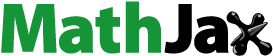
ABSTRACT
This study assesses land-use/land cover (LULC) change in the southwestern region of The Gambia, using remotely sensed satellite imagery. Using a geographic information system (GIS) software, I conducted a pixel-based supervised LULC classification applying the Random Forest Algorithm. I further used the classified LULC maps as part of the input parameters required to run the Integrated Valuation of Ecosystem Services and Tradeoffs (InVEST) Carbon Storage and Sequestration model. This study attributes the critical drivers of LULC change to deforestation, urbanization, industrial expansion, real estate development, climate change impacts, timber extraction, migration, and population density. Some of the factors are necessary for economic growth but not suitable for sustainable growth or development. The analysis reveals a forest cover loss of 22,408 ha (18% decrease) from 1985 to 2020 in the study area. The deforestation and other LULC changes between 2003 and 2020 have contributed to 21,824 metric tons of carbon emissions. The carbon loss is equivalent to an economic value ranging from US$521,526 to US$6,899,830. The study also presents three LULC projection (2020–2050) scenarios, namely, a business-as-usual (BAU), a new capital city (NCC) development, and a sustainability (SUST) pathway. Consideration of the study recommendations will minimize deforestation, increase carbon sequestration rate, support climate change mitigation, and provide adaptation co-benefits (e.g. urban flood mitigation).
Introduction
Land is a principal natural capital on which economic, social, ecological, and other human activities occur. Land-use is simply the anthropogenic activities carried out on the terrestrial surface. Land cover is the biophysical attributes and human structures of the Earth’s surface, including several terrestrial ecosystems (Agarwal et al., Citation2002; Ali Bah et al., Citation2019). Land-use/land cover (LULC) change is as old as human existence (Abere et al., Citation2020; Ali Bah et al., Citation2019; Lambin et al., Citation2003). In Sub-Saharan Africa, LULC compositions are rapidly changing, particularly from forests to developed or urban settlements. These changes are due to increasing population growth, agricultural production, and other economic activities for growth–necessary to reduce poverty (Abere et al., Citation2020; Ali Bah et al., Citation2019; Gashaw et al., Citation2017; Rawat & Kumar, Citation2015). This paper seeks to assess and evaluate the spatio-temporal variability of LULC dynamics in The Gambia, focusing on forest cover and urban land-use changes.
Globally, urbanization is projected to increase at an alarming rate. According to the United Nations (Citation2018), 55% of the world’s population (7.8 Billion) currently live in urban areas, a proportion expected to reach 68% by 2050. In Sub-Saharan Africa, 43% of people live in urban settlements (United Nations, Citation2018). In The Gambia, the urban population is higher than the global and continental averages—over 60% of Gambians (GBoS, Citation2013c, Citation2013b). Increasing urbanization in The Gambia has created a series of development challenges. Urban expansion fuelled by population growth, real estate proliferation, and climate change impacts, such as sea-level rise pose existential threats to critical LULC types, including the risk of destroying vulnerable habitats and settlement areas (Amuzu et al., Citation2018a; Bijl, Citation2011; Dampha, Citation2020). For instance, The Gambia’s capital city, Banjul, is projected to sink if the global mean sea level rises by one-meter (Amuzu et al., Citation2018a; Brown et al., Citation2011; Drammeh, Citation2012; Jallow et al., Citation1996). A recent study shows that 92% of Gambians, including 46% of Banjulians, support developing a new capital city due to Banjul’s high level of exposure and vulnerability to climate change impacts (Dampha, Citation2020). According to Dampha (Citation2020) study, 52% of Gambians identified the West Coast Region (study area for this analysis), the most forested region, as their preferred site for building the country’s next capital city. What are the tradeoffs between forest loss and a new-city development?
Forests—as natural capital assets, are global carbon sinks, natural air conditioners, habitats for biodiversity, homes for millions of people, and producers of foods and other essential ecosystem services such as carbon sequestration, flood mitigation, soil retention, and water purification (Harris et al., Citation2012; Houghton & Hackler, Citation2006; Lewis et al., Citation2009; Le Quéré et al., Citation2018; United Nations, U., European Union, E., Food and Agriculture Organization, F., International Monetary Fund, I., Organization of Economic Co-operration and Development, O. & World Bank, W, Citation2012). Besides, forests regulate surface air temperature and contribute to precipitation via transpiration (Dampha et al., Citation2017; Smith et al., Citation2014). In most developing countries, particularly in Sub-Saharan Africa, forests are the leading suppliers of domestic energy needs. For instance, in The Gambia, forests provide more than 85% of the local energy demand via charcoal production as cited in First National Climate Communication, Citation2003.
Given the above introduction, this paper focuses on LULC change detected between 1985 and 2020 in the southwestern part of The Gambia. It also assesses and projects the changes in future LULC and the impacts on ecosystem services such as carbon sequestration. The research hypothesizes that (a) building a new capital city in the West Coast Region of the country will substantially accelerate the depletion of the remaining forest cover, (b) increasing urbanization has been and will continue to be the leading driver of LULC change, and (c) carbon capture and storage capacity have dropped between 1985 and 2020 and will continue to decline drastically unless aggressive forest restorations are undertaken.
The paper is divided into six sections. Section one reviews the status and trend of deforestation and carbon storage and sequestration. Section two introduces the study area. Section three presents the methodologies used (GIS-based remote sensing) and (InVEST carbon model) for this analysis. Section four shows the results of past, current, and projected LULC changes as part of the first component of the three-scenario-based analysis used in this study. The second component entails carbon stock estimates and sequestration values generated from the InVEST model vis-à-vis the three-scenarios of the study. Section five discusses the research findings with reference to earlier studies. Section six presents conclusions and study limitations.
Deforestation and global forest targets
Forests—accounting for roughly 31% of the Earth’s surface, play a significant role in climate change mitigation and ecosystem service benefits to all (Dampha et al., Citation2017; Pradhan et al., Citation2019). Deforestation is the destruction and conversion of forests into other land-use functions due to human activities and natural disturbances (FAO, Citation2020; Harris et al., Citation2012; Houghton et al., Citation2012). Others consider an ecosystem to be deforested when human activities reduce tree canopy to less than 10–30%, while forest degradation is measured as partial deforestation, with more than a 10–30% tree canopy remaining (Van Der Werf et al., Citation2009). Deforestation is often an outcome of the tragedy of the commons problem, as it deals with a public good or common-pooled resource—forests (Hardin, Citation2009; Heß et al., Citation2018). FAO (Citation2020) report shows that globally, between 2015–2020, 10 million ha are deforested annually, of which Africa records the largest annual net forest loss—at 3.9 million ha 2010–2020 (FAO, Citation2020). Unlike Asia and South America, deforestation rates are increasingly growing in Africa, where most people’s livelihood depends on forest products (FAO, Citation2020; Heß et al., Citation2018).
Curbing deforestation is one of the quickest and most significant climate change mitigation measures available globally. Parties to the United Nations Framework Convention on Climate Change (UNFCCC) at COP 13, 2007 called on developing countries to restore and reduce their emissions from deforestation and land degradation (Pradhan et al., Citation2019). Reducing Emissions from Deforestation and Forest Degradation (REDD+) is considered one of the most cost-effective options for mitigating climate change (Smith et al., Citation2014). Besides, the Sustainable Development Goal (SDGs) number fifteen encourages countries to implement the REDD+ program based on Article 5 of the Paris Agreement. Similarly, the New York Declaration on forests aims at ending forest loss by 2030 and restoring 350 million ha (WWF, Citation2015).
Deforestation and Forest Management (The Gambia)
Since 1946, human activities and climate change impacts have led to the destruction of nearly 50% of the country’s forest cover relative to 2019 estimates (Dampha, Citation2020; Sillah, Citation1999). According to First National Climate Communication (NCC) (2003), the deforestation rate stands at about 6% per annum. The significant loss of closed forestland (2,700 ha per year) is due to forest degradation and, in some regions, conversion to agricultural land (Sillah, Citation1999). Forest type categories and their share of the country’s total land area are presented in Appendix 1. Forest management in The Gambia falls under “control” and “no control” management systems (see Appendix 2 for details). According to Thomas & Sillah (Citation1999), about 7% (30,691 ha) of national forest territory is under a controlled management scheme (Sillah, Citation1999). The government continues to redress deforestation by promoting community forest management initiatives. According to , over 350 villages have implemented a community forest management scheme since the 1990s (Heß et al., Citation2018). The process transfers legal management and ownership rights to local communities (Heß et al., Citation2018). Evidence from the Forestry Department revealed that community forest management programs had yielded positive benefits on forest cover regeneration across all regions of the country (see Appendix 3 for details).
Different forest management approaches, including privatization, centralized control systems by national governments, and co-management schemes with the local communities, have been experimented in several places and implications identified (Ostrom & Cox, Citation2010). Community management or co-management of forests economically empowers local communities and improves their environmental conditions. Several other studies acknowledge that giving property or harvesting rights to local forest users does not only improve forest habitat conditions but also facilitates a more cost-effective way of managing and policing forest resources from illegal operations , as cited in Ostrom & Cox, Citation2010). Other studies concluded that excluding local users in forest management results in deforestation (Banana et al. 2007, cited Ostrom & Cox, Citation2010).
Carbon Storage and Sequestration
Atmospheric carbon content is over 750 billion metric tons. Annually, human activities such as burning fossil fuels and deforestation contribute approximately 6.6 billion tons of carbon into the atmosphere (Forest Ecology Network, Citation2020). Forests do not only emit CO2, but they also absorb it. According to Bellassen and Luyssaert (Citation2014), forests in the past few decades have “absorbed as much as 30% (2 petagrams of carbon per year; Pg C year−1) of annual global anthropogenic CO2 emissions, globally—about the same amount as the oceans.” The world’s forests and oceans sequester nearly half of the total emitted CO2, while the balance remains as surplus in the atmosphere lasting for centuries. Deforestation is one of the leading contributors to climate change (Gaston et al., Citation1998; Gorte, Citation2007; Houghton & Hackler, Citation2006). Deforestation and land-use change account for about 20% of the total carbon emissions into the atmosphere globally (Gaston et al., Citation1998; Gorte, Citation2007; Houghton & Hackler, Citation2006; Van Der Werf et al., Citation2009). Avoiding forest degradation, restoring loss forest area, and following best agricultural practices will significantly enhance the sequestration and storage of large amounts of CO2 (Tallis et al., Citation2011). Forests in sub-Saharan Africa contain 44–66 billion tons of carbon, depending on how forests are defined (Butler, Citation2011; Saatchi et al., Citation2011). In Africa, a forest stores an average of 69–117 tons per ha compared to 125–175 tons/ha in Asia and 87–132 tons/ha in tropical Americas (Butler, Citation2011; Saatchi et al., Citation2011). Butler (Citation2011) and Saatchi et al. (Citation2011) tabulated the mean biomass estimates for several sub-Saharan African countries.
Study area
Country Background
The Gambia’s population is slightly over 2 million and is projected to double by 2050 (Population Pyramid Net, Citation2019). The population’s doubling will accelerate further challenges to LULC composition, as the total territorial land size (10,120 sq. km or 1,012,000 ha) remains predominantly fixed, with some areas (e.g. the capital city) becoming more vulnerable to human habitation due to sea-level rise (Coates & Manneh, Citation2015; Hills & Manneh, Citation2014; Jallow et al., Citation1996). As a low-income developing country, poverty and unemployment rates in The Gambia are over 45 and 35%, respectively. National per capita income stands at US$778 as of 2019 (World Bank, Citation2020a). Access to quality education and primary healthcare remains inadequate across the country but relatively better in the urban areas (GBoS, Citation2013c; WB-Database, Citation2018; World Population Review, Citation2018).
The Gambia has a total productive land area of 1.5 million, defined as its biocapacity with an ecological footprint of 2 million, both measured in global hectares (gha) by the Global Footprint Network (Citation2020). The ecological footprint measures people’s demand or dependence on nature/natural capital assets and flows (Kubiszewski et al., Citation2013; Wackernagel & Beyers, Citation2019). A country is declared ecologically deficit when its footprint exceeds its biocapacity (Wackernagel et al., Citation2019, Citation1999). The Gambia became ecologically bankrupt in 2002, and as of 2016, the country has an ecological deficit of 547,341gha. On a per-capita basis, an average Gambian has an ecological or environmental footprint of 1gha relative to a per capita biocapacity of 0.7gha in 2016 (compared to 4gha in 1961). Over 75% of the population relies on agriculture and natural resources for obtaining their livelihood means. With the increasing dependence on natural capital for consumption, income generation, and wealth accumulation, an average Gambian’s ecological footprint will more than double by 2050 (higher for urban dwellers than rural settlers). Similarly, as population growth increases, the biocapacity deficit will grow exponentially. Consequently, The Gambia will continue to be not only an economically indebted developing country but also an ecological debtor (i.e. importing biocapacity) from countries with some natural capital reserves, referred to as ecological creditors.
Study Site (Southwestern Gambia)
This geospatial analysis covers three administrative regions of The Gambia, namely Banjul City Council (BCC), Kanifing Municipality (KM), and the West Coast Region (WCR). These three regions constitute the “southwestern region” of The Gambia (see study area, ). The southwestern region, also commonly known as the urban Gambia, accommodates nearly 60% of the nation’s population. The urban population has been increasingly growing due to rural-urban migration, natural population growth, and immigration from other African and European countries. Over 20% of the country’s population migrated, mainly from the rural areas, to the study region since 1983 (GBoS, Citation2013a). The migration decisions are principally influenced by prospects for urban employment opportunities and better living conditions. In some parts of the study areas, population density is nearly 5000 people per square kilometer (GBoS, Citation2013a).
Methodology
GIS-based Analysis
This study’s first component uses a GIS software (ArcGIS Pro, Version 2.5.0, 2019) with remotely sensed data to assess the Southwestern region’s land-use/land cover (LULC). The same software was used to generate the LULC projection estimates. As per our study’s objectives, the classification problem requires identifying seven LULC types (i.e. forests, shrubland/grassland, wetland/mangroves, settlement/developed, cultivated/agricultural areas, bare ground, and surface water). In , I outline a schematic model of the GIS-based portion of the data analysis. I used a pixel-based supervised classification method for image processing. Three classification algorithms, namely random forest (RF), maximum likelihood estimation (MLE), and support vector machine (SVM), were tested and compared for considering their relative accuracy. The random forest (RF) algorithmFootnote1 produces a relatively better result than MLE and SVM algorithms.
Besides the LULC classification method, this study uses the normalized difference vegetation index (NDVI) to evaluate forested areas’ habitat conditions and support to validate the classification accuracy (see above). The NDVI is widely used to monitor land-use or vegetation cover dynamics from local, regional, to global scales and has proven to be a useful change detection tool (Hassan et al., Citation2018). The NDVI is calculated as:
Where NIR is near-infrared light and Red is the visible red light of the satellite’s electromagnetic spectrum. According to the US National Aeronautics and Space Administration (NASA), negative and very low NDVI values (0.1 and below) represent bare ground places with rock, sand, or snow. Moderate values (0.2 to 0.3) correspond to shrub and grassland areas, and high values (0.6 to 0.8) indicate temperate and tropical rainforest covers (Weier & Herring, Citation2020).
The primary data used in the GIS-based component was remotely sensed imagery, derived from the Landsat satellites, operated by the US Geological Survey (USGS). I present the details of the various processed Landsat images in . The main criterium for image selection from the USGS database (Earth Explorer) was that the individual satellite image paths must not have more than 16 days temporal difference (i.e. within Landsat’s temporal resolution), either within the same year or between two consecutive years. In remote sensing, the narrower the temporal differences, the lesser the spectral variations, and the better the analysis’s overall accuracy. Second, atmospheric effects, such as scattering and cloud cover, reduce my chances of selecting close-dated images. Thus, I eventually used an image from the previous year, falling within the temporal selection criterium. For example, during preprocessing, I mosaiced satellite paths 205 and 204 images, respectively, captured in 1985 and 1986, to cover the study site’s areal extent. In all cases, path 205 image covers 90% of the study area (see areal image extent in ).
Table 1. Satellite data sources for GIS-based analysis
I also used data from the European Space Agency’s Copernicus satellite, Sentinel-2 A/B, for evaluating the classification accuracy of Landsat images. I used the classified map, derived from Sentinel-2, as the reference map. This is because Sentinel 2 data has a 10-meter spatial resolution,Footnote2 which is more likely to reflect the “ground-truth” of the study site than Landsat data, with a 30 meter resolution. The accuracy assessment tool in ArcGIS Pro was used to conduct an accuracy comparison. The analysis created 301 accuracy points, distributed across the study area, using an equalized stratified random sampling strategy (). This analysis is an “accuracy comparison,” not an accuracy assessment. In performing an accuracy assessment, the reference map should have been predetermined and independent of the producer’s analysis. I could not do an accuracy assessment in this study because no previously classified reference map is traceable and reliable for that purpose. Notwithstanding, using Sentinel-2 imagery for accuracy comparison is better than none and useful enough for fulfilling the study’s objective.
InVEST-Based Analysis
This study’s second methodological component uses a spatially explicit modeling tool, the Integrated Valuation of Ecosystem Services and Tradeoff (InVEST) model, developed by the Natural Capital Project (NatCap, Citation2020; Tallis et al., Citation2011). The InVEST carbon model uses my LULC maps together with estimates of four carbon pools, namely, belowground biomass, aboveground biomass, soil carbon, and dead organic matter, to compute the total carbon storage and carbon sequestered between two periods in the study area (NatCap, Citation2020; Tallis et al., Citation2011). Carbon sequestration and storage in the terrestrial ecosystem are possibly the most widely recognized ecosystem services (IPCC, Citation2014; Tallis et al., Citation2011). The model uses the social cost of carbon and its annual rate of change and a discount rate to compute the economic value of carbon dioxide (C02) to societyFootnote3 (Tallis et al., Citation2011).
The required data used, including their sources and descriptions for running the InVEST Carbon model, are presented in below. The carbon pool values were informed by Saatchi et al. (Citation2011) and FAO’s (2015) estimates of carbon content in the country’s living forest biomass. The sample CSV file of the InVEST Carbon model informs the distribution of the carbon pool values in . The model has few limitations (discussed below), but so far, it is one of the most commonly used opensource models for ecosystem services valuation studies globally (Nelson et al., Citation2009; Yang et al., Citation2018); hence, the primary reason for using it in this study.
Table 2. Data source and description for the running the InVEST carbon model
Table 3. Carbon pools estimates used
The value of the sequestrated carbon over time for a given parcel x isFootnote4:
Where is the difference in carbon stored between the future and the current landscapes,
, respectively, refers to future and current LULC maps, r is the market discount rate reflecting society’s preference for immediate benefits over future benefits, c is the annual rate of change in the price of carbon, adjusting the value of carbon sequestered in relation to the expected impacts of climate change over time due to CO2 emissions. In this study, c was set at −1%, meaning I treated the future societal value of sequestrated carbon to be greater than the amount of carbon sequestrated now. Assuming that future climate change damages to society will be higher as greenhouse gas emissions continue to increase over time (NatCap, Citation2020; Tallis et al., Citation2011).
Table 4. LULC class description
Table 5. Comparison of forest cover in 1983 and 2019
Table 6. Percent change and absolute difference of LULC 1985, 2003, and 2020
Table 7. Confusion matrix for the recent LULC classification
Table 8. Open forest parks -my proposed protected areas (PAs) in the southwestern region
Table 9. Forest parks- area (ha) & conditions in the southwestern gambia (dept. of forestry est. vs. est. of this study)
Table 10. Percent of total area by LULC (trends with BAU, NCC, and SUST. Protections)
It’s important to note that the debate on the social cost of carbon and the discount rate(s) for long-term climate change interventions remains unsettled. For a detailed discussion on this controversial topic, refer to the Stern Review (2006) and several counterarguments from other prominent scholars (e.g. Dasgupta, Citation2007; Davidson, Citation2015; Nordhaus, Citation2007, Citation2007; Tol, Citation2006; Weitzman, Citation2007; Yohe, Citation2006). Considering the divergent views on the price and the discount rate(s) for climate change-related cost-benefit analysis, I used two different estimated values provided by two prominent economists, Nicholas N. Stern (Citation2006) and William Nordhaus (Citation2007) (see below). I present both net present values (NPVs) in the result section for policymakers to determine which economic value estimate to select. The Stern and Nordhaus values are, respectively, based on prescriptive (i.e. normative economics) and descriptive (i.e. positive economics) approaches to discounting (Arrow et al., Citation1996; Davidson, Citation2014, Citation2015). Whether one uses either the positive economics view or the normative economics view, the total economic value of marginal carbon damage to society can vary significantly. On the one hand, advocates for the normative economics approach prefer a lower discount rate and a high social cost or price of carbon per ton (see, e.g. Chichilnisky et al., Citation2020; Davidson, Citation2014; Cline, Citation1992; Garnaut, Citation2008; Rendall, Citation2019; Sen, Citation1982; N. N. Stern, Citation2006; Nicholas Nicholas Stern, Citation2008). On the other hand, the positive economics scholars favor the use of a higher discount rate and a lower social cost of carbon (see, e.g. Nordhaus, Citation2008; Nordhaus & Boyer, Citation2000; Tol, Citation2005).
Table 11. Total carbon stock and its economic value (study area)
Land-use land cover (LULC) change results
National
First, I present the national estimates for the current land-use/land cover (LULC) composition and change detected relative to estimates reported in The Gambia’s Second National Climate Communication Report (2012). Using recent satellite imagery (captured in December 2019 by Landsat 8), this study finds a landmass area of 42.5% as national forests, including planted, shrub/grasslands, and highly dense forests cover types (see ). I present in the working definition of the land cover classes used in this study. Of the country’s total closed and open forest covers, 68% are found in the West Coast Region (WCR), excluding grassland and mangrove forest areas (see ). I find 58,532 ha of degraded forests, including areal losses of nearly 26,312 ha of open and closed forestlands combined and 32,219 ha of shrub/grasslands. Wetlands (including some water bodies) cover roughly 104,485 ha of the total national land area, corresponding to 9.5% of the land area. Overall, my analysis reveals a national forest cover area of 42.5% of the total landmass area.
The Department of Forestry in The Gambia has recently published data on the area of forest parks nationally in a recent Environment Statistics Compendium Report (GBoS, Citation2020). After digitizing the locations of forested areas and assessing their habitat conditions, using elements of image interpretation based on canopy cover density (see ), I also find most open forest parks are located in the southwestern region. These parks are unnamed and unlisted by the Department in the Report (GBoS, Citation2020). In relatively “good” habitat conditions, these forest parks are presumed to be either state-owned or community forestlands.
Southwestern Gambia
After inspecting the national picture, this analysis focuses on the LULC change recorded in The Gambia’s southwestern region. The findings detect a significant difference in LULC between 1985 and 2020 in the region (see ). In 1985, 68% of the study region comprised “green spaces” or “forests,” including shrub/grassland, planted trees, forest areas (open and closed). Forest areas declined by 5% in 2003 and 18% in 2020, relative to the 1985 land cover composition (see ). In contrast, the developed/urban areas increased by 15% from 1985 to 2003 and 65% from 1985 to 2020. In a nutshell, the study area has lost 22,408 ha of green spaces, including shrub/grassland, planted gardens, and highly forested sites, since 1985. In this study, mangrove areas are excluded from my definition of green spaces or forests. Similarly, the normalized difference vegetation index (NDVI) shows the vegetation cover changes across the region since 1985, and the result corresponds with the LULC classification maps (see above).
Accuracy Comparison of LULC Maps
The study includes an accuracy comparison of classified images captured in February 2020 by two different satellites. As mentioned above, the reference map, using Sentinel 2 images (relatively more accurate), and the classified map, using Landsat 8 images, are compared in . The assessment yields an overall map accuracy of 73% (see the confusion matrix in below). The overall map accuracy indicates the proportion of sites in the classified map correctly mapped with reference to the presumably “ground-truth” in this case, the reference map. The Kappa Coefficient stands at 0.69. The Kappa Coefficient has values ranging from 0 and 1. The higher the number, the better the agreement between the classified map and the reference map.
The Producer’s Accuracy shows the map accuracy from the map maker’s perspective, while the User’s Accuracy presents the map’s reliability from the end user’s point of view. The Producer’s Accuracy is the probability that a real feature on the ground (reference map) is correctly shown on the classified map. The User’s Accuracy indicates the likelihood that a class on the classified map is actually present on the ground (reference map). The Producer’s Accuracy is a complement of omission error, while the User’s Accuracy is a complement of commission error. You can compute the errors of omission and commission by, respectively, subtracting the Producer’s Accuracy and User’s Accuracy percent values from 100%. Type I and Type II errors also correspond to omission and commission errors (Humboldt University Geospatial Online Class, Citation2014).
From the confusion matrix below, one can read that “bare soil/sand” and “planted/cultivated” classes have the least reliability from the map user’s viewpoint. In contrast, the “developed/settlements” and “brush/grassland” have the lowest accuracy from the map maker’s perspective. The “developed/settlements” class is most confused with the “bare soil/sand” class. For example, 10 points on pixels that actually represent a “developed/settlements” area on the ground were incorrectly classified as “bare soil/sandy.” This is not a significant concern as developed/settlement sites are inextricably associated with bare soil or sand areas. For the purpose of this analysis, I can assume that these classes are reliable indicators of urban settlements. Besides, the “brush/grassland” class is mostly confused with “planted/cultivated” and “forest.” The forest class (closed and open) has the highest Producer’s Accuracy (91%) among all land cover types. Therefore, I am confident about this map’s reliability in distinguishing urban settlements from areas with green spaces.
Comparison of Estimates with State Listed Forest Parks
Based on the Forestry Department’s categorization of forest types, I find two forest categories in healthy habitat conditions in the Southwestern region. They are classified as closed (officially reported) and open (unofficial) forest parks. Of the study area’s total land area (182,338 ha), closed forest parks account for 2.37% (4352 ha), while unofficial or open forestland represents 3.38% (6162 ha). However, given the positioning of open forested areas in the urban landscape associated with the current property regime type (a common-pool resource), the locations are exposed to moderate and high risk of human interferences (see ). shows the spatial positions of both open and closed forest parks in the study site, as tabulated in .
Similarly, I contrast my assessment of the study area’s estimates of forest parks with the Department’s official records of closed forest parks in the region (see below). I find no evidence of major land-use conversion of the closed forest parks. However, I have discovered a decline in forest size for all the parks, except—Bijilo and Nyambai-Bamba forest parks. I can confirm that the difference between this study and the Department’s estimates of Nyambai-Bamba forest parks’ areal extent is likely a recording error from the latter (i.e. Forestry Department). Though the forest parks’ measurements look similar, forest cover degradation is highly noticeable, especially in Bama Kuno and Finto Manareg, both located in Kombo East district (see below).
Land-use land cover projection (2020-2050) results
The projected LULC change (2020–2050) analyzed in this study are based on three different development pathways, namely, a business-as-usual (BAU) scenario, a new capital city (NCC) scenario, and a sustainability (SUST) scenario. The BAU scenario extrapolates future LULC change based on the past trends observed since 1985 and reasonably accounts for projected population growth and economic prosperity. The NCC scenario predicts LULC changes expected if The Gambia decides to locate its next capital city in the southwestern Gambia, as discussed in Dampha (Citation2020). The SUST scenario includes policy interventions, which can drastically minimize forest cover loss and enhance carbon sequestration capacity in the study area if formulated, implemented, and enforced.
First, given the LULC trends and considering current policy interventions discussed below, the Business-as-Usual (BAU) scenario predicts an 80% decline (79,556 ha loss of forest cover) by 2050 relative to the 2020 level if business-as-usual continues. As a proportion of the total land area, forests/green spaces will likely decrease from 55% in 2020 to 11% in 2050. By mid-century, urban areas will grow from 34% in 2020 to an estimated 74% of the study area’s total land area (see ).
Second, considering the assumptions discussed below, the New Capital City (NCC) Scenario estimates a 91% decline (90,496 ha loss of forest cover) by 2050 relative to the 2020 level, primarily reinforced by additional land-use conversion and forestland appropriation for public and state uses. Approximately 5% of the entire study region will remain forests–only guaranteed if the current closed and open forest parks in the region are protected. The existing urban space will likely increase from 34% to 80% as a share of the total land area by 2050 under the NCC scenario. Wetland areas will increase because of sea-level rise impacts. However, the mangrove ecosystem will be under emerging threats (see ).
Unlike the BAU and the NCC scenarios, the sustainability (SUST) scenario is about what “should be done” differently (policy interventions) to change the status quo. It is less about what could happen if current trends continue (BAU) and more about what may likely occur if a new capital city (NCC) is built. The SUST scenario’s goal is to initiate protection, conservation, and restoration strategies aimed at maintaining 25% of the total land area as forests in the southwestern region of The Gambia and limit urban expansion to only 60% of the total land area (see ). I discussed below the key assumptions for attaining this target by 2050.
Carbon storage and sequestration results
The majority of the terrestrial-based carbon in The Gambia is stored in the country’s southwestern region (see Map 7). In 2020, the total carbon stock in the region was approximately 66,449 megagrams or metric tons compared to 88,262 in 2003 (see ). Due to deforestation and land-use conversion, the area lost 21,824 tons of carbon from 2003 to 2020, equivalent to a loss economic value, ranging from US$521,526 (min) to nearly 7 USDmillion (max) (see ). The minimum value uses a 32 USD/ton carbon price and a discount rate of 5%, as proposed by Professor William Nordhaus. The maximum amount uses Professor Nicholas Stern’s recommended social cost of carbon, 326 USD/ton, with a discount rate of 1.4%. The difference in social cost or marginal damage cost to society is explained by the philosophical and ethical variances on carbon price used and discounting approaches adopted (normative vs. positive economics) (see methods section above). The carbon sequestration values for all three scenarios are presented in and further discussed below. I also compared carbon stocks from 1985, 2003 to 2020, with the 2050 projections of carbon expected to be sequestered in the study site under the BAU and the SUST scenarios ().
Discussion
Land-use Land Cover Change
Given the above analysis, the national forest cover area in The Gambia is approximated at 42.5% instead of 44–48%, as previously determined by FAO and earlier studies (Second National Climate Communication, Citation2012). Similar estimates include 41.7% (Mongabay, Citation2006) and, more recently, 40% and 38%, according to Heß et al. (Citation2018) and Saatchi et al. (Citation2011), respectively. The results of this analysis find a total national forest cover loss of 58,532 ha, relative to the 1983 estimates reported in The Gambia’s Second National Climate Communication Report (2012). According to FAO (2011), forests and other woodlands declined by 19% in The Gambia between 1998–2010 (Heß et al., Citation2018). Since 2014, Umaru Fofana reported that the country has logged and exported nearly 600,000 tons of timber—approximately 1 million rosewood trees (a local tree species) to China, valuing at ~US$300 million (BBC, Citation2020). However, some of the exported wood might have been smuggled from the neighboring country, Senegal (BBC, Citation2020).
For the southwestern region, there is no traceable record of a study on forest cover and land-use change using satellite imagery and GIS tools. This paper’s findings will inform forest and natural resource management and policy decision-making process in The Gambia. It’s relevant because a significant portion of the region’s closed and open forest cover is in “good” but “risky” habitat conditions. The open forests are mainly under community and private management schemes (Sillah, Citation1999). However, the area is highly susceptible to human-induced activities causing irreparable environmental damage and non-substitutable loss of critical natural capital (Dampha, Citation2020). According to earlier work, by 1983, 35,550 ha of forest parks and potential community forest areas were degraded in the study region (Sillah, Citation1999).
The primary drivers of deforestation, forest degradation, and land-use change in The Gambia include climate change impacts (e.g. draughts leading to bushfires), urban expansion (caused by migration and population growth), commercial timber production (mostly through illegal logging), local wood extraction (for domestic energy consumption), and infrastructural development (i.e. building roads, markets), and the proliferation of real estate developments fueled by high remittance inflows from Gambians in the diaspora. Similar causes of deforestation are reported in other developing countries, including in China, Guatemala, Honduras, and Nicaragua (Dampha et al., Citation2017; Heß et al., Citation2018; Houghton et al., Citation2012). The main barriers to mitigating deforestation and land-use change include bribery and corruption, limited institutional capacity to conduct proper monitoring, poor leadership, lack of adequate enforcement of environmental and forestry laws, ineffective co-management strategies, insufficient funding, and lack of clear land ownership rights (NAPA Gambia, Citation2007; Gambia’s 2nd National Communication, 2012; Dampha et al., Citation2017; Heß et al., Citation2018).
As the most commonly applied forest management regime in The Gambia, community forest management also presents several grand challenges. There has been gross mismanagement and various unsustainable practices in community forest management schemes (Heß et al., Citation2018). For example, illegal logging has been rampant and difficult to prevent in many community forest parks. Also, shared forest areas between communities have generated conflict over natural resources (Heß et al., Citation2018). Consequently, the tragedy of the commons problem still rears its ugly face due to the lack of clearly defined property rights over land, including forestland. This has created disputes, violence, and conflict over land-use and forest resource exploitation. Also, the government’s claim of legal ownership of all regional land through the State Lands Act (1991) has triggered tension over landownership rights. In many cases, community members often reclaim traditional landownership rights, which they considered rightful entitlements inherited from their ancestors, who were the first settlers in these areas. This tension has significantly contributed to deforestation and negligence by forest stakeholders in protecting and conserving the forest ecosystem’s biological diversity (Dampha, Citation2020).
Business-as-Usual (BAU) Scenario
Since 1985, this analysis observed a significant reduction of forest area in the southwestern part of The Gambia. Forest cover loss tripled between 2003 and 2020 compared to 1985 and 2003 estimates. The national deforestation rate of 6%, according to the First National Climate Communication (Citation2003), primarily informs the BAU scenario. Given the abovementioned results, the BAU scenario makes the following critical assumptions about land-use change and land cover conversion in the study region.
First, the BAU scenario accounts for forest cover degradation for direct and indirect local livelihood and sustenance uses. The overwhelming majority of Gambians depend on agriculture and forest resources for employment and obtaining their daily survival means. For instance, in 2014, 60,000 tons of charcoal were produced to meet domestic energy demand (Urquhart, Citation2016). Equally, products from forest trees are used for traditional medical treatments (Sillah, Citation1999), and such products will continue to be extracted and sold in the markets. As the national population continues to grow at an annual rate of about 2.9 to 3%—a trend recorded since 1970 (World Bank, Citation2020b), the demand for forestland, forest resources, and forest ecosystem service products will accelerate over time.
Second, the BAU scenario considers land-use conversion from forest to developed areas for urban expansion, industrial and infrastructural development based on observed trends in the study region. There have been lots of land grabbing, including forested areas, from the local communities by the government and through public-private partnership agreements. Another aspect of the land-use conversion of forestland is for urban agricultural production. As current farmlands transformed into real estate areas, local communities will continue to cut down the remaining forested sites to cultivate crops such as groundnut and other cereal products to meet the growing food demand. Commercial or mechanized farming is predicted to rise with an increase in per capita income and private sector investment in agricultural development. Overall, change in forest cover/green spaces will be driven by the rising demand for urban land-use conversion, as the country’s population, its per capita income, and ecological footprint are predicted to increase by two-fold by 2050.
The BAU scenario also factors in current management strategies and national policy objectives. It assumes that all official closed forest parks (4,321 ha) will be managed and maintained as prescribed by the National Forest Policy objectives (Forestry Sub-Sector Policy Forestry Sub-Sector Policy Gambia, Citation2010). The BAU scenario recognizes the current roles of state and non-state actors, including the local communities. It assumes that all current forest stakeholders will participate in the co-management scheme of national forestlands. For example, The Gambia government, in partnership with the United Nations Environment Program (UNEP), secured funding from the Green Climate Fund (GCF) to implement a six-year (2017–2023) ecosystem-based adaptation (EBA) project. One of the project components is to restore and regenerate 10,400 ha of degraded forest, savanna, and mangroves, representing 1% of the national territory as forests, and develop 166 natural resource-based businesses for strengthening the adaptive capacity of local communities (Nyangado & Pouakouyou, Citation2017). Though, the region of focus for this analysis is not benefiting from the EBA forest regeneration efforts. However, ongoing collaborative efforts like the EBA project under the BAU is forecasted to protect 3% (5,470 ha) of open forest areas, 3% of grassland, maintain 2% (3,646 ha) of the planted area, sustain 2% (3,646 ha) of closed forest parks, and restore 1% (1,823 ha) of green spaces through the integration of urban green infrastructure initiatives such as street trees in urban planning. If continued, current stakeholders’ interventions will sustain 11% of the study area’s landmass as forest by 2050. This includes the existing non-state actors’ involvement in forest regeneration and protection, mainly by international conservation organizations and local environmental groups. Such organizations include UNEP, Green-Up Gambia, Household Disaster Resilience Project (HELP-Gambia), and Community Action Platform for Environment and Development (CAPED).
New Capital City (NCC) Scenario
Studies have recommended that The Gambia government build a new capital city (NCC), as the current one is projected to be underwater by 2100 due to the rising trend of mean sea-level (Coates & Manneh, Citation2015; Hills & Manneh, Citation2014; Jallow et al., Citation1996). Taking such a recommendation as a long-term climate change adaptation plan, we learned in Dampha (Citation2020) that over half of Gambians preferred the next capital city to be situated in the West Coast Region (i.e. within the southwestern region). Suppose that the government declares a new site in this study area by 2030; this scenario makes three principal assumptions regarding the expected changes in LULC.
First, it assumes that the government will grab and convert colossal forestlands into developed areas. The landmass estimated to build a new capital city covers 8,945 ha (Dampha, Citation2020). These land plots, including forested sites, will be ceded by the government while basing its actions on the provisions of the current State Lands Act (1991), which permits state appropriation of regional lands for public use (e.g. for constructing buildings and other infrastructural development projects). Recent anecdotal evidence supports this assertion. For example, in 2018, the Barrow Administration decimated over 16 ha of the Bijilo Monkey forest park to build an international conference center, funded by the Chinese government (see ).
Second, developing a new capital city in the study region is assumed to increase land-use demand for human settlements as population size grows and the land area remains constant as a fixed asset. An increase in such demand will trigger a spike in property values, thus motivating landowners to sell the remaining forest areas under their custody to estate developers. This assumption is predicted based on the law of supply and demand and also informed by our understanding of the gravity model of migration. The gravity model predicts spatial interaction between destinations on the pretext of population size and distance between destinations. The model illustrates that movement is directly related to the populations of places and inversely related to the distance between them. The southwestern region is a population center with a population density of over 3,000 people per square kilometer. By 2050, with the doubling of the national population, over 6,000 people will occupy a square kilometer of land in the study area. Besides, constructing a new city in the region will ultimately attract more Gambians and non-Gambians to invest in infrastructural projects and eventually settle in the region.
Finally, I assumed that most of the ongoing restoration and conservation programs, as specified under the BAU scenario, will be significantly undermined and undervalued under the NCC scenario. The battling questions are: Will current residents restore and protect forestland for intergenerational benefits when their survival needs are unmet? In other words, should we protect the environment and its resources for generations expected to be wealthier (i.e. future generations) while the present generation suffers in abject poverty? Policymakers and environmentalists will have to debate and answer these and many such ethical questions. As evident in most developing countries, people in The Gambia will likely prioritize creating spaces to live and cultivate food to survive than to maintain forests for other ecosystem service benefits such as carbon sequestration and air filtration. Theories such as the hierarchy of needs by Abraham Maslow in his 1943 paper “A Theory of Human Motivation” supported this claim. According to Maslow, people satisfy their immediate physiological and safety needs (e.g. food and shelter) before worrying about other elements of self-actualization and sustainability issues (Poston, Citation2009). As Udo and Jansson (Citation2009) stated, “as in Maslow’s hierarchy of needs, nations that are struggling to survive are less concerned with environmental sustainability than advanced and stable nations” (p 3700).
Sustainability (SUST) Scenario
Because of the LULC change trends observed under the BAU and likely possible under the NCC scenarios, the SUST scenario’s goal is to avoid forest loss below the 25% threshold of the study region’s total landmass by 2050. Whether protecting 25% of forest cover is considered an ecologically sustainable path is subjective, as what constitutes sustainability are broad sets of capitals–financial, produced, human, natural, and social, of which some are hard to define and measure (Kumar & Smith, Citation2019; Managi & Kumar, Citation2018; Polasky et al., Citation2015). For the purpose of this analysis, the 25% target is the proposed sustainability goal. Hence, I defined ecological sustainability as the country’s ability to properly manage, protect, and sustain 25% of the southwestern region’s total land area for intra-and intergenerational ecosystem service benefits.
The Gambia’s national forest policies of 1995 and 2019 both proclaim that a national “forest cover of 30% is sufficient for maintaining an ecological balance necessary for sustainable economic growth” (Forestry Policy Gambia, Citation2010 p. 2). The forest policy also seeks to ensure that 75% of forestlands are managed, protected, and promoted for sustainable forest products flow to needy urban and rural populations (Forestry Policy Gambia, Citation2010). Since 1983, the forest policy aims at transferring nearly 200,000 ha of state forestland to community forest management schemes by the year 2005 (Sillah, Citation1999). These include 32,729 ha of national forest parks, 4,321 ha of forest parks in the southwestern region. However, according to my findings, not only has this specified policy target failed, but more deforestation and environmental degradation have occurred, especially since the year 2000. In terms of management structure in the study region, only 13% of the total forested areas are controlled and managed by the authorities. The majority of forestland (86%) is alleged to be managed and controlled by the local communities (Sillah, Citation1999).
To attain the sustainability goal, I proposed four broad policy areas focusing on the formulation, implementation, and enforcement of numerous forest preservation, protection, and restoration strategies and programs. Some of the sustainability strategies presented below are in agreement with the policy objectives specified in the new National Development Plan (NDP- Government of The Gambia, Citation2017) (2018–2022), National Climate Change Policy (Urquhart, Citation2016), Parks and Wildlife Policy (Parks and Wildlife Policy Gambia, Citation2013), Forest Sub-sector Policy (Forestry Sub-Sector Policy Forestry Sub-Sector Policy Gambia, Citation2010), and the National Adaptation Programs of Action (NAPA Gambia, Citation2007). For example, The Gambia’s National Adaptation Programs of Action (NAPA) identified three priority areas for forest stakeholders’ intervention. These include sustainable forest resource utilization for commercial and non-commercial purposes, community awareness-raising, and ecosystem health and biodiversity restorations (NAPA Gambia, Citation2007). As mandated by their various legislative Acts, the Departments of Parks and Wildlife Management, Forestry, Lands, and Agriculture will be principally responsible for the consideration, implementation, and sustainability of the proposed policy directions and management strategies identified under this scenario. I assumed that local communities would be empowered with a shared responsibility to manage and restore community forest parks for solidifying the co-management scheme of forest resources.
The first sustainability strategy focuses on national protected areas (PAs). The United Nations Convention on Biological Diversity calls for an international agreement on establishing PAs based on national laws. The Convention recommends that 10% of national territory be protected for biodiversity conservation (Dampha et al., Citation2017). In The Gambia, protected areas account for nearly 3.3% of the entire national territory (see Appendix 4). The national Parks policy seeks to increase the proportion to 5% (Sillah, Citation1999), which is still 50% less than the globally recommended target. In the study region, only two official forest parks have been gazetted as PAs (Abuko nature and Tanji Bird reserves). For contributing to the attainment of the national PAs target, the SUST scenario considers the formulation of legislation to gazette all my proposed list of open forest parks (6,161 ha) as PAs (see above). This strategic policy action is only applicable and enforceable with community involvement under the current laws of The Gambia. The State Lands Act (1991) makes provisions for the Minister of Lands to designate any “regional land” to be “state land” for the benefit of the community in which the land is located (Bensouda & Co Lp, Citation2013).
However, before enforcing the provision, the Lands Act should be revised, where necessary, amended with local communities’ involvement, especially with support from the traditional landowners. The proposed PAs account for nearly 60% of closed and open forest covers and 3.38% of total land area in the study region. All PAs’ conservation strategies should consider co-management, and polycentric governance approaches as fundamental pillars of sustainability. Polycentric governance structure involves a complex and systematic arrangement of roles and responsibilities between multiple governance levels (Ostrom & Cox, Citation2010). For a detailed discussion on the concepts of co-management and polycentricity in forest resource management, read Ostrom and Cox (Citation2010). Worthwhile to emphasize that the policy objective’s success depends on the level of community-based participation in forest conservation and restoration efforts. The participating communities should yield some benefits from state declared PAs through monitored and sustainable based mechanisms. When stated otherwise in current laws, the so-called PAs’ definition should be reviewed and revised to allow local participation in the co-management of natural resources. This scenario assumes that local community members would continue to be receiving ecosystem service benefits from several forest resources in the PAs, including dry wood for charcoal, wild fruits, and honey productions, amongst others. Also, a sustainable timber harvesting scheme should be incorporated into the co-management plan. Given the tourism sector’s contribution to national GDP, this sustainability approach can further boost the ecotourism sub-industry and attract more arrivals from the growing global ecotourism community. Other ecosystem service benefits of “protected” forests include recreational opportunities for birdwatching, flood mitigation, water quality improvements, and, more importantly, supporting habitats for wildlife conservation. Overall, properly managed forested ecosystems are biodiversity enriched, including species and genetic diversities. According to the First National Climate Communication (Citation2003) report, The Gambia has an estimated 140 trees and shrub species from 12 families.Footnote5 Without adequate protection, the majority of national flora and fauna will continue to be labeled as threatened and endangered species because of their vulnerability to climate change impacts and habitat destructions caused by human activities.
The second sustainability strategy calls for maintaining forestland through the implementation of market-based conservation and restoration programs. It assumes that the government will strengthen collaboration with international partners to introduce market-based payment instruments for promoting forest resource management. As earlier mentioned, this is critically essential as most local communities’ source their livelihoods from forest resources. Payment for ecosystem service programs such as REDD+ and conservation easement projects can be used to incentivize local forest owners from selling their forestland to developers. There are massive community forest areas (i.e. shrubland) expected to be used by local landowners to participate in these schemes. With the availability of funding, 10% (~18,233 ha) of the total landmass can be maintained through market-based conservation and restoration programs. These funding mechanisms will offer economic, conservation, and ecosystem service benefits to local actors and their local partners. Globally, these initiatives will promote carbon sequestration for climate change mitigation benefits. Therefore, the local actors should be sensitized to restore the lost forest areas and afforest new sites while expecting some financial gains.
The third sustainability strategy relates to reserving land for urban agricultural development. This sustainability strategy was recently solicited by a significant proportion of Gambians surveyed by Dampha (Citation2020). Food security threats are plausible in the study region due to growing population demand and land-use change from agricultural land to urban settlements, as discussed above. Through the Department of Lands in partnership with the Ministry of Agriculture, the government and its stakeholders should reserve at least 7–10% (~13,000–18,000 ha) of the study area’s total landmass for agricultural uses only. Through such legislation, landowners will be more innovative in developing irrigation systems and horticultural gardens to plant fruit trees (e.g. orchards) and yield farm produce to enhance the national food basket. The development partners, including the private sector and international development organizations, are presumed to support the country’s highly unemployed youth population with resources to advance their agribusiness industries. This strategy will facilitate self-reliance, ensure food sovereignty, generate employment opportunities, reduce urban poverty level, lessen the income gap between the rich and the poor, and minimize the overall national dependence on unsustainable foreign aid or what Dambisa Moyo calls “death aid” from the global north (Moyo, Citation2009).
The final sustainability strategy requires incorporating green infrastructure projects in already developed urban and new estate development areas. The strategy suggests that government, through the three local municipalities in the study area, create a comprehensive green infrastructure plan to integrate nature-based solutions to mitigate urban flood risk and strengthen urban climate resilience. The development of impervious surface areas in urban areas increases the risk of inundations from frequent and extreme precipitation events (Dampha, Citation2020). The southwestern region is already disaster-prone and highly vulnerable to many climate hazards (Amuzu et al., Citation2018a; Bijl, Citation2011; Dampha, Citation2020; A. Gomez, Citation2015; M. L. A. Gomez et al., Citation2020). Urban flood risk is exacerbated by the lack of proper stormwater infrastructure systems. Green infrastructure projects in urban areas serve as nature-based stormwater management systems. They include street trees, green roofs, urban parks, and rain gardens. The benefits of urban green infrastructure initiatives include dipping flood risk, minimizing urban heat island effect, reducing air pollution impacts, improving health and mental wellbeing, strengthening biodiversity, and promoting livability in the urban landscape (Chenoweth et al., Citation2018). Urban green infrastructure initiatives expected to be pioneered by the local municipalities, private actors, and individual households under the SUST scenario should account for 1–3% (1,823 − 6,000 ha) of the study region’s total land area.
In general, the grand challenges facing the SUST scenario also include (1) lack of data on land ownership rights and ecosystem values, (2) institutional weaknesses and leadership challenges, (3) the potential development of a new capital city in the study region, (4) the doubling of the Gambia’s population by 2050, (5) the increasing intensification of real estate development and industrial expansion leading to reduced biocapacity, and (6) the accelerating economic prosperity of society accelerating the country’s ecological footprint.
Carbon Storage, Sequestration, and Valuation
In The Gambia, forests contain 359,000 metric tons of carbon in living forest biomass (FAO, 2015). Saatchi et al. (Citation2011) study estimated a national forest carbon stock of 400,000 tons, using a 25% forest cover definition. This study finds an estimated total national forest carbon stock of 421,344 megagram or metric tons, with an average per hectare carbon stock of approximately 1.7 metric tons. Carbon stock content is highest in my study region. Evidently, land-use change and land cover (LULC) conversion contribute to terrestrial-based carbon emissions, mainly from the southwestern Gambia. Before the year 2000, the country’s land-use change and forestry sub-sector served as a sink of C02 instead of a net emitter (Urquhart, Citation2016). According to the Gambia Climate Change Policy (2016), besides the energy sector, land-use change and forestry account for the most substantial discharge of C02 (Urquhart, Citation2016). In 2000, land-use change and forestry sub-sector emitted 110 Gg CO2, representing 34% of total emissions (Urquhart, Citation2016).
The above evidence supports this study’s findings that the forestry and land-use sub-sector is currently a net emitter of C02 and has emitted nearly 21,824 tons of carbon between 2003 and 2020 in the southwestern region. The change in carbon stock is associated with an economic value loss ranging from US$521,526 to almost US$7 million in the same area (see above). Under the BAU and NCC scenarios, forest degradation and land-use change will respectively, contribute to the emissions of 24,499 and 45,474 metric tons of carbon by 2050 relative to the 2020 level, corresponding to an average economic cost of US$7.6 million and approximately 11 USDmillion (using Stern’s valuation parameters) (see ). This excludes the cost of biodiversity loss, particularly under the NCC scenario. Suppose that the government and other stakeholders can successfully attain the SUST Scenario goal by implementing the policy actions identified above; the study region will only produce net emissions of only 3,371 tons of carbon by 2050 relative to the 2020 level. The economic loss to society ranges from 65,027 USD to slightly over 1 USDmillion. Based on Stern’s valuation parameters, my SUST scenario would likely save society a monetary value of nearly US$10 million between 2020 and 2050 (see ). Under the SUST scenario, the region can absorb 21,128 tons and 42,103 tons more carbon from the atmosphere than the BAU and NCC scenarios, respectively. Besides, biodiversity and ecosystem service value losses will be grossly minimized if the SUST scenario is considered.
Conclusion
The rate of deforestation, forest degradation, and land-use change will continue in the southwestern region of The Gambia (study area) but vary based on the government and its stakeholders’ pursued development scenario. This analysis reveals a forest cover loss of 22,408 ha from 1985 to 2020 in the study region. The ecosystem damage between 2003 and 2020 has contributed to the emissions of 21,824 metric tons of carbon, corresponding to an economic value loss of approximately US$7million, using Nicholas Stern’s valuation approach. This valuation amount excludes the loss value of ecosystem diversity, species diversity, and genetic diversity of the region. The economic loss to society is highest under the NCC scenario, followed by the BAU and SUST scenarios. Forest ecosystem extermination and extinction of threatened, endangered, and critically endangered species are also most likely first under the NCC and the BAU, relative to the SUST scenario.
With the development of the proposed new capital city in the study region (NCC scenario), the feasibility of reaching the sustainability goal (25%, under SUST scenario) will be grossly limited, difficult, if not impossible, to accomplish, especially in the absence of an integrated environmental management plan. Whether a new capital city is built or not, an aggressive sustainability intervention is required to avoid non-substitutable losses of critical natural capital generating essential ecosystem service benefits to society for poverty alleviation. Lack of state, local, and other non-state actors’ interventions will exacerbate forest resource depletion, contribute to the destruction of wildlife habitats, accelerate ecosystem services losses, and undermine national and global climate change mitigation efforts. Worth to stress that some of the sustainability strategies are even more critically important, considering the effects of constructing a new capital city in the study area, as inferred by public opinion.
This study attributes the critical drivers of LULC change to deforestation, urbanization, industrial expansion, real estate development, climate change impacts, timber extraction, migration, and population density. Some of the factors are necessary for economic growth but not suitable for sustainable growth or development. Therefore, I recommend that the government formulates a comprehensive environmental masterplan. The masterplan should, among other issues, focus on (a) reserving some forestlands for conservation easement projects and designating protected area (PAs) status to all existing open forest parks identified above, (b) providing market-based payment incentive schemes to local forest owners for supporting their livelihood means (c) preserving some grassland sites for urban agricultural development and horticultural production, and (d) leveraging on green infrastructure solutions to mitigate flood risk, accelerate the infiltration rates of stormwater, reduce urban heat island effects. Doing so will also uphold the region’s carbon storage and sequestration capacity, enhance biodiversity protection and conservation, sustain favorable precipitation patterns, recharge the underwater aquifers, and enhance the urban ecosystem’s overall livability.
There are a few limitations to this analysis. First, I could not find a reference map to use for accuracy assessment. Second, the InVEST carbon model is based on a simplified carbon cycle, which assumes a linear change in carbon sequestration over time (Tallis et al., Citation2011). The model does not account for other dynamic factors that influence the carbon cycle, such as carbon transfer from one pool to another. Also, there is still an unsettled debate on the marginal damage cost of a ton of carbon emitted and the discount rate to use for long-term climate change interventions (Almansa & Martínez-Paz, Citation2011; Gollier, Citation2002; Nordhaus, Citation2007; N. N. Stern, Citation2006: Nicholas Stern, Citation2008; Tol, Citation2005; Weitzman, Citation2007).
Public interest statement
The study contributes to land-use land cover (LULC) assessment and ecosystem service valuation (e.g. carbon sequestration) in the developing world– the first LULC change detection study in The Gambia. This paper focuses on changes in forest and settlement areas. Overall, this analysis reveals a forest cover loss of 22,408 ha (18% decrease) from 1985 to 2020 in the study area. The LULC change is primarily driven by increasing urbanization attributed to the growing urban population associated with the accelerating estate development and infrastructure expansion projects.
Supplemental Material
Download MS Word (24.4 KB)Acknowledgements
This research was funded by Stephen Polasky (Regents Professor and Fesler-Lampert Professor of Ecological/Environmental Economics). I would like to thank the reviewers for their time and inputs. Special thanks to Professors Stephen Polasky and Joseph F. Knight for their invaluable feedback.
Supplementary material
Supplemental data for this article can be accessed here.
Disclosure statement
No potential conflict of interest was reported by the author.
Data Availability Statement
The data that support the findings of this study are openly available in USGS’ Database EarthExplorer at https://earthexplorer.usgs.gov/image references are included in .
Additional information
Funding
Notes on contributors
Nfamara K. Dampha
Nfamara K Dampha, a Gambian, is a World Bank Research Consultant/Fellow working with the World Bank-UNHCR Joint Data Center on Forced Displacement. Nfamara holds a Ph.D. in Climate Change &Natural Resource Economics and a Master’s degree in International Development from the University of Minnesota. His professional and research interests focus on international development practice, natural resource economics (i.e., ecosystem service valuation), GIS-based remote sensing applications, global migration studies, climate change policy, and disaster risk reduction, especially in developing countries. Nfamara was onetime the Director of Administration at the National Disaster Management Agency- Gambia. He is also the Founding Executive Director of a registered nonprofit organization called Household Disaster Resilience Project-Gambia (known as HELP-Gambia).
Notes
1. The RF, a nonparametric machine learning classifier, is receiving wide-spread application in the remote sensing community because of its high classification accuracy, insensitivity to noise, ability to handle overtraining effects (Breiman, Citation1999; Chan & Paelinckx, Citation2008; Ghimire et al., Citation2010; Pal, Citation2005).
2. I did not use Sentinel-2 for conducting the LULC change detection from earlier periods because the satellite was recently launched in 2015.
3. It assumes that the sequestered value of a ton of carbon is equivalent to the costs of avoided social damage of not releasing the same ton of carbon into the atmosphere (Tol, Citation2005, Stern 2007, cited; Tallis et al., Citation2011).
4. The InVEST Carbon model equation, copied from model documentation (for more details, see NatCap, Citation2020; Tallis et al., Citation2011).
5. According to the Department of Parks and Wildlife (2013), “there are 125 species of mammals, 77 species of reptiles, 30 species of amphibians, 1005 flowering plants, 576 of birds, and 784 of insects in The Gambia” (Parks and Wildlife Policy Gambia, Citation2013).
6. NCCR stands for the Second National Climate Communication Report (2012).
7. Given that most mangrove forests are generally situated in wetlands, we combine these two classes as a single classification unit in our geographic information system (GIS) based analysis. According to the country’s Second National Climate Communication Report (2012), mangrove forest accounts for 6.5% of the national territory.
8. A hectare equals to 11.12 pixels (Landsat image pixel size is 0.09 or 30*30 or 900sqm).
References
- Abere, T., Adgo, E., & Afework, S. (2020). Trends of land use/cover change in Kecha-Laguna paired micro watersheds, Northwestern Ethiopia. Cogent Environmental Science, 6(1), 1–25. https://doi.org/https://doi.org/10.1080/23311843.2020.1801219
- Agarwal, C., Green, G. M., Grove, J. M., Evans, T. P., & Schweik, C. M. (2002). A review and assessment of land-use change models: Dynamics of space, time, and human choice. Apollo The International Magazine Of Art And Antiques, 62.
- Ali Bah, O., Kone, T., Yaffa, S., & Ndiaye, M. L. (2019). Land use and land cover dynamics in central river region of the Gambia, West Africa from 1984 to 2017. American Journal of Modern Energy, 5(2), 5. https://doi.org/https://doi.org/10.11648/j.ajme.20190502.11
- Almansa, C., & Martínez-Paz, J. M. (2011). What weight should be assigned to future environmental impacts? A probabilistic cost benefit analysis using recent advances on discounting. Science of the Total Environment, 409(7), 1305–1314. https://doi.org/https://doi.org/10.1016/j.scitotenv.2010.12.004
- Amuzu, J., Jallow, B. P., Kabo-Bah, A. T., & Yaffa, S. (2018a). The climate change vulnerability and risk management matrix for the coastal zone of The Gambia. Hydrology, 5(1), 1. https://doi.org/https://doi.org/10.3390/hydrology5010014
- Arrow, K. J., Cline, W. R., Maler, M., Munasinghe, M., Squitieri, R., & Stiglitz, J. E. (1996). Intertemporal equity, discounting, and economic efficiency. In Economic and social dimensions of climate change,130-142. University of Cambridge.
- BBC. (2020). (54) The trees that bleed: How rosewood is smuggled from Senegal into Gambia - BBC africa eye documentary with umaru fofana - youTube. Retrieved January 6, 2021, from https://www.youtube.com/watch?v=G_GmLPPNbGc&t=685s
- Bellassen, V., & Luyssaert, S. (2014). Carbon sequestration: Managing forests in uncertain times. Nature, 506(7487), 153–155. https://doi.org/https://doi.org/10.1038/506153a
- Bensouda, & Co Lp, A. (2013). Improving land sector governance in the gambia implementation of the land governance assessment framework (LGAF). Washington, D.C.: World Bank Group. http://documents.worldbank.org/curated/en/343691504860284518/Improving-land-sector-governance-in-The-Gambia-implementation-of-the-Land-Governance-Assessment-Framework-LGAF
- Bijl, E. (2011). The cause of coastal erosion on a nourished beach in Kololi, The Gambia, (February). Delft University of Technology (TU Delft). https://repository.tudelft.nl/islandora/object/uuid%3Af3d75b6d-356f-4694-b644-6f23b87e4eeb
- Breiman, L. (1999). Randon Forests. Machinelearning202.Pbworks.Com, 1–35. http://machinelearning202.pbworks.com/w/file/fetch/60606349/breiman_randomforests.pdf
- Brown, S., Kebede, A. S., & Nicholls, R. J. (2011). Sea-level rise and impacts in Africa 2000 to 2100, (April 2011), 215. https://www.researchgate.net/publication/307210112_Sea-Level_Rise_and_Impacts_in_Africa_2000_to_210
- Butler, R. (2011). African forests store 25% of tropical forest carbon. Mongabay. Retrieved March 10, 2020, https://news.mongabay.com/2011/06/african-forests-store-25-of-tropical-forest-carbon/
- Chan, J. C. W., & Paelinckx, D. (2008). Evaluation of random forest and adaboost tree-based ensemble classification and spectral band selection for ecotope mapping using airborne hyperspectral imagery. Remote Sensing of Environment, 112(6), 2999–3011. https://doi.org/https://doi.org/10.1016/j.rse.2008.02.011
- Chenoweth, J., Anderson, A. R., Kumar, P., Hunt, W. F., Chimbwandira, S. J., & Moore, T. L. C. (2018). The interrelationship of green infrastructure and natural capital. Land Use Policy, 75, 137–144. https://doi.org/https://doi.org/10.1016/j.landusepol.2018.03.021
- Chichilnisky, G., Hammond, P. J., & Stern, N. (2020). Fundamental utilitarianism and intergenerational equity with extinction discounting. Social Choice and Welfare, 54(2–3), 397–427. Springer Berlin Heidelberg. https://doi.org/https://doi.org/10.1007/s00355-019-01236-z
- Cline, W. R. (1992). The economics of global warming. (Institute for International Economics, Ed.).
- Coates, T., & Manneh, A. (2015). GCCA Integrated Coastal Zone Management and the mainstreaming of climate change. The Gambia-European Union Coorporation. The Global Climate Change Alliance Plus Initiative.
- Dampha, N. K. (2020). Ecosystem services and coastal adaptation to climate change : An interdisciplinary science-based application in The Gambia. University of Minnesota.
- Dampha, N. K., Fogelson, S., Osborne, L., & Shokohzadeh, A. (2017). Mitigating the impact of illegal cattle ranching on deforestation in protected environmental areas in mesoamerica. University of Minnesota.
- Dasgupta, P. (2007). Commentary: The Stern review’s economics of climate change. In National institute economic review,199 (1), 4-7.
- Davidson, M. D. (2014). Zero discounting can compensate future generations for climate damage. Ecological Economics, 105, 40–47. https://doi.org/https://doi.org/10.1016/j.ecolecon.2014.05.018
- Davidson, M. D. (2015). Climate change and the ethics of discounting. Wiley Interdisciplinary Reviews: Climate Change, 6(4), 401–412. https://doi.org/https://doi.org/10.1002/wcc.347
- Drammeh, F. (2012). Assessing and adapting to climate-change induced sea-level rise on the Southern coastline of The Gambia. Oceans and Law of Sea. https://www.un.org/Depts/los/nippon/unnff_programme_home/fellows_pages/fellows_papers/Drammeh_1314_Gam.pdf
- FAO. (2020). Global forest resource assessment 2020. Food and Agricultural Organization of the United Nations (FAO). Retrieved January 6, 2021, from http://www.fao.org/forest-resources-assessment/2020
- First National Climate Communication. (2003). First national communication of the republic of The Gambia to the United nations framework convention on climate change. UNCDF. Retrieved from https://www.uncdf.org/article/4790/first-national-communication-of-the-republic-of-the-gambia-to-the-unfccc
- Forest Ecology Network. (2020). Carbon Sequestration Facts. Retrieved March 10, 2020, from http://www.forestecologynetwork.org/climate_change/sequestration_facts.html
- Forestry Sub-Sector Policy Gambia. (2010). FORESTRY SUB-SECTOR POLICY REPUBLIC OF THE GAMBIA forest policy of The Gambia. Food and Agricultural Organization of the United Nations (FAO). Retrieved from http://extwprlegs1.fao.org/docs/pdf/gam148213.pdf
- Gambia, F. P. (2010). FORESTRY SUB-SECTOR POLICY REPUBLIC OF THE GAMBIA forest policy of The Gambia.
- Garnaut, R. (2008). The garnaut climate change review. Cambridge University Press has.
- Gashaw, T., Tulu, T., Argaw, M., & Worqlul, A. W. (2017). Evaluation and prediction of land use/land cover changes in the Andassa watershed, Blue Nile Basin, Ethiopia. Environmental Systems Research, 6, 1. https://doi.org/https://doi.org/10.1186/s40068-017-0094-5
- Gaston, G., Brown, S., Lorenzini, M., & Singh, K. D. (1998). State and change in carbon pools in the forests of tropical Africa. Global Change Biology, 4(1), 97–114. https://doi.org/https://doi.org/10.1046/j.1365-2486.1998.00114.x
- GBoS. (2013a). National migration analysis. The Gambia Bureau of Statistics (GOBS).
- GBoS. (2013b). Population and housing census- spatial distribution. 2017. https://doi.org/https://doi.org/10.1049/oap-cired.2017.1227
- GBoS. (2013c). The Gambia 2013 population and housing census preliminary results 2013. The Gambia bureau of statistics. Standford Libraries. Retrieved from https://searchworks.stanford.edu/view/11968270
- GBoS. (2020). Gambia environment statistics compendium. The Gambia Bureau of Statistics (GBOS). Retrieved from https://www.gbosdata.org/downloads/environmental-statistics-61
- Ghimire, B., Rogan, J., & Miller, J. (2010). Contextual land-cover classification: Incorporating spatial dependence in land-cover classification models using random forests and the Getis statistic. Remote Sensing Letters, 1(1), 45–54. https://doi.org/https://doi.org/10.1080/01431160903252327
- Global Footprint Network. (2020). Open data platform. Retrieved July 1, 2020, from http://data.footprintnetwork.org/?_ga=2.247077197.87597779.1593553147-1277782639.1592280853&_gac=1.229779182.1593583579.CjwKCAjwxev3BRBBEiwAiB_PWCMxQFVdSDZtn93ku0kKHR5FTgDVl_QyVTQNyWTTZX0t96uaOU9l2xoCw8gQAvD_BwE#/
- Gollier, C. (2002). Discounting an uncertain future. Journal of Public Economics, 85(2), 149–166. https://doi.org/https://doi.org/10.1016/S0047-2727(01)00079-2
- Gomez, A. (2015). Social vulnerability to coastal erosion : Empirical assessment of gunjur village in The Gambia. WASCAL (West African Science Service Centre on Climate Change and Adapted Land Use). Retrieved from http://197.159.135.214/jspui/handle/123456789/79
- Gomez, M. L. A., Adelegan, O. J., Ntajal, J., & Trawally, D. (2020). Vulnerability to coastal erosion in The Gambia: Empirical experience from Gunjur. International Journal of Disaster Risk Reduction, 45(August2019), 101439. https://doi.org/https://doi.org/10.1016/j.ijdrr.2019.101439
- Gorte, R. W. (2007). Carbon sequestration in forests. Congressional Research Service. Retrieved from https://books.google.com/books?hl=en&lr=&id=g-T-rvi33gcC&oi=fnd&pg=PT3&dq=Gorte,+R.+W.+(2007).+Carbon+sequestration+in+forests.&ots=tPqJOYcpuO&sig=d7LmbxVUmXSlacSYTI6OXCC2eHU#v=onepage&q&f=false
- Government of The Gambia. (2017). The Gambia National Development Plan (2018-2021) - An Abridged Version. The Gambia National Development Plan (2018-2021) - An abridged version. 1–36. The Gambia Times. Retrieved from https://www.thegambiatimes.com/wp-content/uploads/2018/02/1.-The-Gambia-National-Development-Plan-2018-2021-Full-Version.pdf
- Hardin, G. (2009). The tragedy of the commons. Journal of Natural Resources Policy Research, 1(3), 243–253. https://doi.org/https://doi.org/10.1080/19390450903037302
- Harris, N. L., Brown, S., Hagen, S. C., Saatchi, S. S., Petrova, S., Salas, W., … Lotsch, A. (2012). Baseline map of carbon emissions from deforestation in tropical regions. Science, 336(6088), 1573–1576. https://doi.org/https://doi.org/10.1126/science.1217962
- Hassan, M. M., Smith, A. C., Walker, K., Rahman, M. K., & Southworth, J. (2018). Rohingya refugee crisis and forest cover change in Teknaf, Bangladesh. Remote Sensing, 10(5), 1–20. https://doi.org/https://doi.org/10.3390/rs10050689
- Heß, S., Jaimovich, D., & Sch, M. (2018). Community-driven deforestation? Experimental evidence from a rural development program in West Africa. Unpublished Manuscript, (September). https://doi.org/https://doi.org/10.13140/RG.2.2.24429.44007
- Hills, J., & Manneh, A. (2014). GCCA support project to The Gambia. Contract No: 324-146 applied coastal vulnerability assessment final report. Nebula. Retrieved from http://nebula.wsimg.com/87983b75bd8601772eb8d83ae7d01276?AccessKeyId=A43CF17DA3C5C7C121C9&disposition=0&alloworigin=1
- Houghton, R. A., & Hackler, J. L. (2006). Emissions of carbon from land use change in sub-Saharan Africa. Journal of Geophysical Research: Biogeosciences, 111(2), n/a-n/a. https://doi.org/https://doi.org/10.1029/2005JG000076
- Houghton, R. A., House, J. I., Pongratz, J., Van Der Werf, G. R., Defries, R. S., Hansen, M. C., … Ramankutty, N. (2012). Carbon emissions from land use and land-cover change. Biogeosciences, 9(12), 5125–5142. https://doi.org/https://doi.org/10.5194/bg-9-5125-2012
- Humboldt University Geospatial Online Class. (2014). Accuracy Metrics. Humboldt State University. Retrieved April 26, 2020, from http://gis.humboldt.edu/OLM/Courses/GSP_216_Online/lesson6-2/metrics.html
- IPCC. (2014). Climate change 2014 synthesis report. Contribution of Working Groups I, II and III to the Fifth Assessment Report of the Intergovernmental Panel on Climate Change,151. https://www.ipcc.ch/report/ar5/syr/
- Jallow, B., Barrow, M., & Leatherman, S. (1996). Vulnerability of the coastal zone of The Gambia to sea level rise and development of response strategies and adaptation options. Climate Research, 6(2), 165–177. https://doi.org/https://doi.org/10.3354/cr006165
- Kubiszewski, I., Costanza, R., Franco, C., Lawn, P., Talberth, J., Jackson, T., & Aylmer, C. (2013). Beyond GDP: Measuring and achieving global genuine progress. Ecological Economics, 93, 57–68. https://doi.org/https://doi.org/10.1016/j.ecolecon.2013.04.019
- Kumar, P., & Smith, R. B. W. (2019). Measuring sustainability and well-being. United Nations Environment Programme.
- Lambin, E. F., Geist, H. J., & Lepers, E. (2003). DYNAMICS OF L AND –USE AND L AND –C OVER C HANGE IN TROPICAL REGIONS. Annual Review of Environment and Resources, 28(1), 205–241. https://doi.org/https://doi.org/10.1146/annurev.energy.28.050302.105459
- Le Quéré, C., Andrew, R. M., Friedlingstein, P., Sitch, S., Pongratz, J., Manning, A. C., … Zhu, D. (2018). Global Carbon Budget 2017. Earth System Science Data, 10(1), 405–448. https://doi.org/https://doi.org/10.5194/essd-10-405-2018
- Lewis, S. L., Lopez-Gonzalez, G., Sonké, B., Affum-Baffoe, K., Baker, T. R., Ojo, L. O., … Wöll, H. (2009). Increasing carbon storage in intact African tropical forests. Nature, 457(7232), 1003–1006. https://doi.org/https://doi.org/10.1038/nature07771
- Managi, S., & Kumar, P. (2018). Inclusive Wealth Report 2018. Inclusive Wealth Report 2018. Routledge. https://doi.org/https://doi.org/10.4324/9781351002080
- Mongabay. (2006). Gambia: Environmental profile. Retrieved March 10, 2020, from https://rainforests.mongabay.com/20gambia.htm
- Moyo, D. (2009). Dead Aid: Why aid is not working and how there is a better way for Africa. Straus and Giroux.
- Banjul . (2007). UN Framework Convention on Climate Change (UNFCCC).NAPA Gambia. https://unfccc.int/resource/docs/napa/gmb01.pdf
- NatCap. (2020). InVEST carbon storage and sequestration model |documentation| natural capital project. Stanford University. Retrieved April 24, 2020, file:///C:/InVEST_3.8.0_x86/documentation/userguide/carbonstorage.html
- Nations, U. (2018). 68% of the world population projected to live in urban areas by 2050, says UN | UN DESA | United Nations Department of Economic and Social Affairs. United Nations. Retrieved February 15, 2020, https://www.un.org/development/desa/en/news/population/2018-revision-of-world-urbanization-prospects.html
- Nelson, E., Mendoza, G., Regetz, J., Polasky, S., Tallis, H., Cameron, D. R., … Shaw, M. R. (2009). Modeling multiple ecosystem services, biodiversity conservation, commodity production, and tradeoffs at landscape scales. Frontiers in Ecology and the Environment, 7(1), 4–11. https://doi.org/https://doi.org/10.1890/080023
- Net, P. P. (2019). Population of Gambia 2050 - PopulationPyramid.net. Retrieved April 21, 2020, https://www.populationpyramid.net/gambia/2050/
- Nordhaus, W, D. 2007. A Review of the Stern Review on the Economics of Climate Change. Journal of Economic Literature, 45 (3): 686–702. doi: https://doi.org/10.1257/jel.45.3.686
- Nordhaus, W. D. (2007). A review of the stern review on the economics of climate change. Climate Change and Water Resources, XLV(September), 153–182. https://doi.org/https://doi.org/10.1201/b16969
- Nordhaus, W. D. (2008). A question of balance: Weighting the options on global warming policies. Yale University Press.
- Nordhaus, W. D., & Boyer, J. (2000). Warming the World. Economic models of global warming. The MIT Press Cambridge.
- Nyangado, L., & Pouakouyou, D. (2017). Project title: Large-scale ecosystem-based adaptation in the Gambia: Developing a climate-resilient, natural resource-based economy executing entity: Government of Gambia. Green Climate Fund. https://www.greenclimate.fund/project/fp011
- Ostrom, E., & Cox, M. (2010). Moving beyond panaceas: A multi-tiered diagnostic approach for social-ecological analysis. Environmental Conservation, 37(4), 451–463. https://doi.org/https://doi.org/10.1017/S0376892910000834
- Pal, M. (2005). Random forest classifier for remote sensing classification. International Journal of Remote Sensing, 26(1), 217–222. https://doi.org/https://doi.org/10.1080/01431160412331269698
- Parks and Wildlife Policy Gambia. (2013). The republic of the gambia the national parks and wildlife policy of the gambia ministry of environment, parks and wildlife february, 2013. Ministry of Environment, Climate Change and Natural Resources. Retrieved from: https://meccnar.gm/
- Polasky, S., Bryant, B., Hawthorne, P., Johnson, J., Keeler, B., & Pennington, D. (2015). Inclusive Wealth as a Metric of Sustainable Development. Annual Review of Environment and Resources, 40(1), 445–466. https://doi.org/https://doi.org/10.1146/annurev-environ-101813-013253
- Poston, B. (2009). An exercise in personal exploration: Maslow’s hierarchy of needs. The Surgical Technologist, 41(8), 347–353. https://doi.org/https://doi.org/10.1093/bjc/azi029
- Pradhan, B. B., Chaichaloempreecha, A., & Limmeechokchai, B. (2019). GHG mitigation in agriculture, forestry and other land use (AFOLU) sector in Thailand. Carbon Balance and Management, 14(1), 3. https://doi.org/https://doi.org/10.1186/s13021-019-0119-7
- Rawat, J. S., & Kumar, M. (2015). Monitoring land use/cover change using remote sensing and GIS techniques: A case study of Hawalbagh block, district Almora, Uttarakhand, India. Egyptian Journal of Remote Sensing and Space Science, 18(1), 77–84. https://doi.org/https://doi.org/10.1016/j.ejrs.2015.02.002
- Rendall, M. (2019). Discounting, climate change, and the ecological fallacy. Ethics, 129(3), 441–463. https://doi.org/https://doi.org/10.1086/701481
- Saatchi, S. S., Harris, N. L., Brown, S., Lefsky, M., Mitchard, E. T. A., Salas, W., … Morel, A. (2011). Benchmark map of forest carbon stocks in tropical regions across three continents. Proceedings of the National Academy of Sciences of the United States of America, 108(24), 9899–9904. https://doi.org/https://doi.org/10.1073/pnas.1019576108
- Second National Climate Communication. (2012). Gambia’s second national communication the gambia’s second national communication under the united nations framework convention on climate change banjul. United Nations Framework Convention on Climate Change (UNFCCC). Retrieved from: https://unfccc.int/resource/docs/natc/gamnc2.pdf
- Sen, A. (1982). Approaches to the choice of discount rates for social benefit-cost analysis. Discounting for time and risk in energy policy. (W. R. Lind, R. C. Arrow, K. J. Corey, G. R. Dasgupta, P. Sen, A. K. Stauffer, T. Stiglitz, & J. E. Stockfisch JA, Eds.). Resources for the Future, Inc., Washington D.C., John Hopkins University Press.
- Sillah, J. S. (1999). Forest resources and plantations of the Gambia, 2015 (4/15/2015). Food and Agriculture Organization of the United Nations (FAO). Retrieved from: http://www.fao.org/3/X6808E/X6808E06.htm
- Smith P., M. Bustamante, H. Ahammad, H. Clark, H. Dong, E.A. Elsiddig, H. Haberl, R. Harper, J. House, M. Jafari, O. Masera, C. Mbow, N.H. Ravindranath, C.W. Rice, C. Robledo Abad, A. Romanovskaya, F. Sperling, and F. Tubiello, 2014:Agriculture, Forestry and Other Land Use (AFOLU). In: Climate Change 2014: Mitigation of Climate Change. Contribution of Working Group III to the Fifth Assessment Report of the Intergovernmental Panel on Climate Change [Edenhofer, O., R. Pichs-Madruga, Y. Sokona, E. Farahani, S. Kadner, K. Seyboth, A. Adler, I. Baum, S. Brunner, P. Eickemeier, B. Kriemann, J. Savolainen, S. Schlömer, C. von Stechow, T. Zwickel and J.C. Minx (eds.)]. Cambridge University Press, Cambridge, United Kingdom and New York, NY, USA.
- Stern, N. (2006). The stern review on the economics of climate change. UK. Cambridge University Press.
- Stern, N. (2008). The economics of climate change. American Economic Review, 98:(2), 1–37. https://doi.org/https://doi.org/10.1257/aer.98.2.1
- Tallis, H. T., Ricketts, T., Guerry, A. D., Wood, S. A., Sharp, R., Nelson, E., … Verutes, J. (2011). InVEST 2.2.0 user’s guide: Integrated valuation of environmental services and tradeoffs. Journal of Chemical Information and Modeling, 53. http://neat.ecosystemsknowledge.net/pdfs/invest_tool_review.pdf
- Tol, R. S. J. (2005). The marginal damage costs of carbon dioxide emissions: An assessment of the uncertainties. Energy Policy, 33(16), 2064–2074. https://doi.org/https://doi.org/10.1016/j.enpol.2004.04.002
- Tol, R. S. J. (2006). The economics of climate change: The stern review. The Economics of Climate Change: The Stern Review, 9780521877(2003), 1–692. https://doi.org/https://doi.org/10.1017/CBO9780511817434
- Udo, V. E., & Jansson, P. M. (2009). Bridging the gaps for global sustainable development: A quantitative analysis. Journal of Environmental Management, 90(12), 3700–3707. https://doi.org/https://doi.org/10.1016/j.jenvman.2008.12.020
- United Nations, U., European Union, E., Food and Agriculture Organization, F., International Monetary Fund, I., Organization of Economic Co-operration and Development, O., & World Bank, W. (2012). System of environmental-economic accounting, central framework. Voenno-meditsinskii zhurnal. https://unstats.un.org/unsd/publication/seriesm/seriesm_86rev1e.pdf
- Urquhart, P. (2016). National climate change policy of The Gambia: Final report: Final draft policy, (January), 1–65. Grantham Institute. https://www.lse.ac.uk/GranthamInstitute/wp-content/uploads/laws/8109.pdf
- Van Der Werf, G. R., Morton, D. C., Defries, R. S., Olivier, J. G. J., Kasibhatla, P. S., Jackson, R. B., … Randerson, J. T. (2009). CO2 emissions from forest loss. Nature Geoscience, 2(11), 737–738. Nature Publishing Group. https://doi.org/https://doi.org/10.1038/ngeo671
- Wackernagel, M., & Beyers, B. (2019). Ecological footprint: Managing our biocapacity budegt (global footprint network). New Society.
- Wackernagel, M., Lin, D., Evans, M., Hanscom, L., & Raven, P. (2019). Defying the footprint oracle: implications of country resource trends. Sustainability, 11(7), 2164. https://doi.org/https://doi.org/10.3390/su11072164
- Wackernagel, M., Onisto, L., Bello, P., Linares, A. C., Falfán, I. S. L., García, J. M., … Guerrero, M. G. S. (1999). National natural capital accounting with the ecological footprint concept. Ecological Economics, 29(3), 375–390. https://doi.org/https://doi.org/10.1016/S0921-8009(98)90063-5
- WB-Database. (2018). World development indicators | databank. World Bank Retrieved December 28, 2018, from https://databank.worldbank.org/data/indicator/NY.GDP.MKTP.CD/1ff4a498/Popular-Indicators#
- Weier, J., & Herring, D. (2020). Measuring vegetation (NDVI & EVI). Earth Observatory, National Aeronautics and Space Administration (NASA). Retrieved December 29, 2020, from https://earthobservatory.nasa.gov/features/MeasuringVegetation
- Weitzman, M. L. (2007). A review of the stern review on the economics of climate change. Social Work (United States), 20(3), 254. https://doi.org/https://doi.org/10.1093/sw/20.3.254-a
- World Bank. (2020a). GDP per capita (current US$) - Gambia, The | Data. Retrieved January 4, 2021, https://data.worldbank.org/indicator/NY.GDP.PCAP.CD?locations=GM
- World Bank. (2020b). World development indicators | databank. Retrieved July 17, 2020, https://databank.worldbank.org/reports.aspx?source=2&series=SP.POP.GROW
- World Population Review. (2018). Gambia population 2018 (demographics, maps, graphs). Retrieved December 13, 2018, http://worldpopulationreview.com/countries/gambia-population/
- WWF. (2015). Policy brief: Expectation paper COP 21 Paris, 1–4. World Wildlife Fund (WWF). Retrieved from https://wwf.panda.org/discover/our_focus/forests_practice/forest_climate/forest_climate_publications/forest_climate_policy_documents/?256973/Policy%2Dexpectations%2Dfor%2Dforests%2Dand%2Dthe%2Dland%2Dsector%2Dat%2DCOP21
- Yang, S., Zhao, W., Liu, Y., Wang, S., Wang, J., & Zhai, R. (2018). Influence of land use change on the ecosystem service trade-offs in the ecological restoration area: Dynamics and scenarios in the Yanhe watershed, China. Science of the Total Environment, 644, 556–566. https://doi.org/https://doi.org/10.1016/j.scitotenv.2018.06.348
- Yohe, G. (2006). Some thoughts on the damage estimates presented in the stern review. Integrated Assessment, 6(3), 65–72. http://gyohe.faculty.wesleyan.edu/files/2018/05/71.pdf