TT + COC and COC-TT + COC groups received cocaine self-administration during the acquisition and re-acquisition phase. In the extinction phase, both groups received daily saline self-infusion. During the extinction phase, rats in the TT group received immunization with the TT vaccine, and animals in the COC-TT group received vaccination with the COC-TT vaccine. Upon completion of the re-acquisition phase, the animals were sacrificed at 45 minutes after treatment.
Experiment 2
This experiment was performed to determine the effect of the COC-TT vaccine on cocaine-induced Fos protein expression. This experiment only has one experimental phase, the sacrifice phase (1 day), which occurs at the end of experiment 1.
During the sacrifice phase, the TT + SAL (n = 10), COC-TT + SAL (n = 10), TT + COC (n = 10), and COC-TT + COC (n = 10) groups received daily saline or cocaine self-administration, respectively. Upon completion of the saline or cocaine self-administration, the animals were sacrificed 45 minutes after treatment. The intracellular cascade of events to produce peak levels of the protein c-fos is estimated to take ∼45 min.
Experiment 3
To evaluate the effect of the COC-TT vaccine on the re-acquisition of cocaine-induced CPP, we used five different consecutive pharmacological treatments, referred to as phases 1 to 5. Phase I for pre-conditioning, which lasted 1 day; Phase II, or the conditioning phase, which lasted 12 days; Phase III for post-conditioning (1 day); Phase IV, or the extinction phase (85 days); and Phase V, or testing for the re-acquisition of cocaine CPP, which lasted 1 day ().
In the pre-conditioning, conditioning, and extinction phase, the TT + SAL (n = 10), and COC-TT + SAL (n = 10) groups were exposed to saline administration. During the post-conditioning phase and the CPP test, no treatment was administered. During the extinction phase, rats in the TT group received immunization with the TT vaccine, and animals in the COC-TT group received vaccination with the COC-TT vaccine.
In contrast, during conditioning, the TT + COC and the COC-TT + COC groups received cocaine or saline, respectively on alternate days. During the extinction phase, cocaine was withdrawn and both groups were administered daily saline only. Two days before the re-acquisition of cocaine CPP test, both groups received cocaine or saline on alternate days. During post-conditioning and CPP testing, no group received any treatment. The time spent in each compartment was recorded for a total of 30 min. During the extinction phase, rats in the TT group received immunization with the TT vaccine, and animals in the COC-TT group received vaccination with the COC-TT vaccine ().
Statistical analysis
Data are expressed as the means ± S.E.M. In Experiment 1, the number of active lever responses was the parameter used to estimate cocaine self-administration responses. A two-way ANOVA was used to compare the active and inactive lever responses between groups, with groups (saline and cocaine) and treatment (TT and COC-TT vaccine) between subjects’ factors. If there was a significant F value in the interaction, a post hoc analysis of differences in responses between groups (p < .05) was performed using an additional Tukey test.
For Experiment 1 and 2, we used a two-way ANOVA with groups and treatment, as the between-subject factors, followed by a post-hoc analysis (Tukey test).
Results of antibody titers were analyzed using two-way repeated-measures ANOVA, where vaccination number was the repeated measures, and treatments (TT or COC-TT) and groups (saline and cocaine), as the between-subject factors; followed by a Tukey test for post hoc comparisons. SPSS software was used to perform all statistical analyses. The level of statistical significance was set at p < .05.
Results
Experiment 1
Titles
As shown in , the TT group immunized with the TT vaccine did not show an increase in anti-cocaine antibody titers. In contrast, the animals immunized with the COC-TT vaccine showed a progressive increase (F = (1,18) 562.241, p < .001) in anti-cocaine antibody titer measured by ELISA.
Figure 1. Experiment timeline (a). Antibody titer responses (to the sixth boost) in rats immunized with the TT or COC-TT vaccine (b). Serum samples were collected 14 days after each immunization. Mean titers (± S.E.M.). *p < .01 significant effects of the antibody titers generated by the COC-TT vaccine to the 6th booster compared to the antibody titers generated by the TT vaccine in wistar rats. The COC-TT vaccine reduces cocaine self-administration. Total cocaine seeking during re-acquisition (c). Mean responses on the active and inactive lever during the re-acquisition period (d). *p < .01 significant effects on the active and inactive lever responses in the TT + COC and COC-TT + COC groups compared to saline-treated groups. #p < .01 significant effects on the active and inactive lever responses in the TT + COC group compared to the COC-TT + COC group. As determined by two-way ANOVA followed by Tukey’s tests.

Tukey test found significant differences in the antibody titers shown by the COC-TT groups compared to the TT (p < .001) group, from the second immunization. Additionally, the Tukey test found that the maximum anti-heroin antibody titer for the COC-TT groups was reached after the fourth booster (p < .001).
Cocaine self-administration
During the re-acquisition of cocaine self-administration, the TT + COC group shows a significant increase (Two-way ANOVA; group; F (1, 36) = 37, 761.564, p < .001; treatment; F (1, 36) = 113, 714.044, p < .001; group X treatment interaction; F (1, 36) = 37, 531.580, p < .001) in the mean active lever press responses compared to the COC-TT + COC (p < .001) groups. Further, a post hoc analysis found no difference between the TT + SAL and COC-TT + SAL (p = .96) groups ().
Additionally, the post-hoc test found differences in the responses on the active lever press showed by the TT + SAL and COC-TT + SAL groups compared to those shown by the COC-TT + COC (p < .001) group.
In addition, two-way ANOVA found significant differences in the mean responses on the inactive lever in the groups (F (1, 36) = 1, 344.709, p < .001); in the treatments (F (1, 36) = 14, 323.228, p < .001); in the groups x treatment interaction (F (1, 36) = 12, 45.997, p < .001). The Tukey test found differences between the TT + COC and COC-TT + COC (p < .003) groups but did not find differences between the TT + SAL and COC-TT + SAL (p = .92) groups ().
Experiment 2
Two-way ANOVA found significant differences in the number of c-fos-IR cells in the IL (F (1, 36) = 1447.548, p < .001), AcbSh (F (1, 36) = 1446.281, p < .001), AcbC (F (1, 36) = 1790.643, p < .001), and VTA (F (1, 36) = 1108.118, p < .001).
Tukey’s test found differences in the number of c-fos-IR cells as shown by the animals of the TT + COC group compared to those shown by the TT + SAL (p < .0001), COC-TT + SAL (p < .0001), and COC-TT + COC (p < .0001) groups in each of the brain nuclei analyzed ( and ).
Figure 2. COC-TT vaccine reduces the number of cells immunoreactive to c-fos. Number of cells immunoreactive to c-fos (±S.E.M.) by group (n = 8 animals per group) in the infralimbic cortex (a), AcbC (b), AcbSh (c), and ventral-tegmental area (d). *p < .01 significant effects on the number of cells immunoreactive to c-fos in the TT + COC group compared to the TT + SAL group. #p < .01 significant effects between the TT + COC and COC-TT + COC groups, as determined by two-way ANOVA followed by Tukey’s tests.

Figure 3. Representative photomicrographs of Fos protein expression in the IL, AcbS, AcbC, and VTA of rats from all experimental groups at 20× magnification, where Fos protein expression was visible as dark ovals (highlighted by arrows). Scale bar = 100 μm.

The post hoc test did not find significant differences in the number of c-fos-IR cells in the IL, AcbSh, AcbC, and VTA between the COC-TT + COC group and the TT + SAL (p = .91) and COC-TT + SAL (p = .88) groups ().
Experiment 3
Titles
TT group immunized with the TT vaccine did not show an increase in anti-cocaine antibody titers. In contrast, the animals immunized with the COC-TT vaccine showed a progressive increase (F = (1,18) 634.269, p < .001) in anti-cocaine antibody titer measured by ELISA ().
Figure 4. COC-TT vaccine attenuated the re-acquisition of cocaine-induced CPP. Experiment timeline (a). Antibody titer responses (to the sixth boost) in rats immunized with the TT or COC-TT vaccine (b). Mean titers (± S.E.M.). *p < .01 significant effects of the antibody titers generated by the COC-TT vaccine to the 6th booster compared to the antibody titers generated by the TT vaccine in wistar rats. Mean time spent in the cocaine-paired chamber (± S.E.M.) by group (n = 8 animals per group), during acquisition (c) and re-acquisition (d). *p < .01 significant effects on the time in the cocaine-paired side in the TT + COC and COC-TT + COC groups compared to the TT + SAL and COC + SAL groups. #p < .01 significant effects between the TT + COC and COC-TT + COC groups, as determined by two-way ANOVA followed by Tukey’s tests.

Tukey test found significant differences in the antibody titers shown by the COC-TT groups compared to the TT (p < .001) group, from the second immunization. Additionally, the Tukey test found that the maximum anti-heroin antibody titer for the COC-TT groups was reached after the fourth booster (p < .001).
Cocaine place-preference
As shown in , cocaine increased significantly (Two-way ANOVA, group; F = (1, 36) 122, 980.483; p < .0001); treatments, (F = (1, 36) 107, 252.809; p < .0001); groups X treatment interaction (F = (1, 36) 118, 263.420; p < .0001) the time spent in the cocaine-paired chamber. The TT + SAL and the COC-TT + SAL (p = .98) groups did not show differences according to the post hoc test.
However, the Tukey test demonstrated that the time spent in the cocaine-paired chamber shown by TT + COC rats was different from that shown by TT + SAL (p < .001) and COC-TT + SAL (p < .0001) groups.
In addition, during the re-acquisition of phase, the Tukey’s test also found significant differences between COC-TT + COC and TT + COC group (p < .0001) groups in the time spent in the cocaine-paired chamber. However, the TT + SAL and the COC-TT + SAL groups (p = .98) did not show significant differences in the time spent in the cocaine-paired chamber.
Discussion
Various preclinical studies support the effectiveness of various vaccines against cocaine to reduce the cocaine-induced reinforcing and psychomotor effects, through the generation of antibodies that sequester cocaine within blood vessels.Citation16–26 However, its usefulness has been limited in clinical trials due to low and/or variable antibody production.Citation22,Citation41–46 In this sense, it is necessary to develop new vaccines and/or improve or optimize the cocaine vaccines already described and evaluated, for them to produce a greater amount of anti-cocaine antibodies.
Various methodological strategies have been evaluated to induce a robust immune response against drugs such as cocaine.Citation17,Citation19,Citation21,Citation29,Citation35,Citation49,Citation62,Citation63 From the generation or optimization of new haptens to the use of new adjuvants capable of simultaneously stimulating various immunological pathways, passing through the use of various carrier proteins and different types of spacer arms.Citation18,Citation20,Citation37,Citation40,Citation48,Citation50
In this study, we evaluated the efficacy of the COC-TT vaccine, which was developed from the M6-TT vaccine. The M6-TT vaccine formulated with aluminum as an adjuvant, demonstrated in various studies can produce high levels of serum antibodies (≈1: 300,000) capable of recognizing heroin, morphine, and their metabolites and attenuating the antinociceptive, reinforcing, and motor effects. induced by heroin or morphine.Citation51–57 These results are in line with what was found in this study, where rats immunized with the COC-TT vaccine absorbed with aluminum hydroxide gel, maintained the immunogenic and immunoprotected properties of the M6-TT vaccine. Rats immunized with the COC-TT vaccine showed anti-heroin antibody titers of ≈ 1: 650,000, which could attenuate the reinforcing effects of cocaine.
Concerning anti-cocaine antibody titers, pioneering studies reported that conjugates such as GNC-KLH,Citation25 COC-BSA,Citation24 and SNC-KLHCitation38,Citation41 showed anti-cocaine antibody titers of ≈ 1: 15000 to 1: 80000. In humans, the picture was similar, immunization of cocaine-dependent patients with the TA-CD vaccine (SNC-rCTB)Citation22 generated antibody levels of ≈ 1: 2,400.
These vaccines, called first generation, were synthesized by coupling cocaine derivatives such as benzoylecgonine, norcocaine, and succinyl norcocaine to carrier proteins such as keyhole limpet hemocyanin (KLH), bovine serum albumin (BSA) and recombinant cholera toxin B. (rCTB) formulated mainly with incomplete Freund’s adjuvant (FCA), Ribi adjuvant (MPL-TDM) or aluminum hydroxide as adjuvants. Citation24,Citation25,Citation38,Citation41 In this sense, the molecular elements that make up the COC-TT vaccine, as well as its formulation, bear a certain similarity to the structural elements and formulation of the GNC-KLH, COC-BSA, SNC-KLH, and SNC vaccines. -rCTB,Citation24,Citation25,Citation38,Citation41 mainly in the hapten and the adjuvant used – benzoylecgonine and aluminum hydroxide—, however, the main difference lies in the carrier protein used; The COC-TT vaccine was synthesized through the combination of benzoylecgonine with tetanus toxoid. Thus, the difference in antibody titers between the GNC-KLH, COC-BSA, SNC-KLH, and SNC-rCTB conjugates and the COC-TT may be, in principle, the carrier protein used.
Tetanus toxoid (TT) is a highly effective and immunogenic protein, it is safe, well tolerated, and nontoxic, It contains many epitopes and one of its uses is as a carrier to deliver molecules to the CNS.Citation64 TT has been used in the development and evaluation of various immunoconjugates against illicit drugs such as heroin/morphine,Citation51 nicotine,Citation65,Citation66 methamphetamines,Citation67,Citation68 and recently fentanyl.Citation69–76 In fact, Kishima et al. suggested that vaccines synthesized with TT as a carrier protein were superior to vaccines conjugated with flagellin.Citation18,Citation48 In all cases these conjugates have shown efficiency in generating significant levels of antibodies capable of reducing the behavioral effects generated by these drugs. This suggests that TT could be one of the structural elements of the COC-TT vaccine that participates in the high production of antibodies against cocaine.
The hypothesis of the importance of the carrier protein as one of the structural elements of a conjugate vaccine that, when optimized, increases the immunogenic properties of a conjugate vaccine, is supported by evidence related to the synthesis of the so-called second or third-generation anti-cocaine vaccines.Citation17,Citation19,Citation21,Citation29,Citation35,Citation49,Citation62,Citation63 In the search for highly immunogenic proteins, the dAd5GNE and dAd5GNC vaccines were developed.Citation21,Citation29,Citation62,Citation73 To these vaccines, cocaine analogs called GNE and GNC were covalently coupled to the capsid proteins of adenoviral vectors (dAd) as carrier proteins. Immunization of rats and primates with dAd5GNE and dAd5GNC conjugates generated a range of anti-cocaine antibody titers from 1: 300,000 to 1: 900,000,Citation21,Citation29,Citation62,Citation73 which decreased cocaine levels in the brain, reduced cocaine DAT occupancy, prevented cocaine-induced locomotor activity and cocaine self -administration behavior.
On the other hand, a new generation of cocaine vaccines called self-adjuvating cocaine vaccines have recently been developed,Citation17,Citation19,Citation35,Citation49,Citation63 in which nano scaffolds or nanoparticle platforms (flagellin, KFE8 peptide, PDD dendrimers, and calix[n]arene) were designed that could increase the surface-volume ratio to increase antigen presentation. optimize hapten coupling, improve hapten spatial organization, and simultaneously show adjuvant activity. The results have shown that immunization with the conjugates GNE-FliC, CocKFE8, GNE-PDD, and GNE-calix[n]arene (UFMG-V4N2) generated high levels of antibodies which can decrease the passage of cocaine through the BBB and reduced cocaine’s effects on animal behavior.Citation17,Citation19,Citation35,Citation49,Citation63 However, an important limitation of the use of these nanoparticle platforms is the long regulatory path that they must comply with to be used in any clinical trial. However, these results together are in line with what was found in our study, where we found that the COC-TT conjugate produces high titers of anti-cocaine antibodies and supports the important role of the carrier protein in the generation of high and sustained anti-cocaine antibody levels.
Additionally, in this study, we found an attenuation in the reinforcing effects induced by cocaine, seen as a decrease in cocaine self-administration and cocaine place preference. These results are in line with previous reports.Citation24,Citation27–29,Citation31,Citation32,Citation34,Citation35
Certainly, it could be considered that the decrease in cocaine-reinforcing effects is associated with the levels of anti-cocaine antibodies evoked by the COC-TT conjugate (≈1: 650,000). However, our results do not agree with this hypothesis. Pioneering studies reported a decrease in cocaine self-administration at a dose of 1 mg/kg of cocaine, a dose like that used in this study.Citation24,Citation27–29,Citation31,Citation32,Citation34,Citation35 But, the anti-cocaine antibody titers generated by these vaccines COC-BSA,Citation24,Citation27 GNC-KLH,Citation28 and IPC-1010Citation31,Citation32 were not greater than ≈ 1: 100,000. On the other hand, Wee et al. (2012) and Evans et al., (2016) reported that immunization with the dAd5GNE vaccine in rats and primates generated a decrease in cocaine self-administration at doses of 0.5 to 1 mg/kg cocaine. In these studies antibody levels were ≈ 1: 300,000 to 1: 900,000.Citation29,Citation34 In this sense, our results are consistent with the results reported by these studies. However, it suggests that the discrepancy between the decrease in cocaine self-administration and anti-cocaine antibody levels is primarily related to the dose of cocaine used in the self-administration studies (0.5 mg/kg to 1 mg/kg cocaine). Therefore, it is necessary to evaluate the capacity of the anti-cocaine antibodies induced by the COC-TT vaccine in pharmacological models that require a range of higher doses of cocaine, such as cocaine-induced locomotor activity or cocaine place preference.
In this sense, in this study, we found that the anti-cocaine antibodies produced by the COC-TT conjugate could reduce the cocaine place preference induced by 10 mg/Kg of cocaine. These results are in line with a study that showed that male Sprague-Dawley rats immunized with the GNE-PDD vaccine exhibited a significant decrease in preference for the chamber associated with cocaine (15 mg/Kg).Citation35 These results suggest that the anti-cocaine antibodies produced by the COC-TT vaccine were sufficient to diminish the effect of high doses of cocaine.
As mentioned above, active vaccination seeks to generate specific serum antibodies, capable of recognizing and sequestering cocaine circulating in the bloodstream and preventing the permeability of cocaine through the BB and its subsequent binding to the DAT, which results in a reduction in cocaine-induced dopamine levels in the CNS.Citation13–15
Some studies in rodents have described that cocaine produces its psychomotor and reinforcing effects by increasing the extracellular dopamine levels in the striatum,Citation75,Citation76 which leads to the activation of dopamine D1 and D2 receptors in the striatum, ventral-tegmental area (VTA) and prefrontal cortex (PFx).Citation77 Which increases the expression of some early expression genes (IEG), which encode transcription factors such as the Fos protein.Citation78–80 These studies suggest that the c-Fos gene is an intracellular regulator of cocaine-induced alterations in gene expression, reorganization of neural circuitry, and expression of behavioral sensitizationCitation81,Citation82 and its expression depends on differential activation of dopamine D1 and D2 receptors.Citation78,Citation79
In this study, we used Fos protein expression as a novel methodological approach to determine the efficacy of anti-cocaine antibodies produced by the COC-TT vaccine in preventing the permeation of cocaine through the BB and its subsequent binding to the DAT. In this sense, animal studies have shown that various external stimuli that increase neuronal activity induce c-fos expression.Citation83,Citation84 This property has given the expression of the c-fos gene to be considered an important experimental tool as a marker of neuronal activity.Citation83,Citation85
Thus, in this study, we found that rats immunized with the COC-TT vaccine showed a significant decrease in the expression of Fos protein in the striatum, VTA, and PFx. This suggests that the anti-cocaine antibodies produced by the COC-TT vaccine were capable of sequestering cocaine in the bloodstream, preventing its passage through the BB and its subsequent binding to the DAT, decreasing extracellular dopamine levels, the activation of D1 and D2 receptors and finally the expression of the FOS protein.
This result is novel, since to our knowledge this methodological approach had not been used to evaluate the efficacy of a vaccine against illicit drugs. However, these results are in line with what was reported by other studies that show that primates vaccinated with the dAd5GNE vaccine showed a reduction in the cocaine binding to the DAT in the striatum.Citation86 Furthermore, they agree with studies that reported that antibodies produced by the dAd5GNE, UFMG-V4N2, and GNE-calix[n]arene vaccines could prevent the crossing of cocaine through the BB, seen as an increase in cocaine levels in blood versus brain.Citation17,Citation63,Citation64,87
Conclusion
Certainly, other factors could affect the production of antibodies, beyond the carrier protein, such as the vaccine dose, the number of immunizations, the adjuvant, the dose of cocaine, stress, etc.Citation18,Citation20,Citation37,Citation40,Citation48,Citation50 However, about the adjuvant, studies that compared the effect of various adjuvants (SAS-Sigma Adjuvant System; CPpG-cytosine-guanine oligodeoxynucleotide; PCEP; etc.,) indicated that they did not significantly increase the titers of anti-cocaine antibodies compared to those obtained with alumina.Citation18,Citation20,Citation37,Citation40,Citation48,Citation50 This suggests that the adjuvant factor may not be relevant in this case.
The above poses important limitations regarding a proposal to transfer the COC-TT vaccine to clinical protocols, perhaps the most important is related to the number of vaccinations required to reach the maximum levels of antibodies (6 immunizations), given that clinical trials have indicated that cocaine-dependent patients show poor adherence to treatment.Citation22,Citation43–46 But despite this limitation, the results of this study suggest that at the dose of vaccine and the dose of cocaine used in the pharmacological evaluations, the antibody titers generated by the COC-TT vaccine are capable of significantly reducing the permeation of cocaine through the BB and subsequently attenuate the reinforcing effects of cocaine. This feature supports its potential use as a treatment for patients with CUD.
Authorship contributions
Participated in research design: Alberto Salazar-Juárez. Conducted Experiments: Alberto Salazar-Juárez, Ricardo Hernandez-Miramontes, Maura Matus-Ortega, and Susana Barbosa Méndez. Performed data analysis: Alberto Salazar-Juárez and Susana Barbosa Méndez. Wrote or contributed to the writing of the manuscript: Alberto Salazar-Juárez and Susana Barbosa Méndez.
Ethical approval
The Institutional Animal Care approved the procedures (CICUAL/0016/2006) and Bioethics Committee (CEI/C/IC092020/2006), in strict compliance with the Guide for the Care and Use of Laboratory Animals published by the National Institutes of Health (NIH).
Salazar et al COC TT Supplementary_clean.doc
Download MS Word (81.5 KB)Disclosure statement
No potential conflict of interest was reported by the author(s).
Supplementary material
Supplemental data for this article can be accessed on the publisher’s website at https://doi.org/10.1080/21645515.2023.2299068.
Additional information
Funding
References
- Schwartz EKC, Wolkowicz NR, De Aquino JP, MacLean RR, Sofuoglu M. Cocaine use disorder (CUD): current clinical perspectives. Subst Abuse Rehabil. 2022;13:25–12. doi:10.2147/SAR.S337338.
- Li MJ, Shoptaw SJ. Clinical management of psychostimulant withdrawal: review of the evidence. Addiction. 2023;118(4):750–62. doi:10.1111/add.16093.
- Poireau M, Milpied T, Maillard A, Delmaire C, Volle E, Bellivier F, Icick R, Azuar J, Marie-Claire C, Bloch V, et al. Biomarkers of relapse in cocaine use disorder: a narrative review. Brain Sci. 2022;12(8):1013. doi:10.3390/brainsci12081013.
- Armoon B, Fleury MJ, Bayat AH, Bayani A, Mohammadi R, Griffiths MD. Quality of life and its correlated factors among patients with substance use disorders: a systematic review and meta-analysis. Arch Public Health. 2022;80(1):179. doi:10.1186/s13690-022-00940-0.
- Stellern J, Xiao KB, Grennell E, Sanches M, Gowin JL, Sloan ME. Emotion regulation in substance use disorders: a systematic review and meta-analysis. Addiction. 2023;118(1):30–47. doi:10.1111/add.16001.
- Liu MT. Pharmacotherapy treatment of stimulant use disorder. Ment Health Clin. 2021;11:347–57. doi:10.9740/mhc.2021.11.347.
- Lassi DLS, Malbergier A, Negrão AB, Florio L, De Aquino JP, Castaldelli-Maia JM. Pharmacological treatments for cocaine craving: what is the way forward? A systematic review. Brain Sci. 2022;12(11):1546. doi:10.3390/brainsci12111546.
- Jensen KL, Jensen SB, Madsen KL. A mechanistic overview of approaches for the treatment of psychostimulant dependence. Front Pharmacol. 2022;13:854176. doi:10.3389/fphar.2022.854176.
- Vasiliu O. Current trends and perspectives in the immune therapy for substance use disorders. Front Psychiatry. 2022;13:882491. doi:10.3389/fpsyt.2022.882491.
- Truong TT, Kosten TR. Current status of vaccines for substance use disorders: a brief review of human studies. J Neurol Sci. 2022;434:120098. doi:10.1016/j.jns.2021.120098.
- Docherty JR, Alsufyani HA. Pharmacology of drugs used as stimulants. J Clin Pharmacol. 2021;61(Suppl 2):53–69. doi:10.1002/jcph.1918.
- Scendoni R, Bury E, Ribeiro ILA, Cameriere R, Cingolani M. Vaccines as a preventive tool for substance use disorder: a systematic review including a meta-analysis on nicotine vaccines’ immunogenicity. Hum Vaccin Immunother. 2022;18(6):2140552. doi:10.1080/21645515.2022.2140552.
- Celik M, Fuehrlein B. A review of immunotherapeutic approaches for substance use disorders: current status and future prospects. Immunotargets Ther. 2022;11:55–66. doi:10.2147/ITT.S370435.
- Salazar-Juárez A, Barbosa-Méndez S, Jurado N, Antón B. Inmunoprotección activa contra la cocaína. Salud Ment. 2015;38:441–7. doi:10.17711/SM.0185-3325.2015.059.
- Malik JA, Agrewala JN. Future perspectives of emerging novel drug targets and immunotherapies to control drug addiction. Int Immunopharmacol. 2023;119:110210. doi:10.1016/j.intimp.2023.110210.
- Madge HYR, Alexander S, Azuar A, Zhang J, Koirala P, Burne TH, Toth I, Stephenson RJ. Synthetic anti-cocaine nanoaccine successfully prevents cocaine-induced hyperlocomotion. J Med Chem. 2023;66(17):12407–12419. doi:10.1021/acs.jmedchem.3c00889.
- da Silva Neto L, da Silva Maia AF, Godin AM, de Almeida Augusto PS, Pereira RLG, Caligiorne SM, Alves RB, Fernandes SOA, Cardoso VN, Goulart GAC, et al. Calix[n]arene-based immunogens: a new non-proteic strategy for anti-cocaine vaccine. J Adv Res. 2021;38:285–98. doi:10.1016/j.jare.2021.09.003.
- Kimishima A, Olson ME, Janda KD. Investigations into the efficacy of multi-component cocaine vaccines. Bioorg Med Chem Lett. 2018;28(16):2779–2783. doi:10.1016/j.bmcl.2017.12.043.
- Rudra JS, Ding Y, Neelakantan H, Ding C, Appavu R, Stutz S, Snook JD, Chen H, Cunningham KA, Zhou J. Suppression of cocaine-evoked hyperactivity by self-adjuvanting and multivalent peptide nanofiber vaccines. ACS Chem Neurosci. 2016;7(5):546–52. doi:10.1021/acschemneuro.5b00345.
- Cai X, Whitfield T, Hixon MS, Grant Y, Koob GF, Janda KD. Probing active cocaine vaccination performance through catalytic and noncatalytic hapten design. J Med Chem. 2013;56(9):3701–9. doi:10.1021/jm400228w.
- Hicks MJ, De BP, Rosenberg JB, Davidson JT, Moreno AY, Janda KD, Wee S, Koob GF, Hackett NR, Kaminsky SM, et al. Cocaine analog coupled to disrupted adenovirus: a vaccine strategy to evoke high-titer immunity against addictive drugs. Mol Ther. 2011;19(3):612–9. doi:10.1038/mt.2010.280.
- Kosten TR, Rosen M, Bond J, Settles M, Roberts JS, Shields J, Jack L, Fox B. Human therapeutic cocaine vaccine: safety and immunogenicity. Vaccine. 2002;20(7–8):1196–204. doi:10.1016/S0264-410X(01)00425-X.
- Ettinger RH, Ettinger WF, Harless WE. Active immunization with cocaine-protein conjugate attenuates cocaine effects. Pharmacol Biochem Behav. 1997;58:215–20. doi:10.1016/S0091-3057(97)00005-1.
- Fox BS, Kantak KM, Edwards MA, Black KM, Bollinger BK, Botka AJ, French TL, Thompson TL, Schad VC, Greenstein JL, et al. Efficacy of a therapeutic cocaine vaccine in rodent models. Nat Med. 1996;2(10):1129–32. doi:10.1038/nm1096-1129.
- Carrera MR, Ashley JA, Parsons LH, Wirsching P, Koob GF, Janda KD. Suppression of psychoactive effects of cocaine by active immunization. Nature. 1995;378(6558):727–30. doi:10.1038/378727a0.
- Bagasra O, Forman LJ, Howeedy A, Whittle P. A potential vaccine for cocaine abuse prophylaxis. Immunopharmacology. 1992;23(3):173–9. doi:10.1016/0162-3109(92)90023-6.
- Fox BS. Development of a therapeutic vaccine for the treatment of cocaine addiction. Drug Alcohol Depend. 1997;48:153–8. doi:10.1016/S0376-8716(97)00121-X.
- Carrera MR, Ashley JA, Zhou B, Wirsching P, Koob GF, Janda KD. Cocaine vaccines: antibody protection against relapse in a rat model. Proc Natl Acad Sci USA. 2000;97(11):6202–6. doi:10.1073/pnas.97.11.6202.
- Wee S, Hicks MJ, De BP, Rosenberg JB, Moreno AY, Kaminsky SM, Janda KD, Crystal RG, Koob GF. Novel cocaine vaccine linked to a disrupted adenovirus gene transfer vector blocks cocaine psychostimulant and reinforcing effects. Neuropsychopharmacology. 2012;37(5):1083–91. doi:10.1038/npp.2011.200.
- De BP, Pagovich OE, Hicks MJ, Rosenberg JB, Moreno AY, Janda KD, Koob GF, Worgall S, Kaminsky SM, Sondhi D, Crystal RG. Disrupted adenovirus-based 638 vaccines against small addictive molecules circumvent anti-adenovirus immunity. Hum Gene Ther. 2013;24(1):58–66. doi:10.1089/hum.2012.163.
- Kantak KM, Collins SL, Lipman EG, Bond J, Giovanoni K, Fox BS. Evaluation of anti-cocaine antibodies and a cocaine vaccine in a rat self-administration model. Psychopharmacol (Berl). 2000;148(3):251–62. doi:10.1007/s002130050049.
- Kantak KM, Collins SL, Bond J, Fox BS. Time course of changes in cocaine self-administration behavior in rats during immunization with the cocaine vaccine IPC-1010. Psychopharmacol (Berl). 2001;153(3):334–40. doi:10.1007/s002130000555.
- Koob G, Hicks MJ, Wee S, Rosenberg JB, De BP, Kaminsky SM, Moreno A, Janda KD, Crystal RG. Anti-cocaine vaccine based on coupling a cocaine analog to a disrupted adenovirus. CNS Neurol Disord Drug Targets. 2011;10:899–904. doi:10.2174/187152711799219334.
- Evans SM, Foltin RW, Hicks MJ, Rosenberg JB, De BP, Janda KD, Kaminsky SM, Crystal RG. Efficacy of an adenovirus-based anti-cocaine vaccine to reduce cocaine self-administration and acquisition using a choice procedure in rhesus macaques. Pharmacol Biochem Behav. 2016;150–151:76–86. doi:10.1016/j.pbb.2016.09.008.
- Lowell JA, Dikici E, Joshi PM, Landgraf R, Lemmon VP, Daunert S, Izenwasser S, Daftarian P. Vaccination against cocaine using a modifiable dendrimer nanoparticle platform. Vaccine. 2020;38(50):7989–7997. doi:10.1016/j.vaccine.2020.10.041.
- Carrera MR, Ashley JA, Wirsching P, Koob GF, Janda KD. A second-generation vaccine protects against the psychoactive effects of cocaine. Proc Natl Acad Sci USA. 2001;98(4):1988–92. doi:10.1073/pnas.98.4.1988.
- Cai X, Whitfield T, Moreno AY, Grant Y, Hixon MS, Koob GF, Janda KD. Probing the effects of hapten stability on cocaine vaccine immunogenicity. Mol Pharm. 2013;10(11):4176–84. doi:10.1021/mp400214w.
- Kosten TA, Shen XY, Kinsey BM, Kosten TR, Orson FM. Attenuation of cocaine-induced locomotor activity in male and female mice by active immunization. Am J Addict. 2014;23(6):604–7. doi:10.1111/j.1521-0391.2014.12152.x.
- St John AL, Choi HW, Walker QD, Blough B, Kuhn CM, Abraham SN, Staats HF. Novel mucosal adjuvant, mastoparan-7, improves cocaine vaccine efficacy. NPJ Vaccines. 2020;5(1):12. doi:10.1038/s41541-020-0161-1.
- Lin M, Marin A, Ellis B, Eubanks LM, Andrianov AK, Janda KD. Polyphosphazene: a new adjuvant platform for cocaine vaccine development. Mol Pharm. 2022;19:3358–66. doi:10.1021/acs.molpharmaceut.2c00489.
- Kosten TR, Domingo CB, Shorter D, Orson F, Green C, Somoza E, Sekerka R, Levin FR, Mariani JJ, Stitzer M, et al. Vaccine for cocaine dependence: a randomized double-blind placebo-controlled efficacy trial. Drug Alcohol Depend. 2014;140:42–7. doi:10.1016/j.drugalcdep.2014.04.003.
- Kosten TR, Haile CN, Domingo CB, Norton EB. Anti-cocaine IgA rather than IgG mediates vaccine protection from cocaine use. Pharmaceutics. 2022;14(11):2368. doi:10.3390/pharmaceutics14112368.
- Martell BA, Orson FM, Poling J, Mitchell E, Rossen RD, Gardner T, Kosten TR. Cocaine vaccine for the treatment of cocaine dependence in methadone-maintained patients: a randomized, double-blind, placebo-controlled efficacy trial. Arch Gen Psychiatry. 2009;66:1116–23. doi:10.1001/archgenpsychiatry.2009.128.
- Stitzer ML, Polk T, Bowles S, Kosten T. Drug users’ adherence to a 6-month vaccination protocol: effects of motivational incentives. Drug Alcohol Depend. 2010;107:76–9. doi:10.1016/j.drugalcdep.2009.09.006.
- Haney M, Gunderson EW, Jiang H, Collins ED, Foltin RW. Cocaine-specific antibodies blunt the subjective effects of smoked cocaine in humans. Biol Psychiatry. 2010;67(1):59–65. doi:10.1016/j.biopsych.2009.08.031.
- Orson FM, Rossen RD, Shen X, Lopez AY, Wu Y, Kosten TR. Spontaneous development of IgM anti-cocaine antibodies in habitual cocaine users: effect on IgG antibody responses to a cocaine cholera toxin B conjugate vaccine. Am J Addict. 2013;22(2):169–74. doi:10.1111/j.1521-0391.2013.00314.x.
- Salazar-Juárez A, Barbosa-Méndez S, Feregrino-Alonso MI, Hernández-Miramontes R, Ramos-Ochoa E, Bonilla-Reyes E, Jacinto-Gutierrez S, Pentel P, Antón B. Nuevas vacunas contra la morfina/heroína. Salud Ment. 2013;36:219–27. doi:10.17711/SM.0185-3325.2013.028.
- Kimishima A, Wenthur CJ, Eubanks LM, Sato S, Janda KD. Cocaine vaccine development: evaluation of carrier and adjuvant combinations that activate multiple toll-like receptors. Mol Pharm. 2016;13(11):3884–3890. doi:10.1021/acs.molpharmaceut.6b00682.
- Lockner JW, Eubanks LM, Choi JL, Lively JM, Schlosburg JE, Collins KC, Globisch D, Rosenfeld-Gunn RJ, Wilson IA, Janda KD. Flagellin as carrier and adjuvant in cocaine vaccine development. Mol Pharm. 2015;12(2):653–62. doi:10.1021/mp500520r.
- Cai X, Tsuchikama K, Janda KD. Modulating cocaine vaccine potency through hapten fluorination. J Am Chem Soc. 2013;135(8):2971–4. doi:10.1021/ja400356g.
- Anton B, Leff P. A novel bivalent morphine/heroin vaccine that prevents relapse to heroin addiction in rodents. Vaccine. 2006;24(16):3232–40. doi:10.1016/j.vaccine.2006.01.047.
- Anton B, Salazar A, Flores A, Matus M, Marin R, Hernandez JA, Leff P. Vaccines against morphine/heroin and its use as effective medication for preventing relapse to opiate addictive behaviors. Hum Vaccine. 2009;5:214–29. doi:10.4161/hv.5.4.7556.
- Barbosa-Méndez S, Matus-Ortega M, Hernandez-Miramontes R, Salazar-Juárez A. Effect of the morphine/heroin vaccine on opioid and non-opioid drug-induced antinociception in mice. Eur J Pharmacol. 2021;891:173718. doi:10.1016/j.ejphar.2020.173718.
- Barbosa-Méndez S, Matus-Ortega M, Hernández-Miramontes R, Salazar-Juárez A. The morphine/heroin vaccine decreased the heroin-induced antinociceptive and reinforcing effects in three inbred strains mouse. Int Immunopharmacol. 2021;98:107887. doi:10.1016/j.intimp.2021.107887.
- Barbosa-Mendez S, Matus-Ortega M, Hernandez-Miramontes R, Salazar-Juárez A. The M3-TT vaccine decreases the antinociceptive effects of morphine and heroin in mice. Int J Ment Health & Addict. 2021;21:783–802. doi:10.1007/s11469-021-00621-z.
- Barbosa-Mendez S, Matus-Ortega M, Hernandez-Miramontes R, Salazar-Juárez A. Synergistic immune and antinociceptive effects induced from the combination of two different vaccines against morphine/heroin in mouse. Hum Vaccin Immunother. 2021;17(10):3515–3528. doi:10.1080/21645515.2021.1935171.
- Barbosa-Mendez S, Matus-Ortega M, Hernandez-Miramontes R, Salazar-Juárez A. The effect of chronic stress on the immunogenicity and immunoprotection of the M6-TT vaccine in female mice. Physiol & Behav. 2023;271:114345. doi:10.1016/j.physbeh.2023.114345.
- Barbosa-Méndez S, Leff P, Arías-Caballero A, Hernández-Miramontes R, Heinze G, Salazar-Juárez A. Mirtazapine attenuates cocaine seeking in rats. J Psychiatr Res. 2017;92:38–46. doi:10.1016/j.jpsychires.2017.03.021.
- Barbosa-Méndez S, Salazar-Juárez A. Mirtazapine attenuates nicotine-seeking behavior in rats. J Psychopharmacol. 2018;32(9):1010–1017. doi:10.1177/0269881118764991.
- Barbosa-Méndez S, Matus-Ortega M, Jacinto-Gutiérrez S, Salazar-Juárez A. Mirtazapine impairs acquisition and reinstatement of cocaine-induced place preference in rats. Eur J Pharmacol. 2018;820:183–190. doi:10.1016/j.ejphar.2017.12.033.
- Barbosa-Méndez S, Pérez-Sánchez G, Becerril-Villanueva E, Salazar-Juárez A. Melatonin decreases cocaine-induced locomotor sensitization and cocaine-conditioned place preference in rats. J Psychiatr Res. 2021;132:97–110. doi:10.1016/j.jpsychires.2020.09.027.
- Hicks MJ, Kaminsky SM, De BP, Rosenberg JB, Evans SM, Foltin RW, Andrenyak DM, Moody DE, Koob GF, Janda KD, et al. Fate of systemically administered cocaine in nonhuman primates treated with the dAd5GNE anticocaine vaccine. Hum Gene Ther Clin Dev. 2014;25(1):40–9. doi:10.1089/humc.2013.231.
- Sabato B, Augusto PSA, Lima Gonçalves Pereira R, Coutinho Batista Esteves F, Caligiorne SM, Rodrigues Dias Assis B, Apolo Correia Marcelino S, Pires Do Espírito Santo L, Dias Dos Reis K, Da Silva Neto L, et al. Safety and immunogenicity of the anti-cocaine vaccine UFMG-VAC-V4N2 in a non-human primate model. Vaccine. 2023;41:2127–36. doi:10.1016/j.vaccine.2023.02.031.
- Bayart C, Mularoni A, Hemmani N, Kerachni S, Jose J, Gouet P, Paladino J, Le Borgne M. Tetanus toxin fragment C: structure, drug discovery research and production. Pharm (Basel). 2022;15(6):756. doi:10.3390/ph15060756.
- Oliver JL, Pashmi G, Barnett P, Mettens P, Biemans R, Monteyne P, Palmantier R, Gallagher T, Ramaya S, Wonnacott S. Development of an anti-cotinine vaccine to potentiate nicotine-based smoking cessation strategies. Vaccine. 2007;25(42):7354–62. doi:10.1016/j.vaccine.2007.08.019.
- Tonstad S, Heggen E, Giljam H, Lagerbäck PÅ, Tønnesen P, Wikingsson LD, Lindblom N, de Villiers S, Svensson TH, Fagerström KO. Niccine®, a nicotine vaccine, for relapse prevention: a phase II, randomized, placebo-controlled, multicenter clinical trial. Nicotine Tob Res. 2013;15:1492–501. doi:10.1093/ntr/ntt003.
- Haile CN, Kosten TA, Shen XY, O’Malley PW, Winoske KJ, Kinsey BM, Wu Y, Huang Z, Lykissa ED, Naidu N, et al. Altered methamphetamine place conditioning in mice vaccinated with a succinyl-methamphetamine-tetanus-toxoid vaccine. Am J Addict. 2015;24(8):748–55. doi:10.1111/ajad.12307.
- Arora R, Haile CN, Kosten TA, Wu Y, Ramakrishnan M, Hawkins LD, Orson FM, Kosten TR. Preclinical efficacy of an anti-methamphetamine vaccine using E6020 adjuvant. Am J Addict. 2019;28(2):119–126. doi:10.1111/ajad.12867.
- Barrientos RC, Whalen C, Torres OB, Sulima A, Bow EW, Komla E, Beck Z, Jacobson AE, Rice KC, Matyas GR. Bivalent conjugate vaccine induces dual immunogenic response that attenuates heroin and fentanyl effects in mice. Bioconjug Chem. 2021;32(11):2295–2306. doi:10.1021/acs.bioconjchem.1c00179.
- Barrientos RC, Bow EW, Whalen C, Torres OB, Sulima A, Beck Z, Jacobson AE, Rice KC, Matyas GR. Novel vaccine that blunts fentanyl effects and sequesters ultrapotent fentanyl analogues. Mol Pharm. 2020;17(9):3447–3460. doi:10.1021/acs.molpharmaceut.0c00497.
- Tenney RD, Blake S, Bremer PT, Zhou B, Hwang CS, Poklis JL, Janda KD, Banks ML. Vaccine blunts fentanyl potency in male rhesus monkeys. Neuropharmacology. 2019;158:107730. doi:10.1016/j.neuropharm.2019.107730.
- Townsend EA, Blake S, Faunce KE, Hwang CS, Natori Y, Zhou B, Bremer PT, Janda KD, Banks ML. Conjugate vaccine produces long-lasting attenuation of fentanyl vs. food choice and blocks expression of opioid withdrawal-induced increases in fentanyl choice in rats. Neuropsychopharmacology. 2019;44:1681–9. doi:10.1038/s41386-019-0385-9.
- Havlicek DF, Rosenberg JB, De BP, Hicks MJ, Sondhi D, Kaminsky SM, Crystal RG, Kremer EJ. Cocaine vaccine dAd5gne protects against moderate daily and high-dose “binge” cocaine use. PLoS One. 2020;15(11):e0239780. doi:10.1371/journal.pone.0239780.
- Kalivas PW, Duffy P. Effect of acute and daily cocaine treatment on extracellular dopamine in the nucleus accumbens. Synapse. 1990;5(1):48–58. doi:10.1002/syn.890050104.
- Moghaddam B, Bunney BS. Differential effect of cocaine on extracellular dopamine levels in rat medial prefrontal cortex and nucleus accumbens: comparison to amphetamine. Synapse. 1989;4(2):156–61. doi:10.1002/syn.890040209.
- Missale C, Nash SR, Robinson SW, Jaber M, Caron MG. Dopamine receptors: from structure to function. Physiol Rev. 1998;78(1):189–225. doi:10.1152/physrev.1998.78.1.189.
- Hope B, Kosofsky B, Hyman SE, Nestler EJ. Regulation of immediate early gene expression and AP-1 binding in the rat nucleus accumbens by chronic cocaine. Proc Natl Acad Sci USA. 1992;89(13):5764–8. doi:10.1073/pnas.89.13.5764.
- Robertson GS, Jian M. D1 and D2 dopamine receptors differentially increase fos-like immunoreactivity in accumbal projections to the ventral pallidum and midbrain. Neuroscience. 1995;64(4):1019–34. doi:10.1016/0306-4522(94)00426-6.
- Young ST, Porrino LJ, Iadarola MJ. Cocaine induces striatal c-fos-immunoreactive proteins via dopaminergic D1 receptors. Proc Natl Acad Sci USA. 1991;88:1291–5. doi:10.1073/pnas.88.4.1291.
- Zhang D, Zhang L, Tang Y, Zhang Q, Lou D, Sharp FR. Gene expression changes induced by repeated cocaine administration through the dopamine D1 receptors. Neuropsychopharmacology. 2005;30:1443–54. doi:10.1038/sj.npp.1300680.
- Zhang J, Zhang L, Jiao H, Zhang Q, Zhang D, Lou D, Katz JL, Xu M. c-Fos facilitates the acquisition and extinction of cocaine-induced persistent changes. J Neurosci. 2006;26(51):13287–96. doi:10.1523/JNEUROSCI.3795-06.2006.
- Morgan JI, Curran T. Stimulus-transcription coupling in the nervous system: involvement of the inducible proto-oncogenes fos and jun. Annu Rev Neurosci. 1991;14(1):421–51. doi:10.1146/annurev.ne.14.030191.002225.
- Herdegen T, Leah JD. Inducible and constitutive transcription factors in the mammalian nervous system: control of gene expression by Jun, Fos and Krox, and CREB/ATF proteins. Brain Res Brain Res Rev. 1998;28(3):370–490. doi:10.1016/S0165-0173(98)00018-6.
- Lucibello FC, Lowag C, Neuberg M, Müller R. Trans-repression of the mouse c-fos promoter: a novel mechanism of fos-mediated trans-regulation. Cell. 1989;59(6):999–1007. doi:10.1016/0092-8674(89)90756-3.
- Maoz A, Hicks MJ, Vallabhjosula S, Synan M, Kothari PJ, Dyke JP, Ballon DJ, Kaminsky SM, De BP, Rosenberg JB, et al. Adenovirus capsid-based anti-cocaine vaccine prevents cocaine from binding to the nonhuman primate CNS dopamine transporter. Neuropsychopharmacology. 2013;38(11):2170–8. doi:10.1038/npp.2013.114.
- Koetzner L, Deng S, Sumpter TL, Weisslitz M, Abner RT, Landry DW, Woods JH. Titer-dependent antagonism of cocaine following active immunization in rhesus monkeys. J Pharmacol Exp Ther. 2001;296:789–96.