ABSTRACT
The poly(A)-binding protein (PABP) binds to the poly(A) tail of eukaryotic cellular mRNAs and contributes to their stability and translational efficiency. In plants, PABP is expressed from an unusually large gene family grouped into 3 classes that expanded during the evolution of land plants. Subsequent to expansion of the family, members diverged in their primary sequence and in expression. Further expansion of the family and divergence of its members in the Brassicaceae demonstrate the continued dynamic evolution of PABP in plants. In this study, the function of the widely-expressed class II PABP family members was examined to determine how individual class II members contribute to plant growth and development. Of the 3 class II PABP members, PAB2 and PAB4 contribute most to vegetative growth and vegetative-to-floral transition whereas PAB2, and the recently-evolved third class II member, PAB8, contribute to inflorescence and silique growth. Interestingly, although class I and class III PABP members are expressed specifically in reproductive organs, class II PABP members are also necessary for fertility in that the combinatorial loss of PAB2 and either PAB4 or PAB8 expression resulted in reduced fertility. Although all 3 class II members are required for protein expression, PAB4 contributes most to the steady-state level of a reporter mRNA and to protein expression. These findings suggest that class II PABP members are partially overlapping in function but also involved in distinct aspects of plant growth and development.
Introduction
Following transcription and mRNA processing, during which a poly(A) tail is added to most eukaryotic, nuclear-encoded mRNAs, the level of protein expressed is determined by the stability and translational efficiency of an mRNA. The poly(A)-binding protein (PABP), which binds to the poly(A) tail, was first identified as important in determining the stability of an mRNA.Citation1 Subsequently, PABP, which is bound at the 3’-end of an mRNA, was found to promote translation initiation through its physical and functional interaction with the translation initiation factor (eIF) 4G.Citation2-4 eIF4G is a subunit of eIF4F that also contains eIF4E and eIF4A.Citation5 As a result of the binding of eIF4E to the 5′-cap structure, eIF4F is recruited to an mRNA and promotes the binding and subsequent scanning of the 40 S ribosomal subunit to an mRNA as part of translation initiation.Citation5-8 The eIF4E-eIF4G interaction and the eIF4G-PABP interaction bridge the termini of an mRNA to stimulate 40 S subunit recruitment.Citation2,4,9 In plants, the interaction between PABP and eIF4G (or its isoform, eIFiso4G) has been shown to promote the binding of eIF4F to the 5′-cap and binding of PABP to poly(A).Citation10, 11
PABP is highly conserved throughout eukaryotes as is its physical and functional interaction with eIF4G.Citation12 In addition to its role in translation, PABP increases mRNA stability by inhibiting DCP1/2-mediated decapping.Citation13, 14 This may involve destabilization of eIF4E binding to the 5’-cap following loss of PABP during mRNA deadenylation, making the cap more susceptible to DCP1/2. Inhibition of decapping by PABP is also observed in mammals.Citation15 PABP promotes poly(A) nuclease (PAN)-mediated deadenylation but inhibits Caf1/Not1-mediated deadenylation in yeast.Citation13, 14
While yeast expresses just a single and essential PABP,Citation1 PABP in Arabidopsis is expressed by a highly divergent, multigene family composed of 8 members that can be grouped into 3 classes based on sequence similarity and gene structure.Citation16 Three members of the family, i.e., PAB2, PAB4, and PAB8, comprise class II PABP genes which are widely expressed, particularly in vegetative tissues.Citation17-20 Expression of class I genes (PAB3 and PAB5) is restricted to reproductive tissues whereas class III genes (PAB6 and PAB7) exhibit weak and restricted expression.Citation17,18,21 PAB1, originally considered an “orphan gene”Citation17 is actually a class I gene expressed weakly in roots, pollen, and during late embryo development.Citation16,21-23
Analysis of the evolution of the PABP gene family in plants revealed that PABP expanded from a single gene in marine algae to 2 genes in fresh water algae which underwent further expansion to 4 highly similar progenitor genes during the evolution of non-vascular plants.Citation16 All 3 classes of PABP genes present in higher plants today evolved from these progenitor genes. By the appearance of Selaginella moellendorffii, an early vascular plant, the first class III gene (i.e., PAB7) had appeared and class I and II family members first appear in gymnosperms.Citation16 The 3 class II PABP family members of Arabidopsis arose from the single class II PABP gene of Amborella trichopoda, the most recent common ancestor of all extant flowering plants. However, one class II PABP member, PAB8, appears only in the Brassicaceae and its sequence similarity suggests that it is a recent duplication of PAB2.Citation16 Similarly, PAB3 likely arose as a duplication of PAB5 and is present only in the Brassicaceae. PAB1 also appears only in the Brassicaceae, demonstrating a recent expansion of the PABP gene family in these species.Citation16 Finally, the class I PAB6 is present in rosids but not in asterid species.Citation16
The presence of a large gene family containing divergent members in plants and in the Brassicaceae in particular, raises the possibility of functional specialization as PABP is involved in the processing, nucleocytoplasmic transport, stability, and translation of mRNAs. Such a notion is supported by the observation that specific members of the Arabidopsis PABP gene family can complement different functions of PABP in pab1 mutant yeast. Although expression of PAB2, PAB3, and PAB5 in pab1 yeast rescued viability, PAB2 and PAB5 promoted translation initiation and poly(A) shortening in yeast; PAB2, but not PAB5, protected the mRNA 5’-cap against decapping; and PAB2 interacted with eIF4G.18, 19, 24 Despite analyses of plant PABP functions in yeast, the underlying biologic significance of the expansion and divergence of the plant PABP gene family remains unknown. In this study, the roles that the widely-expressed class II members of the Arabidopsis PABP gene family play during growth have been investigated through mutant analysis. Although loss of any single class II member had little effect on vegetative growth, loss of PAB2 and PAB4 expression resulted in a substantial reduction in growth. The combinatorial loss of PAB2 and either PAB4 or PAB8 expression resulted in reduced fertility and altered inflorescence and silique development. Loss of PAB4 expression, in particular, resulted in a substantial reduction in reporter mRNA levels and consequently expression. These results suggest that the roles that the 3 widely-expressed class II members of the Arabidopsis PABP gene family play during growth and development partially overlap but also exhibit specialization.
Results
Expression from PAB2 and PAB4 is required to support vegetative growth
Previous work has shown that the 3 class II members of the PABP gene family are widely expressed including during vegetative growth.Citation17-20 T-DNA insertion lines for PAB2, PAB4, and PAB8 () previously characterized Citation25 were used to confirm the lack of expression of each class II PABP member in the respective mutant line (). The presence of all 3 class II isoforms is detected in wild type (WT) seedlings using Western analysis. PAB4 and PAB8 co-migrate whereas PAB2 appears as a faster-migrating band. The identity of each PABP isoform can also be confirmed in the single and double mutants (). Loss of PAB2 or PAB4 expression did not affect the expression level of the other 2 widely-expressed PABP members although an increase in the level of PAB2 and PAB4 was observed in the pab8 mutant (). A faint band above the major bands was observed, particularly in longer exposures (data not shown). As it appeared in all lanes, it is likely a non-specific band or may be another member of the PABP family.
Figure 1. Mutants with altered expression of the widely-expressed class II PABP isoforms. (A) Gene structure and location of the T-DNA insertion in PAB2, PAB4, and PAB8 genes. Bars indicate exons and bent lines indicate introns. The location of the T-DNA insertion in each class II PABP member is indicated by the arrow head. (B) Western analysis of class II single and double PABP mutants to detect PABP (top panel) and eIF4A (bottom panel). PAB4 and PAB8 comigrate whereas PAB2 migrates just below.
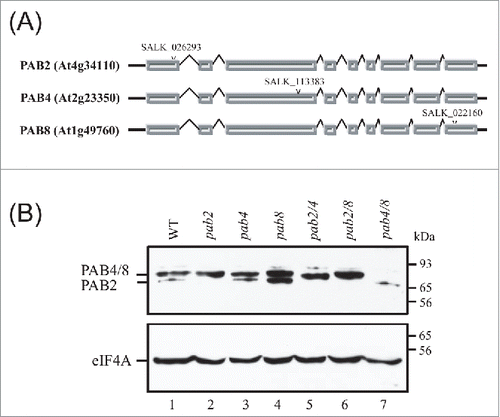
To examine which class II PABP family members contribute to vegetative growth, the growth of single and double mutants was examined. A pab2 pab4 pab8 triple mutant could not be isolated, suggesting that at least one of the 3 PABP members is required for viability. Following the initial appearance of the inflorescence at 3.5 weeks, growth of the single mutants was similar or slightly smaller than wild type (WT) plants as was that of pab4 pab8 double mutant plants (). Little difference in the size of other organs, such as siliques () or inflorescences () was observed. In contrast, growth of the pab2 pab8 double mutant was moderately smaller and pab2 pab4 plants were substantially smaller than WT (). The difference in plant stature of the pab2 pab8 and pab2 pab4 double mutants was also observed in leaf size (). pab2 pab4 plants also had fewer leaves and, as a consequence, underwent a premature vegetative-to-floral transition whereas the leaf number and floral transition of pab2 pab8 plants was unaffected (). pab2 pab4 siliques were smaller than WT and exhibited an enlarged distal end relative to WT siliques (). The siliques of pab2 pab8 plants were slightly shorter than WT but also exhibited an enlarged distal end. The siliques of pab4 pab8 plants were similar to WT.
Figure 2. Growth characteristics of pab mutants. (A) Growth of class II single and double PABP mutants at 3.5 weeks just before the appearance of the inflorescence relative to wild-type plants. (B) All leaves from adult wild-type and mutant plants at the appearance of the inflorescence. (C) Mature siliques from adult wild-type and mutant plants.
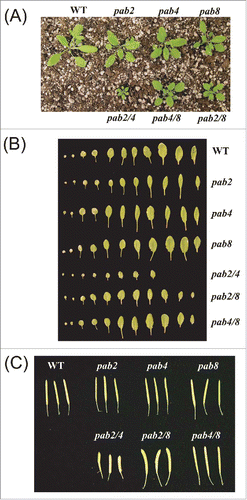
Figure 3. The effect of pab mutants on inflorescence growth. Flowering wild-type and class II PABP mutant plants with the inflorescence displayed.
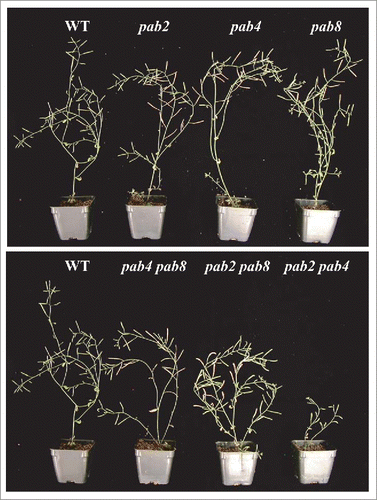
Following completion of flowering, the inflorescences of the mutants were compared. Those of the single mutants and pab4 pab8 plants were similar to WT plants (). The inflorescence of pab2 pab8 plants was more compact as a result of a shorter internode length between siliques (). Combinatorial loss of PAB2 and PAB4 expression resulted in a substantially smaller inflorescence, consistent with the overall smaller stature of the double mutant. These observations suggest that loss of any single class II PABP member has little effect on vegetative growth suggesting a degree of functional overlap. Loss of PAB2 and PAB4 expression, however, resulted in a severe reduction in plant size and an earlier vegetative-to-floral transition. As pab4 pab8 plants exhibit no reduction in growth relative to WT plants, this indicates that PAB2, in particular, as well as PAB4 contribute most to growth.
Expression from PAB2 is required for fertility
PAB2, PAB4, and PAB8 are widely-expressed members of the PABP family while the expression of PAB3 and PAB5 is restricted to reproductive organs.Citation17, 18, 21 To examine whether class II PABP family members may also be important in reproduction, the fertility of the single and double class II PABP mutants was examined. Flowers of the single and double PABP mutants appeared similar to WT plants (). The number of seed per silique (i.e., successful and failed seed per silique) and seed set (i.e., the percent of ovaries that were fertilized and produced mature seed) of pab2, pab4, and pab8 plants were similar to WT plants as was the number of siliques per plant (). Similar results were obtained with the pab4 pab8 double mutant. In contrast, the number of seed per silique and seed set of pab2 pab8 and pab2 pab4 plants was substantially reduced relative to WT plants (). Dissection of siliques during embryogenesis confirmed the reduced fertility of the pab2 pab8 and pab2 pab4 mutants (). Those ovules that failed to result in a seed were either infertile or failed during early embryogenesis as the ovules failed to expand at those positions following pollination. The smaller size and enlarged distal end of the siliques was again observed in the pab2 pab8 and pab2 pab4 mutants. Therefore, loss of PAB2 expression in conjunction with another class II PABP resulted in reduced fertility and altered silique morphology. As pab4 pab8 plants exhibited normal fertility and silique development, this suggests that PAB2 contributes specifically to fertility and normal silique growth whereas the contribution that the other class II PABP members make is less specific.
Figure 4. Flower, silique, and embryo development of pab mutants. Flowers (top panels) and dissected siliques (bottom panels) showing developing embryos are shown for wild-type and class II PABP mutants. Bar represents 5 mm.
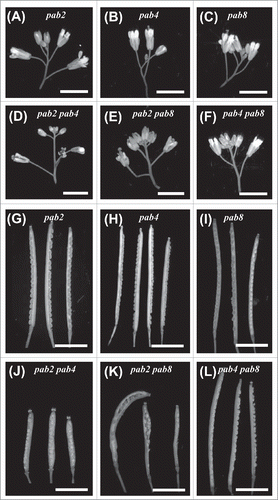
Table 1. Requirement for class II PABP members during seed development.
To examine the requirement for PAB2 in the context of a double mutant in further detail, the fertility of double mutant plants in which one class II PABP gene is null while the second class II PABP gene is present as a heterozygous mutation was examined. Flowers and siliques of these combinatorial mutants were similar in appearance to WT (). PAB4+/− pab2 plants exhibited a significant reduction in seed set () similar to the reduction in seed set observed in the pab2 pab4 mutant (). The reduction in fertility could be seen during embryogenesis (). In contrast, PAB2+/− pab4 plants exhibited seed set similar to WT plants ( and ). PAB8+/− pab2 plants also exhibited a significant reduction in seed set ( and ) similar to the reduction in seed set observed in the pab2 pab8 mutant () whereas PAB2+/− pab8 plants exhibited seed set similar to WT plants ( and ). PAB8+/− pab4 and PAB4+/− pab8 plants exhibited normal seed set ( and ). These results demonstrate that in the absence of PAB2 expression, fertility is reduced when combined with either pab4 or pab8 or when either of the latter two is present in a heterozygous state. As the presence of pab2 in a heterozygous state in combination with either pab4 or pab8 fails to reduce fertility, these findings support the notion that PAB2 is specifically required in supporting fertility whereas PAB4 or PAB8 play a less specific role.
Figure 5. Flower, silique, and embryo development of heterozygous pab mutants. Flowers (top panels) and dissected siliques (bottom panels) showing developing embryos are shown for wild-type and heterozygous class II PABP mutants. Bar represents 5 mm.
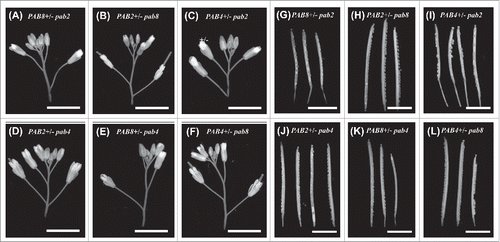
Table 2. Effect of heterozygous class II PABP members during seed development.
All class II PABP members contribute to root growth
To examine how each class II PABP member contributes to root growth, the class II PABP mutants were examined in a root growth assay in which seed were germinated on medium and allowed to grow in a vertical position for 4 d at which point root length was measured. The roots of pab2, pab4, and pab8 mutants were significantly longer than WT plants (). Roots of the pab2 pab8 and pab4 pab8 mutants were also significantly longer than WT plants and while roots of pab2 pab4 plants were significantly longer than WT plants during early seedling growth, the difference was less than that observed for the other single and double class II PABP mutants (). The relatively shorter length of pab2 pab4 mutant roots may be a consequence of the smaller overall stature of this double mutant. These data indicate that class II PABP members serve to negatively regulate root growth or may be a compensatory effect of the slight reduction in growth of the aerial parts of the mutants.
Table 3. Class II PABP members negatively regulate root growth.
Expression from PAB4 is required for reporter gene expression and mRNA stability
PABP promotes expression from an mRNA.Citation12 To determine the extent to which any class II PABP member might contribute to gene expression, the expression from the firefly luciferase (Luc) reporter gene was examined in each class II PABP mutant. To this end, Arabidopsis containing the Luc cDNA under the control of the CaMV promoter as described previously Citation26 was crossed with the class II single and double PABP mutants. Progeny homozygous for the Luc transgene and null for each class II PABP mutation, either as single or double mutants, were isolated. The introduction of the Luc reporter through crosses thereby avoided position effects of the transgene in the genome. Luciferase activity was then measured in seedlings, adult leaves, and flowers to determine the effect of each class II PABP on expression. In 8 day old pab2 seedlings, expression from the Luc reporter, representing total steady-state luciferase activity normalized for equal amounts of total protein, was reduced approximately 4-fold relative to WT seedlings (). In the pab8 mutant, Luc expression was reduced approximately 5-fold. In pab4 plants, however, Luc expression was reduced approximately 66-fold (). Luc expression in the pab2 pab8, pab4 pab8, and pab2 pab4 double mutants was reduced approximately 63-, 666-, and 702-fold, respectively. Similar results were observed in adult leaves and flowers (). These results indicate that all class II PABP members contribute to expression in seedlings but that expression from PAB4 makes the greatest contribution.
Table 4. Class II PABP members are required to support expression from Luc mRNA.
The luciferase expression data represent the contributions of the translatability and stability of the mRNA. As PABP is known to affect transcript stability,Citation13 the transcript level of Luc mRNA in each single and double class II PABP mutant was measured by qPCR to determine whether the reduction in Luc expression observed in the mutants may be explained by a corresponding reduction at the RNA level. Luc transcript level was modestly reduced in the pab2 mutant, reduced approximately 6-fold in the pab8 mutant, but was reduced over 70-fold in the pab4 mutant (). Luc expression in the pab2 pab8, pab4 pab8, and pab2 pab4 double mutants was reduced approximately 20-, 22-, and 29-fold, respectively. These data indicate that loss of a class II PABP member results in reduced transcript levels and that loss of PAB4 expression had a substantial impact on transcript levels. The reduction in transcript levels in the class II PABP mutants does not fully account for the reduction at the level of Luc expression. The difference is likely the effect that loss of one or more class II PABP member has on the translatability of the mRNA. Similar results were observed in adult leaves and in flowers although the reduction in transcript levels was even greater in the latter (). These results suggest that all 3 class II PABP members contribute to maintaining Luc transcript levels in these organs but that expression from PAB4 is particularly important in this regard.
Table 5. Class II PABP members are required to support Luc transcript level.
In addition to eIF4G, plants express an isoform called eIFiso4G that differs substantially in size and sequence from eIF4G but PABP interacts with both.Citation27,28 Arabidopsis expresses 2 eIFiso4G isoforms (eIFiso4G1 and eIFiso4G2) as do other species throughout the Brassicaceae whereas species outside the Brassicaceae express only eIFiso4G1 isoforms.Citation26 eIFiso4G1 and eIFiso4G2 exhibit substantial sequence divergence in those species that express both isoforms. Translation from Luc mRNA containing the 5′-leader sequence from tobacco mosaic virus (TMV) (known as Ω) preferentially uses eIF4G and eIFisoG2 over eIFisoG1 in Arabidopsis.Citation26 As the presence of the Ω 5′-leader sequence alters the requirements for translation factors for Luc mRNA, expression from the Ω-Luc mRNA was examined in the class II PABP mutants. Arabidopsis containing the Ω-Luc transgene as described previously Citation26 was crossed with the single and double class II PABP mutants to avoid position effects of the transgene in the genome. Progeny homozygous for the Ω-Luc transgene and null for each class II PABP mutation, either as single or double mutants, were then isolated and luciferase expression was measured in seedlings, adult leaves, and flowers. Although the presence of the Ω 5′-leader sequence resulted in a greater reduction in luciferase expression in the class II PABP mutants than observed in its absence, the greatest reduction was observed in the absence of PAB4 expression, in particular, or in the absence of PAB2 expression (). Similar results were observed in adult leaves and flowers (. The transcript level of Ω-Luc mRNA in each single and double class II PABP mutant was also measured by qPCR. The Ω-Luc transcript level was reduced to a greater extent and exhibited less preferential dependence for any one class II PABP () than did Luc mRNA (). These results suggest that the requirement for specific class II PABP members to maintain the transcript level can differ among mRNAs, even those that differ only in 5′-leader sequence although PAB4 can be important in supporting overall reporter gene expression.
Table 6. Class II PABP members are required to support expression from Ω-Luc mRNA.
Table 7. Class II PABP members are required to support W-Luc transcript level.
To determine whether loss of one class II PABP member affects the expression of the remaining members, qPCR was performed on the mutants to measure the relative levels of each class II PABP member. As expected, little expression from PAB2 was observed in the pab2, pab2 pab8, and pab2 pab4 mutants (). The level of PAB2 expression in the pab4, pab8¸ and pab4 pab8 mutants was similar to that in WT plants. Similarly, little to no expression of PAB4 was detected in those lines containing the pab4 mutation and its expression was not substantially altered in the pab2, pab8¸ and pab2 pab8 mutants (). Little to no expression of PAB8 was detected in those lines containing the pab8 mutation and its expression was not consistently altered in the other mutants in that it was moderately lower in the pab4 mutant but moderately higher in the pab2 pab4 mutant (). These results are in good agreement with the protein levels of the class II PABP members observed by Western analysis () and suggest that the reduction in Luc expression following loss of expression of one class II PABP member was not a result of a substantial decrease in the expression of the remaining members.
Figure 6. Expression from class II PABP genes in class II PABP mutants. qPCR was performed for PAB2 (black bars), PAB4 (gray bars), and PAB8 (white bars) in 8 day old WT seedlings and class II PABP mutants. Expression for each gene is reported relative to the level in WT seedlings which is set at a value of 1.
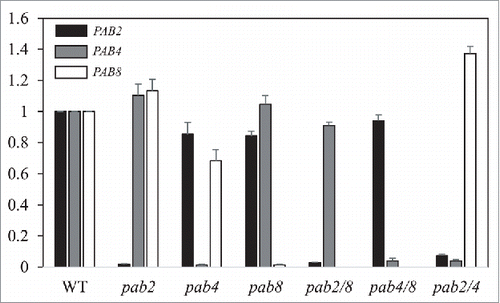
Discussion
The PABP gene family expanded from a single gene in the marine algae Chlamydomonas reinhardtii to 2 genes in fresh water algae (Klebsormidium flaccidum) to 4 genes in Physcomitrella patens, the first known non-vascular plant species.Citation16 The 4 PABP genes in P. patens represent 2 pairs of genes that share similar gene structure, likely arising from duplication of an earlier 2 member gene family such as is present in fresh water algae. The considerable similarity of the PABP proteins in P. patens, however, suggests similar function. The 3 classes of PABP genes present in higher plants likely arose from these 4 progenitor genes in P. patens. Although a class III PABP gene, PAB7, was the first to appear in Selaginella moellendorffii and has been maintained throughout plant evolution,Citation16 its function has not been examined. Expression of the Arabidopsis PAB7 homolog was detected only using RT-PCR and was restricted largely to specific floral organs,Citation17 suggesting a reproductive role.
Class II and class III members, first observed in gymnosperms and in the basal angiosperm, Amborella trichopoda, likely evolved independently from progenitor PABP genes and both classes expanded with plant evolution, particularly within the Brassicaceae.Citation16 The present study focused on the function of class II PABP genes as these are widely expressed whereas expression of class I and class III members are restricted to either reproductive organs or roots.Citation16,17 PAB2 and PAB4 were observed to contribute most to vegetative growth as loss of expression from both resulted in the greatest reduction in growth in Arabidopsis. Loss of expression from both also resulted in an early vegetative-to-floral transition. That loss of expression from either PAB2 or PAB4 had little effect on vegetative growth or appearance of the inflorescence suggests that PAB2 and PAB4 functionally overlap in this respect. The observation that the pab4 pab8 mutant exhibits no reduction in growth or inflorescence appearance and that growth of pab2 pab8 plants is reduced only modestly with no change in the vegetative-to-floral transition suggests that expression from PAB2 makes a more significant contribution to these developmental aspects than does PAB4. Although only a single class II PABP gene is present in basal eudicots and in the early angiosperm, Aquilegia caerulea, PAB2-like and PAB4-like class II genes are present in Vitis vinifera and in Solanum species, suggesting duplication of the single class II gene before the appearance of these species and divergence into the 2 main class II isoforms.Citation16 As expression from PAB2 and PAB4 are required for full vegetative growth and normal vegetative-to-floral transition, the expansion of the class II subfamily resulted in both class II members contributing to these functions. PAB8, the third class II gene in Arabidopsis, appears only in the Brassicaceae, and as its encoded protein is most similar to PAB2, it likely arose from a duplication of PAB2.Citation16 Vegetative growth or the vegetative-to-floral transition in Arabidopsis is not greatly dependent on PAB8 as the combinatorial loss of expression from PAB4 and PAB8 has no effect on growth or the appearance of inflorescence and the combinatorial loss of expression from PAB2 and PAB8 results in only a modest reduction in growth with no change in the vegetative-to-floral transition.
In contrast to the effects that loss of class II PABP expression can have on growth of aerial tissues, root growth was enhanced in class II PABP mutants. This suggests that class II PABP members may negatively regulate root growth. Expression of PAB1, a class I member recently evolved in the Brassicaceae, is predominantly restricted to roots and PAB7, a class III member, may be expressed in roots as well.Citation16,21 It is possible that class II PABP members affect expression of growth-related genes in roots directly. It is also possible that a balance between class II PABP members and those class I or class III members expressed in roots is necessary to achieve correct root growth.
Expression from PAB2 contributes significantly to silique morphology in that pab2 pab8 or pab2 pab4 mutants exhibit siliques with an enlarged distal end. The fact that this is not observed in pab2 mutants suggests that loss of PAB2 expression is insufficient and that loss of another class II member is required. However, as this phenotype is not observed in the pab4 pab8 mutant suggests that PAB2 is specifically required whereas the requirement for expression from PAB4 or PAB8 is less specific. Similarly, expression from PAB2 contributes significantly to inflorescence architecture as loss of PAB2 and PAB8 results in a shorter internode distance between siliques that is not observed in pab4 or pab4 pab8 mutants. Although this may suggest that expression from PAB8 is also specifically required for maintaining appropriate internode distance, the pab2 pab4 mutant also exhibits shorter internode distance. Whether this is a consequence of the substantially smaller overall stature of the pab2 pab4 mutant (which would support the notion that PAB8 specifically contributes to inflorescence architecture) or that loss of either PAB4 or PAB8 expression in conjunction with loss of PAB2 expression results in reduced internode length (which would suggest that the contribution of PAB4 and PAB8 is less specific than PAB2) cannot be resolved at this point.
Reduced fertility was observed to a similar extent in pab2 pab8 and pab2 pab4 mutants whereas any single class II mutant or the pab4 pab8 double mutant remained fully fertile. As no ovule expansion was observed at those positions that failed to result in a seed, this suggests ovule infertility or failure during early embryogenesis. Reduced fertility was also observed in PAB4+/− pab2 and PAB8+/− pab2 plants but not in PAB2+/− pab4 or PAB2+/− pab8 plants, supporting the notion that that PAB2 expression contributes specifically to fertility whereas PAB4 or PAB8 play a non-specific, supporting role. The requirement for class II PABP members during reproduction is unexpected as it is the class I members, PAB3 and PAB5, as well as the class III member, PAB7, whose expression is restricted to reproductive tissues,Citation17,18,21 and might be expected to be solely responsible for fertility.
Although loss of any one class II member resulted in no visible growth phenotype, Luc reporter expression was reduced. Loss of expression from either PAB2 or PAB8 resulted in a moderate reduction in reporter expression whereas a substantial reduction was observed following loss of PAB4 expression. The reduction in reporter expression was even greater in class II double mutants although the reduction was greater in pab2 pab4 and pab4 pab8 than in pab2 pab8 plants, supporting the conclusion that PAB4 expression makes the most substantial contribution to protein expression. Correlating with the reduction in protein expression, loss of any one class II PABP, and PAB4 in particular, resulted in a decrease in the level of the reporter mRNA. These data indicate that PAB4 expression contributes most to the steady-state level of an mRNA and consequently to its expression at the protein level. The presence of the Ω 5′-leader sequence, which has been shown to alter the requirements for translation factors for Luc mRNA Citation26 altered the effect of single class II mutants on expression in that loss of either PAB2 or PAB4 resulted in a reduction in reporter expression, suggesting that mRNAs differing in just the 5′-leader sequence can exhibit differences in their requirements for class II PABP members.
Whether PAB4 and the other class II PABP members contribute to maintaining reporter mRNA levels directly or affect expression of factors that indirectly influence transcript levels is unknown but a combination of direct and indirect effects may be likely for a general expression factor such as PABP that is involved in multiple aspects of mRNA biology. The observation that loss of one class II PABP member does not substantially affect the transcript levels of the remaining class II PABP members supports the notion that loss of a class II PABP protein can affect the transcript levels of different mRNAs to different extents.
These findings suggest that class II PABP gene members contribute to vegetative growth, vegetative-to-floral transition, inflorescence and silique development, fertility, root growth, and protein expression but that specific members make different contributions to these processes. From the findings presented in this study, the role of the 3 class II members in supporting vegetative growth can be ranked as PAB2>PAB4>PAB8 whereas expression from PAB2 and PAB4 but not PAB8 regulates the vegetative-to-floral transition. For silique and possibly inflorescence development, the ranking is likely PAB2>PAB4 = PAB8 as it is for fertility. In contrast, the role of each class II member in maintaining the level of Luc mRNA and consequently luciferase protein expression is PAB4>PAB8≥PAB2 and for Ω-Luc mRNA, PAB4≥PAB2>PAB8. Therefore, although functional overlap exists among the class II PABP members, they also exhibit specialization in distinct aspects of plant growth and development. Consistent with their earlier appearance during plant evolution, PAB2 and PAB4 are more important in supporting the fundamental aspects of plant development, i.e., supporting vegetative growth, vegetative-to-floral transition, and protein expression. PAB8 is a class II member that is absent outside the Brassicaceae and might be expected to play perhaps only a minor role in Arabidopsis, for example in influencing silique and inflorescence development. However, PAB8 expression is needed to support full fertility. As expression from PAB4 has a similar role in maintaining fertility as does PAB8 in a pab2 mutant, the contribution that PAB4 or PAB8 makes in this regard can be viewed as a non-specific, supporting role, particularly when the full fertility of the pab4 pab8 mutant is considered.
How the class II PABP members regulate these aspects of plant growth and development remains to be determined. For a protein that is involved in diverse aspects of gene expression, including the processing, transport, stability, and translation of mRNAs, specific class II PABP members may affect different aspects of gene expression to different extents. It is also possible that specific class II PABP members may regulate the expression of different mRNAs if the divergence that exists among the class II PABP members Citation16 affects their binding to mRNAs or partner proteins such as eIF4G or eIFiso4G isoforms.
The recent expansion of the PABP gene family in the Brassicaceae is notable given that the class II subgroup expanded from 2 to 3 members whereas the class III subgroup expanded from a single gene to 3 members.Citation16 The expansion of the class I subgroup from one to 2 members that occurred with the appearance of rosids demonstrates that expansion of the PABP gene family has accompanied most of plant evolution. Although the specific advantages that such expansion of the gene family within the Brassicaceae may confer are unknown, the analysis of the roles of the widely-expressed class II members play illustrate involvement of the 3 members in specific aspects of plant growth and development in ways that are partially overlapping but are also distinct.
Materials and methods
Plant growth conditions
Arabidopsis seeds were imbibed and cold-treated for 4 d before germination in soil. Plants were grown in a growth room supplemented with Sylvania Gro-Lite fluorescent bulbs (Sylvania, Danvers, MA) at a photon flux density (PFD) of 100 μmol photons m−2s−1.
Root growth assay
Seeds of each line were surface sterilized with 70% (v/v) ethanol for 30 s followed by 50% (v/v) bleach with approximately 1.5% (v/v) Tween 20 and washed 4 times in sterilized water. The seed were placed along a line on 0.25 x Murashige and Skoog (MS) medium agar petri plates supplemented with 1% (w/v) sucrose. The plates containing the seed were cold treated for 4 d and transferred to continuous light (50 μmol m−2s−1) at 22°C in a vertical position to allow the seed to germinate. Root lengths were measured following 4 d of growth. The root lengths of 20–30 seedlings were measured and the average and standard deviation reported.
Western analysis
Protein extracts were resolved using standard SDS-PAGE and the protein transferred to 0.22 mm PVDF membrane by electroblotting. Following transfer, the membranes were blocked in 5% milk in TPBS (0.1% TWEEN 20, 137 mM NaCl, 2.7 mM KCl, 10 mM Na2HPO4, 1.4 mM KH2PO4, pH 7.4) followed by incubation with antiserum raised against PABP or eIF4A diluted 1:250 in TPBS with 1% milk for 1.5 hrs. The blots were then washed twice with TPBS and incubated with goat anti-rabbit horseradish peroxidase-conjugated antibodies (Southern Biotechnology Associates, Inc.) diluted 1:20,000 for 1 hr. The blots were washed twice with TPBS and the signal detected using chemiluminescence (Amersham Corp).
Luciferase assay
Plant samples were ground in liquid nitrogen and the soluble protein fraction resuspended in luciferase assay buffer (25 mM Tricine pH 8, 5 mM MgCl2, 0.1 mM EDTA supplemented with 33.3 mM DTT, 270 µM coenzyme A, 500 µM ATP). Aliquots of total cell extract were assayed for luciferase activity following injection of 0.5 mM luciferin using a Monolight 2010 Luminometer (Analytical Luminescence Laboratory). Triplicate plant samples were prepared for each line and the average value reported. All luciferase expression data are normalized for equal amounts of total protein.
PCR analysis
DNA was isolated by quick-freezing plant material in liquid nitrogen, grounding to a fine powder, and resuspending in 400 μl extraction buffer (100 mM Tris-Cl pH 9.0, 20 mM EDTA, 200 mM NaCl, 1% Sarcosyl, and 1% β-ME). Following centrifugation, the supernatant was extracted with 400 μl phenol: chloroform (1:1) and centrifuged to separate the phases. The DNA was precipitated from the aqueous phase by sodium acetate and isopropyl alcohol, washed with 75% ethanol and resuspended in H2O. PCR amplification was performed in 20 µl reactions containing 1 x PCR buffer, 0.4 u Taq DNA polymerase (Bioneer Inc. Alameda, CA), 250 µM dNTPs, 10 µM forward and reverse primers, and 50 ng genomic DNA. Reactions were performed using the following conditions: 95°C/5 min (1 cycle); 95°C/30 sec, 55°C/30 sec, 72°C/1 min (35 cycles); and a final extension at 72°C/5 min (1 cycle). To detect the presence of PAB2, a forward primer, PAB2-LP (5′-AACGGCTGAGATCAATCTCAC-3′), and a reverse primer, PAB2-RP (5′-GCGCTGGCAACATTTTTATTA-3′), were used and for pab2, PAB2-RP and LBb1.3 (5′- ATTTTGCCGATTTCGGAAC-3′) were used. To detect the presence of PAB4, a forward primer, PAB4-LP (5′-TGGGGTTGGGAATTTGTTTGT-3′), and a reverse primer, PAB4-RP (5′-GCCTTCTCTGCTGCCAGTGAA-3′), were used and for pab4, PAB4-RP and LBb1.3 were used. To detect the presence of PAB8, a forward primer, PAB8-LP (5′-TCATGCCTATGATGCAACAAG-3′), and a reverse primer, PAB8-RP (5′-GGTTTAAAAGCTTCGAATGATCA-3′), were used and for pab8, PAB8-RP and LBb1.3 were used.
qPCR analysis
Plant material was frozen in liquid nitrogen and ground to a fine powder, and 100 mg was resuspended in 1 ml of TRIzol® reagent (Invitrogen). Following centrifugation, the supernatant was extracted with 200 μl of chloroform and centrifuged to separate the phases. RNA was precipitated from the aqueous phase using isopropyl alcohol, and the RNA pellet was washed with 75% ethanol and resuspended in RNase-free H2O. 1 μg of RNA was used to obtain the first-strand cDNA by Omniscript RT kit (Qiagen) in a 20-μl reaction. The qPCR analysis was performed using a iQ5 real time PCR detection system (Bio-Rad) in 25-μl reactions containing 1× SYBR Green SuperMix 500 nM forward and reverse primers and 10 ng of cDNA. Reactions were performed using the following conditions: 95 °C for 5 min (1 cycle) and 95 °C for 30 s, 55 °C for 30 s, and 72 °C for 30 s (35 cycles). Protein phosphatase PP2A (At1g13320) were used as the reference gene for the quantitation of Luc expression in Arabidopsis leaves. To detect the expression of PP2A, a forward primer, PP2A-FW (5′-AGTATCGCTTCTCGCTCCAG-3′) and a reverse primer, PP2A-RV (5′-GTTCTCCACAACCGCTTGGT-3′), were used. The efficiency of PCR was determined by 5 10-fold serial dilutions of the template DNAs in triplicate.
Abbreviation
DTT | = | dithiothreitol |
eIF | = | translation initiation factor |
GST | = | glutathione-S-transferase |
HEATHuntington, Elongation Factor 3, PR65/A | = | Huntington, Elongation Factor 3, PR65/ATOR |
His | = | histidine |
IPTG | = | isopropyl β-D-1-thiogalactopyranoside |
PABP | = | poly(A) binding protein |
RRM | = | RNA recognition motif |
Disclosure of potential conflicts of interest
No potential conflicts of interest were disclosed.
Acknowledgments
This work was supported by grants from the National Science Foundation (DBI-0820047) and the University of California Agricultural Experiment Station to D.R.G.
References
- Sachs AB, Davis RW, Kornberg RD. A single domain of yeast poly(A)-binding protein is necessary and sufficient for RNA binding and cell viability. Mol Cell Biol 1987; 7:3268-76; PMID:3313012; https://doi.org/10.1128/MCB.7.9.3268
- Tarun SZ, Sachs AB. Association of the yeast poly(A) tail binding protein with translation initiation factor eIF-4G. EMBO J 1996; 15:7168-77. PMID:9003792
- Le H, Tanguay RL, Balasta ML, Wei C-C, Browning KS, Metz AM, Goss DJ, Gallie DR. Translation initiation factors eIF-iso4G and eIF-4B interact with the poly(A)-binding protein and increase its RNA binding activity. J Biol Chem 1997; 272:16247-55; PMID:9195926; https://doi.org/10.1074/jbc.272.26.16247
- Wells SE, Hillner PE, Vale RD, Sachs AB. Circularization of mRNA by eukaryotic translation initiation factors. Mol Cell 1998; 2:135-40; PMID:9702200; https://doi.org/10.1016/S1097-2765(00)80122-7
- Hinnebusch AG. The scanning mechanism of eukaryotic translation initiation. Annu Rev Biochem 2014; 83:779-812; PMID:24499181; https://doi.org/10.1146/annurev-biochem-060713-035802
- Preiss T, Hentze M. Starting the protein synthesis machine: eukaryotic translation initiation. Bioessay 2003; 10:1201-11; https://doi.org/10.1002/bies.10362
- Kapp LD, Lorsch JR. The molecular mechanics of eukaryotic translation. Annu Rev Biochem 2004; 73:657-704; PMID:15189156; https://doi.org/10.1146/annurev.biochem.73.030403.080419
- Pestova TV, Lorsch JR, Hellen CUT. The mechanism of translation initiation in eukaryotes. In: Mathews M.B., Sonenberg N., Hershey J.W.B. eds. Translational Control in Biology and Medicine. Cold Spring Harbor, NY: Cold Spring Harbor Laboratory Press, 2007:87-128.
- Tarun SZ, Sachs AB. A common function for mRNA 5′ and 3′ ends in translation initiation in yeast. Genes and Devel 1995; 9:2997-3007; https://doi.org/10.1101/gad.9.23.2997
- Wei C-C, Balasta ML, Ren J, Goss DJ. Wheat germ poly(A) binding protein enhances the binding affinity of eukaryotic initiation factor 4F and (iso)4F for cap analogues. Biochem 1998; 37:1910-6; https://doi.org/10.1021/bi9724570
- Bi XP, Ren JH, Goss DJ. Wheat germ translation initiation factor eIF4B affects eIF4A and eIFiso4F helicase activity by increasing the ATP binding affinity of eIF4A. Biochem 2000; 39:5758-65; https://doi.org/10.1021/bi992322p
- Gallie DR. The role of the poly(A) binding protein in the assembly of the cap-binding complex during translation initiation in plants. Translation 2014; 2:e959378; PMID:26779409; https://doi.org/10.4161/2169074X.2014.959378
- Caponigro G, Parker R. Multiple functions for the poly(A) binding protein in mRNA decapping and deadenylation in yeast. Genes Develop 1995; 9:2421-32; PMID:7557393; https://doi.org/10.1101/gad.9.19.2421
- Wilusz CJ, Gao M, Jones CL, Wilusz J, Peltz SW. Poly(A)-binding proteins regulate both mRNA deadenylation and decapping in yeast cytoplasmic extracts. RNA 2001; 7:1416-24; PMID:11680846
- Gao M, Wilusz CJ, Peltz SW, Wilusz J. A novel mRNA decapping activity in HeLa cytoplasmic extracts is regulated by AU-rich elements. EMBO J 2001; 20:1134-43; PMID:11230136; https://doi.org/10.1093/emboj/20.5.1134
- Gallie DR, Liu R. Phylogenetic analysis reveals dynamic evolution of the poly(A)-binding protein gene family in plants. BMC Evol Biol 2014; 14:238; PMID:25421536; https://doi.org/10.1186/s12862-014-0238-4
- Belostotsky DA. Unexpected complexity of poly(A)-binding protein gene families in flowering plants: three conserved lineages that are at least 200 million years old and possible auto-cross-regulation. Genetics 2003; 163:311-9. PMID:12586718
- Belostotsky DA, Meagher RB. A pollen-, ovule-, and early embryo-specific poly(A) binding protein from Arabidopsis complements essential functions in yeast. Plant Cell 1996; 8:1261-75; PMID:8776896; https://doi.org/10.1105/tpc.8.8.1261
- Palanivelu R, Belostotsky DA, Meagher RB. Conserved expression of Arabidopsis thaliana poly (A) binding protein 2 (PAB2) in distinct vegetative and reproductive tissues. Plant J 2000; 22:199-210; PMID:10849338; https://doi.org/10.1046/j.1365-313x.2000.00720.x
- Hilson P, Carroll KL, Masson PH. Molecular characterization of PAB2, a member of the multigene family coding for poly(A)-binding proteins in Arabidopsis thaliana. Plant Physiol 1993; 103:525-33; PMID:8029336; https://doi.org/10.1104/pp.103.2.525
- Belostotsky DA, Meagher RB. Differential organ-specific expression of three poly(A)-binding protein genes from Arabidopsis thaliana. Proc Natl Acad Sci USA 1993; 90:6686-90; PMID:8341686; https://doi.org/10.1073/pnas.90.14.6686
- Ford L, Bagga PS, Wilusz J. The poly(A) tail inhibits the assembly of a 3’-to-5’ exonuclease in an in vitro RNA stability system. Mol Cell Biol 1997; 17:398-406; PMID:8972220; https://doi.org/10.1128/MCB.17.1.398
- Minvielle-Sebastia L, Preker PJ, Wiederkehr T, Strahm Y, Keller W. The major yeast poly(A)-binding protein is associated with cleavage factor IA and functions in premessenger RNA 3’-end formation. Proc Natl Acad Sci USA 1997; 94:7897-902; PMID:9223284; https://doi.org/10.1073/pnas.94.15.7897
- Chekanova JA, Shaw RJ, Belostotsky DA. Analysis of an essential requirement for the poly(A) binding protein function using cross-species complementation. Curr Biol 2001; 11:1207-14; PMID:11516954; https://doi.org/10.1016/S0960-9822(01)00371-2
- Dufresne PJ, Ubalijoro E, Fortin MG, Laliberté JF. Arabidopsis thaliana class II poly(A)-binding proteins are required for efficient multiplication of turnip mosaic virus. J Gen Virol 2008; 89:2339-48; PMID:18753244; https://doi.org/10.1099/vir.0.2008/002139-0
- Gallie DR. Eukaryotic initiation factor eIFiso4G1 and eIFiso4G2 are isoforms exhibiting distinct functional differences in supporting translation in Arabidopsis. J Biol Chem 2016; 291:1501-13; PMID:26578519; https://doi.org/10.1074/jbc.M115.692939
- Cheng S, Gallie DR. Competitive and noncompetitive binding of eIF4B, eIF4A, and the poly(A) binding protein to wheat translation initiation factor eIFiso4G. Biochem 2010; 49:8251-65; https://doi.org/10.1021/bi1008529
- Cheng S, Gallie DR. Eukaryotic initiation factor 4B and the poly(A)-binding protein bind eIF4G competitively. Translation 2013; 1:e24038; PMID:26824014; https://doi.org/10.4161/trla.24038