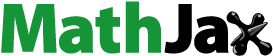
Abstract
This article presents the experimental results on the effects of surface functionalization of plantain pseudo-stem fiber (PF) and sugar cane fiber (SF) sorbents on the absorption of crude oil and the separation oil/water mixture. The modification involved coating the SF and PF with TiO2, graphene oxide (GO), and stearic acid (SA). The fibers were cut and sonicated in hexane and ethanol in succession for ∼45 min and dried at 60 °C for 24 h. 0.5 g of PF and SF were bagged into 2 g empty tea bags. The GO and ethanol solutions of ∼3–5 mg/mL were coated onto the SF and PF and dried for 12 h and subsequently contacted with instant ocean salt and crude oil fractions. The results show that the SA, TiO2 nanoparticles, and GO sheets contribute a large surface area and high surface roughness which provide excellent hydrophobicity. The modified SF and PF recorded ∼144° and ∼126° from ∼63° and ∼46° contact angles as well as ∼10.29 g/g and ∼5.77 g/g absorption respectively. The sorbent materials demonstrate crude oil and oil/water separation indicating a promising technique for oil spill remediation.
1. Introduction
Oil spills have deleterious effects on the environment, especially the marine ecosystem (Abidli et al., Citation2020; Chu et al., Citation2015; Garcia et al., Citation2020; Niu et al., Citation2021; Turco et al., Citation2015; Wang et al., Citation2015; Wang et al., Citation2015). It is estimated that ∼120 million gallons of crude oil and its refined products are spilled into the ocean annually (Peterson et al., Citation2003; Wu et al., Citation2021).
Several strategies have been implemented to address crude oil spills including ultrasonics, chemical dispersants, in-situ combustion, skimming, and vacuuming. However, these existing approaches present several challenges, including substantial energy use, resource, and time requirements. Moreover, they frequently demonstrate high toxicity and often fall short in terms of efficiency (Abidli et al., Citation2020; Garcia et al., Citation2020; Huang et al., Citation2020; Sadatshojaie et al., Citation2021; Torres et al., Citation2020).
Therefore, the need for enhanced technologies with reduced toxicity, cheap, rapid response, superior performance, and reusability in response to oil spills to mitigate the environmental impacts cannot be overemphasized (ben Hammouda et al., Citation2021; Dhaka & Chattopadhyay, Citation2021; Ghafoori et al., Citation2022; Gupta & Tai, Citation2016; Hoang et al., Citation2021). Photocatalysts and their modified forms have shown great promise in tackling oil spill and have been reported in prior studies by Agyei-Tuffour et al. (Citation2020), Shi et al. (Citation2021) and Zoghi and Allahyari (Citation2022), environmentally friendly dispersants by Adofo et al. (Citation2022), Nyankson, DeCuir, et al. (Citation2015), Nyankson, Olasehinde, et al. (Citation2015), and Shah et al. (Citation2020). Photothermal materials development for highly viscous crude oil clean up (Chang et al., Citation2018; Ku et al., Citation2021; Niu et al., Citation2021; Wu et al., Citation2021) and the use of enhanced sorbent materials (ben Hammouda et al., Citation2021; Idris et al., Citation2014; Songsaeng et al., Citation2019; Vocciante et al., Citation2019; Yen Tan et al., Citation2021). The development of environmentally friendly and low-cost oil sorbent materials with high absorption capacity and hydrophobicity (Yen Tan et al., Citation2021) for oil spill remediation (Ku et al., Citation2021; Laitinen et al., Citation2017; Wang et al., Citation2015) is currently attracting significant attention in research endeavors.
These researches point to the effectiveness of sorbents (Bhardwaj & Bhaskarwar, Citation2018; Mardiyati et al., Citation2022; Yurak et al., Citation2021; Zamparas et al., Citation2020) in recovering liquids, particularly crude oil, employing a sorption mechanism (Bazargan et al., Citation2015; Hoang et al., Citation2021; Yurak et al., Citation2021).
Both natural and synthetic sorbents have been researched (Nyankson, Rodene, et al., Citation2015; Yurak et al., Citation2021) with preferences for natural fibers since they are less expensive, easier to handle, have high mechanical qualities, use less energy during production, and are biodegradable (Ahmad et al., Citation2015; Dhaka & Chattopadhyay, Citation2021; Dittenber & GangaRao, Citation2012; Hoang et al., Citation2021; Mojžiš et al., Citation2019; Rangappa & Siengchin, Citation2018; Singh et al., Citation2020; Zamparas et al., Citation2020). In addition, the handling of these fibrous materials in their natural state does not change their chemical constitution (Holbery & Houston, Citation2006). They provide fast absorption of oil through a mechanism that enables them to fill the pores within them (Ku et al., Citation2021).
Natural sorbents can be obtained from various plant components, including the stems, leaves, seeds, or fruits of plants (Dey & Haripavan, Citation2023; Loh et al., Citation2013; Narayanasamy et al., Citation2022). Examples such as cotton fibers, corn stalks, and non-woven wool have been employed as sorbent materials in the past (Deschamps et al., Citation2003; Devarshi et al., Citation2023; Kovačević et al., Citation2023; Santos et al., Citation2021; Yang et al., Citation2022) (Husseien et al., Citation2009). Sugar cane fiber (SF) and plantain pseudo-stem fiber (PF) are some other cheap examples of natural fibers obtained from waste (Danso, Citation2021; Juela et al., Citation2021). They contain lignocellulosic residues, exhibiting significant potential for utilization as sorbents (Danso, Citation2021; Juela et al., Citation2021). Sugar cane fiber is primarily made up of 50–70% SiO2 (Faisal & Muhammad, Citation2016; Prabhath et al., Citation2023; Sholeh et al., Citation2021). The inherent combination of silica and lignin in the sugar cane fiber makes it a good candidate for sorption activities (Mardiyati et al., Citation2022). Given the sterling qualities of the sugar cane and plantain pseudo-stem fibers, they can be employed as typical porous substrates to fabricate modified sorbents (Huda et al., Citation2008; Loh et al., Citation2016; Loh et al., Citation2013; Zhou et al., Citation2021) for crude oil spill remediation.
Standardization of sorbent materials, as outlined by ASTM F726-12, categorizes them into four types. Bagged sugar cane and plantain pseudo-stem fibers fall into the Type III category, characterized by enclosed sorbents in outer permeable fabric or netting resembling pillows or booms (Bazargan et al., Citation2015).
In developing sorbent materials for oil spill remediation, high selectivity, sorption capacity, easy collection and recyclability of the materials is critical (Singh et al., Citation2020; Turco et al., Citation2015). Functionalization using TiO2, graphene oxide (GO), and stearic acid (SA) as agents can enhance the intrinsic hydrophobicity of the otherwise hydrophilic natural sorbent materials (Iliyasu et al., Citation2022; Ma et al., Citation2016; Ramesh et al., Citation2022; Zhou et al., Citation2021) and make them good candidates for oil spill remediation.
The excellent TiO2 photocatalyst combined with hydrophilic GO (Cote et al., Citation2010; de Oliveira et al., Citation2022; Ganesh et al., Citation2013; Nguyen et al., Citation2019; Some et al., Citation2013; Zhang et al., Citation2022) can increase the surface roughness of the material, therefore, providing it with special wettability (Baig et al., Citation2019; Liu et al., Citation2021; Zhou et al., Citation2021).
Stearic acid (SA) is a low-cost saturated fatty acid in the human body and therefore has no physical toxicity and is capable of enhancing the hydrophobicity of a material (Ma et al., Citation2016; Patti et al., Citation2021; Satria & Saleh, Citation2022; Zhou et al., Citation2021). Again, it is employed widely in research regarding medicine preparation (Galvão et al., Citation2020; Huang et al., Citation2008; Karnnet et al., Citation2005; Ma et al., Citation2016; Yang et al., Citation2020).
In this study, natural sorbent materials made up of sugar cane (SF) and plantain pseudo-stem (PF) fibers were loaded into tea bags to fabricate a viable type III sorbent material. The materials employed in this fabrication were inexpensive, common, biodegradable, and for that matter environmentally friendly, and supported proper utilization of waste.
The modification of the fabricated sorbent was accomplished through a low-cost, environmentally friendly, and easy-to-perform method of dip coating making use of the solution of ethanol, TiO2, SA, and GO. The effects of the individual and combined surface modifiers on the SF and PF fibers and their oil uptake capacity have been presented. The potential of the modified SF and PF sorbent materials as an economical and environmentally benign approach toward oil spill remediation was demonstrated.
Thus, this research may open a new avenue for exploring bagged SF and PF sorbents as robust viable, economically, oleophilic, hydrophobic, and environmentally friendly sorbents for cleaning up oil spills.
2. Materials and methods
2.1. Materials
Sugar cane fiber (SF) and Plantain Pseudo-stem (PF) were obtained locally. Graphene oxide (GO), Stearic acid (SA), hexane, ethanol, and TiO2 nanoparticles were purchased from Sigma-Aldrich, UK. All chemicals were analytical grade Deionized (DI) water, produced from an Elga water purification system (Medica DV25), with a resistance of 18.2 MΩ/cm was used in all experiments. Agbami Crude oil from Nigeria with a density of 889.9 kg/m3, and an American Petroleum Institute (API) gravity of 27.03 at 15 °C was obtained from Tema Oil Refinery (TOR), Tema, Ghana. 2 g Yellow-label Lipton tea bags were obtained from the market. Petrol and diesel were obtained from Shell Ghana. Shell Helix HX 3 20W-50 Multi-Grade Motor Oil for diesel and gasoline engines was obtained from Shell Ghana.
2.1.1. Preparation of SF and PF sorbent materials
The SF and PF sorbent materials were prepared to the type III standard of sorbent materials (Bazargan et al., Citation2015). The plantain stem fibre was obtained from the stem of the plantain tree while the sugar cane fibre was also obtained from the stalk of the sugar cane. They were washed with water and sun-dried. The fibers were rewashed using hexane and ethanol in succession. Hexane was added first and agitated with a shaker for 45 min After drawing off the hexane, ethanol was added and agitated for another 45 min before pouring off. The residue was then dried in an oven at 60 °C for 24 h.
0.5 g of smooth fibers of the plantain and sugar cane were bagged into new empty 2 g yellow label Lipton tea bags to form the SF and PF sorbent materials.
2.1.2. Modification of the SF and PF sorbent materials
GO and ethanol solution were prepared separately to different concentrations of 3, 4, and 5 mg/ml subjected to an ultrasonic treatment with a sonicator for 30 min. Then, 100 mg or a variable amount (150, 200, and 250 mg) of TiO2 and 400 mg or a variable amount (200, 300, 500, and 600 mg) of stearic acid were added to 4 mg/ml GO-ethanol solution and subjected to further ultrasonic treatment for another 30 min. Subsequently, the SF and PF sorbent materials were immersed into the GO-ethanol, SA, and TiO2 solution for 30 min of ultrasonic treatment. They were then continuously immersed in the solution for 24 h before the products were taken out. Finally, the samples were dried at 40 °C for 12 h in an oven.
The following acronyms/abbreviations were adopted along the manuscript to represent different substances as they appear in . Specifically, “T” stands for TiO2, “G” for GO (Graphene Oxide), and "S" for SA (Stearic Acid). A combination of abbreviations and numbers has been used to label various samples of SF and PF sorbents used in this study. Each label consists of abbreviated letters prefixed with numbers indicating the quantity of each substance used for the surface modification. To make it more user-friendly, the numbers have been downscaled by a factor of 100 (e.g. “×100 mg”).
Figure 1. (a–d) Water contact angle (WCA) and time for water droplets to linger on the surface of the modified and unmodified PF sorbents and (e and f) water contact angle (WCA) and time for the water droplets to linger on the surface of the SF and its modified form showing the optimization of the chemicals used in the modification process. 4 mg/mL (2) of GO (G) and 400 mg (4) of stearic acid (SA), and 100 mg (1) of TiO2 (T) were used.

Figure 2. (a). Uptake/absorption capacities of the SF and PF modified and unmodified sorbents for some oils (b) uptake capacity/absorption capacity of the unmodified and modified SF sorbents in a crude oil/seawater environment, (c and d) recyclability of the modified PF and SF sorbents respectively for 5 cycles making use of crude oil.

Figure 3. Schematic diagram of the sorption mechanism of the bagged sorbents (a) at the initial stage and (b) at the final stage.

Figure 4. Microscopic images of (a) packed fibers in the tea bag, (b) the surface of the unmodified bagged sorbent (c) the surface of the modified bagged sorbent, (d) the modified fibers’ interaction with crude oil, (e) unmodified sorbent interaction with water and (f) modified sorbent’s interaction with crude oil.

For example, the sample labeled “4S2G1T/SF” represents a sorbent material formed by dip coating SF in a mixture of SA, GO, and TiO2 in the proportions of 400 mg (4):200 mg (2):100 mg (1) respectively in 50 mL of ethanol.
2.1.3. Preparation of the synthetic sea salt
Synthetic seawater was prepared by dissolving 35 g of instant ocean salt obtained from Instant Ocean (Blacksburg, VA) in 1 L of distilled water. The mixture was stirred continuously for 24 h at room temperature to ensure complete dissolution of the salt particles.
2.2. Characterization
2.2.1. Fourier transform infra-red (FTIR) spectroscopy
A Vertex 70 v (Bruker) Fourier Transform Infrared (FTIR) spectrometer was used to record the FTIR spectra in the transmission mode of the modified and unmodified PF and SF sorbent materials. The spectra were carried out within the wavenumber range of 4000–400 cm−1 with 4 cm−1 resolution. The data was analyzed using the Opus software.
2.2.2. Scanning electron microscopy-energy dispersive X-ray spectroscopy (SEM-EDS)
The SEM image analysis used the Phenom-World ProX desktop scanning electron microscope. Optical images were captured at the lowest magnification of 20×. Backscattered images were captured at different magnifications (minimum to maximum) using an image intensity and high-resolution voltage mode of 10 kV using the backscatter detector until the best image focusing ends. Further, using the Phenom ProSuite software (element identification), EDS point analysis at 15 KV, duration of 30 s, and map analysis at 15 KV, duration of 4 min 26 s were used for the elemental identification, distribution, and concentration respectively.
2.2.3. X-ray fluorescence (XRF) spectroscopy analysis
The XRF analysis was carried out using the Rigaku NEX CG desktop XRF which analyzes from 11Na to 92U non-destructively. Four grams (4 g) of the sample was weighed in a sample cup and subjected to X-ray fluorescence to obtain major oxides and elemental constituents using the fundamental parameters method double determination approach analysis. It was conducted in an atmosphere of Helium at a flow rate of 0.660 L/min for powder samples, tube voltage = 50 kV, and tube current = 1.00 mA.
2.2.4. Thermogravimetric analysis (TGA)
The TA Instruments SDT 2960 Simultaneous DTA-TGA was employed for thermogravimetric analysis (TGA) of the tea bags, plantain pseudo-stem fiber (PF), sugar cane fiber (SF), unmodified SF and PF sorbents (UMSF and UMPF respectively), and modified SF and PF sorbents (MSF and MPF respectively). The analysis was carried out in an air environment, using a 10 °C/min heating rate.
2.2.5. Mechanical testing of the properties of unmodified and modified tea bag
The mechanical testing of the tensile properties of the modified and unmodified sorbents bags were done using the universal testing machine, Mark 10 at 10 min mm−1.
2.2.6. Static contact angle measurement
The water static contact angle (WCA) and oil static contact angle were measured by dropping a deionized water droplet or oil droplet from a micropipette as an indicator on the sponge at room temperature and pictures were taken using iPhone 11 camera and calculated with ImageJ 1.53t from Wayne Rashband and contributors, National Institute of Health, USA (http://imagej.nih.gov/ij.Java1.8.0_345) in conjunction with Drop analysis plugin. Three measurements with different positions on sponges were performed per sample and the average was calculated.
2.2.7. Water repellency experiment
The sorbent materials were entirely immersed in a 250 mL beaker with 150 mL water by applying an external force which was removed after 2 min. The modified sorbent materials floated on the surface of the water whereas the unmodified sorbent materials were submerged beneath the surface of the water.
2.2.8. Hydrophobicity and oleophilicity test
The hydrophobic/oleophilic properties of unmodified and modified sorbent materials were tested by placing the crude oil and water droplets on the top surface of both the modified and unmodified sorbent materials. The water droplets stayed on the surface of the modified material for some time whereas the crude oil droplets were absorbed almost immediately. On the other hand, both the water and crude oil droplets were almost immediately absorbed when exposed to the surface of the unmodified sorbent materials.
2.3. Absorption studies
In the oil sorption tests crude oil (CO), petrol (P), diesel (D), hexane (H), and Shell Helix HX 3 20W-50 Multi-Grade Motor Oil were used. The initial weight of the unmodified and modified sorbent materials before being immersed in the crude oil was recorded as M1. After contact with the oils, the sorbent materials became quickly saturated after 30 min. After, they were allowed a dripping time of 10 min. The final weight was then recorded as M2. The oil sorption capacity/uptake capacities of the sorbents were calculated and estimated using the following equation:
(1)
(1)
2.4. Crude oil/water separation capacity
An attempt was made to examine the crude oil/water separation capacity of the modified and unmodified SF and PF sorbent materials. A 250 mL beaker was filled with a mixture of 50/50 v/v of crude oil and synthetic seawater. The initial weights of the sorbent materials were recorded and then they were placed in the mixture for a contact time of 24 h. After this, they were taken out of the mixture and permitted a dripping time of one (1) minute before their respective final weights were measured. Their absorption capacities were calculated using EquationEquation (1)(1)
(1) . The absorbed crude oil was recovered by squeezing it out of the sorbent materials.
2.5. Reusability
The test of the absorption-desorption properties of the sorbents was modeled after the method used by Bayraktaroglu et al. (Citation2021). It was used for the reusability experiments conducted in crude oil. First, the sorbents were contacted with the crude oil for 30 min around which they reached their maximum absorbency. After, they were allowed to release the absorbed liquids under gravity for one hour. This process was repeated 5 times.
3. Results and discussions
3.1. Surface morphological analysis of SF and PF sorbents
The surface characteristics of both the sorbent materials were examined using SEM/EDX analysis, with the outcomes depicted in . The fibrous materials, namely SF and PF, utilized in the fabrication of the sorbents exhibited a smooth surface, as evident in , along with the accompanying microscopy image and the SEM image of the bagged surface in and , respectively. Furthermore, the SEM image of the SF revealed the presence of pores in the structure, as depicted in . Subsequently, following the modification process illustrated in , the porous skeleton structure of the fibrous materials remained predominantly unchanged. However, a few pores were discreetly coated by the modifying materials, as observed in comparison to the SEM image in . Notably, the modified surfaces () also exhibited increased roughness in comparison to their untreated counterparts (). This increase in roughness serves as a tangible indicator of the incorporation of surface-modifying materials, namely SA, GO, and TiO2, both on the surface ( and within the bulk of the sorbent materials, as illustrated in and . This transformation is further corroborated by microscopy images of the surface of the sorbent materials in .
Figure 5. (a–d) SEM-EDS of unmodified and modified surfaces of the bagged sorbent material respectively, (e–h) SEM-EDS of unmodified and modified surfaces of the plantain pseudo-stem fiber respectively, and (i–l) SEM-EDS of the unmodified and modified surfaces of the sugar cane fibers respectively.

Figure 6. (a and b) Microscopy images of unmodified and modified surfaces of the sorbent material respectively.

The presence of these surface-modifying materials, SA, GO, and TiO2, was again confirmed through EDS analysis, both on the surface and within the fibers of the sorbent materials, as evidenced in by the presence of Ti in the peaks as compared the unmodified surfaces shown in which showed no Ti. This analysis underscores the efficacy of the dip coating method in successfully modifying the SF and PF sorbent materials.
The impact of the modification on the pores of the fibers of sorbent materials manifested in a slight reduction in their sorption capacities, a phenomenon illustrated in . Moreover, the efficacy of the modification process has been sufficiently demonstrated by the notable increase in the Water Contact Angle (WCA) of the modified sorbents as seen in , , and .
Figure 7. (a–d) Photographic images of the water droplets on the surfaces of the unmodified SF, modified SF, unmodified PF, and unmodified PF sorbent materials respectively, and (e–h) Photographic images of crude oil droplets on the surfaces of the unmodified SF, modified SF, unmodified PF, and unmodified PF sorbent materials respectively.

3.2. Chemical and structural analyses of S.F and P.F
FT-IR was used to investigate the TiO2, GO, and SA and how they interact with the sorbent materials. The investigation on the interaction was undertaken on the surface of the bagged modified and unmodified sorbents as well as on the fibers within the bags. The results are presented in .
Figure 9. a–c. FTIR of a. unmodified and modified surfaces of the bagged PF and SF sorbent materials, b. the unmodified and modified PF and SF fibers in the bagged sorbent materials and c. SA, TiO2 and GO.

The FTIR spectra of the SA () exhibit major peaks at 2919 cm−1 and 2848 cm−1, corresponding to the asymmetric and symmetric stretching vibrations of the –CH2– band, respectively (Chen et al., Citation2011; He et al., Citation2019; Yang et al., Citation2011). The peak at 1701 cm−1 is also associated with –COOH (Li et al., Citation2017; Sood et al., Citation2022; Zhu et al., Citation2016). Concerning the GO () the characteristic peaks (GO) spanning 400–900 cm−1, 2847 – 3721 cm−1, and the peaks at 1638 cm−1 and 1056 cm−1 can be assigned to O–H, C–H, C = C stretching, and C–O, respectively (Andrijanto et al., Citation2016; Faniyi et al., Citation2019; Khalili, Citation2016; Wang et al., Citation2011; Zhu et al., Citation2010). In addition, in the FTIR spectrum of TiO2 (), the broad peak at 3500 cm−1 is indicative of the hydroxyl group O–H (Mamaghani et al., Citation2018; Zhong et al., Citation2022), while Choi et al. (Citation2004) also ascribe Ti–O bond to the wavenumbers between 600 cm−1 and 800 cm−1 (León et al., Citation2017; Mahmoudi et al., Citation2024; Mihaly Cozmuta et al., Citation2015; Mugundan et al., Citation2015).
The FTIR analyses conducted on both the unmodified fibers within the bagged sorbent materials and the surface of the bagged sorbent materials revealed striking similarities ( and ). This uniformity suggests that all components involved are derived from plant-based materials, underscoring their shared environmentally friendly nature. The spectral bands at 432, 525, 556, and 860 cm−1 are indicative of the C–H showing the presence of lignin (Scheufele et al., Citation2015). The results show that typical strong bands of the fibers are attributed to O–H stretching intramolecular hydrogen bonds for cellulose at 3330 cm−1, C–H stretching at 2852–2919 cm−1, C–O stretching vibration for the acetyl and ester linkages in lignin, hemicellulose, pectin at 1727 cm−1 and the aromatic ring present in lignin and absorbed water at 1603 cm−1 which are all associated with the SF and PF before the surface treatments (Ahmad et al., Citation2018; Kumar et al., Citation2020; Melesse et al., Citation2022; Zaheer et al., Citation2014).
After the surface modification/functionalization with SA, GO, and TiO2, the spectral bands observed in the unmodified SF and PF sorbent materials were seen to be altered in the FTIR spectrum ( and ). The effect of this surface modification can be observed through the main spectral bands. The spectral bands at 432, 525, 556, and 860 cm−1 indicate Ti-O stretching vibration for TiO2 with 1603 cm−1 and 3330 cm−1 indicating O–H. There were significant alterations seen between the unmodified PF and modified PF sorbent materials both internally with the fibers and externally on the surface of the bagged sorbent materials suggesting hydrogen bonds between GO and TiO2. It was observed that the bands in the modified fibers as well as the surfaces were reduced significantly which can be attributed to the introduction of the SA, GO, and TiO2 during the modification process.
Considering the unmodified SF and modified SF fibers, the spectral band at 2852–2919 cm−1 indicating the C–H bond reduced drastically and the band at 3330 cm−1 indicating O–H increased in the modified SF, suggesting an interaction between C–H and O–H of SA and GO respectively as compared to the unmodified. These alterations observed in the modified PF and SF fibers and the sorbent material surfaces could be attributed to the interaction between the GO, SA, TiO2, and the sorbent material and the fibers contained in it. Zhou and co-workers present a similar interaction between the SA, TiO2, and the GO with the melamine sponge in their work (Zhou et al., Citation2021). The above results show that the surface modification of the sorbent material was successful and confirmed by the SEM/EDX results in .
3.3. Compositional analysis of the SF and PF
In , we present the chemical composition of PF and SF, as assessed via X-ray fluorescence (XRF) analysis. Notably, SF stands out with a significantly elevated SiO2 content, registering at 17.35, in contrast to the PF's SiO2 content of 10.2. This SiO2 component holds significant importance in the material’s absorption capacity, playing a pivotal role in its oil-absorbing capabilities (Filipović et al., Citation2010; Kamgar & Hassanajili, Citation2020; Lu et al., Citation2007; Mazrouei-Sebdani et al., Citation2019).
Table 1. Chemical Composition of the SF and PF.
The inherent silica content with lignin found in sugar cane and plantain fibers enhances its oil-absorption capabilities (Mardiyati et al., Citation2022). The GO combines with the silica in the fibers to create a strong affinity for oil absorption (Ben Hammouda et al., Citation2021). It creates a strong adhesion between the sorbents and the oil. This phenomenon elucidates why the sorbent material composed of sugar cane fibers demonstrates superior absorption performance in comparison to that composed of plantain fibers as presented in .
3.4. Mechanical properties of tea bag, PF and SF fibers, modified PF and SF sorbents
Thermogravimetric analysis (TGA) was utilized to assess the thermal stability, moisture absorption, and decomposition characteristics of the tea bags, plantain pseudo-stem fiber (PF), sugar cane fiber (SF), unmodified SF and PF sorbents (UMSF and UMPF, respectively), and modified SF and PF sorbents (MSF and MPF, respectively).
Asim et al. (Citation2020) outline the thermal degradation of natural fibers typically occurs in three stages. First, there is the loss of moisture through evaporation, a stage in which chemically treated or modified fibers generally exhibit lower moisture content. This is primarily because the modified sorbents become resistant to moisture hence the reduction in moisture contents (Aravindh et al., Citation2022; Gholampour & Ozbakkaloglu, Citation2020). The second stage involves the decomposition of cellulose and hemicellulose, occurring within the temperature range of 220–350 °C. The third stage is attributed to the decomposition of non-cellulosic substances such as lignin, spanning a broader temperature range of 200–500 °C (Hossain et al., Citation2014).
and generally adhere to this degradation pattern, albeit with slight variations. The initial mass loss occurs within the temperature range of 10–110 °C for all analyzed samples, with mass losses ranging from 6% to 8%. The second stage of degradation takes place within the temperature range of 180–390 °C, accompanied by corresponding mass losses ranging from 2% to 8%. Notably, the modified sorbents (MSF and MPF) exhibit lower mass losses compared to the unmodified sorbents (UMSF and UMPF) in this stage.
Figure 10. (a) TGA and (b) thermographic analysis for derivatives of mass min−1 versus temperature for the tea bag, plantain pseudo-stem fiber (PF), sugar cane fiber (SF), unmodified SF and PF sorbents (UMSF and UMPF, respectively), and modified SF and PF sorbents (MSF and MPF, respectively).

The third degradation stage occurs within the temperature range of 410–480 °C. Additionally, a fourth stage of degradation is observed around the temperature range of 600–650 °C which may be due to further decomposition of the remaining non-cellulosic content.
It is observed that the modified sorbents are more thermally stable than the fibers or the bags alone. Researchers agree that this observation may be due absence or reduction in hemicellulose and lignin content of the modified sorbents (Aravindh et al., Citation2022; Gholampour & Ozbakkaloglu, Citation2020; Hossain et al., Citation2014). These findings provide insights into the thermal behavior and decomposition kinetics of the investigated sorbents, shedding light on their suitability for oil spill remediation applications.
3.5. Mechanical properties of unmodified and modified tea bag
The mechanical properties of the tea bag must be outlined since the durability and utilization of the sorbent material are dependent on it. Given this, the tensile strength and strain as well as Young’s modulus were determined.
Using the data from and EquationEquations (1)–(3) the tensile strength, strain, and Young’s modulus of the unmodified and modified tea bags were calculated and presented in .
(1)
(1)
(2)
(2)
(3)
(3)
Table 2. Parameters used in the calculation of the tensile properties of the unmodified and modified tea bag.
Table 3. Tensile properties of the unmodified and modified tea bag.
From it is observed that the tensile properties of the unmodified tea bags increased upon their modification. An increase in strain means the introduction of ductility into the tea bags after the modification. In effect, the chemical modification with TiO2, SA, and GO resulted in an improvement in the mechanical properties. This improvement is due to the removal or a reduction in the hemicellulose and lignin content (Aravindh et al., Citation2022; Gholampour & Ozbakkaloglu, Citation2020). Similar observations were made by researchers upon the treatment of fibers with other chemicals (Aravindh et al., Citation2022; Gholampour & Ozbakkaloglu, Citation2020; Hossain et al., Citation2014).
3.6. Oleophilicity and hydrophobicity of SF and PF sorbent materials
To study the crude oil/water separation ability, the crude oil and water droplets were dropped separately onto the modified and unmodified sorbent materials. The crude oil was absorbed immediately by both the modified and unmodified materials in a matter of seconds exhibiting their oleophilic nature. In contrast, water droplets exhibited prolonged retention on the surface of the modified sorbents before permeating them while the unmodified ones rapidly absorbed them within a matter of seconds (refer to and ). The above observations show that the unmodified SF and PF sorbent materials were hydrophilic and oleophilic while the modified materials became hydrophobic and oleophilic. This means the surface modification using SA, GO, and TiO2 was able to make the otherwise hydrophilic materials hydrophobic.
Again, the fact that the water droplets could be observed in spherical shapes on the surface of the modified sorbent materials while the crude oil was absorbed into the matrix of the sorbent materials confirms the porous nature of the sorbent materials. Other researchers also noted a similar observation when working with both untreated and treated cotton, as well as treated and untreated melamine sponges (Deschamps et al., Citation2003; Zhou et al., Citation2021).
3.7. Water repellency
Water repellency is a crucial property for absorbent or sorbent materials, particularly in the context of oil spill remediation (Rather et al., Citation2017; Wang et al., Citation2019). To test this property, the modified and unmodified sorbent materials were entirely immersed in water in a beaker by applying an external force. After the external force was removed, the modified sorbent materials instantaneously floated on the top of the water surface without any surrounding water droplets adhering to the surface, exhibiting water repellency. However, the unmodified sorbent material floated beneath the surface of the water showing it is not able to repel water. The above-observed water repellency of the modified sorbents points to their hydrophobic nature.
3.8. Surface wettability
In determining the surface wettability, Zhou et al. (Citation2021) made use of surface water static contact angle (WCA) as an important factor to determine the wettability and oil absorption selectivity of the modified sorbent materials. The photographic images of the water droplets on the surface of the SA, GO, and TiO2 modified and unmodified natural sugar cane fibers (SF) and the plantain pseudo-stem fiber (PF) sorbent materials have been presented in .
The calculated water contact angles are presented in and and , , and . Notably, the water contact angle increased from an intrinsic value of 63°–144° and 46°–126° for the modified SF (refer to ) and PF (refer to and ) sorbent materials respectively indicating the introduction of a significant hydrophobic property. This agrees with what Law (Citation2014, Citation2015), Hebbar et al. (Citation2017), and co-workers have postulated that a water contact angle between 90° and 150° suggests hydrophobicity whereas above 150° suggests superhydrophobicity. Given the foregoing, the evidence points to the fact that the hydrophilic natural sorbent materials through surface modification using TiO2, GO, and SA were made hydrophobic.
Through the surface modification, the TiO2 nanoparticles and GO (i.e. 2G1T/SF) introduced surface roughness, which enhanced the hydrophobicity of the modified sorbent materials as seen in . The water contact angle recorded was 136.71° and it took the water droplet on the surface of the SF-modified material 6180 s to be absorbed into the sorbate. This was slightly lower than the modification using SA alone (i.e. 4S/SF) which recorded an increase in the intrinsic water contact angle from 62.86° to 140.84° for the 4S/SF () suggesting an introduction of hydrophobicity into the otherwise hydrophilic SF material even though it took 1260 s () for a water droplet to stay on the surface before being absorbed. It can be inferred from the preceding that the addition of stearic acid largely contributes to the hydrophobicity of the modified sorbent material. An observation that is in agreement with what Patti et al. (Citation2021) and Satria and Saleh (Citation2022) and co-workers have opined.
The hydrophilic characteristics of GO (2G/SF) were confirmed, even with a noticeable rise in the intrinsic water contact angle of the SF sorbent material from 62.86° to 82.59° (refer to ). It’s noteworthy that the angle remains below 90°, the threshold for hydrophobicity. This observation emphasizes the water affinity resulting from the modification with GO. Again, the hydrophilicity of the GO, as evidenced in the (2G/SF) modified sorbent material, is further shown by the fact that it took only 2 s for a water droplet to stay on the surface of the sorbent material before being absorbed (). Other studies (Cote et al., Citation2010; Ganesh et al., Citation2013; Some et al., Citation2013) have confirmed the hydrophilicity of the GO. This means the GO contributed to the reduction in the water contact angle of the 4S2G/SF compared to the 4S/SF. In effect, the GO’s presence in the SA and GO combination might have reduced the hydrophobicity of the SA, hence the reduced contact angle recorded by the 4S2G/SF in .
Furthermore, by taking advantage of the long hydrophobic CH2 chains of stearic acid and the surface roughness of the duo, TiO2, and the GO, the combination of the TiO2, GO, and SA and the SF sorbent materials (i.e. 4S2G1T/SF) exhibited superior surface hydrophobicity. It recorded a water contact angle of 144.04° () and the time it took the water droplet to stay on the surface of the sorbent material was 12,600 s (). As alluded to earlier, the GO and TiO2 increase the surface roughness, while the SA introduces hydrophobicity into the sorbent material. Therefore, the combination of these three chemicals in the surface modification of hydrophilic SF and PF sorbent materials made them hydrophobic and thus a suitable candidate for the separation of oil/water mixtures, hence efficient for oil spill remediation. This result is corroborated by Zhou et al. (Citation2021).
and a–f present the durations water droplets lingered on both modified and unmodified sorbent material surfaces before permeating into them and their water contact angles. To optimize contact angle and water droplet staying time on the modified sorbent materials, the compositions of stearic acid (SA), TiO2, and graphene oxide (GO) were systematically varied. Examined samples included 2S2G1T/SF, 3S2G1T/SF, 4S2G1T/SF, 5S2G1T/SF, 6S2G1T/SF for modified sugar cane fibers (SF), and 2S2G1T/PF, 3S2G1T/PF, 4S2G1T/PF, 5S2G1T/PF, 6S2G1T/PF for plantain pseudo-stem fibers (PF). indicate 4S2G1T/PF reached a peak contact angle of 126.31° with a droplet staying 7335 s (). Furthermore, 6S2G1T/PF sustained a droplet for 8100 s permeating it () but achieved a lower contact angle of 103.6° ().
Remarkably, and d, which show that the TiO2 was varied, reveal that 4S2G1T/SF exhibited a contact angle of 144.04° () and sustained a water droplet on the surface for an extended 12,600 s (), signifying its optimal performance.
Focusing on and , GO variations were explored alongside steady SA (400 mg) and TiO2 (100 mg). Notably, 4S2G1T/PF achieved the highest contact angle of 126° () from plantain pseudo-stem fiber samples (4S1.5G1T/PF, 4S2G1T/PF, and 4S2.5G1T/PF) and maintained water droplet on its surface for 7335 s (). Similarly, sugar cane fiber samples (4S1.5G1T/SF, 4S2G1T/SF, 4S2.5G1T/SF) mirrored these trends, revealing that 4S2G1T/SF yielded optimal results, with a contact angle of 139° () and sustaining water droplet on its surface for 10,500 seconds (). In addition, varying the amounts of TiO2 and keeping the amounts of SA and GO constant (refer ) also shows that the 4S2G1T/SF had the highest water contact angle and longest water retention time on the surface of the sorbent materials of 144° and 12,600 s, respectively.
Both 4S2G1T/SF and 4S2G1T/PF demonstrated peak performance. Between the PF and SF sorbents, SF materials exhibited superior results. This may be attributed to the fact that the high silica content of the sugar cane combined well with the surface-modifying materials used to enhance its intrinsic hydrophobicity.
Again, the static contact angle was utilized to assess the wettability characteristics of oil on both modified and unmodified sorbents of SF and PF, with the results depicted in . All samples exhibited contact angles in the range of 34°–52°, which are less than 90°, indicating their oleophilic nature. Zamparas et al. (Citation2020) even though the modification process altered the contact angles, it was observed that the modified sorbents showed a slight increase in contact angles compared to their unmodified counterparts. This difference may contribute to the higher oil sorption capacity of the unmodified sorbents compared to their modified counterparts (), as they possess a greater oleophilic tendency.
Table 4. Static contact angles of crude oil at 1 s on the unmodified and modified sorbent surfaces.
Similarly, both unmodified and modified SF demonstrated slightly lower contact angles compared to their PF counterparts, which could explain why SF sorbs more oil than PF (). The above observations demonstrate the success of the modification procedure in converting the natural-based SF and PF sorbents into hydrophobic-oleophilic materials.
3.9. Uptake capacity of and PF unmodified and modified sorbents
The investigation into the sorption/uptake capacities of both modified and unmodified sorbents (SF, PF, 4S2G1T/SF, and 4S2G1T/PF) concerning crude oil, diesel, petrol, hexane, and motor oil yielded interesting findings.
The unmodified SF sorbents exhibited significant sorption capacities for crude oil, petrol, diesel, hexane, and motor oil, with values of 11.43 ± 0.57, 6.67 ± 0.33, 9.30 ± 0.47, 6.37 ± 0.32, and 9.58 ± 0.48 g g−1, respectively, compared to their PF counterparts, which demonstrated values of 6.40 ± 0.32, 2.97 ± 0.15, 4.76 ± 0.24, 2.32 ± 0.12, and 7.22 ± 0.36 g g−1, as depicted in .
Similarly, the modified SF sorbents displayed enhanced sorption capacities for crude oil, petrol, diesel, hexane, and motor oil, with values of 10.29 ± 0.51, 6.02 ± 0.30, 8.38 ± 0.42, 5.87 ± 0.29, and 8.62 ± 0.43 g g−1, respectively, compared to their PF counterparts, which showed values of 5.77 ± 0.29, 2.70 ± 0.14, 4.56 ± 0.23, 2.10 ± 0.11, and 6.72 ± 0.34 g g−1, respectively.
These findings underscore the efficacy of both unmodified and modified SF sorbents in absorbing various types of hydrocarbons, highlighting their potential utility in oil spill remediation and related applications.
Despite this, it was observed that the sorption capacities of the modified sorbents recorded slightly reduced values compared to the unmodified sorbents, which stands contrary to most findings on the modification of natural-based sorbents (Zamparas et al., Citation2020). This may be due to the surface-modifying materials that appear to occupy pore spaces within the modified PF and SF sorbent matrix, as highlighted in . Consequently, the modification materials potentially hinder the pore accessibility to a limited extent, thereby affecting the uptake/absorption capacities of the modified materials. This observation is consistent with the findings of other researchers (Bae & Choi, Citation2003; Deschamps et al., Citation2003).
These observations collectively highlight the relationship between the surface modification method used in this study and sorption performance, shedding light on the intricate mechanisms that influence material porosity and sorbent functionality. Further investigation would seem appropriate to precisely elucidate the factors contributing to this observed behavior and to refine our understanding of the interrelationship between material modifications and sorption dynamics.
3.10. Oil/water separation capacity
To better assess the practical effectiveness of the sorbent materials, we conducted a comprehensive examination of both their unmodified and modified forms in terms of their oil/water separation efficacy, as depicted in . These experiments, conducted with a 24-h contact time and a 1-min dripping time, offer valuable insights into their performance.
In the context of crude oil/seawater mixtures, the modified sorbent (4S2G1T/SF) exhibited the capacity to absorb crude oil effectively. Notably, the unmodified SF sorbent displayed a higher oil absorption rate than the modified SF variants. This disparity could potentially stem from the inherent tendency of SF to absorb some water along with the crude oil. Zhou et al. (Citation2021) point to a similar observation in their work.
Moreover, the reduced oil absorption observed in the modified SF materials can be attributed to the presence of SA, GO, and TiO2, which potentially fill the matrix pores that would otherwise, accommodate crude oil. The filling of the matrix pores by the modifying materials (SA, GO, and TiO2) was observed in the SEM images in . An observation Deschamps et al. (Citation2003) and Choi and Cloud (1992) have also noted.
After saturation with crude oil, the sorbent materials (4S2G1T/SF and SF) could readily release the absorbed crude oil by connecting them to a pump capable of extracting the crude oil from them (Jiang et al., Citation2016; Niu et al., Citation2021; Wang et al., Citation2019), highlighting their practical applicability in recovering the absorbed oil. This ease of recovery adds to the attractiveness of these materials for real-world oil spill cleanup scenarios.
Once the sorbent material comes into contact with the oil particles, they are initially attracted to the surface of the sorbent material through adsorption (), utilizing intermolecular forces such as London–van der Waals forces and hydrogen bonding (Ponec et al., Citation2018; Tran et al., Citation2017; Wang & Hou, Citation2024).
After 30 min, the oil particles then migrate to the surfaces of the fibers within the enclosed sorbent material, where they are adsorbed, and then penetrate the pores of the fibers through absorption (). The pores of the fibers are penetrated by capillary action (Zamparas et al., Citation2020). In effect, the oil particles interact with the sorbent material through the adsorption-absorption mechanism.
The surface morphological comparison between the unmodified and the modified sorbents before and after interacting with crude oil is shown in . The surface of the sorbents exhibits a porous network of interconnected structures (), allowing the crude oil to be both adsorbed on the surface and absorbed by the fibers within it. The fibers are closely packed within the structure, enabling them to sorb oil as well. In effect, the sorbent material can interact with the crude oil through a sorption mechanism (). Figure A shows the oleophilic unmodified surface of the sorbent and its interaction with water ().
3.11. Reusability of SF and PF sorbents
The reusability of sorbents plays another crucial role in oil spill applications (Zhou et al., Citation2021; Bayraktaroglu et al., Citation2021). In doing this experiment, the sorbed oil was allowed to drain under gravity without any external as opposed to compression extrusion owing to the concerns raised by some researchers against it (Zamparas et al., Citation2020). The reusability performance of modified sorbents (PF and SF) was assessed by subjecting them to repeated absorption-desorption cycles in crude oil, conducted five times sequentially (). The results demonstrate that the maximum absorption performance remained consistent throughout each cycle for both sorbents, indicating their reusability in crude oil. For each cycle of adsorption-desorption, about 30% of oil is drained from the sorbent after one hour under gravity.
Following the five cycles, the PF sorbent () exhibited a water contact angle of 100.5°, while the SF sorbent () showed a water contact angle of 102°. Although slightly reduced, both contact angles remained within the hydrophobic range. Therefore, the hydrophobicity, absorption capacity, recovery rate, and reusability of the modified sorbents position them as promising candidates for oil spill applications.
Figure 11. (a and b) Photographic images of the PF and SF sorbents, respectively, after 5 cycles with water droplets on the surface to analyze their hydrophobic state.

Comparing the sorption capacities of the SF and PF sorbent materials with those documented for various natural sorbent materials in from existing literature, it is evident that the SF and PF sorbents exhibit favorable performance. When compared to most natural sorbents (both modified and unmodified), except for wastepaper and pomelo peel. This suggests that the prepared sorbents have substantial potential for effective use in the remediation of crude oil spills.
Table 5. Absorption/sorption capacities of different natural sorbents.
4. Conclusions and summary remarks
A hydrophobic and oleophilic natural sorbent material, economically derived from sugar cane (SF) and plantain pseudo-stem (PF) fibers, has been successfully developed in this study. Through a systematic exploration of varying concentrations of graphene oxide (GO), stearic acid (SA), and titanium dioxide (TiO2), an optimal combination has emerged. Specifically, an ethanol solution with a GO concentration of 4 mg/ml, paired with 100 mg TiO2 and 400 mg SA, yielded SF and PF sorbent materials with exceptional results.
Surface modification resulted in significant enhancements in water contact angles, with SF achieving 144° from an intrinsic 63°, and PF reaching 126° from an intrinsic 46°. These improvements underscore the successful augmentation of hydrophobic characteristics, a key objective of our study.
The modified SF exhibited an impressive crude oil uptake capacity of 10.29 g/g, while the PF variant reached 5.77 g/g. Notably, these remarkable results were achieved with just 30 min of contact time and 10 min of dripping time, emphasizing the efficiency of our developed sorbent materials.
In conclusion, our study demonstrates the successful synthesis of an economical and environmentally friendly sorbent material from SF and PF fibers. The optimized combination of surface modifiers has led to enhanced hydrophobic properties and remarkable oil uptake capacities. These findings underscore the potential of our sorbent materials as a practical and efficient solution for oil spill remediation.
The deficiency in the sorption capacity and reusability of SF and PF is less superior compared with some other synthetic-based sorbents. Another challenge encountered with the SF and PF natural sorbents stems from their poor oleophilic/hydrophobic properties. The synergy between the TiO2 nanoparticles, GO, SA, and the fibers can enhance the hydrophobic characteristics of the sorbents. These are some suggestions for further work.
Author contributions
All authors contributed to the study. The research conception and design were developed by EN, BAT, and SG. Materials preparation, experimental procedures, and data collection were performed by SG, DN, and YKA. The data analyses were carried out by SG, DN, YKA, BM, BAT, and EN. The first draft of the manuscript was written by SG and all authors commented on previous versions of the manuscript. A final review of the manuscript was performed by BAT and EN. All authors read and approved the final manuscript.
Consent to participate
All authors have been personally and actively involved in substantial work leading to the paper and will take public responsibility for its content.
Consent to publish
The paper is not currently being considered for publication elsewhere.
Disclosure statement
No potential conflict of interest was reported by the authors.
Data availability statement
The datasets generated during and/or analyzed during the current study are available from the corresponding authors upon reasonable request.
Additional information
Funding
Notes on contributors
Selassie Gbogbo
Selassie Gbogbo and Yaw Kwakye Adofo are PhD students in the Department Materials Science and Engineering, University of Ghana researching into photocatalysts, nanofibers and dispersants for contaminated water and oil spill remediations.
Daniel Narh
Daniel Narh is a Research Assistant in the Department of Materials Science and Engineering. He completed his Bachelor’s degree from the same department in the area photocatalyses.
Yaw Kwakye Adofo
Selassie Gbogbo and Yaw Kwakye Adofo are PhD students in the Department Materials Science and Engineering, University of Ghana researching into photocatalysts, nanofibers and dispersants for contaminated water and oil spill remediations.
Bismark Mensah
Prof. Benjamin Agyei-Tuffour, Prof. Emmanuel Nyankson and Dr. Bismark Mensah are researchers in the Department of Materials Science and Engineering. The research interests are in composites materials for energy and environmental remediation, photocatalyses and dispersants, and mechanical behaviour of materials.
Benjamin Agyei-Tuffour
Prof. Benjamin Agyei-Tuffour, Prof. Emmanuel Nyankson and Dr. Bismark Mensah are researchers in the Department of Materials Science and Engineering. The research interests are in composites materials for energy and environmental remediation, photocatalyses and dispersants, and mechanical behaviour of materials.
Emmanuel Nyankson
Prof. Benjamin Agyei-Tuffour, Prof. Emmanuel Nyankson and Dr. Bismark Mensah are researchers in the Department of Materials Science and Engineering. The research interests are in composites materials for energy and environmental remediation, photocatalyses and dispersants, and mechanical behaviour of materials.
References
- Abidli, A., Huang, Y., Cherukupally, P., Bilton, A. M., & Park, C. B. (2020). Novel separator skimmer for oil spill cleanup and oily wastewater treatment: From conceptual system design to the first pilot-scale prototype development. Environmental Technology & Innovation, 18, 100598. https://doi.org/10.1016/j.eti.2019.100598
- Adofo, Y. K., Nyankson, E., & Agyei-Tuffour, B. (2022). Dispersants as an oil spill clean-up technique in the marine environment: A review. Heliyon, 8(8), e10153. https://doi.org/10.1016/j.heliyon.2022.e10153
- Agyei-Tuffour, B., Gbogbo, S., Dodoo-Arhin, D., Damoah, L. N. W., Efavi, J. K., Yaya, A., & Nyankson, E. (2020). Photocatalytic degradation of fractionated crude oil: potential application in oil spill remediation. Cogent Engineering, 7(1), 1744944. https://doi.org/10.1080/23311916.2020.1744944
- Ahmad, F., Choi, H. S., & Park, M. K. (2015). A review: natural fiber composites selection given mechanical, lightweight, and economic properties. Macromolecular Materials and Engineering, 300(1), 10–24. https://doi.org/10.1002/mame.201400089
- Ahmad, S., Wong, Y. C., & Veloo, K. V. (2018 Sugarcane bagasse powder as biosorbent for reactive red 120 removals from aqueous solution [Paper presentation]. IOP Conference Series: Earth and Environmental Science, 140, 012027. https://doi.org/10.1088/1755-1315/140/1/012027
- Andrijanto, E., Shoelarta, S., Subiyanto, G., & Rifki, S. (2016 Facile synthesis of graphene from graphite using ascorbic acid as a reducing agent [Paper presentation]. AIP Conference Proceedings, 1725(1).
- Angelova, D., Uzunov, I., Uzunova, S., Gigova, A., & Minchev, L. (2011). Kinetics of oil and oil products adsorption by carbonized rice husks. Chemical Engineering Journal, 172(1), 306–311. https://doi.org/10.1016/j.cej.2011.05.114
- Annunciado, T. R., Sydenstricker, T. H. D., & Amico, S. C. (2005). Experimental investigation of various vegetable fibers as sorbent materials for oil spills. Marine Pollution Bulletin, 50(11), 1340–1346. https://doi.org/10.1016/j.marpolbul.2005.04.043
- Aravindh, M., Sathish, S., Ranga Raj, R., Karthick, A., Mohanavel, V., Patil, P. P., Muhibbullah, M., & Osman, S. M. (2022). A review of the effect of various chemical treatments on the mechanical properties of renewable fiber-reinforced composites. Advances in Materials Science and Engineering, 2022, 1–24. https://doi.org/10.1155/2022/2009691
- Asim, M., Paridah, M. T., Chandrasekar, M., Shahroze, R. M., Jawaid, M., Nasir, M., & Siakeng, R. (2020). Thermal stability of natural fibers and their polymer composites. Iranian Polymer Journal, 29(7), 625–648. https://doi.org/10.1007/s13726-020-00824-6
- Bae, E., & Choi, W. (2003). Highly enhanced photoreductive degradation of perchlorinated compounds on dye-sensitized metal/TiO2 under visible light. Environmental Science & Technology, 37(1), 147–152. https://doi.org/10.1021/es025617q
- Baig, M. I., Ingole, P. G., Jeon, J., Hong, S. U., Choi, W. K., & Lee, H. K. (2019). Water vapor transport properties of interfacially polymerized thin film nanocomposite membranes modified with graphene oxide and GO-TiO2 nanofillers. Chemical Engineering Journal, 373, 1190–1202. https://doi.org/10.1016/j.cej.2019.05.122
- Bayraktaroglu, S., Kizil, S., & Sonmez, H. B. (2021). A highly reusable polydimethylsiloxane sorbents for oil/organic solvent clean-up from water. Journal of Environmental Chemical Engineering, 9(5), 106002. https://doi.org/10.1016/j.jece.2021.106002
- Bazargan, A., Tan, J., & McKay, G. (2015). Standardization of oil sorbent performance testing. Journal of Testing and Evaluation, 43(6), 1271–1278. https://doi.org/10.1520/JTE20140227
- Ben Hammouda, S., Chen, Z., An, C., & Lee, K. (2021). Recent advances in developing cellulosic sorbent materials for oil spill cleanup: A state-of-the-art review. Journal of Cleaner Production, 311, 127630. https://doi.org/10.1016/j.jclepro.2021.127630
- Bhardwaj, N., & Bhaskarwar, A. N. (2018). A review on sorbent devices for oil spill control. Environmental Pollution, 243, 1758–1771. https://doi.org/10.1016/j.envpol.2018.09.141
- Chang, J., Shi, Y., Wu, M., Li, R., Shi, L., Jin, Y., Qing, W., Tang, C., & Wang, P. (2018). Solar-assisted fast cleanup of heavy oil spills using a photothermal sponge. Journal of Materials Chemistry A, 6(19), 9192–9199. https://doi.org/10.1039/C8TA00779A
- Chen, Z., Li, F., Hao, L., Chen, A., & Kong, Y. (2011). One-step electrodeposition process to fabricate cathodic superhydrophobic surface. Applied Surface Science, 258(4), 1395–1398. https://doi.org/10.1016/j.apsusc.2011.09.086
- Choi, S.-S., Chu, B., Lee, S. G., Lee, S. W., Im, S. S., Kim, S. H., & Park, J. K. (2004). Titania-doped silica fibers are prepared by electrospinning and sol-gel process. Journal of Sol-Gel Science and Technology, 30(3), 215–221. https://doi.org/10.1023/B:JSST.0000039530.09380.bc
- Chu, Z., Feng, Y., & Seeger, S. (2015). Oil/water separation with selective superantiwetting/superwetting surface materials. Angewandte Chemie International Edition, 54(8), 2328–2338. https://doi.org/10.1002/anie.201405785
- Cote, L. J., Kim, J., Tung, V. C., Luo, J., Kim, F., & Huang, J. (2010). Graphene oxide as surfactant sheets. Pure and Applied Chemistry, 83(1), 95–110. https://doi.org/10.1351/PAC-CON-10-10-25
- Danso, H. (2021). Effect of plantain pseudostem fibres and lime on the properties of cement mortar.
- de Oliveira, C. P. M., Farah, I. F., Koch, K., Drewes, J. E., Viana, M. M., & Amaral, M. C. S. (2022). TiO2-Graphene oxide nanocomposite membranes: A review. Separation and Purification Technology, 280, 119836. https://doi.org/10.1016/j.seppur.2021.119836
- Deschamps, G., Caruel, H., Borredon, M.-E., Bonnin, C., & Vignoles, C. (2003). Oil removal from water by selective sorption on hydrophobic cotton fibers. 1. Study of sorption properties and comparison with other cotton fiber-based sorbents. Environmental Science & Technology, 37(5), 1013–1015. https://doi.org/10.1021/es020061s
- Devarshi, S., Pndhare, A., Lokhande, P. E., Mooney, J. P., & Chakrabarti, S. (2023). Natural fiber-reinforced thermoplastic composite synthesis methods and potential applications. ES Materials & Manufacturing, 21, 827. https://doi.org/10.30919/esmm5f827
- Dey, S., & Haripavan, N. (2023). Applications of plants leaf-based biosorbents for removal of iron and phosphorus from contaminated water: A review. Biomedical Materials & Devices, 1(1), 122–145. https://doi.org/10.1007/s44174-022-00029-w
- Dhaka, A., & Chattopadhyay, P. (2021). A review on physical remediation techniques for treatment of marine oil spills. Journal of Environmental Management, 288, 112428. https://doi.org/10.1016/j.jenvman.2021.112428
- Dittenber, D. B., & GangaRao, H. V. S. (2012). Critical review of recent publications on the use of natural composites in infrastructure. Composites Part A: Applied Science and Manufacturing, 43(8), 1419–1429. Composites Part A: Applied Science and Manufacturing, https://doi.org/10.1016/j.compositesa.2011.11.019
- El Gheriany, I. A., El Saqa, F. A., Amer, A. A. E. R., & Hussein, M. (2020). Oil spill sorption capacity of raw and thermally modified orange peel waste. Alexandria Engineering Journal, 59(2), 925–932. https://doi.org/10.1016/j.aej.2020.03.024
- El-Din, G. A., Amer, A. A., Malsh, G., & Hussein, M. (2018). Study on the use of banana peels for oil spill removal. Alexandria Engineering Journal, 57(3), 2061–2068. https://doi.org/10.1016/j.aej.2017.05.020
- Faisal, M., Muhammad, K., Gul, S., Noor-ul-Amin. (2016). Synthesis and characterization of geopolymer from bagasse bottom ash, waste of sugar industries, and naturally available china clay. Journal of Cleaner Production, 129, 491–495. https://doi.org/10.1016/j.jclepro.2016.04.024
- Faniyi, I. O., Fasakin, O., Olofinjana, B., Adekunle, A. S., Oluwasusi, T. V., Eleruja, M. A., & Ajayi, E. O. B. (2019). The comparative analyses of reduced graphene oxide (RGO) were prepared via green, mild, and chemical approaches. SN Applied Sciences, 1(10), 1–7. https://doi.org/10.1007/s42452-019-1188-7
- Filipović, R., Lazić, D., Perušić, M., & Stijepović, I. (2010). Oil absorption in mesoporous silica particles. Processing and Application of Ceramics, 4(4), 265–269.
- Galvão, J. G., Santos, R. L., Lira, A. A. M., Kaminski, R., Sarmento, V. H., Severino, P., Dolabella, S. S., Scher, R., Souto, E. B., & Nunes, R. S. (2020). Stearic acid, beeswax, and carnauba wax as green raw materials for the loading of carvacrol into nanostructured lipid carriers. Applied Sciences, 10(18), 6267. https://doi.org/10.3390/app10186267
- Ganesh, B. M., Isloor, A. M., & Ismail, A. F. (2013). Enhanced hydrophilicity and salt rejection study of graphene oxide-polysulfone mixed matrix membrane. Desalination, 313, 199–207. https://doi.org/10.1016/j.desal.2012.11.037
- Garcia, S. M., Du Clos, K. T., Hawkins, O. H., & Gemmell, B. J. (2020). Sublethal effects of crude oil and chemical dispersants on multiple life history stages of the eastern oyster, Crassostrea virginica. Journal of Marine Science and Engineering, 8(10), 808. https://doi.org/10.3390/jmse8100808
- Ghafoori, S., Omar, M., Koutahzadeh, N., Zendehboudi, S., Malhas, R. N., Mohamed, M., Al-Zubaidi, S., Redha, K., Baraki, F., & Mehrvar, M. (2022). New advancements, challenges, and future need on the treatment of oilfield produced water: A state-of-the-art review. Separation and Purification Technology, 289, 120652. https://doi.org/10.1016/j.seppur.2022.120652
- Gholampour, A., & Ozbakkaloglu, T. (2020). A review of natural fiber composites: Properties, modification and processing techniques, characterization, applications. Journal of Materials Science, 55(3), 829–892. https://doi.org/10.1007/s10853-019-03990-y
- Gupta, S., & Tai, N. H. (2016). Carbon materials as oil sorbents: A review on the synthesis and performance. Journal of Materials Chemistry A, 4(5), 1550–1565. https://doi.org/10.1039/C5TA08321D
- He, Y., Wan, M., Wang, Z., Zhang, X., Zhao, Y., & Sun, L. (2019). Fabrication and characterization of degradable and durable fluoride-free super-hydrophobic cotton fabrics for oil/water separation. Surface and Coatings Technology, 378, 125079. https://doi.org/10.1016/j.surfcoat.2019.125079
- Hebbar, R. S., Isloor, A. M., & Ismail, A. F. (2017). Contact angle measurements. In Membrane characterization (pp. 219–255). Elsevier.
- Hoang, A. T., Nguyen, X. P., Duong, X. Q., & Huynh, T. T. (2021). Sorbent-based devices for the removal of spilled oil from water: a review. Environmental Science and Pollution Research, 28(23), 28876–28910. https://doi.org/10.1007/s11356-021-13775-z
- Holbery, J., & Houston, D. (2006). Natural-fiber-reinforced polymer composites in automotive applications. JOM Journal of the Minerals Metals and Materials Society, 58(11), 80–86. https://doi.org/10.1007/s11837-006-0234-2
- Hossain, M. K., Karim, M. R., Chowdhury, M. R., Imam, M. A., Hosur, M., Jeelani, S., & Farag, R. (2014). Comparative mechanical and thermal study of chemically treated and untreated single sugarcane fiber bundle. Industrial Crops and Products, 58, 78–90. https://doi.org/10.1016/j.indcrop.2014.04.002
- Huang, H., Tian, M., Yang, J., Li, H., Liang, W., Zhang, L., & Li, X. (2008). Stearic acid surface modifying Mg (OH) 2: Mechanism and its effect on properties of ethylene vinyl acetate/Mg (OH) 2 composites. Journal of Applied Polymer Science, 107(5), 3325–3331. https://doi.org/10.1002/app.24894
- Huang, W., Zhang, L., Lai, X., Li, H., & Zeng, X. (2020). Highly hydrophobic F-rGO@ wood sponge for efficient clean-up of viscous crude oil. Chemical Engineering Journal, 386, 123994. https://doi.org/10.1016/j.cej.2019.123994
- Huda, M. S., Drzal, L. T., Mohanty, A. K., & Misra, M. (2008). Effect of fiber surface treatments on the properties of laminated biocomposites from poly (lactic acid)(PLA) and kenaf fibers. Composites Science and Technology, 68(2), 424–432. https://doi.org/10.1016/j.compscitech.2007.06.022
- Husseien, M., Amer, A. A., El-Maghraby, A., & Taha, N. A. (2009). Availability of barley straw application on oil spill clean up. International Journal of Environmental Science & Technology, 6(1), 123–130. https://doi.org/10.1007/BF03326066
- Idris, J., Eyu, G. D., Mansor, A. M., Ahmad, Z., & Chukwuekezie, C. S. (2014). A preliminary study of biodegradable waste as sorbent material for oil spill cleanup. The Scientific World Journal, 2014, 1–5. https://doi.org/10.1155/2014/638687
- Iliyasu, I., Bello, J. B., Dibal, M. N., Oyedeji, A. N., Salami, K. A., & Oyedeji, E. O. (2022). Response surface methodology for the optimization of the effect of fiber parameters on the physical and mechanical properties of celeb palm fiber reinforced epoxy composites. Scientific African, 16, e01269. https://doi.org/10.1016/j.sciaf.2022.e01269
- Jiang, Z.-R., Ge, J., Zhou, Y.-X., Wang, Z. U., Chen, D., Yu, S.-H., & Jiang, H.-L. (2016). Coating sponge with a hydrophobic porous coordination polymer containing a low-energy CF3-decorated surface for continuous pumping recovery of an oil spill from water. NPG Asia Materials, 8(3), e253–e253. https://doi.org/10.1038/am.2016.22
- Juela, D., Vera, M., Cruzat, C., Alvarez, X., & Vanegas, E. (2021). Adsorption properties of sugarcane bagasse and corn cob for the sulfamethoxazole removal in a fixed-bed column. Sustainable Environment Research, 31(1), 1–14. https://doi.org/10.1186/s42834-021-00102-x
- Kamgar, A., & Hassanajili, S. (2020). Super-hydrophobic Fe3O4@ SiO2@ MPS nanoparticles for oil remediation: the influence of pH and concentration on clustering phenomenon and oil sorption. Journal of Molecular Liquids, 315, 113709. https://doi.org/10.1016/j.molliq.2020.113709
- Karnnet, S., Potiyaraj, P., & Pimpan, V. (2005). Preparation and properties of biodegradable stearic acid-modified gelatin films. Polymer Degradation and Stability, 90(1), 106–110. https://doi.org/10.1016/j.polymdegradstab.2005.02.016
- Khalili, D. (2016). Graphene oxide: A promising carbon catalyst for the regioselective thiocyanation of aromatic amines, phenols, anisols, and enolizable ketones by hydrogen peroxide/KSCN in water. New Journal of Chemistry, 40(3), 2547–2553. https://doi.org/10.1039/C5NJ02314A
- Kovačević, A., Radoičić, M., Marković, D., Ponjavić, M., Nikodinovic-Runic, J., & Radetić, M. (2023). Non-woven sorbent based on recycled jute fibers for efficient oil spill clean-up: From production to biodegradation. Environmental Technology & Innovation, 31, 103170. https://doi.org/10.1016/j.eti.2023.103170
- Ku, B.-J., Lee, B.-M., Kim, D. H., Mnoyan, A., Hong, S.-K., Go, K. S., Kwon, E. H., Kim, S.-H., Choi, J.-H., & Lee, K. (2021). Photothermal fabrics for efficient oil-spill remediation via solar-driven evaporation combined with adsorption. ACS Applied Materials & Interfaces, 13(11), 13106–13113. https://doi.org/10.1021/acsami.0c21656
- Kudaybergenov, K. K., Ongarbayev, E. K., & Mansurov, Z. A. (2012). Petroleum sorption by thermally treated rice husks derived from agricultural byproducts. Eurasian Chemico-Technological Journal, 15(1), 57–66. https://doi.org/10.18321/ectj141
- Kumagai, S., Noguchi, Y., Kurimoto, Y., & Takeda, K. (2007). Oil adsorbent is produced by the carbonization of rice husks. Waste Management (New York, N.Y.), 27(4), 554–561. https://doi.org/10.1016/j.wasman.2006.04.006
- Kumar, A., Singh Negi, Y., Choudhary, V., & Kant Bhardwaj, N. (2020). Characterization of cellulose nanocrystals produced by acid-hydrolysis from sugarcane bagasse as agro-waste. Journal of Materials Physics and Chemistry, 2(1), 1–8. https://doi.org/10.12691/jmpc-2-1-1
- Laitinen, O., Suopajärvi, T., Österberg, M., & Liimatainen, H. (2017). Hydrophobic, superabsorbing aerogels from choline chloride-based deep eutectic solvent pretreated and silylated cellulose nanofibrils for selective oil removal. ACS Applied Materials & Interfaces, 9(29), 25029–25037. https://doi.org/10.1021/acsami.7b06304
- Law, K.-Y. (2014). Definitions for hydrophilicity, hydrophobicity, and superhydrophobicity: getting the basics right. The Journal of Physical Chemistry Letters, 5 (4), 686–688. https://doi.org/10.1021/jz402762h
- Law, K.-Y. (2015). Water–surface interactions and definitions for hydrophilicity, hydrophobicity, and superhydrophobicity. Pure and Applied Chemistry, 87(8), 759–765. https://doi.org/10.1515/pac-2014-1206
- León, A., Reuquen, P., Garín, C., Segura, R., Vargas, P., Zapata, P., & Orihuela, P. A. (2017). FTIR and Raman characterization of TiO2 nanoparticles coated with polyethylene glycol as a carrier for 2-methoxyestradiol. Applied Sciences, 7(1), 49. https://doi.org/10.3390/app7010049
- Li, S., Du, L., Wei, Z., & Wang, W. (2017). Aqueous-phase aerosols on the air-water interface: Response of fatty acid Langmuir monolayers to atmospheric inorganic ions. The Science of the Total Environment, 580, 1155–1161. https://doi.org/10.1016/j.scitotenv.2016.12.072
- Liu, Z., Zhang, C., Zhang, X., Wang, C., Liu, F., Yuan, R., & Wang, H. (2021). Durable superhydrophobic PVDF/FEVE/GO@ TiO2 composite coating with excellent anti-scaling and UV resistance properties. Chemical Engineering Journal, 411, 128632. https://doi.org/10.1016/j.cej.2021.128632
- Loh, X. H., Daud, M. A. M., & Selamat, M. Z. (2016). Mechanical properties of kenaf/polypropylene composite: An investigation. Journal of Mechanical Engineering and Sciences, 10(2), 2098–2110.
- Loh, Y. R., Sujan, D., Rahman, M. E., & Das, C. A. (2013). Sugarcane bagasse—The future composite material: A literature review. Resources, Conservation and Recycling, 75, 14–22. https://doi.org/10.1016/j.resconrec.2013.03.002
- Lu, A., Salabas, E. e., & Schüth, F. (2007). Magnetic nanoparticles: synthesis, protection, functionalization, and application. Angewandte Chemie (International ed. in English), 46(8), 1222–1244. https://doi.org/10.1002/anie.200602866
- Ma, F., Chen, S., Liu, P., Geng, F., Li, W., Liu, X., He, D., & Pan, D. (2016). Improvement of β-TCP/PLLA biodegradable material by surface modification with stearic acid. Materials Science & Engineering. C, Materials for Biological Applications, 62, 407–413. https://doi.org/10.1016/j.msec.2016.01.087
- Mahmoudi, Z., Soleymani, F., Abbasi, S. M., Khalaj, G., & Najafi, A. (2024). Sol-gel synthesis and characterization of mesoporous TiB2 nanopowder via Titanium isopropoxide/trimethyl borate precursors. Ceramics International. https://doi.org/10.1016/j.ceramint.2024.02.304
- Mamaghani, A. H., Haghighat, F., & Lee, C.-S. (2018). Gas phase adsorption of volatile organic compounds onto titanium dioxide photocatalysts. Chemical Engineering Journal, 337, 60–73. https://doi.org/10.1016/j.cej.2017.12.082
- Mardiyati, Y., Fauza, A. N., Rachman, O. A., Steven, S., & Santosa, S. P. (2022). A Silica–Lignin Hybrid Filler in a Natural Rubber Foam Composite as a Green Oil Spill Absorbent. Polymers, 14(14), 2930. https://doi.org/10.3390/polym14142930
- Mazrouei-Sebdani, Z., Salimian, S., Khoddami, A., & Shams-Ghahfarokhi, F. (2019). Sodium silicate-based aerogel for absorbing oil from water: the impact of surface energy on the oil/water separation. Materials Research Express, 6(8), 085059. https://doi.org/10.1088/2053-1591/ab1eed
- Melesse, G. T., Hone, F. G., & Mekonnen, M. A. (2022). Extraction of cellulose from sugarcane bagasse optimization and characterization. Advances in Materials Science and Engineering, 2022, 1–10. https://doi.org/10.1155/2022/1712207
- Mihaly Cozmuta, A., Peter, A., Mihaly Cozmuta, L., Nicula, C., Crisan, L., Baia, L., & Turila, A. (2015). Active packaging system based on Ag/TiO2 nanocomposite used for extending the shelf life of bread. Chemical and microbiological investigations. Packaging Technology and Science, 28(4), 271–284. https://doi.org/10.1002/pts.2103
- Mojžiš, M., Bubeníková, T., Zachar, M., Kačíková, D., & Štefková, J. (2019). Comparison of natural and synthetic sorbents’ efficiency at oil spill removal. BioResources, 14(4), 8738–8752. https://doi.org/10.15376/biores.14.4.8738-8752
- Mugundan, S., Rajamannan, B., Viruthagiri, G., Shanmugam, N., Gobi, R., & Praveen, P. (2015). Synthesis and characterization of undoped and cobalt-doped TiO2 nanoparticles via sol–gel technique. Applied Nanoscience, 5(4), 449–456. https://doi.org/10.1007/s13204-014-0337-y
- Narayanasamy, S., Sundaram, V., Sundaram, T., & Vo, D.-V N. (2022). Biosorptive ascendency of plant-based biosorbents in removing hexavalent chromium from aqueous solutions–Insights into isotherm and kinetic studies. Environmental Research, 210, 112902. https://doi.org/10.1016/j.envres.2022.112902
- Nguyen, H. T. V., Ngo, T. H. A., Do, K. D., Nguyen, M. N., Dang, N. T. T., Nguyen, T. T. H., Vien, V., & Vu, T. A. (2019). Preparation and characterization of a hydrophilic polysulfone membrane using graphene oxide. Journal of Chemistry, 2019, 1–10. https://doi.org/10.1155/2019/3164373
- Niu, H., Li, J., Wang, X., Luo, F., Qiang, Z., & Ren, J. (2021). Solar-assisted, fast, and in situ recovery of crude oil spill by a superhydrophobic and photothermal sponge. ACS Applied Materials & Interfaces, 13(18), 21175–21185. https://doi.org/10.1021/acsami.1c00452
- Nyankson, E. J., DeCuir, M. B., & Gupta, R. (2015). Soybean lecithin as a dispersant for crude oil spills. ACS Sustainable Chemistry & Engineering, 3(5), 920–931. https://doi.org/10.1021/acssuschemeng.5b00027
- Nyankson, E., Olasehinde, O., John, V. T., & Gupta, R. B. (2015). Surfactant-loaded halloysite clay nanotube dispersants for crude oil spill remediation. Industrial & Engineering Chemistry Research, 54(38), 9328–9341. https://doi.org/10.1021/acs.iecr.5b02032
- Nyankson, E., Rodene, D., & Gupta, R. B. (2015). Advancements in crude oil spill remediation research after the deepwater horizon oil spill. Water, Air, & Soil Pollution, 227(1), 29. https://doi.org/10.1007/s11270-015-2727-5
- Onwuka, J. C., Agbaji, E. B., Ajibola, V. O., & Okibe, F. G. (2018). Treatment of crude oil-contaminated water with chemically modified natural fiber. Applied Water Science, 8(3), 1–10. https://doi.org/10.1007/s13201-018-0727-5
- Patti, A., Lecocq, H., Serghei, A., Acierno, D., & Cassagnau, P. (2021). The universal usefulness of stearic acid as a surface modifier: applications to the polymer formulations and composite processing. Journal of Industrial and Engineering Chemistry, 96, 1–33. https://doi.org/10.1016/j.jiec.2021.01.024
- Peterson, C. H., Rice, S. D., Short, J. W., Esler, D., Bodkin, J. L., Ballachey, B. E., & Irons, D. B. (2003). Long-term ecosystem response to the exxon valdez oil spill. Science (New York, N.Y.), 302(5653), 2082–2086. (https://doi.org/10.1126/science.1084282
- Ponec, V., Knor, Z., & Cerny, S. (2018). Adsorption on solids. Butterworth.
- Prabhath, N., Kumara, B. S., Vithanage, V., Samarathunga, A. I., Sewwandi, N., Damruwan, H.-G H., Lewangamage, S., & Koswattage, K. R. (2023). Investigation of pozzolanic properties of sugarcane bagasse ash for commercial applications. ACS Omega, 8(13), 12052–12061. https://doi.org/10.1021/acsomega.2c07844
- Ramesh, M., Rajeshkumar, L., & Bhuvaneswari, V. (2022). Leaf fibers as reinforcements in green composites: a review on processing, properties, and applications. Emergent Materials, 5(3), 833–857. https://doi.org/10.1007/s42247-021-00310-6
- Rangappa, S. M., & Siengchin, S. (2018). Natural fibers as perspective materials. Applied Science and Engineering Progress, 11(4).
- Ratcha, A., Yoosuk, B., & Kongparakul, S. (2013). Grafted methyl methacrylate and butyl methacrylate onto natural rubber foam for oil sorbent. Advanced Materials Research, 844, 385–390. https://doi.org/10.4028/www.scientific.net/AMR.844.385
- Rather, A. M., Jana, N., Hazarika, P., & Manna, U. (2017). Sustainable polymeric material for the facile and repetitive removal of oil spills through the complementary use of both selective absorption and active-filtration processes. Journal of Materials Chemistry A, 5(44), 23339–23348. https://doi.org/10.1039/C7TA07982F
- Sadatshojaie, A., Wood, D. A., Jokar, S. M., & Rahimpour, M. R. (2021). Applying ultrasonic fields to separate water contained in medium-gravity crude oil emulsions and determining crude oil adhesion coefficients. Ultrasonics Sonochemistry, 72, 105448. https://doi.org/10.1016/j.ultsonch.2020.105448
- Santos, A. S., Ferreira, P. J. T., & Maloney, T. (2021). Bio-based materials for nonwovens. Cellulose, 28(14), 8939–8969. https://doi.org/10.1007/s10570-021-04125-w
- Satria, M., & Saleh, T. A. (2022). Effect of loading various nanoparticles on superhydrophobic/superoleophilic stearic acid-modified polyurethane foams for oil-water separation. Journal of Environmental Chemical Engineering, 10(6), 108577. https://doi.org/10.1016/j.jece.2022.108577
- Scheufele, F. B., Ribeiro, C., Módenes, A. N., Espinoza-Quiñones, F. R., Bergamasco, R., & Pereira, N. C. (2015). Assessment of drying temperature of sugarcane bagasse on sorption of reactive blue 5G dye. Fibers and Polymers, 16(8), 1646–1656. https://doi.org/10.1007/s12221-015-5087-2
- Shah, M. U. H., Moniruzzaman, M., Reddy, A. V. B., Talukder, M. M. R., Yusup, S. B., & Goto, M. (2020). An environmentally benign ionic liquid based formulation for enhanced oil spill remediation: Optimization of environmental factors. Journal of Molecular Liquids, 314, 113603. https://doi.org/10.1016/j.molliq.2020.113603
- Shi, Z., Li, Y., Dong, L., Guan, Y., & Bao, M. (2021). Deep remediation of oil spill based on the dispersion and photocatalytic degradation of biosurfactant-modified TiO2. Chemosphere, 281, 130744. https://doi.org/10.1016/j.chemosphere.2021.130744
- Sholeh, M., Rochmadi, R., Sulistyo, H., & Budhijanto, B. (2021). Nanostructured silica from bagasse ash: the effect of synthesis temperature and pH on its properties. Journal of Sol-Gel Science and Technology, 97(1), 126–137. https://doi.org/10.1007/s10971-020-05416-7
- Singh, H., Bhardwaj, N., Arya, S. K., & Khatri, M. (2020). Environmental impacts of oil spills and their remediation by magnetic nanomaterials. Environmental Nanotechnology, Monitoring & Management, 14, 100305. https://doi.org/10.1016/j.enmm.2020.100305
- Some, S., Xu, Y., Kim, Y., Yoon, Y., Qin, H., Kulkarni, A., Kim, T., & Lee, H. (2013). Highly sensitive and selective gas sensor using hydrophilic and hydrophobic graphenes. Scientific Reports, 3(1), 1868. https://doi.org/10.1038/srep01868
- Songsaeng, S., Thamyongkit, P., & Poompradub, S. (2019). Natural rubber/reduced-graphene oxide composite materials: Morphological and oil adsorption properties for treatment of oil spills. Journal of Advanced Research, 20, 79–89. https://doi.org/10.1016/j.jare.2019.05.007
- Sood, A., Gupta, A., Bharadwaj, R., Ranganath, P., Silverman, N., & Agrawal, G. (2022). Biodegradable disulfide crosslinked chitosan/stearic acid nanoparticles for dual drug delivery for colorectal cancer. Carbohydrate Polymers, 294, 119833. https://doi.org/10.1016/j.carbpol.2022.119833
- Tang, Z., Hess, D. W., & Breedveld, V. (2015). Fabrication of oleophobic paper with tunable hydrophilicity by treatment with non-fluorinated chemicals. Journal of Materials Chemistry A, 3(28), 14651–14660. https://doi.org/10.1039/C5TA03520A
- Torres, D. H. A., da Costa Dias, F., Bahiana, B. R., Haddad, A. N., Chinelli, C. K., & Soares, C. A. P. (2020). Oil spill simulation and analysis of its behavior under the effect of weathering and chemical dispersant: a case study of the Bacia de Campos—Brazil. Water, Air, & Soil Pollution, 231(10), 1–21. https://doi.org/10.1007/s11270-020-04857-8
- Tran, H. N., You, S.-J., Hosseini-Bandegharaei, A., & Chao, H.-P. (2017). Mistakes and inconsistencies regarding adsorption of contaminants from aqueous solutions: a critical review. Water Research, 120, 88–116. https://doi.org/10.1016/j.watres.2017.04.014
- Turco, A., Malitesta, C., Barillaro, G., Greco, A., Maffezzoli, A., & Mazzotta, E. (2015). A magnetic and highly reusable macroporous superhydrophobic/superoleophilic PDMS/MWNT nanocomposite for oil sorption from water. Journal of Materials Chemistry A, 3(34), 17685–17696. https://doi.org/10.1039/C5TA04353K
- Vlaev, L., Petkov, P., Dimitrov, A., & Genieva, S. (2011). Cleanup of water polluted with crude oil or diesel fuel using rice husk ash. Journal of the Taiwan Institute of Chemical Engineers, 42(6), 957–964. https://doi.org/10.1016/j.jtice.2011.04.004
- Vocciante, M., Finocchi, A., De Folly D′ Auris, A., Conte, A., Tonziello, J., Pola, A., & Reverberi, A. Pietro. (2019). Enhanced oil spill remediation by adsorption with interlinked multilayered graphene. Materials, 12(14), 2231. https://doi.org/10.3390/ma12142231
- Wang, B., Liang, W., Guo, Z., & Liu, W. (2015). Biomimetic super-lyophobic and super-lyophilic materials applied for oil/water separation: A new strategy beyond nature. Chemical Society Reviews, 44Issue(1), 336–361. (https://doi.org/10.1039/c4cs00220b
- Wang, H., Wang, E., Liu, Z., Gao, D., Yuan, R., Sun, L., & Zhu, Y. (2015). Novel carbon nanotubes reinforced superhydrophobic and superoleophilic polyurethane sponge for selective oil–water separation through a chemical fabrication. Journal of Materials Chemistry A, 3(1), 266–273. https://doi.org/10.1039/C4TA03945A
- Wang, L., & Hou, D. (2024). Underlying mechanisms involved in biochar-induced metal stabilization. In Biochar application in soil to immobilize heavy metals (pp. 9–43). Elsevier.
- Wang, Y., Shi, Z., & Yin, J. (2011). Facile synthesis of soluble graphene via a green reduction of graphene oxide in tea solution and its biocomposites. ACS Applied Materials & Interfaces, 3(4), 1127–1133. https://doi.org/10.1021/am1012613
- Wang, Y., Zhou, L., Luo, X., Zhang, Y., Sun, J., Ning, X., & Yuan, Y. (2019). Solar-heated graphene sponge for high-efficiency clean-up of viscous crude oil spill. Journal of Cleaner Production, 230, 995–1002. https://doi.org/10.1016/j.jclepro.2019.05.178
- Wong, C., McGowan, T., Bajwa, S. G., & Bajwa, D. S. (2016). Impact of fiber treatment on the oil absorption characteristics of plant fibers. BioResources, 11(3), 6452–6463. https://doi.org/10.15376/biores.11.3.6452-6463
- Wu, X., Lei, Y., Li, S., Huang, J., Teng, L., Chen, Z., & Lai, Y. (2021). Photothermal and Joule heating-assisted thermal management sponge for efficient cleanup of highly viscous crude oil. Journal of Hazardous Materials, 403, 124090. https://doi.org/10.1016/j.jhazmat.2020.124090
- Yang, J., Zhang, Z., Men, X., Xu, X., & Zhu, X. (2011). A simple approach to fabricate superoleophobic coatings. New Journal of Chemistry, 35(3), 576–580. https://doi.org/10.1039/C0NJ00826E
- Yang, T., Feng, J., Zhang, Q., Wu, W., Mo, H., Huang, L., & Zhang, W. (2020). l-Carnitine conjugated chitosan-stearic acid polymeric micelles for improving the oral bioavailability of paclitaxel. Drug Delivery, 27(1), 575–584. https://doi.org/10.1080/10717544.2020.1748762
- Yang, X., Fan, W., Wang, H., Shi, Y., Wang, S., Liew, R. K., & Ge, S. (2022). Recycling of bast textile wastes into high value-added products: a review. Environmental Chemistry Letters, 20(6), 3747–3763. https://doi.org/10.1007/s10311-022-01484-z
- Yen Tan, J., Yan Low, S., Ban, Z. H., & Siwayanan, P. (2021). A review on oil spill clean-up using bio-sorbent materials with special emphasis on utilization of Kenaf Core Fibers. BioResources, 16(4), 8394–8416. https://doi.org/10.15376/biores.16.4.8394-8416
- Yurak, V., Apakashev, R., Dushin, A., Usmanov, A., Lebzin, M., & Malyshev, A. (2021). Testing of natural sorbents for the assessment of heavy metal ions’ adsorption. Applied Sciences, 11(8), 3723. https://doi.org/10.3390/app11083723
- Zaheer, S., Bhatti, H. N., Sadaf, S., Safa, Y., & Zia-Ur-Rehman, M. (2014). Biosorption characteristics of sugarcane bagasse for the removal of Foron Blue E-BL dye from aqueous solutions. JAPS: Journal of Animal & Plant Sciences, 24(1).
- Zamparas, M., Tzivras, D., Dracopoulos, V., & Ioannides, T. (2020). Application of sorbents for oil spill cleanup focusing on natural-based modified materials: A review. Molecules (Basel, Switzerland), 25(19), 4522. https://doi.org/10.3390/molecules25194522
- Zhang, L., He, Y., Luo, P., Ma, L., Li, S., Nie, Y., Zhong, F., Wang, Y., & Chen, L. (2022). Photocatalytic GO/M88A “interceptor plate” assembled nanofibrous membrane with photo-Fenton self-cleaning performance for oil/water emulsion separation. Chemical Engineering Journal, 427, 130948. https://doi.org/10.1016/j.cej.2021.130948
- Zhong, Y., Wang, R., Chen, J., Duan, C., Huang, Z., Yu, S., Guo, H., & Zhou, Y. (2022). Surface-terminated hydroxyl groups for deciphering the facet-dependent photocatalysis of anatase TiO2. ACS Applied Materials & Interfaces, 14(15), 17601–17609. https://doi.org/10.1021/acsami.2c04302
- Zhou, J., Zhang, Y., Jia, G., Chen, Z., Yang, Y., & Zhang, L. (2021). A multifunctional sponge incorporated with TiO 2 and graphene oxide as a reusable absorbent for oil/water separation and dye absorption. New Journal of Chemistry, 45(10), 4835–4842. https://doi.org/10.1039/D0NJ06298G
- Zhu, C., Guo, S., Fang, Y., & Dong, S. (2010). Reducing sugar: new functional molecules for the green synthesis of graphene nanosheets. ACS Nano, 4(4), 2429–2437. https://doi.org/10.1021/nn1002387
- Zhu, J., Liu, B., Li, L., Zeng, Z., Zhao, W., Wang, G., & Guan, X. (2016). Simple and green fabrication of a superhydrophobic surface by one-step immersion for continuous oil/water separation. The Journal of Physical Chemistry. A, 120(28), 5617–5623. https://doi.org/10.1021/acs.jpca.6b06146
- Zoghi, A. M., & Allahyari, S. (2022). Multifunctional magnetic C3N4-rGO adsorbent with high hydrophobicity and simulated solar light-driven photocatalytic activity for oil spill removal. Solar Energy, 237, 320–332. https://doi.org/10.1016/j.solener.2022.04.005