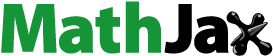
Abstract
One of the functions of a brake system is to reduce or maintain the speed of a vehicle. The braking process generally converts kinetic energy into heat. While a direct contact brake system functions using friction, a non-contact brake system such as an eddy current brake (ECB) relies on eddy currents. As repeated braking increases the temperature of the brake components, it decreases performance and, in extreme cases, can result in brake failure. In an ECB system, heat typically accumulates in the conductor material used to generate the braking force. When a conductor material is repeatedly subjected to excessive heat, its properties change affecting braking performance. This has prompted to the use of liquid- and air-based cooling in ECB systems, both of which have their respective advantages and disadvantages. Therefore, this present review extensively investigates the potential of using these refrigerants in various types of ECB systems.
Reviewing Editor:
1. Introduction
The purpose of a brake system is to reduce the speed of a vehicle and stop its movement. While braking, a brake system absorbs kinetic and potential energy and releases this energy in the form of heat (Day & Newcomb, Citation1984). As such, the heat generated by repeated braking must be removed using a cooling process, as failure to remove all the heat will adversely affect braking performance and lead to brake failure. As such, brake components require regular maintenance and replacement. Multiple brake systems can be combined to extend the life cycles of the brake components. For instance, a mechanical brake system can be combined with an engine brake system to provide good braking performance as well as extend component life (Zou et al., Citation2009).
Brake components undergo regular maintenance to extend the lifespan of a brake system. Friction-based brake systems are rife with risks of failure. While examining the risk of disc brake failure in motorcycles, Boniardi et al. (Citation2006) concluded that disc brake failure occurs at specific intervals and that brake shoes suffer from damage. Abutu et al. (Citation2018) examined reinforcing brake shoes, while Collignon et al. (Citation2013) discovered that premature brake failure occurs in trucks due to thermal fatigue cracks (Bagnoli et al., Citation2009; Mackin et al., Citation2002; Mazzù et al., Citation2019; Wang et al., Citation2019). Brake failures can often be traced to damaged mechanical components with contact parts; such as disc brakes and brake shoes, withstanding the worst of the damage. Apart from functioning as friction receivers, discs are also heat releasers (Ma et al., Citation2020; Reyes et al., Citation2019; Zhang et al., Citation2019). Therefore, a practical and durable brake system can significantly reduce the likelihood of road accidents (Pawar et al., Citation2020). Extremely high strain is one of the reasons for brake system damage (Kamla et al., Citation2019). Multiple studies have shown that brake system failure is one of the leading causes of road accidents (Ghaleh et al., Citation2019; Kamla et al., Citation2019; Rainey et al., Citation2019). As such, drivers who repeatedly accelerate and decelerate at high speeds face a greater risk of brake system failure (Dubos et al., Citation2016).
Developments in transportation technology have led to the introduction of electric and hybrid vehicles. Although electric vehicles have better energy efficiency and are environmentally friendly, it is not feasible to equip them with engine brakes. As such, electric vehicles are equipped with regenerative brakes that can both support and generate electricity (“Effect of Regenerative Braking Energy,” Citation2016; N. Luo et al., Citation2014) that is then stored in batteries for reuse. A non-contact brake system; such as an eddy current brake (ECB) system; can be used as a support system. An ECB system consists of a magnetic field source and a conductor, where the conductor uses eddy current to produce a braking force. Therefore, an ECB system can be combined with and serve as a support system for a regenerative brake system to enhance energy efficiency (Yazdanpanah & Mirsalim, Citation2015b). An ECB system is typically used to improve braking performance and reduce the risk of mechanical failure. For instance, an ECB system can be used to ensure that the wind turbine's turbine speed does not exceed critical levels (Jee et al., Citation2012). Apart from light vehicles, such as cars and motorcycles, an ECB system can also be used in high-speed vehicles, such as aircraft and locomotives (L. Luo et al., Citation2017; A. K. Singh, Citation2015).
An ECB is an electric braking system that relies on eddy currents created using a magnetic field source and a conductor. In terms of structure, ECBs can be grouped according to axial, radial, linear, and retarder types. This study focuses on the heat that ECBs produce in accordance with their structure. Some ECBs perform better than others do. For instance, a unipolar ECB design has several advantages over other ECB designs (Anon, Citation2000). An electromagnet has also been found to provide more easily adjustable braking performance than a permanent magnet. In an ECB system, an electric coil is used to produce a magnetic field, and the volume of electrical power determines the amount of magnetic flux produced by winding. The higher the electrical power, the greater the resulting magnetic field. Good ECB performance also depends on an appropriate current signal frequency and shape. Alternating current (AC) has been found to increase braking torque (Haas, 2008). However, one of the main difficulties in producing good ECB braking performance is its settings for low speeds.
The performance of an ECB system can be improved by increasing the density of the magnetic field in the air gap. A commonly held belief is that a magnetic field from one side is required to produce inductance. However, studies have indicated that using magnetic fields on both sides increases the density of the resulting magnetic field. Extant studies have also examined altering the air gap (Anantha Krishna & Sathish Kumar, Citation2014) as it affects braking performance. However, these studies focused more on altering the density of the magnetic field in the air gap than the influence of the magnetic field used. Meanwhile, other studies have concluded that changing the position of the magnetic field also affects braking performance (A. K. Singh & Sharma, Citation2014). For instance, altering the position of the shoe pole affects the braking performance of an ECB system.
At present, not many studies have investigated the production of eddy currents, particularly the heat generated during braking. Anantha Krishna and Sathish Kumar (Citation2014) only discussed ECB systems in general, while A. K. Singh and Sharma (Citation2014) conducted a systematic review of the various uses of ECB system designs. Similarly, Thompson (Citation2009) conducted a systematic review of using permanent neodymium magnets in various applications, one of which was an ECB system, while Waloyo et al. (Citation2019) focused on the development of ECB designs. Only a handful of studies have reviewed the effect of rotor materials; such as aluminium, copper, and ferromagnetic metals; on braking torque (Gulec et al., Citation2016) and adding grooves to rotor surfaces (Garbiec et al., Citation2014). Meanwhile, some concluded that stators made of permanent magnet materials increase the magnitude of the magnetic field (Yazdanpanah & Mirsalim, Citation2015a) and that the addition of a back iron structure increases magnetic conductivity (Sinmaz et al., Citation2015), while others investigated the use of a ferrous metal construction covered with non-ferrous metal (Cho et al., Citation2017; Jin et al., Citation2016; Park et al., Citation2014) and the effect of using a Halbach array in an ECB system (Jee et al., Citation2012; L. Luo et al., Citation2017; A. K. Singh, Citation2015; Yazdanpanah & Mirsalim, Citation2015b).
Although the heat generated during braking is a part of the normal energy conversion process, it has to be regulated to prevent overheating. As heat is usually generated in contact areas, it affects the properties of these areas. The magnetic field applied to the braking energy source in ECBs affects the contact area, which is an active region. Therefore, the surface properties of the active region warrant analysis to determine the effects of repeated braking and its effect on braking ability. Heat dissipation is influenced by conduction, convection and radiation resistance. Therefore, the effect of contact area shape on heat generation should be examined as well. The resistance and thermal conductivity of a material have been found to play a significant role as well. However, not many studies have thermally analysed ECB performance. This present review comprehensively assesses all ECB design- and model development-related extant studies. It also discusses the basic principles of ECBs, existing ECB designs, ECB design development, ECB classifications, the performance of each ECB type and the heat analysis of the ECB braking process as well. Recommendations for the further development of ECB designs are also provided.
2. Eddy current working principle
Eddy currents are induced currents produces by changes in the magnetic field of a conductor. In a stationary conductor, eddy currents appear to be affected by magnetic field changes or when an object moves across a fixed magnetic field. As seen in , the induced current forms a loop. Eddy currents are released as heat due to the resistance of the conductor material. This induced eddy current, which flows in a loop within the conductor, produces an eddy current magnetic field (). Furthermore, the magnitude of the magnetic field produced is proportional to that of the induced current.
The skin effect significantly affects the braking torque of an ECB (Jang et al., Citation2001, Citation2002) and increases as the speed increases. Sharif and Sharif (Citation2009) posit that brake torque calculations should include the skin effect variable. According to Sharif et al. (Citation2012), the thickness of a conductor effects the skin effect. Schieber (Citation1972) reported that the skin effect was insignificant on thin plates, while A. Singh (Citation1977) reported that the skin effect was profound on thick plates. The skin effect significantly affects braking torque at high speeds (Choi & Jang, Citation2012).
The type of inductance material also significantly affects ECB braking performance. Inductance materials can be categorised as either magnetic materials with high permeability or non-magnetic materials with low permeability. Venkataratnam and Raju (Citation1977) examined the use of non-magnetic materials in axial ECBs while Edwards et al. (Citation1999) investigated the use of non-magnetic materials in radial ECBs. According to Kou et al. (Citation2014b), copper is a better inductance material than aluminium and zinc as it produces a relatively high braking torque at low speeds. However, this braking torque decreased at high speeds. Z. Zhang et al. (Citation2013) reported that the maximum braking torque of multiple inductance materials was the same; within the 5x S to 35 x
S range; however, materials with higher conductivity provided better braking torque at low speeds. A theoretical study by Gulec et al. (Citation2016) combined the varying conductivities and resistivities of the materials and the braking torque and expressed them in a graphic format. The study concluded that, theoretically, low-carbon steel provides the best braking torque. Conversely, Nehl et al. (Citation1994) argue that aluminium produces better braking torque than iron.
As ECBs perform poorly at low speeds, they can be improved by combining them with other systems that provide good braking performance. Obara et al. (Citation1995) developed and examined a hybrid braking system that combined ECBs and magnetic track brakes. As ECBs provide good braking at high speeds while reducing mechanical contact, it decreases the likelihood of brake system failure. The proposed braking system, which used a low-speed magnetic track, was equipped with brake pads enveloped in the brake material. In a similar study, Li et al. (Citation2011) combined ECBs with an electrically controlled hydraulic system that sets the distance between the source of the magnetic field and the rail. Meanwhile, J. Ma et al. (Citation2011) optimised ECB design using a non-magnetic barrier to reduce flux leakage. The use of a leakage barrier was found to increase the magnetic field density of the air gap, thereby, boosting the braking force. However, the system needs to be correctly adjusted to produce optimal braking torque. Ryoo et al. (Citation2000), on the other hand, determined the torque required by examining the current settings.
The cost of an ECB system is high as an external power source or a permanent magnet is required to activate it. A self-generating ECB system; that produces a magnetic field using electricity from an induction generator instead of a permanent magnet; can be used to overcome this drawback. Bae et al. (Citation2015) reported that self-generating ECB systems provide energy-savings and are cost-effective, while Cho et al. (Citation2017) reported improved energy efficiency by adjusting the capacitor size to fit the needs of the system.
3. Heat transfer mechanism on ECB
The fundamental idea behind calculating the heat an ECB generates is heat transfer. There are three types of heat transfer mechanisms: conduction, convection and radiation. During conduction, heat transfers through a material without moving its mass. This could be a solid object; such as a metal block; where the temperature of one surface is high and that of the other is lower. The turbine blades of a jet engine are a good example of heat conduction. The temperature of the outer surfaces of the turbine blades is high as they are exposed to the gases from the combustion chamber, while the temperature of the inner surfaces of the blades is lower as it encounter cooling air. Therefore, the wall temperature is essential for the turbine blades. The following is a simplified formula that can be used to analyse conduction:
where L (m) is the length of the cross-section, A ( is the cross-sectional area, and k (w/m°C) is the conductivity of the material. The L and A of a brake system appear in the conductor material area, which is the active region.
Convection, on the other hand, is the transfer of heat via flowing fluids, such as gas or liquid, both of which are commonly used in the aerospace industry. During convection, the bulk of the heat is transferred and displaced via the movement of fluids with non-uniform temperatures. In natural convection, differences in buoyancy due to density variations determine fluid motion, while an external force, such as a fan, blower, or pump; is used to control fluid motion in a forced convection system. High fluid velocity causes turbulence, which results in a significant drop in pressure. In such cases, a fan or pump can be used to reduce the fluid flow rate. As such, it is more efficient to mix hot and cold air as it increases heat transfer. The following equation can be used to easily calculate the convection thermal resistance of a surface:
This equation is, essentially, the same equation that is used to calculate radiation, but with the radiant heat transfer coefficient replaced with the convection heat transfer coefficient (w/m°C). A proven empirical heat transfer correlation, that is based on dimensionless analysis, can used to predict the
of all convection surfaces in a machine.
Meanwhile, radiation is the transfer of energy through a space that is devoid of transfer matter. It is the only method of heat transfer in space and is important in situations where there is an intermediate transfer material. Using a shiny piece of metal to start a fire is a good example of radiation heat transfer. The radiant thermal resistance of a surface can be easily calculated, where is the surface area and
(w/m°C) is the heat transfer coefficient. The surface area can be calculated using the geometry of the surface. The following equation can be used to calculate the radiation heat transfer coefficient:
where
is the surface emissivity,
is the display factor for scattering surface 1 onto absorbent surface 2 (ambient temperature for external radiation), and
are the surface temperatures.
Several processes occur during heat generation in an axial ECB. The primary process is braking, which causes a current to be generated on the surface of the discs. Heat is then released and transferred from the magnetic field source to the discs (). In a radial ECB, however, the active region does not come into direct contact with the air, as it is located on the inside of the drum. As this makes it even more difficult for its components to dissipate heat, a cooling system is required.
4. Commonly cooling technique
ECB cooling techniques; such as water- and liquid-cooling; can be combined with cooling techniques that are commonly used in electric motors or an eddy current clutch, where cooling is required due to the heat generated by the electronic process.
Eddy current clutches are generally cooled using forced air convection. Over continuous operation, the temperature of a rotor can rapidly rise to 600 °C. This can decrease braking torque by up to 40% in as little as 5 min. Forced air convection can also be used to cool braking torque and decrease the temperature by up to 160 °C in 5 min. According to Lu et al., permanent magnet linear motors are often supplied with large currents to achieve maximum performance. However, this causes a rapid and significant increase in temperature. ECBs also use magnets supplied with large currents and suffer from increases in temperature. To overcome this issue examined the efficacy of multiple types of cooling systems. The water-based cooling system was found to significantly decrease temperature while increasing performance. At the winding temperature limit of 155 °C, the water-based cooling system performed 2.76 times better than the non-water-based cooling system. The temperature of the permanent magnet also decreased by 72 K. lists the weaknesses and improvements that can be made to commonly used ECB cooling techniques.
Table 1. Air- vs. water-based cooling techniques.
5. Types of eddy current brakes and prediction of heat generation
An ECB is an electric braking system that operates on the eddy current principle. The main components of an ECB system are a magnetic field source and an inductor. ECBs are grouped according to axial, radial and linear structures. As different types of ECBs have varying braking processes, the heat patterns that they produce differ as well. The design of an ECB also significantly affects the heat patterns produced as well as heat dissipation ability. As the mechanical energy that ECBs absorb during braking is converted into heat, it increases the temperature of the conductor. Therefore, the choice of inductor must be able to efficiently dissipate heat into the atmosphere, as an increase in temperature will affect ECB performance.
5.1. Radial ECBs
Radial ECBs are shaped like a radial electric motor, where the main magnetic field and the eddy current radiate outwards from the centre of the axis. Due to this structure, radial ECBs are also called ECB drums. As seen in and , the magnetic field source of this type of ECB can be located internally or externally.
Figure 4. Radial eddy current brake: (a) inner stator (Sinmaz et al., Citation2015) and (b) outer stator (Karakoc et al., Citation2016).

Figure 5. Eddy current retarder with water cooling (Bigeon et al., Citation1983).

The braking torque setting of a radial permanent magnet ECB differs from that of an axial permanent magnet ECB. In a radial ECB, fine-tuning the location of the permanent magnet in the conductor rather than the size of the air gap adjusts the torque. While studying radial permanent magnet ECBs, Choi and Jang (Citation2012) inserted a permanent magnet into the magnetic induction hole. The magnetic induction disc had an impact on the size of the induced magnetic field, which in turn determined the strength of the magnetic field. Meanwhile, Jee et al. (Citation2012) used two layers of aluminium in the braking process and applied this system to a wind turbine.
Two layers of materials that bear different properties have been used to increase braking torque. Anwar and Stevenson (Citation2011) and (Bigeon & Sabonnadiere, Citation1985) investigated the use of a copper layer in a PCB while (Gulbahce et al., Citation2013) determined that coatings that were more than 0.15 mm in thickness decreased torque. Meanwhile, Cho et al. (Citation2017) added a ferromagnetic coating to the inductance drum of an ECB model (). Copper plating was added to the ECB model and its performance was compared using finite element analysis (FEA). Thicker coatings were found to increase braking torque at low speeds but decrease it at high speeds.
Figure 6. Design with coating (Cho et al., Citation2017).

Modifying the induction motor can also improve braking performance. Garbiec et al. (Citation2014) added a layer of material as well as grooves of varying amounts and depths to the surface of a braking drum to increase braking torque. Braking torque was found to decrease as the number of grooves and groove depth increased. This varied significantly from the results obtained from the induction motor, where adding more grooves and deeper grooves increased braking torque (). Longer rotors were also found to produce higher torque but this decreased when the rotor length exceeded 16 mm due to impedance.
Figure 7. (a) Torque at variations of groove depth and (b) uniform rotor (left) and slitted rotor with slits/grooves depth (right) (Garbiec et al., Citation2014).

The poles of the magnetic field can also be changed to increase braking torque. Park et al. (Citation2014) examined the use of a Halbach array to increase braking performance. The study aimed to determine the optimum number of permanent magnets or permanent magnet parts required to increase the braking torque of a system. The addition of a non-linear array of permanent magnet parts was found to increase braking torque.
The cost of an ECB system is high, as an external power source is required to activate it. Therefore, a self-generating ECB system; which produces a magnetic field using the electricity generated by an induction generator instead of a permanent magnet; can be used to overcome this limitation. According to Bae et al. (Citation2015), a self-generating ECB system not only conserves energy but is also cost-effective. Cho et al. (Citation2017), who adjusted the capacitor size to meet the needs of the system, also reported that a self-generating ECB system improves energy efficiency.
As the magnet in a radial ECB system is located within the drum, its heat dissipation is relatively low. Bigeon et al. (Citation1983) proposed installing a cooling system on the exterior of the drum to overcome this issue ().
Figure 8. Air-cooling system (Bigeon et al., Citation1983).

Furthermore, air-cooled ECBs provide relatively good braking up to medium speeds. However, liquid-cooled ECBs outperform air-cooled ECBs at high speeds ().
Figure 9. Air-cooled system versus water cooled system (Bigeon et al., Citation1983).

Gulec & Aydin (Citation2016) examined the use of a liquid coolant in an ECB system. depicts the design used. The use of a liquid coolant was found to maintain ECB performance during continuous use over a period. According to extant studies, the temperature of air-cooled ECBs can quickly climb to 600 °C and decrease braking performance by as much as 40%. At present, not many studies have examined cooling systems for radial ECBs.
Figure 10. Liquid-cooled system (Gulec & Aydin, Citation2016).

5.2. Linear ECBs
Unlike rotation-based models, linear ECBs have a relatively translational motion between the source and the conductor. In this type of system, several poles are arranged in series to form a single line (Lesobre et al., Citation2001). The source of the magnetic field moves while the conductor remains stationary. The relative motion between the magnetic field and the conductor induces a magnetic field. This magnetic field exerts a force in the opposite direction of the relative motion of the magnetic field to create braking. Linear ECBs are widely used in locomotives. As seen in , the magnetic field source is located on the bogie of the locomotive, with the railway tracks acting as the conductor.
Figure 11. Application of the electric braking system on the train bogie (Zhou et al., Citation2015).

Locomotives utilise ECB brakes at high speeds, as electric braking provides optimal braking that does not rely on friction. Albertz et al. (Citation1996) (Gosline & Hayward, Citation2008) investigated the braking process of linear ECBs while P. J. Wang and Chiueh (Citation1998) (Lesobre et al., Citation2001) provide an overview of their working ideology. depicts the structure and principles of railway ECBs.
Figure 12. Linear eddy current brake working principle (Ying et al., Citation2010).

As the mechanical energy, that the ECB absorbs during braking is converted into heat, the temperature of the conductor increases. As railway tracks act as conductors, they will experience an increase in temperature due to the eddy currents. Lu et al. (Citation2014) (Zhou et al., Citation2015) examined the temperature increase of linear ECBs during braking. This present review only analysed the results of their calculations to better understand the increase in temperature under multiple regulated conditions and did not analyse the torque generated. Lu et al. (Citation2014) found that increasing the distance of the magnetic source caused the temperature to decrease while increasing the applied current increased the temperature at high speeds.
By comparison, the temperature only rose marginally at low speeds. The temperature increase in railway tracks with densities of 60 kg/m (high-speed lines) and 75 kg/m (heavy-haul railways) was relatively the same at multiple different speeds. Therefore, the mass of the braking load does not affect the magnitude of temperature increase that the conductor material experiences. Jin et al. (Citation2016) investigated the effect of temperature on the components of a Halbach array linear ECB. The effect on each braking component was calculated as heat experienced. An increase in temperature on the side of the conductor was due to eddy losses. Furthermore, the back iron contained most of the heat propagated, while the other components were not significantly affected. Temperature increases of up to the maximum of 45 °C were recorded in the permanent magnets.
An electromagnet and a permanent magnet can be combined to increase the magnetic field density, which will, in turn, improve braking performance. Kou et al. (Citation2014a) (Baharom et al., Citation2012) used an analytical method to explain the performance of a linear ECB with a series arrangement (). This was accomplished by placing permanent magnets at the base of the winding core. The use of a combination of magnetic field sources was found to increase braking torque.
Figure 13. Series inserted permanent magnet (Baharom et al., Citation2012).

During the braking process, the railway track acts as the conductor and will experience an increase in temperature due to the eddy currents. Lu et al. (Citation2014) (Zhou et al., Citation2015) examined the correlation between braking and temperature increase in a linear ECB. This present review only analysed the results of their calculations to better understand the increase in temperature under multiple regulated conditions and did not analyse the torque generated. Lu et al. (Citation2014) found that increasing the distance of the magnetic source caused the temperature to decrease while increasing the applied current increased the temperature at high speeds.
5.3. Axial ECBs
Due to its design; which consists of a braking disc and a magnetic field source, axial ECBs are sometimes referred to as ECB discs. Its magnetic field can either be produced using a coil or a permanent magnet. Depending on their structure, axial ECBs are either (a) single or (b) dual magnetic field-powered (). Smythe (1943) was the first to examine the use of eddy currents in rotating discs for braking (Karakoc et al., Citation2012).
Figure 14. Eddy current brake axial: (a) single magnetic-field source (Yaojing et al., Citation2017) and (b) dual magnetic field source (Ye et al., Citation2010).

According to existing studies, the conductor or disc of an axial ECB is responsible for dissipating the generated heat (Anwar & Stevenson, Citation2011). Liu and Zhang Yan (Citation2010) (Anwar & Stevenson, Citation2011) used a testbed to examine the heat generation of axial ECBs. The increase in heat was also calculated. However, one of the limitations of the study was that it temporarily stopped the current flowing in the electromagnet to examine the heat generation phenomenon when the temperature began to rise significantly. Nevertheless, despite a lack of studies, axial ECBs exhibit acceptable heat dissipation capacity.
A single rotor structure consists of a disc brake and a magnetic field source that face each other. As the magnetic field travels in an anti-parallel path, it only affects one side of the conductor disc (). The magnetic field of a single rotor structure is produced using an electromagnet or a permanent magnet.
Figure 15. Axial eddy current brake single rotor (Gulec et al., Citation2016).

Permanent magnet ECBs rely on permanent magnets as their magnetic field source. The braking force can be set to a constant speed by adjusting the width of the air gap, which is the distance between the permanent magnet and the inductor plate. At present, no attempts have been made to develop permanent magnet axial ECBs. The electromagnetic magnetic source of an ECB comprises several magnetic poles, which are generated directly using a current-carrying winding. The magnetic field source is often permanently attached opposite the conducting plate (). Cho et al. (Citation2017) was the first to examine a single stator, in which an FEA was conducted to determine ECB performance. A mathematical analysis method (Albertz et al., Citation1996) was then developed in 1985 to simplify this process. Since then, multiple studies have attempted to increase braking torque by adjusting the thickness of the conductor, hybrid excitation and AC.
In an ECB system, the conductor receives a magnetic field and produces eddy currents. Gulbahce et al. (Citation2013) (P. J. Wang & Chiueh, Citation1998) examined the effect of thickness on braking torque. An increase in thickness was found to decrease braking torque and critical speed. The maximum torque generated at a disc thickness of 1–1.5 cm was reasonable. This decreased by almost a third when the thickness increased to 2 cm. However, as these results were obtained using theoretical analysis, experimental studies must be conducted to verify them.
Braking capacity can also be enhanced by increasing the strength of the magnetic field. The fixed capacity of a permanent magnet depends on its material and its strength, which can no longer be increased. Conducting coils, on the other hand, have limited electric current generating capacity. However, the limitations of the inductance material due to surface forces restrict the main affected area of the magnetic field, which generates the eddy current. Furthermore, enlarging the outer diameter size will affect the size of the bearing, shaft and other sites. Disc flexibility also affects the design. Apart from outlining design parameters, braking torque can also be increased using modifications. The degree of braking torque is directly proportional to the magnetic flux density of the air gap. As such, permanent magnets can be used as an additional magnetic field to increase braking torque. For instance, Yazdanpanah and Mirsalim (Citation2015a) used two magnetic field sources simultaneously. Permanent magnets were also used as the core of the winding ().
Figure 17. Inserted permanent magnet coil (Yazdanpanah & Mirsalim, Citation2015a).

As single stator ECBs have poor braking performance, they have not been extensively developed. However, the low torque that they generate can be combined with a regenerative braking system to create an alternative option (Yazdanpanah & Mirsalim, Citation2015b). The simultaneous use of two braking systems could also increase energy usage efficiency. Furthermore, as a regenerative braking system can fulfil the electrical requirements of an ECB braking system, it may reduce reliance on a battery to power the braking process.
The braking performance of ECBs can also be enhanced by increasing the density of the magnetic field in the air gap. This can be accomplished using two magnetic field sources in pairs (Lu et al., Citation2014). Induction discs receive magnetic field sources from both sides, which increase the density of the magnetic field and affects inductance. Therefore, single or separate magnetic field sources can be used to generate the two magnetic field poles (). Monopolar ECBs usually have a single magnetic field source, where the magnetic flux is generated by a pair of magnetic poles. The winding of the magnetic field can be set to either monopolar or bipolar. A monopolar setting is commonly used if the source of the magnetic field is a U profile winding. Wu et al. (Citation2013) examined a monopolar ECB with magnetic fields produced solely by a coil. The passing magnetic flux was found to travel along a single path while the magnetic field formed a loop. Meanwhile, a bipolar ECB uses two magnetic fields. In a study of bipolar magnetic fields, A. Singh (Citation1977) used separate magnetic field sources for both sides of the winding core.
Figure 18. Axial eddy current brake (a) single excitation (L. Luo et al., Citation2017) and (b) separate excitation (Kou et al., Citation2014a).

According to Zhou et al. (Citation2015) (Kou et al., Citation2014a), the location of the magnetic poles from the centre of the disc does not affect braking torque. Increasing the distance of the magnetic pole from the centre point of the disc only yielded a marginal increase in braking torque. However, the cross-sectional shape of the magnetic pole does affect braking torque as it carries a magnetic flux. (Liu & Zhang Yan, Citation2010) investigated the orientation of magnetic poles arranged in a square shape, with the poles arranged transversely and longitudinally relative to the motion of the conducting disc. At the same magnetic field strength, the longitudinal arrangement was found to produce 1.3 times more torque than the transverse arrangement.
Apart from the parameters of the magnetic pole; which produces the magnetic field; the conductor also affects ECB performance as it produces the induced current required for braking. Schieber (Citation1972) examined thin inductors while A. Singh (Citation1977) investigated thick discs. Thick discs were found to affect braking torque at high speeds as the skin effect reduces the amount of braking torque produced by a thick inductor. Meanwhile, Ying et al. (Citation2010) (Jiao et al., Citation2014) concluded that the braking force increases as the thickness increases and continues to increase until the optimal thickness is achieved. The type of disc material also affects braking performance. Venkataratnam and Raju (Citation1977) examined the use of non-magnetic materials in discs while Baharom et al. (Citation2012) (Worley, Citation2015) concluded that aluminium containing certain elements affect the braking process.
The braking torque of an ECB increases as the rotational speed increases. Therefore, it will only provide marginal braking torque at low speeds. As induced electricity is produced by a change in the magnetic field, a low rotational speed will reduce changes in the magnetic field and produce minimal current. Therefore, a specific frequency of alternating electricity can be used to increase braking performance at low speeds. Karakoc et al. (Citation2012) (Monzón-Verona et al., Citation2019) theoretically examined using AC electricity in the ECB braking process (). The use of AC electricity at a frequency of not more than 20 Hz was found to produce more braking torque than direct current (DC) electricity. However, increasing the frequency of the AC electricity to 50 Hz produced less than half of the braking torque of DC electricity. A few years later, Karakoc et al. (Citation2016) (Ye et al., Citation2010) performed a modelling analysis to investigate the issue further.
In an axial ECB, the heat source is centred at the bottom of the magnetic field source. Apart from the process of converting energy from motion to heat; ie the braking process; the heat generated by the magnetic field source also plays a part. Furthermore, the generated heat tends to travel towards the centre or central part of the disc.
Several studies have used mathematical models to examine heat generation and storage. provides a list of equations from extant studies on ECB heat generation. The findings of Monzon-Verona et al. are of particular interest as they report that the higher the heff, the easier it is to dissipate the heat generated in the disc and lower the temperature.
Table 2. Equations from previous studies on heat generation.
6. Future opportunity
The heat generated during the braking process should be taken into consideration when using an ECB system. Adding grooves to the surface of an ECB conductor has been found to help the cooling process. They also improve the braking performance and cooling process of axial ECBs. Cooling techniques generally involve air- or water-based cooling via forced convection or other methods. However, not many studies have looked into ECB cooling systems and the heat they produce or created effective ECB cooling techniques. Therefore, there is a need to further analyse and study the heat generated by the ECBs as well as optimal ECB cooling methods. This present review endeavors to provide a comprehensive review of the aforementioned topics in the hopes that future studies may use it as reference material. The heat generated by ECBs significantly increases the temperature, damages the insulation, deforms the structure, and demagnetises the magnet. As radial ECBs have a flexible design and are quite large, there is a greater degree of flexibility in the choice of cooling system. Liquid-based cooling systems are the most effective for this type of ECB system. At present, the priority is to cool the exterior of a radial ECB, as it also acts as the conductor. However, the present configuration is incapable of dissipating the heat trapped within the radial ECB. Furthermore, there is still a lot of potential of further developing the cooling configuration; such as adjusting the settings so that the dimensions can be more compact. On the other hand, are commonly found in vehicles that run on rails; such as locomotives. However, unlike axial and radial ECBs, which experience repeated contact during braking, linear ECBs do not require much cooling, as its conductor does not experience repeated contact during braking. Nevertheless, linear ECBs can be further improved by adding a cooling element to the magnetic field source. This is necessary as linear ECBs are commonly used in heavy vehicles at high speeds. Meanwhile, the advantages of an axial ECB include a relatively compact design and more contact area with the outside air. However, as the rotor component serves as the conductor in this type of ECB, it is difficult to add a liquid cooling system to its surface. Therefore, air-cooling is the most suitable method of cooling. Air-cooling can be divided into several forms; such as changing or adding grooves or dimples to the surface of the conductor ().
7. Discussion
The purpose of developing ECB design is to create an exceptional braking system, but this depends on several factors. At present, ECBs are viewed as a support system that can be integrated into existing braking systems or as an alternative to conventional braking systems. However, they are not without their advantages and disadvantages. For instance, the heat that ECBs generate during the braking process is of particular concern. Therefore, an effective cooling system will significantly aid the delivery of good braking performance. One of the advantages of the axial ECB design is that its conductor effectively dissipates heat. This is because the design has a large contact area that exposes the conductor to air. Even still, axial ECBs can be further improved by using a good control system to regulate the current usage of its electromagnets. This would enable the duration of current flowing to the electromagnet to be adjusted, which will, in turn, ensure that the conductor has sufficient time to dissipate heat. As for radial ECBs, liquid cooling has proven to be more effective than air-cooling. This differs for the permanent magnets in a linear ECB, as the heat generated does not exceed 45 °C. This is acceptable as permanent magnets are highly sensitive to increases in temperature.
Acknowledgments
Indicate names of individuals, institutions or programmes (if any) who have made important (especially financial) contributions for authors’ related study and research.
Disclosure statement
No potential conflict of interest was reported by the author(s).
Additional information
Funding
Notes on contributors
M. R. A Putra
Mufti Reza Aulia Putra, currently completing his doctoral degree at Sebelas Maret University, specializes in researching energy conversion processes related to electric vehicle (EV) technology. With a focus on EV development, he has authored 19 publications in respected indexed journals and conference proceedings, along with several Intellectual Property Rights (IPR) related to EV advancement. For further communication, Mufti Reza can be reached via email at [email protected].
Ubaidillah
Ubaidillah holds the position of a professor at Universitas Sebelas Maret. His academic journey culminated in the completion of his doctoral studies at Universiti Teknologi Malaysia. Possessing a keen interest in research, Prof. Ubaidillah’s expertise extends across various fields, encompassing Functional Materials, Mechanical Design, Vehicle Dynamics, Acoustic Material, as well as the Mitigation of Noise and Vibration. He actively contributes to the advancement of knowledge and innovation within these realms, facilitating significant research outcomes. Those seeking further communication with Prof. Ubaidillah can reach him via email at [email protected].
D. D. D. P. Tjahjana
D. Danardono Dwi P. T., currently a professor at Universitas Sebelas Maret, obtained his Ph.D. in Mechanical Engineering from Chonnam National University, South Korea. His areas of expertise lie within Wind Energy and Computational Fluid Dynamics (CFD), where his research aims to drive forward sustainable energy solutions and deepen our understanding of fluid dynamics phenomena. Prof. Danardono is actively engaged in academic research, contributing significantly to the field. Those interested in contacting him can do so via email at [email protected] or [email protected].
M. Nizam
Muhammad Nizam, a professor at Sebelas Maret University, was born in Surakarta on July 20, 1970. He earned his bachelor’s and master’s degrees in electrical engineering from Gadjah Mada University, Yogyakarta, in 1994 and 2002 respectively. He then pursued his doctoral studies at Universiti Kebangsaan Malaysia, graduating in the same field from 2005 to 2008, followed by post-doctoral research until 2009 at the same institution. His research interests encompass electric power systems, energy management systems, power quality, electric vehicles, and energy storage. Actively engaged in professional associations, he is a member of PII, IEEE, and the Power and Energy Society. Currently, Professor Muhammad Nizam holds the role of Priority Coordinator for National Research on Battery Products, and serves as the Secretary of the UNS Center for Excellence in Electrical Energy Storage Technology. With over 110 publications in esteemed indexed journals and conference proceedings, he also holds several patents and Intellectual Property Rights (IPRs) in the field of batteries and electric vehicles. For communication purposes, Professor Muhammad Nizam can be reached via email at [email protected].
Z. Arifin
Zainal Arifin, currently serves as a professor at Universitas Sebelas Maret. He obtained his Ph.D. in Mechanical Engineering from Universitas Brawijaya. With a focus on Solar Cell technology, Nanomaterials, and Manufacturing processes, Prof. Zainal Arifin actively contributes to advancements in these areas through his research endeavors. For further communication, he can be reached via email at [email protected].
H. T. Waloyo
Hery Tri Waluyo, a renowned academic, has earned degrees across all academic levels in Mechanical Engineering from Universitas Sebelas Maret. His educational path encompasses accomplishments in doctoral, master’s, and undergraduate studies. Passionate about Renewable Energy, Manufacturing Processes, and Design Processes, Hery Tri Waluyo is deeply involved in research and academic endeavors in these fields. His commitment to advancing knowledge and fostering innovation is reflected in his scholarly works. For communication purposes, he can be reached via email at [email protected].
Bhre Wangsa Lenggana
Bhre Wangsa Lenggana was a graduate of the master’s program at Universitas Sebelas Maret, Surakarta, Indonesia. Currently he is continuing his doctoral program in the mechanical engineering. His research is related to smart materials such as magnetorheological fluids and magnetorheological elastomers in various applications. Apart from that, his research is also related to structure and is currently conducting preliminary research on metamaterials. He can be contacted at email: [email protected]
References
- Abutu, J., Lawal, S. A., Ndaliman, M. B., Lafia-Araga, R. A., Adedipe, O., & Choudhury, I. A. (2018). Effects of process parameters on the properties of brake pad developed from seashell as reinforcement material using grey relational analysis. Engineering Science and Technology, an International Journal, 21(4), 787–797. https://doi.org/10.1016/j.jestch.2018.05.014
- Albertz, D., Dappen, S., & Henneberger, G. (1996). Calculation of the 3d non-linear eddy current field in moving conductors and its application to braking systems. IEEE Transactions on Magnetics, 32(3), 768–771. https://doi.org/10.1109/20.497353
- Anantha Krishna, G. L., & Sathish Kumar, K. M. (2014). Investigation on eddy current braking systems – a review. Applied Mechanics and Materials, 592-594, 1089–1093. https://doi.org/10.4028/www.scientific.net/AMM.592-594.1089
- Anon. (2000). ICE3 pioneers commercial application of eddy-current rail brakes. Railway Gazette International, 156(9), 583–585.
- Anwar, S., & Stevenson, R. C. (2011). Torque characteristics analysis for optimal design of a copper-layered eddy current brake system. International Journal of Automotive Technology, 12(4), 497–502. https://doi.org/10.1007/s12239−011−0058−0
- Bae, J.-N., Kim, Y.-E., Son, Y.-W., Moon, H.-S., Yoo, C.-H., & Lee, J. (2015). Self-excited induction generator as an auxiliary brake for heavy vehicles and its analog controller. IEEE Transactions on Industrial Electronics, 62(5), 3091–3100. https://doi.org/10.1109/TIE.2014.2379218
- Bagnoli, F., Dolce, F., & Bernabei, M. (2009). Thermal fatigue cracks of fire fighting vehicles gray iron brake discs. Engineering Failure Analysis, 16(1), 152–163. https://doi.org/10.1016/j.engfailanal.2008.01.009
- Baharom, M. Z., Nuawi, M. Z., Priyandoko, G., & Harris, S. M. (2012). Eddy current braking experiment using brake disc from aluminium series of a16061 and a17075. IOP Conference Series: Materials Science and Engineering, 36, 012005. https://doi.org/10.1088/1757-899X/36/1/012005
- Bigeon, J., & Sabonnadiere, J. C. (1985). Analysis of an electromagnetic brake. Electric Machines & Power Systems, 10(4), 285–297. https://doi.org/10.1080/07313568508909128
- Bigeon, J., Sabonnadiere, J. C., & Coulomb, J. l (1983). Finite element analysis of an electromagnetic brake. IEEE Transactions on Magnetics, 19(6), 2632–2634. https://doi.org/10.1109/TMAG.1983.1062848
- Boniardi, M., D’Errico, F., Tagliabue, C., Gotti, G., & Perricone, G. (2006). Failure analysis of a motorcycle brake disc. Engineering Failure Analysis. 13(6), 933–945. https://doi.org/10.1016/j.engfailanal.2005.04.012
- Cho, S., Jeong, T., Bae, J., Yoo, C., & Lee, J. (2017). Analysis and performance of the self-excited eddy current brake. Journal of Electrical Engineering and Technology. 12(1), 459–465. https://doi.org/10.5370/JEET.2017.12.1.459
- Cho, S., Liu, H., Ahn, H. W., Lee, J., & Lee, H.-W. (2017). Eddy current brake with a two-layer structure: Calculation and characterization of braking performance. IEEE Transactions on Magnetics, 53(11), 1–5. https://doi.org/10.1109/TMAG.2017.2707555
- Cho, S., Liu, H.-C., Lee, J., Lee, C.-M., Go, S.-C., Ham, S.-H., Woo, J.-H., & Lee, H.-W. (2017). Design and analysis of the eddy current brake with the winding change. Journal of Magnetics, 22(1), 23–28. https://doi.org/10.4283/JMAG.2017.22.1.023
- Choi, J.-Y., & Jang, S.-M. (2012). Analytical magnetic torque calculations and experimental testing of radial flux permanent magnet-type eddy current brakes. Journal of Applied Physics, 111(7), 07E712. https://doi.org/10.1063/1.3672408
- Collignon, M., Cristol, A. L., Dufrénoy, P., Desplanques, Y., & Balloy, D. (2013). Failure of truck brake discs: A coupled numerical-experimental approach to identifying critical thermomechanical loadings. Tribology International, 59, 114–120. https://doi.org/10.1016/j.triboint.2012.01.001
- Day, A. J., & Newcomb, T. P. (1984). Dissipation of frictional energy from the interface of an annular disc brake. Proceedings of the Institution of Mechanical Engineers, Part D: Transport Engineering, 198(3), 201–209. https://doi.org/10.1243/PIME_PROC_1984_198_146_02
- Dubos, N., Varin, B., & Bisson, O. (2016). A better knowledge of powered two wheelers’ accidents. Transportation Research Procedia, 14, 2274–2283. https://doi.org/10.1016/j.trpro.2016.05.243
- Edwards, J. D., Jayawant, B. V., Dawson, W. R. C., & Wright, D. T. (1999). Permanent-magnet linear eddy-current brake with a non-magnetic reaction plate. IEE Proceedings - Electric Power Applications, 146(6), 627. https://doi.org/10.1049/ip-epa:19990574
- Effect of regenerative braking energy on battery current balance in a parallel hybrid gasoline-electric vehicle under ftp-75 driving mode. (2016). International Journal of Automotive Technology, 17(5), 865–872. https://doi.org/10.1007/s12239-016-0084-z
- Garbiec, T., Kowol, M., & Kołodziej, J. (2014). Design considerations of high-speed eddy-current brake. Archives of Electrical Engineering, 63(2), 295–304. https://doi.org/10.2478/aee-2014-0022
- Ghaleh, S., Omidvari, M., Nassiri, P., Momeni, M., & Mohammadreza Miri Lavasani, S. (2019). Pattern of safety risk assessment in road fleet transportation of hazardous materials (oil materials). Safety Science, 116(February), 1–12. https://doi.org/10.1016/j.ssci.2019.02.039
- Gosline, A. H. C., & Hayward, V. (2008). Eddy current brakes for haptic interfaces: Design, identification, and control. IEEE/ASME Transactions on Mechatronics, 13(6), 669–677. https://doi.org/10.1109/TMECH.2008.2004623
- Gulbahce, M. O., Kocabas, D. A., & Atalay, A. K. (2013). Determination of the effect of conductive disk thickness on braking torque for a low power eddy current brake. In 4th International Conference on Power Engineering, Energy and Electrical Drives, Istanbul, Turkey (pp. 1272–1276). IEEE. https://doi.org/10.1109/PowerEng.2013.6635795
- Gulec, M., & Aydin, M. (2016). Modelling and analysis of a new axial flux permanent magnet biased eddy current brake. In 22nd International Conference on Electrical Machines (ICEM) (pp. 459–465). Lausanne, Switzerland. https://doi.org/10.1109/ICELMACH.2016.7732566
- Gulec, M., Yolacan, E., & Aydin, M. (2016). Design, analysis and real time dynamic torque control of single-rotor–single-stator axial flux eddy current brake. IET Electric Power Applications. 10(9), 869–876. https://doi.org/10.1049/iet-epa.2016.0022
- Haas, S,. (2008, June). Eddy-current braking: A long road to success. Railway Gazette International, 2(1), 1–5.
- Hu, D., Yan, Y., & Xu, X. (2019). Energy saving optimal design and control of electromagnetic brake on passenger car. Mechanical Sciences, 10(1), 57–70. https://doi.org/10.5194/ms-10-57-2019
- Jang, S.-M., Jeong, S.-S., & Cha, S.-D. (2001). The application of linear Halbach array to eddy current rail brake system. IEEE Transactions on Magnetics, 37(4), 2627–2629. https://doi.org/10.1109/20.951256
- Jang, S.-M., Lee, S.-H., & Jeong, S.-S. (2002). Characteristic analysis of eddy-current brake system using the linear Halbach array. IEEE Transactions on Magnetics, 38(5), 2994–2996. https://doi.org/10.1109/TMAG.2002.803191
- Jee, I. H., Nahm, S. Y., Kang, S. J., & Ryu, K.-S. (2012). A magnetic brake for small wind turbines. Journal of Magnetics, 17(1), 33–35. https://doi.org/10.4283/JMAG.2012.17.1.033
- Jiao, B., Li, D., Du, X., & Zhang, K. (2014). Performance analysis and experimentation of a liquid-cooled eddy current retarder with a dual salient poles design. IEEE Transactions on Energy Conversion, 29(1), 84–90. https://doi.org/10.1109/TEC.2013.2288695
- Jin, Y., Kou, B., Zhang, L., & Zhang, H. (2016). Magnetic and thermal analysis of a Halbach permanent magnet eddy current brake. In 19th International Conference on Electrical Machines and Systems (ICEMS). Chiba, Japan, 2016, pp. 1–4.
- Kamla, J., Parry, T., & Dawson, A. (2019). Analysing truck harsh braking incidents to study roundabout accident risk. Accident; Analysis and Prevention, 122, 365–377. https://doi.org/10.1016/j.aap.2018.04.031
- Karakoc, K., Park, E. J., & Suleman, A. (2012). Improved braking torque generation capacity of an eddy current brake with time varying magnetic fields: A numerical study. Finite Elements in Analysis and Design, 59, 66–75. https://doi.org/10.1016/j.finel.2012.05.005
- Karakoc, K., Suleman, A., & Park, E. J. (2016). Analytical modelling of eddy current brakes with the application of time varying magnetic fields. Applied Mathematical Modelling, 40(2), 1168–1179. https://doi.org/10.1016/j.apm.2015.07.006
- Kou, B., Jin, Y., Zhang, H., Zhang, L., & Zhang, H. (2014a). Characteristic analysis of a novel series magnetic path hybrid excitation linear eddy current brake. In 17th International Conference on Electrical System (ICEMS). Hangzhou, China, 2014, pp. 1719–1722. https://doi.org/10.1109/ICEMS.2014.7013757
- Kou, B., Jin, Y., Zhang, H., Zhang, L., & Zhang, H. (2014b). Modelling and analysis of force characteristics for hybrid excitation linear eddy current brake. IEEE Transactions on Magnetics, 50(11), 1–5. https://doi.org/10.1109/TMAG.2014.2323334
- Lesobre, A., Ben Ahmed, A. H., & Drecq, D. (2001). An analytical dynamic model of eddy-current brakes. In IEEE International Electric Machines and Drives Conference (Cat. No.01EX485). Cambridge, MA, USA, 2001, pp. 122–125. https://doi.org/10.1109/IEMDC.2001.939285.
- Li, P., Ma, J., & Fang, Y. (2011). Design and analysis of hybrid excitation rail eddy current brake system of high-speed train. In Proceedings of 2011 IEEE International Conference on Service Operations and Logistics Informatics (pp. 565–569), Beijing, China. IEEE. https://doi.org/10.1109/SOLI.2011.5986624
- Liu, C., & Zhang Yan, J. K. (2010). Study on temperature rise performance of eddy current retarder in automobile. In 2010 International Conference on Future Information Technology and Management Engineering (pp. 550–553). IEEE. https://doi.org/10.1109/FITME.2010.5655807
- Lu, X., Li, Y., Wu, M., Zuo, J., & Hu, W. (2014). Rail temperature rise characteristics caused by linear eddy current brake of high-speed train. Journal of Traffic and Transportation Engineering (English Edition), 1(6), 448–456. https://doi.org/10.1016/S2095-7564(15)30295-6
- Lubin, T., & Rezzoug, A. (2015). 3-d analytical model for axial-flux eddy-current couplings and brakes under steady-state conditions. IEEE Transactions on Magnetics, 51(10), 1–12. https://doi.org/10.1109/TMAG.2015.2455955
- Luo, L., Zhai, Q., Li, W., Qian, C., & Liu, H. (2017). Research on an integrated electromagnetic auxiliary disc brake device for motor vehicle. IEEJ Transactions on Electrical and Electronic Engineering, 12(3), 434–439. https://doi.org/10.1002/tee.22395
- Luo, N., Jiang, J., & Yu, A. (2014). Research on the control strategy of the regenerative braking system. In 2014 International Conference on Mechatronics and Control (pp. 2514–2517). IEEE. https://doi.org/10.1109/ICMC.2014.7232021
- Ma, J., Zhang, B., Huang, X., Fang, Y., & Cao, W. (2011). Design and analysis of the hybrid excitation rail eddy brake system of high-speed trains. Journal of Zhejiang University-SCIENCE A, 12(12), 936–944. https://doi.org/10.1631/jzus.A11GT002
- Ma, X., Fan, S., Sun, H., Luan, C., Deng, J., Zhang, L., & Cheng, L. (2020). Investigation on braking performance and wear mechanism of full-carbon/ceramic braking pairs. Tribology International. 142(September 2019), 105981.https://doi.org/10.1016/j.triboint.2019
- Mackin, T. J., Noe, S. C., Ball, K. J., Bedell, B. C., Bim-Merle, D. P., Bingaman, M. C., Bomleny, D. M., Chemlir, G. J., Clayton, D. B., Evans, H. A., Gau, R., Hart, J. L., Karney, J. S., Kiple, B. P., Kaluga, R. C., Kung, P., Law, A. K., Lim, D., Merema, R. C., … Zimmerman, R. S. (2002). Thermal cracking in disc brakes. Engineering Failure Analysis. 9(1), 63–76. https://doi.org/10.1016/S1350-6307(00)00037-6
- Mazzù, A., Provezza, L., Zani, N., Petrogalli, C., Ghidini, A., & Faccoli, M. (2019). Effect of shoe braking on wear and fatigue damage of various railway wheel steels for high-speed applications. Wear, 434-435, 203005. https://doi.org/10.1016/j.wear.2019.203005
- Monzón-Verona, J. M., González-Domínguez, P. I., García-Alonso, S., Santana-Martín, F. J., & Cárdenes-Martín, J. F. (2019). Thermal analysis of a magnetic brake using infrared techniques and 3D cell method with a new convective constitutive matrix. Sensors, 19(9), 2028. https://doi.org/10.3390/s19092028
- Nehl, T. W., Lequesne, B., Gangla, V., Gutkowski, S. A., Robinson, M. J., & Sebastian, T. (1994). Nonlinear two-dimensional finite element modelling of permanent magnet eddy current couplings and brakes. IEEE Transactions on Magnetics, 30(5), 3000–3003. https://doi.org/10.1109/20.312568
- Obara, T., Kumagai, N., & Takiguchi, T. (1995). Development of hybrid rail brake. Proceedings of the Institution of Mechanical Engineers, Part F: Journal of Rail and Rapid Transit, 209(2), 61–65. https://doi.org/10.1243/PIME_PROC_1995_209_257_02
- Park, M.-G., Choi, J.-Y., Shin, H.-J., & Jang, S.-M. (2014). Torque analysis and measurements of a permanent magnet type eddy current brake with a Halbach magnet array based on analytical magnetic field calculations. Journal of Applied Physics, 115(17), 17E707. https://doi.org/10.1063/1.4862523
- Pawar, N. M., Khanuja, R. K., Choudhary, P., & Velaga, N. R. (2020). Modelling braking behaviour and accident probability of drivers under increasing time pressure conditions. Accident; Analysis and Prevention, 136, 105401. https://doi.org/10.1016/j.aap.2019.105401
- Rainey, D., Parenteau, M. A., & Kales, S. N. (2019). Sleep and transportation safety: Role of the employer. Sleep Medicine Clinics, 14(4), 499–508. https://doi.org/10.1016/j.jsmc.2019.08.007
- Reyes, A. M., Dela Cruz, C. J., Diaz, L. J., & Olegario, E. M. (2019). Microstructure evaluation of the damage and wear characteristics of a failed disc brake of a provincial bus. Materials Today: Proceedings, 16, 1789–1795. https://doi.org/10.1016/j.matpr.2019.06.052
- Ryoo, H.-J., Kim, J.-S., Kang, D.-H., Rim, G.-H., Kim, Y.-J., & Won, C.-Y. (2000). Design and analysis of an eddy current brake for a high-speed railway train with constant torque control. In Conference Record of the 2000 IEEE Industry Applications Conference. Thirty-Fifth IAS Annual Meeting and World Conference on Industrial Applications of Electrical Energy (Cat. No.00CH37129), Rome, Italy. pp. 277–281 vol.1. https://doi.org/10.1109/IAS.2000.881123
- Schieber, D. (1972). Unipolar induction braking of thin metal sheets. Proceedings of the Institution of Electrical Engineers, 119(10), 1499. https://doi.org/10.1049/piee.1972.0296
- Sharif, S., Faiz, J., & Sharif, K. (2012). Performance analysis of a cylindrical eddy current brake. IET Electric Power Applications, 6(9), 661. https://doi.org/10.1049/iet-epa.2012.0006
- Sharif, S., & Sharif, K. (2009). Influence of skin effect on torque of cylindrical eddy current brake. In 2009 International Conference on Power Engineering, Energy and Electrical Drives, Lisbon, Portugal (pp. 535–539). IEEE. https://doi.org/10.1109/POWERENG.2009.4915143
- Singh, A. (1977). Theory of eddy-current brakes with thick rotating disc. Proceedings of the Institution of Electrical Engineers, 124(4), 373. https://doi.org/10.1049/piee.1977.0067
- Singh, A. K., & Ibraheem, N. (2015). Model development of eddy current brakes for energy absorbing system. In 2015 International Conference on Recent Developments in Control, Automation and Power Engineering (RDCAPE). Noida, India. pp. 382–384. https://doi.org/10.1109/RDCAPE.2015.7281429
- Singh, A. K., & Sharma, A. K. (2014). Parameter identification of eddy current braking system for various applications. In 2014 Innovative Applications of Computational Intelligence on Power, Energy and Controls with Their Impact of Humanity (pp. 191–195). IEEE. https://doi.org/10.1109/CIPECH.2014.7019113
- Sinmaz, A., Gulbahce, M. O., & Kocabas, D. A. (2015). Design and finite element analysis of a radial-flux salient-pole eddy current brake. In 2015 9th International Conference on Electrical and Electronics Engineering (pp. 590–594). IEEE. https://doi.org/10.1109/ELECO.2015.7394612
- Smythe, W. R. (1942). On eddy currents in a rotating disk. Electrical Engineering, 61(9), 681–684. https://doi.org/10.1109/EE.1942.6436528
- Thompson, M. T. (2009). Practical issues in the use of NdFeB permanent magnets in maglev, motors, bearings, and eddy current brakes. In Proceedings of the IEEE (vol. 97, no. 11, pp. 1758–1767). https://doi.org/10.1109/JPROC.2009.2030231
- Tian, J., Li, D., Ning, K., & Ye, L. (2020). A timesaving transient magneto-thermal coupling model for the eddy current brake. IEEE Transactions on Vehicular Technology, 69(10), 10832–10841. https://doi.org/10.1109/TVT.2020.3013342
- Venkataratnam, K., & Raju, D. R. (1977). Analysis of eddy-current brakes with nonmagnetic rotors. Proceedings of the Institution of Electrical Engineers, 124(1), 67. https://doi.org/10.1049/piee.1977.0010
- Waloyo, H., Ubaidillah, U., Tjahjana, D. D. D. P., Nizam, M., & Koga, T. (2019). Mini review on the design of axial type eddy current braking technology. International Journal of Power Electronics and Drive Systems (IJPEDS), 10(4), 2198. https://doi.org/10.11591/ijpeds.v10.i4.pp2198-2205
- Wang, K., He, R., Tang, J., & Liu, R. (2020). Design and thermal analysis of a novel permanent magnet-friction integrated brake for vehicle. Thermal Science, 24(3 Part A), 1827–1834. https://doi.org/10.2298/TSCI190609070W
- Wang, P. J., & Chiueh, S. J. (1998). Analysis of eddy-current brakes for high-speed railway. IEEE Transactions on Magnetics, 34(4), 1237–1239. https://doi.org/10.1109/20.706507
- Wang, Z., Han, J., Domblesky, J. P., Li, Z., Fan, X., & Liu, X. (2019). Crack propagation and microstructural transformation on the friction surface of a high-speed railway brake disc. Wear, 428-429(February), 45–54. https://doi.org/10.1016/j.wear.2019.01.124
- Worley, P. (2015). Heating water with wind or hydro power: Direct conversion of mechanical energy to heat using eddy currents. Ohio State University.
- Wu, M. L., Zhang, X. J., Tian, C., & Lu, X. S. (2013). Simulation and analysis of rail eddy current brake system of high-speed train. Advanced Materials Research, 774-776, 207–211. https://doi.org/10.4028/www.scientific.net/AMR.774-776.207
- Yaojing, F., Xiangshuai, X., & Shoudao, H. (2017). Design research of high-power oil-cooled electrical eddy current retarder for heavy vehicles. In 2016,19th International Conference on Electrical Machines and Systems (ICEMS), Chiba, Japan, pp. 1–5.
- Yazdanpanah, R., & Mirsalim, M. (2015a). Analytical study of axial-flux hybrid excitation eddy current brakes. International Journal of Applied Electromagnetics and Mechanics, 47(4), 885–896. https://doi.org/10.3233/JAE-140019
- Yazdanpanah, R., & Mirsalim, M. (2015b). Hybrid electromagnetic brakes: Design and performance evaluation. IEEE Transactions on Energy Conversion, 30(1), 60–69. https://doi.org/10.1109/TEC.2014.2333777
- Ye, L., Li, D., & Jiao, B. (2010). Three-dimensional electromagnetic analysis and design of permanent magnet retarder. Frontiers of Mechanical Engineering in China, 5(4), 438–441. https://doi.org/10.1007/s11465-010-0111-0
- Ying, Z., Xu, X., & Zhu, J. (2010). Analysis of simulation design of the disc eddy current braking device. In 2010 International Conference on Computer, Mechatronics, Control and Electronic Engineering. Hindawi. 2015, 1–10. https://doi.org/10.1155/2015/436721
- Zhang, P., Zhang, L., Fu, K., Wu, P., Cao, J., Shijia, C., & Qu, X. (2019). The effect of al2o3 fibre additive on braking performance of copper-based brake pads utilized in high-speed railway train. Tribology International, 135, 444–456. https://doi.org/10.1016/j.triboint.2019.03.034
- Zhang, Z., Shi, L., & Li, Y. (2013). Characteristic analysis of linear eddy-current brake used in high-acceleration system. In 2013 International Conference on Electrical Machines and Systems (ICEMS), Busan, Korea (South), pp. 1971–1974. https://doi.org/10.1109/ICEMS.2013.6713229
- Zhou, Q., Guo, X., Tan, G., Shen, X., Ye, Y., & Wang, Z. (2015). Parameter analysis on torque stabilization for the eddy current brake: A developed model, simulation, and sensitive analysis. Mathematical Problems in Engineering, 2015, 1–10. https://doi.org/10.1155/2015/436721
- Zou, N., Wang, Q., Wang, W., & Zeng, X. (2009). Simulation study of CVT ratio control for engine braking. In 2009 International Conference on Measuring Technology and Mechatronics Automation (pp. 367–370). IEEE. https://doi.org/10.1109/ICMTMA.2009.541