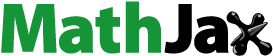
Abstract
Fish protein concentrate (FPC) is considered as a promising alternative to overcome the world’s shortage of affordable animal-based protein. Snakehead fish (Channa striata) offers a large potential as a raw material for FPC manufacture. This work aims to investigate the influence of solvent types (ethanol, n-hexane, and an azeotrope mixture of ethanol + n-hexane), extraction time (0, 10, 15, 20, 25, and 30 minutes), temperature (30, 40, 50, and 60°C), liquid solvent volume to solid feed fish flesh mass ratio (LSSFR) (1, 2, 3, 4, 5, 6, and 7 mL/g), and ultrasonic power (100, 200, 300, and 400 W) on the ultrasound-assisted extraction (UAE) to manufacture high-quality FPC from snakehead fish. After being subjected to UAE, the fish flesh was filtered and dried under reduced pressure at 55°C. The optimum UAE conditions for the manufacture of FPC from snakehead fish flesh were using ethanol as solvent, LSSFR (6 mL/g), temperature (40°C), ultrasonic power (200 W), and extraction time (25 minutes). The resulted FPC can be categorized as type A, which contains protein (73.17%) and fat (0.73%). An SDS-PAGE electrophoresis evaluation proved that the FPC contained 12 types of peptides having molecular weight (MW) ranging from 4.00 to 174.00 kDa with the major fractions being those with MWs ranged from 4.00 to 11.00 kDa and the minor fractions with MWs between 71.70 to 174.00 kDa. Indeed, the presence of both low and high MW peptides in the FPC indicates its potential applications in the development of nutritious food products.
PUBLIC INTEREST STATEMENT
This paper provides a rigorous finding of fish protein concentrate (FPC) manufacturing from the flesh of Snakehead fish via ultrasound-assisted extraction using ethanol for lipid removal,followed by vacuum drying for water removal. The FPC obtained is classified as the A-type, which exhibits white in color, no fishy odor, high protein content, and low moisture and lipid contents that make it suitable for functional food development. The method can also be applied to the processing of protein concentrate from other sources, including plants, fish, poultry, and mammalian residues.
1. Introduction
Global demand for healthy proteinaceous materials to fulfill the macronutrient needs to create functional foods and ready-to-eat products for the world’s population continues to increase (Shaviklo, Citation2015). Fish protein concentrate (FPC) is a dry stabilized protein preparation product designated for human consumption, which is manufactured via removal all non-protein components, especially undesirable fats and water to achieve high protein content compared to the raw fish flesh (Amoo et al., Citation2016; Ibrahim, Citation2009). FPC has emerged as an extremely useful protein source for people living at the protein malnourished areas of the world for decades (Akhade et al., Citation2016). Indeed, fish in the form of FPC is one of the several alternatives that can be utilized as a substitute or fortification element in various low-protein food products. Food industries employ FPC as a binder, dispersant, and emulsifier in the production of red meat and poultry re-structured products, herring roes, and fillet blocks. It is due to exceptional protein interactions and high gelation capacity (Pires et al., Citation2012). Furthermore, they also utilize FPC to manufacture formulated seafood and enrich various food products (Shaviklo, Citation2015). For example, the FPC can be converted into surimi through the addition of 4 times the weight of water and possesses exceptional gelling properties (Shaviklo, Citation2015). As expected, the dry form of FPC provides numerous strong points in the food enterprise, namely ease of handling and mixing with other ingredients, comfortable storage, low transportation cost, and a longer shelf life (Shaviklo, Citation2015). In addition, the elimination of fishy odor from fish flesh that is mainly contained in the fats during FPC manufacturing improves consumer acceptance to this fish products (Asfar et al., Citation2014).
Important determinants of FPC quality include the variety of fish, the extraction method, the type of process and solvent (Ibrahim, Citation2009; Romadhoni et al., Citation2016). As of now, it has been reported that fish possessing white flesh and a low lipid content are the most suitable resources for the manufacturing of FPC (Hultin et al., Citation2005; Park & Lin, Citation2005). Additionally, the quality of raw materials is also essential in the manufacturing of FPC. Therefore, appropriate postharvest technology in fresh fish and by-products is crucial to avoid degradation and denaturation of myofibria proteins (Shaviklo, Citation2015). Therefore, freezing is recommended to maintain fish quality during fishing in tropical regions. As a maritime country, Indonesia has a large potential to produce FPC due to its vast availability of fish sources. Snakehead fish (Channa striata) is mostly found in swamps, lakes, and small rivers along East Kalimantan’s Mahakam watershed, Java, Sulawesi, and other islands in Indonesia as well as some other countries. Snakehead fish is considered a valuable biological resource due to its substantial protein content, which holds its potential for application in the manufacturing of FPC (Romadhoni et al., Citation2016). Previous report revealed that fresh snakehead fish contain a higher amount of protein (25%), which is slightly higher than golden fish (16.05%), snapper (20.0%), whitefish (20.0%), and sardines (21.1%) (Asfar et al., Citation2014).
Diverse techniques for extracting valuable proteins from fish flesh have been developed. Overarchingly, these processes can be classified into six categories: repeated water washing and refining, pH-shift, solvent extraction, thermal treatment, enzyme or acid hydrolysis, and combinations thereof (Shaviklo, Citation2015). The repeated water washing and refining method uses fresh and chilled fish in which the majority of water-soluble proteins are removed, leaving behind only water-insoluble proteins. These water insoluble proteins are highly-elastic and suitable to produce surimi. To separate the myofibrillar proteins from oily or dark muscle fish, such as mackerels and sardines, alkaline chelation must be employed to revoke the influences of haem proteins and oils (Hultin et al., Citation2005). The quantity and species of water utilized, the intended quality of the product, the washing apparatus in use, and the number of washing cycles all influence the water quantity and frequency. In general, the acceptable product can be obtained by carrying out the procedure by 2 to 4 repetitions (Park & Lin, Citation2005). Frozen surimi should be stored at a temperature of −20°C (Park & Lin, Citation2005; Venugopal, Citation2006). In the pH shift method, the minced fish flesh is homogenized in five to ten times the volumes of an alkali (pH 11) or acidic (pH 2.5) solution to allow solubilization of its proteins (Hultin et al., Citation2005). A high-speed centrifugation is usually applied to completely eliminate of the oil and other insoluble materials from the mixture. Upon complete precipitation of the myofibrillar proteins at the isoelectric point, around pH 5.2 to 5.5 (Park & Lin, Citation2005), the proteins are further separated from the liquid using a high-speed centrifuge. The FPC obtained should be stabilized against freezing using suitable cryoprotectants (Campo-Deano et al., Citation2010; Shaviklo, Citation2015; Thawornchinsombut & Park, Citation2006). Solvent extraction method utilizes ethanol, propanol, n-hexane, ethylene dichloride or their mixture as solvent to eliminate water, fat and fishy-tasting constituents from fish flesh (Kumoro et al., Citation2022). The quality of the extracted fish protein is contingent upon the extraction conditions and the quality of the raw materials. This method results in three types of FPC, namely Type A (an almost tasteless and odorless powder with a maximum lipid content of 0.75%), Type B (a powder lacking specific flavor and odor limits but possessing an undeniable fishy aroma; the lipid content should not exceed 3%) and Type C (ordinary fish powder manufactured under adequate hygienic conditions) (Sen, Citation2005; Windsor, Citation2022). Although the FPC obtained remains stable for 6 months at 5°C when stored in an airtight packing, it usually lacks of functional properties that become its main drawback (Shaviklo, Citation2015; Windsor, Citation2022). The heating treatment method involves blending minced fish flesh in an equivalent volume of distilled water at a high speed for two minutes. Subsequently, the mixture is stirred continuously at 85°C for sixty minutes, facilitating the liberation of oil from the ruptured fat cells into the water. After 15 minutes of centrifugation at 2,560 g, the insoluble proteins and the heavier liquid middle layer are collected and desiccated by freeze or spray drying. The FPC manufactured by this technique contains 63–81.4% protein with preferable nutritional and functional properties, especially the emulsifying and lipid adsorption capacities (Sathivel et al., Citation2009). For protein extraction using enzyme/acid hydrolysis, the minced fish or fish by-products are suspended in water at a certain pH, temperature and stirring condition that is suitable for the chosen enzyme to break the peptide bonds in proteins for one to several hours (Kristinsson & Rasco, Citation2000). Every so often, endogenous proteases are denaturated by subjecting the unprocessed material to initial heating (Shaviklo, Citation2015). The functional and biological properties of the resulting FPC are significantly affected by the degree of hydrolysis, which can be controlled by inactivation of the enzyme by pH or temperature adjustment (Kim et al., Citation2001; Kristinsson & Rasco, Citation2000). Considering the techno-economical nature of the aforesaid methods and their product quality, the solvent extraction method can be the best option. The currently available commercial FPC processing using solvent extraction methods include the British, Lever-brother, Viobin, Canadian, Swedish, Indian (azeotropic), Peruvian, Mexican, and Chilean processing methods (Kumoro et al., Citation2022).
The FPC processing methods that involve extraction stage requires the appropriate and safe solvent because it has significant effects not only on the extraction yield and selectivity, but also the safety of the product (Shaviklo, Citation2015). Commercial solvents, such as ethanol and n-hexane are frequently employed to remove the undesirable fats and water from fish flesh in the manufacture of FPC either in pure state or their mixture. As a polar organic solvent, ethanol can result in a brighter-colored and more neutral-tasting FPC (Kristinsson et al., Citation2006; Kumoro et al., Citation2022). Previous studies reported that the use of ethanol as a solvent to manufacture FPC results in a FPC with protein content ranging from 60%-80% (Asfar et al., Citation2014; Wiharja et al., Citation2013). On the other hand, n-hexane as a non-polar organic solvent has found extensive application in the chemical and food industries both in its pure form and as a mixture with other solvents (Holdsworth & Ellis, Citation2005). Nevertheless, there are only a few studies on the use of pure n-hexane and ethanol, as well as their mixtures in the manufacture of FPC. Furthermore, in order to enhance the efficacy of these solvent extraction techniques, one may incorporate ultrasonic wave irradiation, a process referred to as ultrasound-assisted extraction (UAE) (Chemat et al., Citation2017).
Although the UAE is environmentally benign, fast, and effective in non-thermal extraction, it is still under development (Wen et al., Citation2018). In the UAE method, acoustic cavitation arises as a result of ultrasonic wave propagation in a liquid extraction medium. During the UAE, the resulting ultrasonic waves promote the enhancement of both pressure and temperature of the liquid in the vicinity of the microbubbles, causing severe cells and membranes damage as well as solvent penetration into the raw material and thus improving extraction efficiency (Balachandran et al., Citation2006; Wen et al., Citation2018). For example, Bing et al. extracted sheep abomasum protein using the UAE method resulted in a comparable yield with the conventional methods at a shorter extraction time (1/10 time) (Bing et al., Citation2019). Yang et al. reported that the Chondroitin sulfate (ChS) extract yield 1.3-fold when it is extracted from jumbo squid (Dosidicus gigas) using UAE for 30 min compared to the conventional extraction (Yang et al., Citation2021). Similarly, Sun et al. also found that UAE successfully extracted 5 times amount of lutein from chicken liver compared to the conventional solvent extraction using the same operating conditions (Sun et al., Citation2006). Moreover, Li et al. reported several additional advantages of ultrasound-assisted electrolyzed water extraction for Antarctic krill (Euphausia superba) proteins: increased protein yield (9.4%) and solubility (8.5%), decreased powder size (57 nm), advantageous transition of α-helix and β-turn to β-sheet, and enhanced surface hydrophobicity and disulfide bond formation (Li et al., Citation2021).
Based on the aforementioned facts, this work was carried out to separate protein from non-protein components found in the raw snakehead fish flesh to manufacture snakehead fish FPC. In addition, this work also aims to determine an appropriate solvent and temperature for the manufacture high-quality snakehead FPC via UAE method employing ethanol and n-hexane as solvents, and identify the chemical composition and amino acids profile of snakehead FPC.
2. Materials and methods
2.1. Materials and equipment
Snakehead fish (Channa striata) as the raw material was obtained from a local fish farm in Karangawen, Demak, Indonesia. The HPLC grade ethanol and n-hexane used as the solvent were obtained from Merck KgaA (Darmstadt, Hesse, Germany). The other chemicals were of analytical grade (with purity ≥ 98.5%) and procured from Sigma-Aldrich (Singapore). The ultrasonic instrument used was the Toption TUE-500 Digital Sonifier 500 W Ultrasonic Cell Disruptor/Homogenizer (Shaanxi, China). In addition, a set of high-performance liquid chromatography (Waters Alliance HPLC 2695 Separations Module, Waters Corporation- USA) was utilized to examine the amino acids composition of the Snakehead FPC.
3. Methods
3.1. Preparation of snakehead fish flesh
The snakehead fish used in this research were matured farmed fish whose whole-body length measured between 25–30 cm. The freshly harvested snakehead fish are slaughtered with a stainless-steel knife and cleaned off the entrails, spines, scales, fins, tail, and head. Then, the flesh was carefully separated from the unwanted parts. Subsequently, the fish flesh was rinsed under running water and minced into small pieces (2–3 cm). Prior to the conventional extraction and UAE, the minced fish flesh was mashed for 1 minute in a grinder and stored at −5°C to prevent decay.
3.2. Ultrasonic-assisted extraction of phospholipids from snakehead fish flesh
The UAE was carried out in two stages, namely the preliminary and main experiments. The preliminary stage was performed to determine the best extraction time to allow exhaustive extraction of phospholipids from snakehead fish flesh using UAE. The preliminary UAE experiments were carried out for 5, 10, 15, 20, 25, and 30 minutes duration in triplicates, from which 25 minutes was found to be the most acceptable extraction time based on technical and economical point of views, especially the protein content (70% at minimum) and the amount of energy required to conduct the extraction with the assistance of ultrasonic wave irradiation and maintain the temperature. Accordingly, the main UAE experimental stage was subsequently conducted in an effort to determine an appropriate solvent and optimum extraction temperature. The extraction method for snakehead fish flesh was modified from Romadhoni et al (Romadhoni et al., Citation2016). In the UAE’s main experiment, the minced snakehead fish flesh was introduced into a 500 mL beaker glass and then vigorously mixed with each solvent in a 1:6 ratio (100 g sample: 600 mL solvent). The fish flesh and solvent mixture was then subjected to ultrasound irradiation for 25 minutes (the best extraction time obtained from preliminary experiment) at temperatures of 30, 40, 50, and 60°C in an ultrasonic processor (Toption TUE-500 Digital Sonifier 500 W Ultrasonic Cell Disruptor) equipped with an 8 mm microtip. The power setting for the extraction was 200 W, with the processor operating at a frequency of 25 kHz, unless otherwise specified. The effects of solvent types (ethanol, n-hexane, and an azeotrope mixture of ethanol + n-hexane), extraction time (0, 10, 15, 20, 25, 30 minutes), temperature (30, 40, 50, and 60°C), solvent volume to fish flesh mass ratio (1, 2, 3, 4, 5, 6, 7), and ultrasonic power (100, 200, 300, 400 W) were investigated. The treatment parameters were selected based on the literature study that the lipids contained in Snakehead fish comprise neutral lipids, glycolipids, and phospholipids, which require appropriate solvent to dissolve. Hence, the recommended solvents are ethanol, n-hexane, and an azeotrope mixture of ethanol + n-hexane. Accordingly, the extraction temperature (30, 40, 50, and 60°C) was selected based on the consideration that the surface tension of the solvent should be high enough to avoid microbubbles explosion before hitting the minced Snakehead fish flesh. Meanwhile, the solvent volume to fish flesh mass ratio (1, 2, 3, 4, 5, 6, 7) and ultrasonic power (100, 200, 300, 400 W) are also chosen based on literature study (Ali et al., Citation2019), that reported the optimum solid-solvent ratio and temperature were 1:6 w/v and 40°C, respectively. The pre-sonicated mixtures were filtered using a separator funnel to allow complete separation between the filtrate (liquid) and substrate (solid). The resulting substrate was then oven dried for 12 hours in a vacuum drying oven at 55°C with a pressure of 0.05 MPa until the achievement of a constant weight. A vacuum-drying oven facilitates drying under reduced pressure (±0.05 MPa), which lowers the heat required for rapid drying and reducing the economic and environmental costs affiliated with drying a product for storage, sale or other intentions. Vacuum-drying is also capable to lower the boiling point of the solvent and facilitate faster removal of moisture or other solvent at lower temperatures to the recommended final moisture content (usually less than 10 wt.%). Therefore, thermal degradation of the proteins and their amino acids can be reduced or avoided. In addition, vacuum drying effectively reduces oxidation and preserves the color, texture, and flavor of desiccated products by eliminating the presence of air. This characteristic provides vacuum drying a viable method for handling heat-sensitive and volatile substances (Parikh, Citation2015). The collected FPC results were then examined for their chemical composition. As a comparison, the conventional solvent extraction was also performed to the fish flesh at the same operating condition. The conventional extraction was conducted by vigorous stirring of snakehead fish flesh sample in ethanol as solvent with the ratio of minced fish flesh weight (g) to solvent volume (mL) was 1/6 at 40°C for 24 hours to allow exhaustive lipids removal, segregating the fish flesh from the solvent and followed by vacuum drying of the resulting solid substrates at 55°C to dryness. Protein yield (Y) was calculated as the percentage ratio of the FPC obtained with the raw minced fish flesh according to Eq. (1). Each run of the experiment was conducted in triplicates, and the reported value was the average value of them.
In industrial applications, the enterprises are more interested to know the apparent FPC yield calculated using EquationEquation (1)(1)
(1) rather than the specific yield calculated based on the protein content alone because it is easier for them to evaluate the economic feasibility of the respective FPC manufacturing methods.
3.3. Physicochemical characteristics analysis
Protein content (P). The quantification of total protein contents in the samples was performed using the Kjeldahl method in accordance with the Association of Official Analytical Chemists (AOAC) protocols, with a conversion factor of 6.25 (AOAC Association of Official Analytical Chemists, Citation2012). 2.0 g protein sample was subjected to digestion by simmering it in 20 mL of concentrated H2SO4 containing 5.0 g Kjeldahl catalyst and one glass bead to avoid bumping until the solution became transparent in color. To ensure that every organic substance was completely digested, the resulting solution was heated for an additional hour. Prior to distillation, the digest solution was then let to equilibrated to room temperature before carefully added with 100 mL of distilled water and 70 mL of 50% sodium hydroxide solution. A 50 mL Erlenmeyer flask containing 4% H3BO3 solution was utilized to capture the ammonia during distillation. Out of the distillate collected, around 150 mL was titrated with 0.1 N HCl using 6–7 drops of methyl red as an indicator until the solution changed color from green to pink. A blank titration was also conducted in the same manner for the solution lacking the sample. Finally, the protein content (P%) was determined by employing EquationEquation (2)(2)
(2) :
where Vts and Vtb are the volume of HCl required to titrate the sample and blank solution, whereas NHCl donates the normality of HCl solution (g eq./L).
Lipid content (L). The total lipids of the fish flesh were extracted using the established method detailed by Folch et al (Hewavitharana et al., Citation2020; Liu et al., Citation2013; Misir & Koral, Citation2019). A Potter-Elvehjem homogenizer was utilized to combine 20 mL chloroform—methanol (2:1, v/v) with 1 g of fish flesh or FPC sample. The tube containing the homogenizer was weighed and calibrated at the volume of the final dilution of the desired tissue homogenate, which was 20 times the final dilution. Once the volume and temperature have been adjusted to equilibrate, the homogenate is filtered into a glass-stoppered vessel using fat-free paper. After that, the crude lipid extract was then meticulously rinsed with 0.2 volume of water to facilitate the separation of two phases without interfacial debris. This was accomplished either by leaving the sample undisturbed for 12 hours or by subjecting it to centrifugation at 3,500 rpm for 10 minutes. The lower organic phase represented the total pure lipids extract, which was subsequently dried under nitrogen gas at 105°C to allow drying until the achievement of constant weight. After drying, the total lipids were allowed to equilibrated to room temperature and were quantified gravimetrically in triplicate through calculation using EquationEquation (3)(3)
(3) :
Ash content (A). The mineral content in fish sample was examined by ash contents analysis according to the protocols established by the AOAC (AOAC Association of Official Analytical Chemists, Citation2012). Approximately 5 g triplicate fish sample was weighed into empty carefully pre-weighed crucibles and introduced into a muffle furnace that was set to ignite at 550°C for 12 hours. Upon the completion of washing, the furnace was turned off and allowed to decrease to 250°C to ensure a safe sample removal. Prior to weighing, the samples were desiccated and allowed to equilibrate at ambient temperature. The ash content was then calculated using EquationEquation (4)(4)
(4) :
Water content (W). Water content of the fish sample was examined using proximate analysis method suggested by AOAC (AOAC Association of Official Analytical Chemists, Citation2012). A carefully prepared 0.5 g of homogenized fish sample was positioned in a pre-weighed aluminum dish and subjected to a 24-hour heating process in a hot air oven set at 105oC. Following the sample’s cooling to room temperature in a desiccator, the water content was determined using EquationEquation (5)(5)
(5) :
To ensure data reproducibility, water content examination was performed in triplicate.
Total carbohydrate content (C). Following the AOAC (AOAC Association of Official Analytical Chemists, Citation2012) recommendation, the total carbohydrate content of the FPC was estimated by deduction of the sums of the percentage the crude protein, total lipid, water, and ash from the total percentage (100) of the fish sample using EquationEquation (6)(6)
(6) :
3.3.1. Molecular weight analysis
3.3.1.1. Sample preparation. A carefully weighed 1 gram dried and mashed fish protein was prepared. The fish protein was dissolved in 9.5 mL of physiological saline (NaCl 0.9%) in a 50 mL beaker glass and further homogenized for 1 minute with the assistance of a magnetic bar and a magnetic stirrer at 4°C. The resulting solution was then stirred continuously for 20 minutes at 2°C prior to centrifugation for 30 minutes at 4°C at a speed of 4000 rpm. Before SDS-PAGE analysis, the supernatant was stored in a −18°C freezer.
3.3.1.2. Gel preparation. After preparing the separating gel, butanol is applied to the surface and left until polymerization occurs before being rinsed with distilled water and addition of stacking gel. The comb was inserted and allowed to polymerize. The comb was lifted in order for life to (sort of) form. The sample was placed in a 4:1 well ratio (16 μL sample: 4 μL sample buffer). When a running buffer is added to the appliance, the power supply was switched on. Then, the process was let to accomplish as indicated by Bromo Phenol Blue’s descent to the bottom. The gel is then stained for 120 minutes with Commasie Brilliant Blue G-250 to highlight the protein band. After staining the gel three or four times until it appears clean, place it in a 10% glacial acetic acid solution, then in a press, and dry for 48 hours in a dark room.
3.3.1.3. Protein profile observation. The samples’ protein profiles were elucidated via sodium dodecyl sulfate polyacrylamide gel electrophoresis (SDS-PAGE) using Precision Plus Protein™ precast gels (Bio-Rad Laboratories, Inc., Singapore). The procedure was executed nearly in its entirety as described, with only the requisite adjustments (Arteaga et al., Citation2021; Buhl et al., Citation2019). The acrylamide contents in the electrophoresis gels used for SDS-PAGE evaluation were 4% and 12.5% for stacking gel and resolving gel, respectively. Firstly, a supernatant was mixed with a 1:1 buffer consists of 20% glycerol, 2% SDS, 20 mM Tris, and pyronin Y) addition and disulfide bond was reduced by addition of 0.2 M dithioerythritol (DTE) prior to heating at 100°C for 5 min. Then, each sample with concentration of 1 g/L was introduced into the gel wells. The electrophoresis was performed at a constant current of 20 mA and a voltage of 100 volt in a running buffer (0.1% SDS, 0.25 M Tris, and 0.192 M glycine) for 70 minutes. For enhanced visibility of certain protein bands, the gel was subsequently immersed for 60 minutes at room temperature in a staining solution comprising one gram of Coomasie Brillant Blue G-250 in a mixture of 450 mL methanol, 100 mL glacial acetic acid, and 450 mL water. The next step was to destain the gel immediately after it has been colored. The standard curve was employed to determine the molecular weight (MW) of the protein, which ranged from 2 to 250 kDa. The MW of the protein bands was predicted using known commercial MW marker (Precision Plus Protein™ Dual Xtra Prestained Protein Standards #1610377 Protein marker). The Rf value of a protein with a known MW is represented on a logarithmic correlation. Furthermore, the quantitative analysis of the amino acids present in the Snakehead FPC was determined by acid hydrolysis with a solution of hydrochloric acid, derivatization of amino acids with 6-aminoquinolyl-N-hydroxysuccinimidyl carbamate (AQC), and high-performance liquid chromatography (Alliance HPLC System). The details of the analytical procedures are the same to those described by Gam et al (Gam et al., Citation2005).
3.3.2. Microstructure of snakehead FPC granules
The microstructure of the snakehead FPC obtained from UAE was analyzed using a scanning electron microscope (SEM) (JEOLSeries, JSM-6510-LA, Japan) and according to the procedure described by previous researchers (Nawaz et al., Citation2020; Walayat et al., Citation2020). Briefly, the FPC samples were affixed on an aluminum slid, and were sputter-coated with 250 Å of gold. The samples were scanned with 10,000× magnification using a 15 kV accelerating voltage.
3.3.3. Statistical analysis
Each experiment and assay were carried out in triplicate. The mean value ± standard deviation (SD) was used to represent the average of the results obtained from each experiment and assay. One-way analysis of variance (ANOVA) was used to examine the significant differences between samples using Microsoft Excel (Microsoft Corp., Redmond, Wash., U.S.A.) and Minitab 19 with significance level 95%. Statistical interpretations of continuous variables were conducted based on the t-test with all p values were two-tailed. The t-test is a type statistical test to find out whether there is a significant difference between the estimated value and the statistical calculation result. The t-test is more versatile compared to other tests, since it can be used to test a one-sided alternative and is useful for testing those samples whose size is less than 30. A significance level of 95% (a p-value of < 0.05) was regarded as statistically significant throughout the analysis.
4. Results and Discussion
4.1. Proximate composition of snakehead fish flesh
Prior to the FPC manufacturing study, the flesh of snakehead fish was subjected to proximate composition analysis. The proximate composition analysis confirmed that the raw snakehead fish flesh used in this research comprised 24.05 % protein, 2.92 % total lipids, 1.88 % ash, 1.12% carbohydrate and 70.03 % water. It is clearly observed that the protein content of snakehead fish flesh is about 30 % higher than the protein content of snakehead fish flesh farmed in Bangladesh (18.24 %) reported by Paul et al (Paul et al., Citation2013). In addition, the protein content of the flesh of snakehead fish used in this work also higher than the protein content of snakehead fish farmed in Sulawesi (Indonesia) and Yogyakarta (Indonesia) were 19.60 ± 0.33% and 19.71 ± 0.28%, respectively (Chasanah et al., Citation2015; Nurfaidah et al., Citation2021). Furthermore, the wild Snakehead fish caught in East Java (Indonesia) and Papua (Indonesia) possessed 19.85 ± 0.59 % and 20.14% protein contents, respectively (Chasanah et al., Citation2015; Suwandi et al., Citation2014). Generally, feeding habit, age, sex, environmental condition, type of food and season significantly influence the chemical compositions and nutritional values of fish (Fawole et al., Citation2013). Wild Snakehead fishes that were caught during rainy season contained significantly higher protein compared to that were caught during the dry seasons because during rainy season food supply is much more plentiful for carnivorous fish species, such as the snakehead fish (Gam et al., Citation2006). Protein is an important food nutrient that plays roles as energy producer, building agent, and regulator in the body (Sun et al., Citation2006). However, the other nutrient contents are of comparable values. The result also agrees well with the general proximate composition of fish muscle tissue reported by Strzelczak et al. where they documented that fish muscle tissue bears around 70–84% water, 15–24% protein, 0.1–22% lipids, 1–2% minerals, and 2% non-protein nitrogenous fractions (Strzelczak et al., Citation2021)
4.2. Selection of solvent for ultrasound-assisted extraction
The solvent extraction method was chosen to manufacture FPC by which lipids and water are removed. Therefore, a safe and feasible solvent must be selected to facilitate lipids and water removal from the fish flesh. Based on the literature study, the total lipids in snakehead fish flesh comprise neutral lipids (72.9%) and followed by phospholipids (17.7%) and glycolipids (9.4%) (Rao et al., Citation2010). Considering their molecular structures, neutral lipids, and glycolipids are practically insoluble in water, but they are miscible in the non-polar or weakly polar organic solvents, including ether, benzene, chloroform, acetone, and ethanol (Gerde & White, Citation2008). However, other important nutrients, such as nucleic acids, amino acids, proteins, and carbohydrates are insoluble in non-polar organic solvents. Besides, phospholipids are completely soluble in both water and chloroform, ethanol, and hexane containing 3% ethanol because they have hydrophobic hydrocarbon tails of their two fatty acids and a hydrophilic phosphate group end Yang, & Hu, Citation2014. Nonetheless, the solubility of phospholipids in water is also dependent upon both the type of the functional group located at the polar head and the hydrocarbon chain length. Therefore, three different solvents were picked in this research, namely water, ethanol, hexane and a mixture of hexane containing 3% (v/v) of ethanol (Chasanah et al., Citation2015). A set of experiment was performed by extracting snakehead fish flesh in a UAE system using the aforementioned solvents employed ultrasonic power of 200 W at 40°C and 6:1 v/m (liquid solvent to solid feed ratio) for 25 minutes (Ali et al., Citation2019).
Table clearly demonstrates the superiority of ethanol in extracting lipids and water from the flesh of snakehead fish over other solvents. The ultrasound assisted extraction is targeted to remove water and lipids of the minced Snakehead fish flesh, therefore the mass of the final remaining solid upon lipids and water removal was lower compared to other solvents. This is because more water and lipids have been removed during extraction and vaporized during vacuum drying. As a result, the FPC yield when ethanol was used as the solvent is lower compared to those obtained from extraction using other solvents. In addition, FPC with the highest protein content and the lowest lipid content was attained when ethanol was employed as the extracting medium. Although fats are also essential food nutrients that provide energy, the high fats content of FPC product will hasten the development of rancid and fishy odors. A lower fat content value indicates a higher quality of FPC, because of the FPC will have a lower tendency of fat oxidation or microbial decomposition that produce rancidity (Asfar et al., Citation2014). Table also shows that the ash and carbohydrate content in the FPCs were comparable, regardless the solvent type used. This result is consistent with the findings of Rao et al., who discovered that the total lipids of snakehead fish flesh are dominated by neutral lipids (72.9%) and followed by phospholipids (17.7%) and glycolipids (9.4%) (Rao et al., Citation2010). Out of all the solvents, ethanol is the preferred one for total lipids and water extraction. It is proven that ethanol has an excellent ability to dissolve water, neutral lipids, glycolipids and phospholipids due to its highest affinity to these biomolecules. In addition, ethanol can also dissolve certain type of carbohydrates. As expected, hexane dissolves more neutral lipids than glycolipids and phospholipids from the snakehead fish flesh. Nevertheless, hexane cannot dissolve water because it is a non-polar solvent. Despite water successfully dissolves phospholipids and some carbohydrates, as a polar solvent it cannot dissolve neutral lipids and glycolipids (Gerde & White, Citation2008). Apparently, mixing of hexane with ethanol enhances its polarity (Batista et al., Citation2015; Kumoro et al., Citation2009). Therefore, a mixture of hexane containing 3% v/v ethanol has been proven to be the better solvent than both water and hexane due to its improved ability to dissolve both polar and non-polar substances in the snakehead fish flesh. In addition, ethanol also possesses many advantages compared to other solvents because it is inexpensive, readily available in the market, generally recognized as safe (GRAS) status for food applications, and can be prepared from various botanical sources using uncomplicated technologies (Ferreira-Dias et al., Citation2003; Ramić et al., Citation2015). As expected, the protein content of the snakehead FPC obtained in this work is higher than that reported in previous study (58.77% to 64.01%) (Kumoro et al., Citation2022). Based on the above-mentioned findings, ethanol is selected as the solvent for the manufacture of FPC from snakehead fish flesh through lipids and water removal.
Table 1. Effect of solvent type on the proximate composition of snakehead FPC
4.3. Determination of ultrasound-assisted extraction time
The optimum extraction time required for UAE was determined by extracting snakehead fish flesh using HPLC grade ethanol at 40°C, 6:1 v/m (liquid solvent to solid feed ratio or LSSFR), microwave power of 200 W and different extraction time, namely 0, 10, 15, 20, 25, and 30 minutes. The FPCs obtained were subjected to proximate analysis to evaluate their nutritional value. Table summarizes the proximate composition of the FPCs derived from snakehead fish obtained from UAE at various extraction times. Extraction at 0 minute represented the minced snakehead fish flesh without exposure to ultrasonic irradiation (un-sonicated minced fish flesh).
Table 2. Effect of UAE time on the proximate composition of snakehead FPC
As seen in Table , the protein content of the un-sonicated minced snakehead fish flesh was 58.64%. Meanwhile, the lipid and water contents were 1.38% and 11.49%, respectively. This information suggests that snakehead fish flesh possesses a remarkable prospect of the raw material for the manufacture of FPC. The ultrasound assisted extraction is intended to remove water and lipids from the minced Snakehead fish flesh, therefore the mass of the final remaining solid upon lipids and water removal was lower when extraction was carried out for a longer duration. This is because more water and lipids have been removed during extraction of the minced Snakehead fish flesh. As a result, the FPC yield decreased when extraction was carried out for a longer duration. In general, the increase in extraction time of up to 25 minutes resulted in a higher protein content and lower lipid and water contents. Certainly, the increase in sonication time provided a longer duration for cavitation process to occur in the extraction system (Abdullah et al., Citation2010). In addition, an enhanced exposure of extracting solvent to fish flesh and prolonged cavitation process may induce more fragmentations, significant cell wall rupture, pore formation and shearing in the cellular matrix of fish flesh tissues that led to ease the release of oil and water into the solvent (Mehta et al., Citation2022). As a consequence, more amount of oil and water could be removed from the fish flesh and subsequently enhanced the protein yields.
Table also presents that the snakehead FPC obtained from UAE for 25 minutes contains the highest protein concentration of 73.17% with the lowest water and fat contents, which were 7.25% and 0.73%, respectively. However, beyond 25 minutes, the UAE resulted in a reduction of extraction yield because by virtue the extraction time also possesses a threshold value (Mehta et al., Citation2022). However, as seen in Table , the increase in FPC yield upon extraction for 30 minutes was likely due to the increase in water content. In fact, the protein content of the FPC obtained from UAE for 25 and 30 minutes were not significantly different. Beyond that extraction time threshold, many cavitational bubble clouds can be developed, which dwindle the overall performance of a UAE system (Kentish et al., Citation2008). Actually, the behavior of droplet development with time is a better measure of extraction time, which also controls cavitation behavior. The sonication time also influences the temperature of the whole system as the collapsing bubbles will instantly cause a drastic increment of local temperature that can achieve a thousand degrees Celsius (Canselier et al., Citation2002). As a result, the prolonged exposure to ultrasonication may lead to the occurrence of local overheating of the fish flesh sample and thereafter induce structural destruction or denaturation of the targeted proteins (Santos et al., Citation2009). Considering the energy requirement and the facts that the quality of the FPC is mainly determined by the protein, lipid and water content (Sen, Citation2005), the extraction time of 25 minutes was selected as the optimum time for UAE extraction and was further used in all experiments for the manufacture of snakehead fish protein concentrate. The optimum UAE time obtained in this work is shorter than that reported by Abdullah et al. to extract oil from Monopterus albus, which was 60 minutes (Abdullah et al., Citation2010). Recently, Çavdar et al. found the optimum time needed for oil extraction from gilthead seabream (Sparus aurata) using UAE was 38 minutes (Çavdar et al., Citation2023). However, the optimum UAE time obtained in this work is slightly longer than that reported by Ali et al., who successfully extracted squalene from a spot-tail shark liver using UAE in just 15 minutes (Ali et al., Citation2019).
4.4. Effect of liquid solvent to solid feed ratio
Another important parameter that potentially affects the UAE performance is the liquid solvent to solid feed ratio (LSSFR), which can be defined as the ratio between the volume of the liquid solvent and mass of the solid feed used for extraction. To obtain the optimum LSSFR, a set of UAE experiment was performed using ethanol as the extracting medium at 40°C for 25 minutes at various LSSFR values ranged from 1 to 7. The results are presented in Table .
Table 3. Effect of LSSFR on the proximate composition of snakehead FPC
Identical to the trend of the extraction time, the protein extract (FPC) yield of UAE also increases with the rise in LSSFR up to a maximum value and declines thereafter. As seen in Table , the protein content of the FPCs increased as the LSSFR values were increased up to 6. However, as the LSSFR value was further increased to 7, the protein content of the FPCs slightly declined. Although the lipid content of the FPCs remains unchanged (0.73%), the water content increases due to more residual water upon vacuum drying.
The LSSFR value determines the degree of mixing, which is enhanced when the sound waves produced a vigorous turbulence in the extracting medium and promoted the convective mass transfer during the extraction process, resulting in a higher extraction yield (Chemat et al., Citation2017). A lower LSSFR value leads to a higher viscosity of the mixture and the cavitation effect is somewhat burdensome due to more cohesion tendency between particles of the liquid solvent. In contrast, an increasing LSSFR value leads to a decrease in the viscosity of the extraction medium. A low viscosity extraction medium causes an appreciable cavitation effect that gives rise to more advanced fragmentation and sonoporation of the solid material. Eventually, these situations result in higher extraction yields. However, after a certain limit, this increase in LSSFR causes an excessive cavitation enhancement resulting in the degradation of the extracted proteins, and thus reducing the extraction yield (Maran et al., Citation2015).
Based on the protein, lipid, and water contents of the FPCs shown in Table , the optimum LSSFR value for the UAE of snakehead protein is 6:1. Ali et al. also reported the same optimum value of LSSFR (6:1) for UAE of squalene from various fish livers (Ali et al., Citation2019).
4.5. Effect of temperature
Considering that temperature can affect the physical properties of the solvent, in particular the density, viscosity, and surface tension, that influence of temperature on the UAE performance was studied at temperatures ranged from 30 to 60°C. The UAE was conducted using ethanol as the extracting solvent at LSSFR value of 6 for 25 minutes. The results are summarized in Table .
Table 4. Effect of temperature on the proximate composition of snakehead FPC
As seen in Table , the effect of temperature on the FPC yield and quality was also found to be similar to that of extraction time. Table shows that with an increase in temperature, protein yield increases initially but decreases eventually. Therefore, the optimum temperature for UAE of lipids and water for FPC manufacturing studied in this research is 40°C. Refer to the statistical analysis tabulated in Table , both total FPC yield and protein content at 40°C and 50°C were not significantly different. The reasons of choosing 40°C as the best extraction temperature were based on the fact that the energy required to maintain UAE at 50°C is definitely higher than at 40°C. In addition, the moisture content of the resulted FPC at 50°C is higher than that obtained from UAE at 40°C, which is not preferred. Ali et al (Ali et al., Citation2019). also found that the maximum unsaponifiable yield of spot-tail shark liver using the ultrasound-assisted direct in situ saponification (U-DS) process was obtained at an amplitude of 80% and a temperature of 40°C. They concluded that the drastic increase in yield could be caused by the intense effect of cavitation, which enhanced mass transfer rate subsequently expedited the release of the intended components (Peshkovsky et al., Citation2013). A similar observation has been reported during the UAE of phenolic compound from spent coffee grounds where the yield increased with the increase in temperature from 30 to 45°C, and the yield began to decline beyond 45°C (Al-Dhabi et al., Citation2017). In addition, the oil yield was found to increase gradually with increase in temperature up to 40°C, whereas beyond 40°C there was no significant increment on oil yield during the UAE of vegetable oil from flaxseed (Gutte et al., Citation2015).
In fact, elevated temperature provides a dual effect on both the solute and solvent (Jafari et al., Citation2008). As the temperature decreases, the solvent’s vapor pressure also decreases. As the temperature of the solvent rose, so did its vapor pressure, which had a significant impact on both the occurrence and intensity of acoustic cavitation. A small number of cavitational droplets are generated by ultrasound due to its high acoustic cavitation threshold. However, the relatively greater force with which the bubbles burst during extraction increased the disruption of cell tissues. Higher temperatures cause reduction of viscosity of the solvent, which ensures increased solvent penetration into the tissue matrix. Moreover, an increase in temperature can also be expected to enhance the solubility of the targeted solute in the solvent (Ramić et al., Citation2015; Yang & Hu, Citation2014). However, once the maximum extraction yield has been reached, an increase in extraction temperature induces mild cavitation, resulting in a diminished extraction yield (Kentish et al., Citation2008). Another factor that could be implicated is the correlation between the rise in extraction temperature and the reduction in surface tension of the solvent, which impacts the formation and collapse of bubbles. Increased temperature resulted in elevated vapor pressure and increased bubble formation; however, the bubbles collapsed with diminished intensity owing to a reduced pressure gradient between their interior and exterior (Hromádková et al., Citation1999). At elevated temperatures, the bubbles may undergo rapid collapse, thereby diminishing the magnitude of the mass transfer mechanism (Gutte et al., Citation2015).
Furthermore, the increase in the extraction temperature also expedites overall protein denaturation in fish flesh. Average denaturation temperatures (Td) of fish muscle proteins are reported to be 43.45°C, 56.79°C, 69.01°C, and 77.9°C for myosin, sarcoplasm, connective tissue, and actin respectively (Larrea-Wachtendorff et al., Citation2015; Strzelczak et al., Citation2021). During heating, fish flesh can suffer from both textural alterations and muscle fiber shrinkage, specifically induced by thermal denaturation of muscle proteins, such as actin, myosin, and collagen (Ishiwatari et al., Citation2013; Ovissipour et al., Citation2017).
4.6. Effect of ultrasonic power
In order to attain cavitation threshold, a minimum ultrasonic intensity (UI) value is required. The value of UI can be estimated from the power delivered to the media through the emitting surface of the transducer. In general, the increase in UI leads to increase the sonochemical or cavitation effects (Chemat et al., Citation2017). However, because the shape of the minced snakehead fish flesh is irregular and its contact area cannot be correctly determined. Therefore, to study the effect of ultrasonic intensity, a set of UAE experiment was conducted using ethanol as the extracting medium at LSSFR value of 6 at 40°C for 25 minutes by varying ultrasonic power from 100 to 400 W.
As seen in Table , protein content in the FPC from snakehead fish flesh enhanced as the ultrasonic power increased from 100 to 200 W, which can be elaborated in terms of cavitational effects induced by the introduction of the ultrasonic waves. Cavitation typically takes place within a liquid medium when subjected to abrupt and variable high pressure. When the pressure differential between the amplitude pressure of ultrasonic waves and the hydrostatic pressure in the liquid medium exceeds the local tensile strength of the liquid extracting medium, void spaces filled with minuscule microbubbles form. These bubbles undergo inflation during the negative phase of the pressure cycle or rarefaction cycle, attain their maximal radius, and subsequently collapse as the positive pressure cycle or compression cycle commences (Abdullah et al., Citation2010). Indeed, the bubble collapse may result in stronger shear forces that ultimately cause severe micro ruptures of the biological tissues (Vardanega et al., Citation2014). Eventually, this condition will facilitate the penetration of lipids or oil to be released from the snakehead fish flesh.
Table 5. Effect of ultrasonic power on the proximate composition of snakehead FPC
However, as the ultrasonic power increased exceeded 200 W, protein content in the FPCs decreased. This could be attributed to the generation of numerous cavitation microbubbles with energies exceeding 200 W. The collision propensity of cavitation bubbles is enhanced due to the presence of a multitude of them within the liquid medium. At levels of ultrasonic power exceeding 200 W, larger microbubbles are generated upon collision. Undoubtedly, the lack of adequate time for the bubbles to disintegrate results in the development of a bubble cushion on the ultrasonic transducer’s radiating surface. Consequently, this circumstance leads to a diminished transfer of ultrasonic energy to the solvent, thereby minimizing cavitational effects. In due course, the formation of microfractures in the flesh tissues of fish is reduced. This phenomenon provides an explanation for the observed decrease in protein yields with increasing ultrasonic power exceed 200 W.
Table shows that based on the protein and lipid contents; the optimum ultrasonic power suitable for FPC manufacturing from snakehead fish flesh is 200 W. The finding is in accordance with optimum ultrasonic power suitable for extraction of oil from eel (Monopterus albus) flesh reported by Abdullah et al (Abdullah et al., Citation2010).
4.7. Characteristics of snakehead fish protein concentrate
The Food and Agriculture Organization categorizes FPC into different types, with type A being an odorless, tasteless, and colorless FPC. The protein content of this FPC must be at least 67.6%, fat content must be at maximum of 0.75%, and water content must be no higher than 10%. The type B FPC is an FPC that lacks details, such as smell, taste, and color, but when added to meal products, it still retains the taste of fish. To achieve those characteristics, the protein content of this FPC should be at least 65%, whereas the fat and water contents should be no more than 3% and 10%, respectively. Meanwhile, the Type C FPC is a hygienic fish concentrate with a protein content of no less than 60%, but the maximum value of both fat and water contents should be 10%. Hence, the Type C FPC still retains the smell and flavor of fish (Chemat et al., Citation2017). Taking into account of the protein (73.17 %), fat (0.73 %) and water content (7.25 %), the FPC obtained from UAE of snakehead fish flesh using ethanol with LSSFR of 6 at 40°C and ultrasonic power of 200 W for 25 minutes can be categorized as type A FPC.
Figure shows the physical appearance of the best quality snakehead FPC obtained from this work from UAE using ethanol as solvent at 40°C for 25 minutes, which can be classified as the type A FPC that contains 73.17% protein, 0.73% fat, white in color and has no fishy odor. Another FPC preparation study was reported by Riewpassa & Cahyono where a type B FPC was successfully prepared from Sunglir fish through conventional extraction method, with ethanol solvent using the soaking method for 3 hours with a relatively high protein content (77.34%), a fat content of 3%, but still bears distinct fish aroma (Rieuwpassa & Cahyono, Citation2019). Meanwhile, the type C FPC was produced from Tilapia fish by immersion of Tilapia fish flesh in ethanol for 24 hours. The FPC contains 61.13% protein, 7.11% fat and 11% water, which resembled fish meat powder and strong fishy odor (Rieuwpassa et al., Citation2020). Figure demonstrates the microstructural appearance of snakehead FPC granules obtained from conventional solvent extraction that resemble the agglomerates of porous polyhedral granules of approximately 8 µm diameter with sprinkling fat particles almost evenly distributed on the top layer. Meanwhile, Figure presents the microstructure image of snakehead FPC granules that resemble incompact porous polyhedral granules of approximately 2 µm diameter. Hence, they have a higher tendency of being broken down in to smaller granules. The morphology, microstructure and size of FPC granules will affect the final processed products because granule size will influence water and fat absorption capacity. In general, proteins with a porous smaller granule size and lower density have the capacity to absorb and imbibe more water and oil than those rigid protein granules with higher density.
4.8. Protein profiles of the Snakehead FPC
Proteins consist of amino acids, which number in the hundreds or thousands (Çavdar et al., Citation2023). The protein profile of the resulting snakehead FPC obtained from UAE using ethanol was characterized based on their MW obtained from analysis using the SDS-PAGE electrophoresis method (Roy et al., Citation2012). The Snakehead FPC obtained from conventional extraction was not subjected to SDS-PAGE analysis because it was obtained from extraction using ethanol as solvent using the same extraction condition with the selected condition used for UAE. Hence, the resulting FPC from conventional extraction should have the same amino acids profile with those obtained from UAE. The protein composition examination using the electrophoresis technique developed 12 protein bands that appear on the gel demonstrated in Figure . The difference in the thickness and thinness of the bands formed is due to differences in the number of migrated molecules, whereas the thick bands are the result of the electrophoresis process fixation of several bands. The pattern of the formed bands can be used to perform MW estimation on each band using the Rf formula, which is then used to determine the types of protein (Edvotek, Citation2011).
Figure 2. The results of electrophoresis (SDS–PAGE) show the FPC profile of snakehead fish using the UAE method. Lanes 1–4 represent molecular weight, 20 µL of sample protein, 15 µL of sample protein, and 10 µL of sample protein markers, respectively.

Based on the SDS-PAGE electrophoresis test, the banding pattern of the snakehead FPC showed the presence of 12 types of proteins with MWs ranging from 4.00 kDa to 174.00 kDa, namely aprotinin, parvalbumin, alpha-lactalbumin, lysozyme, myoglobin, trypsin inhibitors, glutamate dehydrogenase, bovine serum albumin (BSA), paramyosin, α-Actinin, myosin VI and maltose-binding protein or MBP-β-galactosidase. The result presents more types of protein than that previously reported by Haniffa et al. who found only 7 proteins in C. striatus farmed in Iran (Haniffa et al., Citation2017). This is thought to be due to differences in the treatment, type of solvent, and methods used in the production of FPC, which affects the amino acid profile of the FPC. As seen in Figure , the major fractions of the snakehead FPC were small peptide compounds with MWs ranges from 4.00 kDa to 11.00 kDa, whereas the minor ones were large MW peptide fractions with MW between 71.70 kDa to 174.00 kDa.
The fractions with MW between 1 to 4 kDa are particularly interesting for nutritional and pharmaceuticals uses (Opheim et al., Citation2015). Additionally, protein hydrolysates from turbot viscera showed the highest antioxidant and antihypertensive activities, which could possibly be associated to the existence of the highest percentage of > 1 kDa peptides. Previously, Miyashita et al. reported that peptides of shark, salmon, herring roes, and trout with MW of < 5.00 kDa demonstrate excellent antioxidant activity. Then, they further came to the conclusion that roe protein concentrates obtained from solvent extraction process using isopropyl alcohol comprised of both high and low MW proteins, suggesting that solvent treatment had a negligible effect on the nature of the peptides during the manufacture of roe protein concentrate (Guedes et al., Citation2022). Najafian and Babji revealed that peptides with antimicrobial activity are commonly hydrophobic and are composed of < 50 amino acids in length with MW < 10 kDa (Najafian & Babji, Citation2012). Low MW fractions from tuna by-products were also observed to demonstrate excellent antimicrobial activity compared with the larger MW fractions (Heffernan et al., Citation2021; Pezeshk et al., Citation2019). Low MW protein fractions (5–10 kDa) rich in histidine obtained from red lionfish protein demonstrate high antioxidant activity and strong binding affinity to copper ions, thereby preventing copper toxicity Chel-Guerrero et al. (Citation2020). Galla et al. reported that protein concentrate obtained from Channa striatus containing peptides with ~ 30 kDa MW exhibited higher reducing power than that derived from Lates calcarifer (Fukuda & Hasegawa, Citation2014). For example, low MW peptides derived from catfish protein isolate exhibited higher value of oxygen radicals’ absorbance capacity (ORAC) and metal-chelating activities. Meanwhile, the high MW peptides demonstrated higher Ferric-reducing antioxidant power (FRAP) and DPPH radical scavenging (Theodore et al., Citation2008). Fukuda and Hasegawa explained that ~ 90 kDa protein shows protease activity and was the most active at 60°C under alkali condition (Fukuda & Hasegawa, Citation2014). Additionally, Chobert et al. observed that smaller peptides possess higher solubility than the higher MW proteins (Chobert et al., Citation1988). Gauthier et al. stated that functional properties of protein, i.e., solubility, and hydrophobicity simulate emulsifying properties (Gauthier et al., Citation1993). Based on their investigation, Bhaskar et al. confirmed that highly nutritious fish proteins should contain a high content of low MW peptides. Hence, a successful mass production of such desirable peptides showed their potential in various food applications (Bhaskar & Mahendrakar, Citation2008).
The three main groups of muscle proteins, which vary in solubility and functional properties are myofibrillar (50–60%), sarcoplasmic (30%), and stromal (10–20%) proteins (Dekic et al., Citation2016). Sarcoplasmic and myofibrillar proteins are the main proteins of fish muscle observed in this study, whereas only a smaller number of stromal proteins were identified (Windsor, Citation2022). A previous study proved that actin works for muscle contraction, helps to heal wound-injured, bone builder, and skin (Tottoli et al., Citation2020). In addition, albumin is the plentiful plasma protein in the human body for about 50–65% (3.5 to 5 g/L), and exhibits some essential functions for health, specifically the formation of new cell tissue to promote the recovery of damaged body tissue (Ali et al., Citation2019; Larrea-Wachtendorff et al., Citation2015). Fish sarcoplasmic proteins (SP) are a large family of proteins that include myogens (myoglobin and globulin), and are soluble in water as well as low ionic strength solution (Rocha et al., Citation2017). In addition, they are also composed of enzymes associated with energy-producing metabolism, such as glycolysis and citrate cycle (Gao et al., Citation2021).
The amino acids evaluation method employed in this work only enabled the analysis of 17 amino acids (Gam et al., Citation2005). As a consequence, asparagines and glutamine were hydrolyzed into aspartic acid and glutamic acid during acid hydrolysis. Therefore, the reported aspartic acid content denotes the total content of asparagines and aspartic acid, and the same is also applicable to glutamic acid. Because tryptophan is totally degraded at the time of acid hydrolysis, thus it was not identified in this work. The most abundant amino acids in Snakehead fish (in reducing order) were glutamic acid, aspartic acid, lysine, arginine, leucine, alanine, glycine, threonine, valine, isoleucine, serine, and phenylalanine. the amounts of tyrosine, proline, methionine, histidine, and cysteine were considerably diminished. The data unveiled indicate that the essential amino acids constituted over 50% of the overall amino acid composition of Snakehead fish. Histidine, isoleucine, leucine, lysine, methionine, phenylalanine, threonine, tryptophan, and valine are the nine essential amino acids (Tessari et al., Citation2016).
Concerning its utilization as a remedy, Snakehead fish is observed to be rich in arginine, an amino acid that is responsible for wound healing. In addition, other amino acids that are reported to be beneficial in wound healing (valine, alanine, glycine, proline, and histidine) were also present in Snakehead fish, but at relatively lower quantity. The high quantity of arginine is crucial because it functions various roles in the system of wound healing. Arginine energizes the liberate of hormones predominant to wound healing, regulate endothelial function, and immune function (Arribas-López et al., Citation2021). An excess of dietary arginine beyond the quantities necessary for optimal growth, nitrogen balance, or overall health in healthy rodents and humans results in an accumulation of wound collagen (Williams et al., Citation2002). Additionally, arginine is an important feed for two synergistic enzymes to wound healing, which are nitric oxide synthetase (NOS) and arginase. Arginine is classified as a semi-essential amino acid in the diet, but it becomes conditionally indispensable in the presence of severe trauma and critical illness (Williams et al., Citation2002). Eventually, in the course of connective tissue formation, arginine is utilized to produce hydroxyproline, which is essential in collagen formation because normally it carries around 9.1% of the total amino acid residues in the collagen sequence (Albina et al., Citation1990). Moreover, the mass percentage ratio of the essential to non-essential amino acids was 1:30 suggesting that Snakehead fish could bear high quality proteins or well-balanced protein content as the primary source of amino acids.
4.9. Comparison of UAE and conventional extraction
Because extracting fish protein to manufacture its concentrated form using conventional solvent extraction method has been reported to suffer from numerous drawbacks, a new technology was combined with the conventional extraction method to obtain better results. In this work, ultrasonic wave was employed to assist the conventional solvent extraction method and regarded as the UAE. The UAE method has been reported to be more effective than the conventional method for isolating the target compounds (Rouhani et al., Citation2009). For comparison purpose, the protein and fat contents of snakehead fish protein concentrates obtained from both conventional solvent extraction and UAE methods carried out using ethanol as solvent, mass ethanol volume to minced snakehead fish flesh ratio of 6:1 at 40°C for 25 minutes are elaborated.
As expected, the UAE manufacturing method successfully produced FPC with a higher protein content (73.17 %) than the conventional method, which resulted in FPC with the protein content was only 58.64%. This is because UAE process allowed better removal of fat from the minced fish flesh that resulted in a lower residual fat content in the snakehead FPC. The residual fat content of FPC obtained from UAE process is 0.73 %, whereas the residual fat content of the FPC prepared using the conventional extraction method is 4.26 %. Indeed, the results confirm the existing literature that shows superior performance of UAE method over the conventional solvent extraction method in terms of yield, energy efficiency, investment, and operational cost, solvent volume, extraction time, and potential degradation of the targeted substances (Chemat et al., Citation2017; Silva et al., Citation2020). These facts are achieved as the results of the cavitation effect during UAE process (Chemat et al., Citation2017). Acoustic cavitation is basically a sequential stage that includes formation, growth, and rupture of microbubbles caused by successive bubble expansion and compression cycles. This situation results in internal pressure between the surface of the liquid and the solids that leads to the rupture of the sample’s cell wall. Accordingly, the sample’s ruptured walls allow the solvent to intensively penetrate the raw material and promote the release of the target compounds, which in turn increases the extraction efficiency (Gauthier et al., Citation1993).
5. Conclusions
The UAE method resulted in FPC from minced Snakehead fish with a higher protein content (73.17%) than the conventional solvent extraction method, which only reached a maximum protein content of 65.77%. The best operating condition for the UAE method was using mass ethanol volume to minced snakehead fish flesh ratio of 6:1 at 40°C employing 200 W ultrasound power for 25 minutes and drying at 55°C under reduced pressure (0.05MPa). Snakehead FPC can be classified as type A fish protein concentrate, which is white in color with no fishy odor and contains 73.17% protein, 0.73% fat, and 7.36 % water. Based on the SDS-PAGE electrophoresis, the FPC comprises mostly myofibrillar and sarcoplasmic proteins with a lower number of stromal proteins, with MWs ranging from 4.00 kDa to 174.00 kDa, including aprotinin, parvalbumin, alpha-lactalbumin, lysozyme, myoglobin, trypsin inhibitors, glutamate dehydrogenase, BSA, paramyosin, α-actinin, myosin VI and maltose-binding protein or MBP-β-galactosidase. Hence, the FPC possesses great potential for food applications with more affordable price compared to other animal-derived proteins.
Correction
This article has been corrected with minor changes. These changes do not impact the academic content of the article.
Acknowledgments
No second or third party are required to be acknowledged.
Disclosure statement
No potential conflict of interest was reported by the author(s).
Data availability statement
If required, the data of this research will be available to readers upon direct request to the corresponding author: Andri Cahyo Kumoro through [email protected].
Additional information
Funding
Notes on contributors

Andri Cahyo Kumoro
Andri Cahyo Kumoro, PhD is an active full professor in the Department of Chemical Engineering, Faculty of Engineering, Universitas Diponegoro. Jl. Prof. H. Soedarto, SH Semarang, Indonesia 50275. He is the founder of the Institute of Food and Remedies Biomaterials (InFaRMa) at Universitas Diponegoro. His main research interest is in the conversion of biomass (plant, animal and household) residues into fuel, food, nutraceuticals and other useful monomeric and polymeric biomaterials via implementation of bioprocess and separation technologies.
References
- Abdullah, S., Mudalip, S. K. A. A., Shaarani, S. M., & Pi, N. A. C. (2010). Ultrasonic extraction of oil from Monopterus albus: Effects of different ultrasonic power, solvent volume and sonication time. Journal of Applied Sciences, 10(21), 2713–23. https://doi.org/10.3923/jas.2010.2713.2716
- Akhade, A. R., Koli, J. M., Sadawarte, R. K., & Akhade, R. R. (2016). Functional properties of fish protein concentrate extracted from ribbon fish, lepturacanthus savala by different methods. International Journal of Processing and Postharvest Technology, 7(2), 274–283. https://doi.org/10.15740/HAS/IJPPHT/7.2/274-283
- Albina, J. E., Mills, C. D., & Henry, W. L., Jr. (1990). Temporal expression of different pathways of L-arginine in healing wounds. Journal of Immunology, 144(10), 3877–3880. https://doi.org/10.4049/jimmunol.144.10.3877
- Al-Dhabi, N. A., Ponmurugan, K., & Jeganathan, P. M. (2017). Development and validation of ultrasound-assisted solid-liquid extraction of phenolic compounds from waste spent coffee grounds. Ultrasonics Sonochemistry, 34, 206–213. https://doi.org/10.1016/j.ultsonch.2016.05.005
- Ali, A. M. M., Bavisetty, S. C. B., Prodpran, T., & Benjakul, S. (2019). Squalene from fish livers extracted by ultrasound-assisted direct in situ saponification: Purification and molecular characteristics, “journal of the American oil Chemists. Journal of the American Oil Chemists’ Society, 96(9), 1059–1071. https://doi.org/10.1002/aocs.12262
- Amoo, I. A., Adebayo, O. T., & Oyeleye, A. O. (2016). Chemical evaluation of winged beans (psophocarpus Tetragonolobus), Pitanga cherries (eugenia uniflora) and orchid fruit (orchid fruit myristica). African Journal of Food Agriculture Nutrition & Development, 6(2), 1–12. https://doi.org/10.4314/ajfand.v6i2.71734
- AOAC, Association of Official Analytical Chemists. (2012). Official methods of analysis of AOAC International. In Latimer, G. W. (Ed.), Official Journal of the European Communities (19 th ed., Vol. II, pp. 18–19). AOAC International.
- Arribas-López, E., Zand, N., Ojo, O., Snowden, M. J., & Kochhar, T. (2021). The Effect of amino acids on wound healing: A systematic review and meta-analysis on arginine and glutamine. Nutrients, 13(8), 2498. https://doi.org/10.3390/nu13082498
- Arteaga, V. G., Kraus, S., Schott, M., Muranyi, I., Schweiggert-Weisz, U., & Eisner, P. (2021). Screening of twelve pea (pisum sativum L.) cultivars and their isolates focusing on the protein characterization, functionality, and sensory profiles. Foods, 10(4), 758. https://doi.org/10.3390/foods10040758
- Asfar, M., Tawali, A. B., Abdullah, N., & Mahendradatta, M. (2014). Extraction of albumin of snakehead fish (Channa Striatus) in producing the fish protein concentrate (FPC). International Journal of Scientific & Technology Research, 3(4), 85–88.
- Balachandran, S., Kentish, E., Mawson, R., & Ashokkumar, M. (2006). Ultrasonic enhancement of the supercritical extraction from ginger. Ultrasonics Sonochemistry, 13(6), 471–479. https://doi.org/10.1016/j.ultsonch.2005.11.006
- Batista, M. M., Guirardello, R., & Krähenbühl, M. A. (2015). Determination of the Hansen solubility parameters of vegetable oils, biodiesel, diesel, and biodiesel–diesel blends. Journal of the American Oil Chemists’ Society, 92(1), 95109. https://doi.org/10.1007/s11746-014-2575-2
- Bhaskar, N. B. N. S., & Mahendrakar, N. S. (2008). Protein hydrolysate from visceral waste proteins of Catla (Catla catla): Optimization of hydrolysis conditions for a commercial neutral protease. Bioresource Technology, 99(10), 4105–4111. https://doi.org/10.1016/j.biortech.2007.09.006
- Bing, L., Akber, A. H., & Abulimiti, Y. (2019). Optimization of ultrasound-assisted extraction of sheep abomasum protein concentrates by response surface methodology and evaluation of their properties. Food Science and Technology Campinas, 39(Suppl. 1), 85–92. https://doi.org/10.1590/fst.37317
- Buhl, T. F., Christensen, C. H., & Hammershøj, M. (2019). Aquafaba as an egg white substitute in food foams and emulsions: Protein composition and functional behavior. Food Hydrocolloids, 96, 354–364. https://doi.org/10.1016/j.foodhyd.2019.05.041
- Campo-Deano, L., Tovar, C. A., & Borderias, J. (2010). Effect of several cryoprotectants on the physicochemical and rheological properties of suwari gels from frozen squid surimi made by two methods. Journal of Food Engineering, 97(4), 457–464. https://doi.org/10.1016/j.jfoodeng.2009.11.001
- Canselier, J. P., Delmas, H., Wilhelm, A. M., & Abismaïl, B. (2002). Ultrasound emulsification—an overview. Journal of Dispersion Science and Technology, 23(1–3), 333–349. https://doi.org/10.1080/01932690208984209
- Çavdar, H. K., Bilgin, H., Fadıloğlu, S., & Yılmaz, F. M. (2023). Ultrasound- and microwave-assisted extraction facilitate oil recovery from gilthead seabream (sparus aurata) by-products and enhance fish oil quality parameters. European Journal of Lipid Science and Technology, 125(1). https://doi.org/10.1002/ejlt.202200089
- Chasanah, E., Nurilmala, M., Purnamasari, A. R., & Fithriani, D. (2015). Chemical composition, albumin content and bioactivity of crude protein extract of native and cultured Channa striata. Jurnal Pascapanen dan Bioteknologi Kelautan dan Perikanan, 10(2), 123–132. https://doi.org/10.15578/jpbkp.v10i2.364
- Chel-Guerrero, L., Estrella-Mill´an, Y., Betancur-Ancona, D., Aranda-González, I., Castellanos-Ruelas, A., & Gallegos-Tintoré, S.(2020). Antioxidant, chelating, and angiotensin-converting enzyme inhibitory activities of peptide fractions from red lionfish (pterois volitans L.) muscle protein hydrolysates. International Food Research Journal, 27(2), 224–233.
- Chemat, F., Rombaut, N., Meullemiestre, A., Turk, M., Perino, S., Fabiano-Tixier, A.-S., & Abert-Vian, M. (2017). Review of green food processing techniques. Preservation, transformation, and extraction. Innovative Food Science & Emerging Technologies, 41, 357–377. https://doi.org/10.1016/j.ifset.2017.04.016
- Chemat, F., Rombaut, N., Sicaire, A. G., Meullemiestre, A., Fabiano-Tixier, A.-S., & Abert-Vian, M. (2017). Ultrasound assisted extraction of food and natural products. Mechanisms, techniques, combinations, protocols and applications. A review. Ultrasonics Sonochemistry, 34, 540–560. https://doi.org/10.1016/j.ultsonch.2016.06.035
- Chobert, J. M., Bertrand, H. C., & Nicolas, M. G. (1988). Solubility and emulsifying properties of caseins and whey proteins modified enzymatically by trypsin. Journal of Agricultural and Food Chemistry, 36(5), 883–889. https://doi.org/10.1021/jf00083a002
- Dekic, R., Friščić, J., Ivanc, A., & Kukavica, B. (2016). Characterization of proteins from popovo minnow (Delminichthys ghetaldii Steindachner, 1882) muscle. Turkish Journal of Fisheries and Aquatic Sciences, 16(3), 637–642. https://doi.org/10.4194/1303-2712-v16_3_17
- Edvotek, A. (2011). Purification & size determinationof green (gfp) & Blue (bfp) fluorescent proteins. The Biotechnology Education Company.
- Fawole, O. O., Yekeen, T. A., Adewoye, S. O., Ogundiran, M. A., Ajayi, O. E., & Nwaiya, M. N. (2013). Nutritional qualities and trace metals concentration of six fish species from Oba Reservoir, Ogbomoso, Nigeria. African Journal of Food Science, 7(8), 246–252. https://doi.org/10.5897/AJFS2013.0994
- Ferreira-Dias, S., Valente, D. G., & Abreu, J. M. F. (2003). Comparison between ethanol and hexane for oil extraction from quercus suber L. fruits. Grasas y Aceites, 54(4), 378–383. https://doi.org/10.3989/gya.2003.v54.i4.225
- Fukuda, M., & Hasegawa, Y. (2014). Protease activity of a 90-kDa protein isolated from scallop shells. Turkish Journal of Fisheries and Aquatic Sciences, 14(1), 247–254. https://doi.org/10.4194/1303-2712-v14_1_26
- Galla, N. R., Karakala, B., Akula, S. A. P. R., & Pamidighantam, P. R. (2012). Physico-chemical, amino acid composition, functional and antioxidant properties of roe protein concentrates obtained from Channa striatus and lates calcarifer. Food Chemistry, 132(3), 1171–1176. https://doi.org/10.1016/j.foodchem.2011.11.055
- Gam, L. H., Leow, C. Y., & Baie, S. (2005). Amino acid composition of snakehead fish (Channa striatus) of various sizes obtained at different times of the year. Malaysian Journal of Pharmaceutical Sciences, 3(2), 19–30.
- Gam, L. H., Leow, C. Y., & Baie, S. (2006). Proteomic analysis of snakehead fish (Channa striata) muscle tissue. Malaysian Journal of Biochemistry and Molecular Biology, 14, 25–32.
- Gao, X., Zhao, D., Wang, L., Cui, Y., Wang, S., Lv, M., Zang, F., & Dai, R. (2021). Proteomic changes in sarcoplasmic and myofibrillar proteins associated with color stability of ovine muscle during post-mortem storage. Foods, 10(12), 2989. https://doi.org/10.3390/foods10122989
- Gauthier, S. F., Paquin, P., Pouliot, Y., & Turgeon, S. (1993). Surface activity and related functional properties of peptides obtained from whey proteins. Journal of Dairy Science, 76(1), 321–328. https://doi.org/10.3168/jds.S0022-0302(93)77353-1
- Gerde, J. A., & White, P. J. (2008). “Lipids, “in: Soybeans Chemistry, production, processing, and utilization. (L.A. Johnson, White, P.J. and Galloway, R., Eds.). AOCS Press.
- Guedes, M., Vieira, S. F., Reis, R. L., Ferreira, H., & Neves, N. M. (2022). Potent antioxidant and anti-inflammatory bioactivities of fish roe-derived extracts. Innovative Food Science and Emerging Technologies, 82, 103198. https://doi.org/10.1016/j.ifset.2022.103198
- Gutte, K. B., Sahoo, A. K., & Ranveer, R. C. (2015). Effect of ultrasonic treatment on extraction and fatty acid profile of flaxseed oil. Oilseeds & Fats Crops and Lipids, 22(6), D606. https://doi.org/10.1051/ocl/2015038
- Haniffa, M. A., Jeya, S. P., James, M. M., & Kavitha, K. (2017). Protein profiling for phylogenetic relationship in snakehead species. Iranian Journal of Fisheries Sciences, 16(4), 1178–1186.
- Heffernan, S., Giblin, L., & O’Brien, N. (2021). Assessment of the biological activity of fish muscle protein hydrolysates using in vitro model systems. Food Chemistry, 359(1), 129852. https://doi.org/10.1016/j.foodchem.2021.129852
- Hewavitharana, G. G., Perer, D. N., Navaratne, S. B., & Wickramasinghe, I. (2020). Extraction methods of fat from food samples and preparation of fatty acid methyl esters for gas chromatography: A review. Arabian Journal Chemistry, 13(8), 6865–6875. https://doi.org/10.1016/j.arabjc.2020.06.039
- Holdsworth, G., & Ellis, E. D. (2005). Toxicological review of n-hexane. U.S. Environmental Protection Agency.
- Hromádková, Z., Kováčiková, J., & Ebringerová, A. (1999). Study of the classical and ultrasound-assisted extraction of the corn cob xylan. Industrial Crops and Products, 9(2), 101–109. https://doi.org/10.1016/S0926-6690(98)00020-X
- Hultin, H. O., Lanier, H. G. K. T. C., & Park, J. W. (2005). Process for recovery of functional proteins by pH shifts. In J. Park (Ed.), Surimi and surimi sea food (pp. 107–139). Taylor and Francis Group.
- Ibrahim, S. M. (2009). Evaluation of production and quality of salt-biscuits supplemented with fish protein concentrate. World Journal of Dairy & Food Sciences, 4(1), 28–31.
- Ishiwatari, N., Fukuoka, M., & Sakai, N. (2013). Effect of protein denaturation degree on texture and water state of cooked meat. Journal of Food Engineering, 117(3), 361–369. https://doi.org/10.1016/j.jfoodeng.2013.03.013
- Jafari, S. M., Assadpoor, E., He, Y., & Bhandari, B. (2008). Re-coalescence of emulsion droplets during high-energy emulsification. Food Hydrocolloids, 22(7), 1191–1202. https://doi.org/10.1016/j.foodhyd.2007.09.006
- Kentish, S., Wooster, T. J., Ashokkumar, M., Balachandran, S., Mawson, R., & Simons, L. (2008). The use of ultrasonics for nanoemulsion preparation. Innovative Food Science & Emerging Technologies, 9(2), 170–175. https://doi.org/10.1016/j.ifset.2007.07.005
- Kim, S., Kim, Y. T., Byun, H., Nam, K., & Shahidi, F. (2001). Isolation and characterization of antioxidative peptides from gelatin hydrolysate of Alaska Pollock skin. Journal of Agricultural and Food Chemistry, 49(4), 1984–1989. https://doi.org/10.1021/jf000494j
- Kristinsson, H., & Rasco, B. A. (2000). Fish protein hydrolysates: Production, biochemical and functional properties. Critical Reviews in Food Science and Nutrition, 40(1), 43–81. https://doi.org/10.1080/10408690091189266
- Kristinsson, H. G., Theodore, A. E., & Ingadottir, B. (2006). Chemical processing methods for protein recovery from marine by-products and underutilized fish species. In Sahidi F., maximising the value of Marine by-products (pp. 144–168). Woodhead Publishing. https://doi.org/10.1533/9781845692087.1.144
- Kumoro, A. C., Hasan, M., & Singh, H. (2009). Effects of solvent properties on the soxhlet extraction of diterpenoid lactones from andrographis paniculata leaves. “ScienceAsia, 35(3), 306–309. https://doi.org/10.2306/scienceasia1513-1874.2009.35.306
- Kumoro, A. C., Wardhani, D. H., Kusworo, T. D., Djaeni, M., Ping, T. C., & Ma’rifat Fajar Azis, Y. (2022). Fish protein concentrate for human consumption: A review of its preparation by solvent extraction methods and potential for food applications. Annals of Agricultural Sciences, 67(1), 42–59. https://doi.org/10.1016/j.aoas.2022.04.003
- Larrea-Wachtendorff, D., Tabilo-Munizaga, G., Moreno-Osorio, L., Villalobos-Carvajal, R., & Pérez-Won, M. (2015). Protein changes caused by high hydrostatic pressure (HHP): A study using differential scanning calorimetry (DSC) and fourier transform infrared (FTIR) spectroscopy. Food Engineering Reviews, 7(2), 222–230. https://doi.org/10.1007/s12393-015-9107-1
- Liu, L., Na, L., Niu, Y., Guo, F., Li, Y., & Sun, C. (2013). An ultrasonic assisted extraction procedure to free fatty acids from the liver samples of mice. Journal of Chromatographic Science, 51(4), 376–382. https://doi.org/10.1093/chromsci/bms151
- Li, Y., Zheng, Q. H., Liu, G., Peng, Z., Wang, Y., Zhu, Y., Liu, H., Zhao, Y., & Jing Wang, J. (2021). Effects of ultrasound-assisted basic electrolyzed water (BEW) extraction on structural and functional properties of Antarctic krill (euphausia superba) proteins. Ultrasonics Sonochemistry, 71, 105364. https://doi.org/10.1016/j.ultsonch.2020.105364
- Maran, J. P., Priya, B., & Nivetha, C. V. (2015). Optimization of ultrasound-assisted extraction of natural pigments from bougainvillea glabra flowers. Industrial Crops and Products, 63, 182–189. https://doi.org/10.1016/j.indcrop.2014.09.059
- Mehta, N., Jeyapriya, S., Kumar, P., Verma, A. K., Umaraw, P., Khatkar, S. K., Khatkar, A. B., Pathak, D., Kaka, U., & Sazili, A. Q. (2022). Ultrasound-assisted extraction and the encapsulation of bioactive components for food applications. Foods, 11(19), 2973. https://doi.org/10.3390/foods11192973
- Misir, G. B., & Koral, S. (2019). Effects of ultrasound treatment on structural, chemical and functional properties of protein hydrolysate of rainbow trout (oncorhynchus mykiss) by-products. Italian Journal of Food Science, 31(2), 205–223.
- Najafian, L., & Babji, A. A. (2012). A review of fish-derived antioxidant and antimicrobial peptides: Their production, assessment, and applications. Peptides, 33(1), 178–185. https://doi.org/10.1016/j.peptides.2011.11.013
- Nawaz, A., Ali, S. W., Irshad, S., Irshad, F., Ahmed, A., Sharmeen, Z., & Khan, I. (2020). Effect of peeling and unpeeling on yield, chemical structure, morphology and pasting properties of starch extracted from three diverse potato cultivars of Pakistan. International Journal of Food Science and Technology, 55(6), 2344–2351. https://doi.org/10.1111/ijfs.14412
- Nurfaidah, N., Metusalach, M., Sukarno S., & Mahendradatta, M. (2021). Protein and albumin contents in several freshwater fish species of Makassar, South Sulawesi. International Food Research Journal, 28(4), 745–751. https://doi.org/10.47836/ifrj.28.4.11
- Opheim, M., Šližytė, R., Sterten, H., Provan, F., Larssen, E., & Kjos, N. P. (2015). Hydrolysis of Atlantic salmon (Salmo salar) rest raw materialseffect of raw material and processing on composition, nutritional value, and potential bioactive peptides in the hydrolysates. Process Biochemistry, 50(8), 1247–1257. https://doi.org/10.1016/j.procbio.2015.04.017
- Ovissipour, M., Rasco, B., Tang, J., & Sablani, S. (2017). Kinetics of protein degradation and physical changes in thermally processed Atlantic salmon (salmo salar). Food and Bioprocess Technology, 10(10), 1865–1882. https://doi.org/10.1007/s11947-017-1958-4
- Parikh, D. M. (2015). Vacuum drying: Basics and application. Chemical Engineering, 122(4), 48–54.
- Park, J. W., & Lin, J. T. M. (2005). Surimi: manufacturing and evaluation. In J. Park (Ed.), Surimi and surimi seafood (pp. 33–106). CRC Press/Taylor & Francis.
- Paul, D. K., Islam, R., & Sattar, M. A. (2013). Physico-chemical studies of lipids and nutrient contents of Channa striatus and Channa marulius. Turkish Journal of Fisheries and Aquatic Sciences, 13(3), 487–493. https://doi.org/10.4194/1303-2712-v13_3_11
- Peshkovsky, A. S., Peshkovsky, S. L., & Bystryak, S. (2013). Scalable high-power ultrasonic technology for the production of translucent nanoemulsions. Chemical Engineering and Processing: Process Intensification, 69, 77–82. https://doi.org/10.1016/j.cep.2013.02.010
- Pezeshk, S., Ojagh, S. M., Rezaei, M., & Shabanpour, B. (2019). Fractionation of protein hydrolysates of fish waste using membrane ultrafiltration: Investigation of antibacterial and antioxidant activities. Probiotics and Antimicrobial Proteins, 11(3), 1015–1022. https://doi.org/10.1007/s12602-018-9483-y
- Pires, C., Costa, S., Batista, A. P., Nunes, M. C., Raymundo, A., & Batista, I. (2012). Properties of protein powder prepared from Cape hake byproducts. Journal of Food Engineering, 108(2), 268–275. https://doi.org/10.1016/j.jfoodeng.2011.08.020
- Ramić, M., Vidović, S., Zeković, Z., Vladić, J., Cvejin, A., & Pavlić, B. (2015). “Modeling and optimization of ultrasound-assisted extraction of polyphenolic compounds from Aronia melanocarpa by-products from filter-tea factory. Ultrasonics Sonochemistry, 23, 360–368. https://doi.org/10.1016/j.ultsonch.2014.10.002
- Rao, P. G., Jyothirmayi, T., Karuna, M. S. L., & Prasad, R. B. N. (2010). Studies on lipid profiles and fatty acid composition of roe from Rohu (labeo rohita) and Murrel (Channa striatus. Journal of Oleo Science, 59(10), 515–519. https://doi.org/10.5650/jos.59.515
- Rieuwpassa, F. J., & Cahyono, E. (2019). Karakteristik fisiko-kimia konsentrat protein ikan Sunglir (Elagatis bipinnulatus). Jurnal MIPA, 8(3), 164–167. https://doi.org/10.35799/jmuo.8.3.2019.26189
- Rieuwpassa, F. J., Karimela, E. J., & Karaeng, M. C. (2020). Analisis fisiko kimia konsentrat protein ikan Nila (Oreochromis niloticus) yang diekstrak menggunakan pelarut etanol. Jurnal Teknologi Perikanan dan Kelautan, 11(1), 45–52. https://doi.org/10.24319/jtpk.11.45-52
- Rocha, M., Cardozo, M. A., & Raffi, J. E. (2017). Functional, thermal, and physicochemical properties of proteins from Argentine croaker (umbrina canosai) recovered by solubilization/precipitation or a washing process. International Food Research Journal, 24(2), 579–588.
- Romadhoni, A. R., Afrianto, E., Pratama, R. I., & Grandiosa, R. (2016). Extraction of snakehead fish [ophiocephalus striatus (Bloch, 1793)] into fish protein concentrate as albumin source using various solvent. Aquatic Procedia, 7, 4–11. https://doi.org/10.1016/j.aqpro.2016.07.001
- Rouhani, S., Alizadeh, N., Salimi, S., & Haji-Ghasemi, T. (2009). Ultrasonic assisted extraction of natural pigments from rhizomes of Curcuma Longa L. Progress in Color, Colorants and Coatings, 2(2), 103–113.
- Roy, V. K., Kumar, N. S., & Gurusubramanian, G. (2012). Proteins-structure, properties and their separation by SDS-polyacrylamide gel electrophoresis. Science Vision, 12(4), 170–181.
- Santos, H. M., Lodeiro, C., & Capelo-Martinez, J. L. (2009). The power of ultrasound. In Capelo-Martinez, J. L. (Ed)., Ultrasound in chemistry: Analytical applications (pp. 1–16). Wiley.
- Sathivel, A., Yin, H., Bechtel, P. J., & King, J. M. (2009). Physical and nutritional properties of catfish roe spray dried protein powder and its application in an emulsion system. Journal of Food Engineering, 95(1), 76–81. https://doi.org/10.1016/j.jfoodeng.2009.04.011
- Sen, D. P. (2005). Advances in fish processing technology. Allied Publishers.
- Shaviklo, A. R. (2015). Development of fish protein powder as an ingredient for food applications: A review. Journal of Food Science and Technology, 52(2), 648–661. https://doi.org/10.1007/s13197-013-1042-7
- Silva, P. B., Mendes, L. G., Rehder, A. P. B., Duarte, C. R., & Barrozo, M. A. S. (2020). Optimization of ultrasound-assisted extraction of bioactive compounds from acerola waste. Journal of Food Science and Technology, 57(12), 4627–4636. https://doi.org/10.1007/s13197-020-04500-8
- Strzelczak, A., Balejko, J., Szymczak, M., & Witczak, A. (2021). Effect of protein denaturation temperature on rheological properties of Baltic herring (clupea harengus membras) muscle tissue. Foods, 10(4), 829. https://doi.org/10.3390/foods10040829
- Sun, T., Xu, Z., & Godber, S. (2006). Ultrasound assisted extraction in quantifying lutein from chicken liver using high-performance liquid chromatography. Journal of Chromatography B, 830(1), 158–160. https://doi.org/10.1016/j.jchromb.2005.10.029
- Suwandi, R., Nurjanah, N., & Margaretha, W. (2014). Body parts proportion and proximate levels of Snakehead on various sizes, “Jurnal Pengolahan dan Bioteknologi Hasil Perikanan Indonesia. Indonesian Fisheries Processing Journal, 17(1), 22–28. https://doi.org/10.17844/jphpi.v17i1.8134
- Tessari, P., Lante, A., & Mosca, G. (2016). Essential amino acids: master regulators of nutrition and environmental footprint? Scientific Reports, 6(1), 26074. https://doi.org/10.1038/srep26074
- Thawornchinsombut, S., & Park, J. W. (2006). Frozen stability of fish protein isolate under various storage conditions. Journal of Food Science, 71(3), 227–230. https://doi.org/10.1111/j.1365-2621.2006.tb15622.x
- Theodore, A., Sivakumar, R., & Kristinsson, H. G. (2008). Antioxidative activity of protein hydrolysates prepared from alkaline-aided channel catfish protein isolates. Journal of Agricultural and Food Chemistry, 56(16), 7459–7466. https://doi.org/10.1021/jf800185f
- Tottoli, E. M., Dorati, R., Genta, I., Chiesa, E., Pisani, S., & Conti, B. (2020). Skin wound healing process and new emerging technologies for skin wound care and regeneration. Pharmaceutics, 12(8), 735. https://doi.org/10.3390/pharmaceutics12080735
- Vardanega, R., Santos, D. T., & Meireles, M. A. A. (2014). Intensification of bioactive compounds extraction from medicinal plants using ultrasonic irradiation. Pharmacognosy Reviews, 8(16), 88–95. https://doi.org/10.4103/0973-7847.134231
- Venugopal, V. (2006). Seafood processing adding value through quick freezing, retortable packaging, and cook-chilling. Taylor & Francis Group, CRC.
- Walayat, N., Xiong, Z., Xiong, H., Moreno, H. M., Niaz, N., Ahmad, M. N., Hassan, A., Nawaz, A., Ahmad, I., & Wang, P.-K. (2020). Cryoprotective effect of egg white proteins and xylooligosaccharides mixture on oxidative and structural changes in myofibrillar proteins of culter alburnus during frozen storage. International Journal of Biological Macromolecules, 158, 865–874. https://doi.org/10.1016/j.ijbiomac.2020.04.093
- Wen, C., Zhang, J., Zhang, H., Dzah, C. S., Zandile, M., Duan, Y., Ma, H., & Luo, X. (2018). Advances in ultrasound assisted extraction of bioactive compounds from cash crops – a review. Ultrasonics Sonochemistry, 48, 538–549. https://doi.org/10.1016/j.ultsonch.2018.07.018
- Wiharja, S. Y., Santoso, J., & Yakhin, L. A. (2013). Utilization of yellowfin tuna and red snapper roe protein concentrate as emulsifier in mayonnaise. Journal of Food Science and Engineering, 3(12), 678–687. https://doi.org/10.17265/2159-5828/2013.12.006
- Williams, J. Z., Abumrad, N., & Barbul, A. (2002). Effect of a specialized amino acid mixture on human collagen deposition. Annals of Surgery, 236(3), 369–375. https://doi.org/10.1097/00000658-200209000-00013
- Windsor, M. L., “Fish protein concentrate,” torrey advisory note nr.39. FAO fisheries and aquaculture department, Rome. http://www.fao.org/wairdocs/tan/x5917E/x5917e01.htm. Retrieved May 1, 2022, 2001
- Yang, Y., & Hu, B. (2014). Bio-based chemicals from biorefining: Lipid and wax conversion and utilization. In K. Waldron (Ed.), “In: Advances in biorefineries (pp. 693–720). Woodhead Publishing Limited.
- Yang, K. R., Tsai, M. F., Shieh, C. J., Arakawa, O., Dong, C.-D., Huang, C.-Y., & Kuo, C.-H. (2021). Ultrasonic-assisted extraction and structural characterization of chondroitin sulfate derived from jumbo squid cartilage. Foods, 10(10), 2363. https://doi.org/10.3390/foods10102363