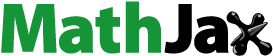
Abstract
The rising interest in bioactive phyto-compounds, known for their antioxidant, phenolic, and flavonoid properties, has focused attention on Moringa oleifera. With its abundant phyto-molecules, Moringa is a promising source for biomolecule extraction. As a significant vegetable crop, Moringa provides ample nutrients that can be efficiently extracted using novel methodologies. This study aims to optimize ultrasound-assisted extraction from Moringa leaves, considering diverse parameter combinations for improved yield and quality. Ultrasound-assisted extraction, a notable advancement, considers parameters like amplitude, temperature, and extraction time. Employing Response Surface Methodology (RSM) with 20 simulated runs, this investigation assessed extraction yield, Total Phenol Content (TPC), Total Flavonoid Content (TFC), and Radical Scavenging Activity (RSA). The optimized technique yielded an extraction yield of 12.78-23.28%, TPC of 100.12-149.22 mg GAE/g, TFC of 14.43-20.19 mg QE/g, and RSA of 58.87-85.08%. Increased amplitude power, higher extraction temperature, and shorter extraction time contributed to enhanced biomolecule extraction. GC-MS profiling identified various bioactive compounds in the Moringa leaf extracts, showcasing potential applications in nutraceutical industries.
REVIEWING EDITOR:
1. Introduction
In contemporary times, there has been a notable surge in delve and interest regarding the extraction of biomolecules from plant sources (Alvin et al., Citation2014). These biomolecules from plant sources are cheap, effective, healthy and also could be considered an alternative to chemical drugs, as cause various side effects in humans and animals (Ping 2010). The secondary metabolites from various plants are reported with multitude of medicinal, pharmaceutical and nutraceutical properties (Olson & Fahey, Citation2011 & Zhao et al., Citation2019). In recent research studies, it has been studied that the intake of natural antioxidants prevents many diseases like oxidative stress, obesity and some types of cancer (Bharali et al., Citation2003, Mehta et al., Citation2003, Ahmad et al., Citation2016). Among several plant species, most of the essential bioactive moleculesare present in Moringa leaves and its extracts (Vaishnav et al., Citation2011, Vongsak et al., Citation2013, Vázquez-Espinosa et al., Citation2019). Several studies have presented evidence indicating that the antioxidant capacity is higher in dried or desiccated leaves when compared to their fresh counterparts (Siddhuraju & Becker, Citation2003, Singh et al., Citation2009).
Moringa oleifera is originally indigenous to the regions of Cis-Himalaya, including India, Afghanistan, Bangladesh, and Pakistan. It has since gained widespread cultivation worldwide due to its exceptional blend of phenolic compounds, flavonoids, antioxidants, and its ability to thrive in various soil types, especially in tropical and subtropical climates. The Moringa plant also contains a majority of useful nutrients like Iron, Potassium, calcium, Vitamin A, E, C and amino acids (Rodríguez-pérez 2015). Moringa is one of the major sources of various dietary supplements for treating malnutrition and also for medicinal purposes (Castillo-Lopez et al., Citation2017). Numerous research studies indicate that moringa leaf extracts exhibit extensive biological activities, including but not limited to antioxidative, anti-inflammatory, anti-microbial, anti-helminthic, anti-obesity, anti-sclerotic, anti-HIV, and anti-diabetic properties (Kilany et al., Citation2020; Alkafafy et al., Citation2021). The antioxidant compounds like ascorbic acid, Vitamin E and chlorophyll in the leaves have the ability to defend cells from oxidative damage and thesephytomolecules are responsible in protecting human health (Ramić et al., Citation2015). Based on the plant species, the recovery yield, quality and nature of phytocompounds varies from plant to plant and also influenced by the method of extraction, extraction time, extraction temperature, solvent: water ratio, and polarity of the solvent (Rodríguez-Pérez et al., Citation2016). Various extraction methods exist that prioritize economic efficiency, simplicity, environmental friendliness, and the achievement of high extraction yields (Ramić et al., Citation2015).
Various methods, such as conventional and non-conventional methods are employed to extract biomolecules from the plant matrix (Uysal et al., Citation2019). The solid-liquid extraction method is the widely employed approach for extracting phenols, flavonoids, and other crucial biomolecules. Biomolecules are usually located within the plant matrix, and their extraction typically involves transferring them from the dried leaves (solid-phase) into the solvent (liquid-phase) through the utilization of various extraction techniques. During this process, the solvent permeates into the plant matrix, liquifying the compounds, and transitions into the liquid phase. This procedure is referred to as solid-liquid extraction or leaching (Geankoplis et al., Citation2018). The traditional extraction methods has countless drawbacks such as the high requirement of solvents, prolonged period of extraction leading to oxidation of biomolecules and deprotonation of desired phytocompounds (Vinatoru, Citation2001). To address the limitations associated with conventional extraction methods, alternative novel extraction techniques (UAE, MAE & SFE) have been introduced. These approaches offer benefits such as reduced solvent usage, shorter processing time, faster extraction, environmentally friendly operations, and enhanced efficiency (Lasta et al., Citation2019). Various research has been conducted in similar fashion using UAE under RSM model with better inhibitory enzyme activity (87.99%), phenolic (97.26 mg GAE/g) and flavonoid content (56.63 mg QE/g) (Setyani et al., Citation2023 & Yerena-Prieto et al., Citation2022). UAE functions based on the cavitation principle namely formation, growth and sudden bursting of bubbles with high energy released from hot spots, where the cell wall gets ruptured by these generated sonic waves and the target molecules get released into the liquid solvent phase from natural plant matrices (Mason et al., Citation1996). The so-called hot spots do not generate thermal damage to the heat sensitive compounds as they are momentary with high cooling rates with a range 1010 K/s (Suslick et al., Citation1986). In modern era, UAE is widely adopted in extracting various types of biomolecules from plant tissue (Mason et al., Citation1996).
The main goal of this study is to evaluate the efficiency of ultrasound-assisted extraction (UAE) for extracting specific biomolecules from Moringa oleifera leaves. To avoid the difficulties in the conventional methods of extraction and to optimize the conditions favouring extraction process with high recovery yield (EY), total phenolic compound (TPC), total flavonoid compound (TFC) and radical scavenging activities (RSA) using a response surface Methodology is applied based on Central Composite Design (CCD). This statistical method is to optimize, evaluate and determine the interactions of independent variables namely amplitude (%), temperature (°C) and time (Min) with dependent variables such as EY (%), TPC (GA mg/g), TFC (QE mg/g) and RSA (%) for predicting the optimal parameters during the experimental trial.
2. Materials and methods
2.1. Chemicals and reagents
All the chemical compounds required for the biochemical analysis and extraction process like ethanol (99%), sodium hydroxide, 2,2’-Diphenyl-1-Picryl-Hydrazyl (DPPH), methanol, sodium carbonate, sodium nitrate, potassium acetate, aluminium chloride, Folin-Ciocalteu (FC) reagent was purchased from HiMedia Laboratories, Merk Life Science and Central Drug House Fine Chemicals. The analytical reference standards were acquired from Sigma Aldrich, USA.
2.2. Preparation of plant material
Organic Drumstick or Moringa leaves were harvested at Western Block of Horticultural College and Research Institute (TNAU), Periyakulam, Tamil Nadu, India. Moringa leaves of PKM 1 were freshly harvested from a two-year-old trees at 45 days interval and used for analysis. Harvested fresh leaves were rinsed twice with clean water. Leaflets were collected separately from the rachis and dried at 40 ± 2 °C for 4 hours using a cabinet solar drier (Ali et al., Citation2014 and Aggarwal et al., Citation2021). With the aid of a semi-automatic pulveriser (commercial-grade), the dried leaves were ground to a fine powder and passed through 250 µm sieve. Moringa leaves are milled and passed through a sieve to increase the interaction of solvent with the plant sample. Finally, the milled moringa leaf powder was stockpiled in refrigerated, moisture free conditions at 4 °C for other supplemental analysis.
2.3. Ultrasound-assisted extraction
In this optimization study, UAE was performed using an Ultrasound Probe Sonicator VCX 1500 equipped with 1” diameter probe (20 kHz, 15 amps, 1500 W) manufactured by Sonics & Materials Inc. USA. The instrument is equipped with a thermostat probe and versatile power meter, that allows to maintain the temperature and vary the power values of the instrument respectively. Dried moringa leaf sample of 5 g were sonicated with 70% ethanol in the ratio 1:10 (Wang et al., Citation2017) with pulse mode, 5s on and off. The samples were extracted under ice-cold conditions to avoid the degradation. After sonication, moringa extracts were centrifuged (10 min @ 6000 rpm), concentrated using a rotavap at 40 °C and lyophilized. Freeze-dried moringa extracts were stored for further analysis under deep freeze connditon.
2.4. Estimation of moringa leaf extract recovery yield
The moringa leaf extract yield was estimated based on the mass of lyophilized extract after complete removal of the solvent and the quantity of the leaf sample used for extraction. A complete portion of the hydroethanolic extract was concentrated under vacuum using rotary evaporator at 40 °C and later lyophilized. Dried extracts were weighed and the results were expressed in percentages (Cho et al., Citation2020). The following equation was used to compute the extraction yield:
2.5. Estimation of total phenolic contents in moringa extracts
The total phenol content (TPC) in the moringa leaf extract was estimated spectrophotometrically by the modified Folin-Ciocalteau method proposed by Chavan et al., Citation2013 and Rakesh et al., Citation2021. Total phenolic content in the extract was calculated using gallic acid as reference standard.
2.6. Estimation of totalflavonoid content in moringa extracts
The total flavonoid content within the moringa leaf extract was assessed using an aluminum chloride method, which incorporated minor adjustments as suggested by Daghaghele et al., 2021 and Rakesh et al., Citation2021. Total flavonoid content in the extract was calculated using quercetin as reference standard.
2.7. Free radical scavenging activity by DPPH assay
The scavenging activity of the free radicals in moringa leaf extract was estimated by DPPH (2,2 -Diphenyl-1-Picryl-Hydrazyl Method with slight modifications proposed by Aryal et al., Citation2019 and Rakesh et al., Citation2021. The experiment was conducted in a dark environment due to the light sensitivity of the DPPH chemical. Ascorbic acid served as the reference standard and RSA was calculated using the following formula:
Where, Acontroland ASampledenotes absorbance at 517 nm for the blank and moringa extract, respectively
2.8. RSM experimental modelling and statistical analysis
The optimization of moringa leaf extraction using ultrasound assisted method was simulated and modeled using the multivariate tool i.e. Response Surface Methodology (RSM) using Design Expert 13 software. RSM represents a fusion of statistical and mathematical techniques employed to enhance, create, and optimize a process (Kaderides et al., Citation2019). The optimization investigations were conducted through a set of 20 designed experiments, encompassing both 6 central and 14 non-central points, as per the Central Composite Design (CCD). In these experiments, three factors were considered as independent variables () (Amplitude, Temperature, and Time), while four responses were observed as dependent variables () (EY, TPC, TFC and Extraction yield, Total phenol content, Total Flavonoid Content, and Antioxidant activity). The relationship between the independent and dependent variables mentioned above was analyzed using the least-square multiple regression approach. A multiple regression equation was employed to establish a second-order polynomial equation derived from the experimental data, as illustrated in the following equation:
Table 1. Independent variables and the specified parameter ranges in the experimental design for ultrasound-assisted extraction.
Table 2. Dependent variables specified in the experimental design for ultrasound-assisted extraction.
Where Y represents the corresponding response of the extraction process (i.e., EY, TPC, TFC, RSA); Independent variables are represented by Xi and Xj are the independent input variables;while ‘n’ signifies the number of independent parameters involved in the experiment.β0, βi, βii, βij represent the coefficients in the regression model, where, β0 corresponds to the intercept, βi relates to linear terms, βii pertains to quadratic terms, and βij signifies interaction terms; while ‘r’ represents, mean sum of squares for experimental errors. Predetermined values of the parameters are mentioned and the different steps of multi-objective optimization for both independent and dependent variables are illustrated through the use of RSM (). The primary goal of the research study is to achieve maximum extraction yield by means of higher phenol, flavonoid and antioxidant activity concerning optimized independent parameters.
Table 3. Parameters for the central composite design regarding the independent variables and experimental responses.
2.9. GC-MS profiling of moringa leaf extract
The biomolecule profiling in the moringa leaf extract from the Ultrasound Assisted Extraction was done using Trace GC ultra-chromatograph system (Thermo Fischer Scientific, Austria) coupled to Thermo Scientific DSQ II quadruple MS. Extracted moringa leaf extract was lyophilized and reconstituted in 99.99% pure HPLC grade methanol (1 mg/ml). The mixture is sonicated using a water bath sonicator for 2 cycles. The moringa leaf extracts was were passed through a syringe filter and the feeding samples were transferred fully automated using a Tri-plus RSH head space auto sampler. Methanolic extract was separated using a 5% phenyl methyl silicone fused silica capillary column (TG-SQC, 15 m in length, 0.25 mm I.D. and 0.25 µm film thickness). An ionizing energy of 70 eV was used in the electron ionization system with 99.99% pure helium carrier gas with 1 ml/min flow rate and 10 ml split flow. About 60 °C of injector temperature was maintained with column temperature programs such as 50 °C (initial temperature) held for 1 min and gradually increased to 150 °C by 25 °C/min rate. Around 265 °C and 200 °C were maintained for transfer line and MS source temperature respectively. The relative percentage amount of each component was calculated by comparing its average peak area to the total areas, using Xcalibur software (Qual, Quan and library) to view spectra and chromatograms analysis. Based on their retention time, peak area percentage, and mass spectra pattern, the chromatogram is compared with the National Institute of Standards and Technology’s library (NIST library) of NIST11.LIB database and the unknown phytochemicals present in moringa extracts were identified.
3. Results and discussion
3.1. The impact of amplitude, temperature and extraction time on extraction yield
Using numerical data acquired from CCD, quadratic regression models were developed through RSM model to assess the influence of independent varaibles of UAE on the recovery yield (EY) of moringa leaves. The statistical examinationof the extraction yield were provided in .
Table 4. Analysis of Variance (ANOVA) findings for the extraction yield.
From , Theconstructed model exhibitedsignificance of the experimental process with an F value of 34.97 at 5% level for amplitude, temperature and extraction time. The well-fitted model, significant at a 5% level, demonstrates a strong fit for the factors mentioned above. Model terms with p-values (< 0.0500) indicate their significance. In this case, terms A, B, C, A2, B2, and C2 are all significant model factors.Comparable findings were documented in a study by Şahin (Citation2015). The response model, a predicted second-order polynomial derived from the CCD, for the extraction yield of moringa leaf extract is represented by coded equations
The analysis of variance (ANOVA) for the quadratic regression model reveals that the predicted R2 of 0.7904 agrees with value of adjusted R2 = 0.9415 and R2 = 0.9692. The critical F-values for A, B, and C were 32.09, 15.50, and 6.05, respectively. The low P-value in comparison to the F-value indicates that the RSM technique may be the most suitable model for the optimization of extraction process. Based on the P values in the ANOVA results (), variable amplitude is found to be the most important factor having an effect on the yield of moringa leaf extract (p < 0.001) followed by temperature and time as the P-value is greater than 0.01.
The distinctive connection between the independent and dependent variables can be elucidated through a schematic interpretation using a 3D response surface graph model (Utharalakshmi et al., Citation2015). The 3D model graph provides a simple, easy way to understand complex phenomena. illustrates the 2D and 3D interactions between amplitude and temperature with respect to extraction yield. showed the interaction between amplitude and extraction temperature at a fixed solvent ratio of 500 ml/5g. The extraction yield exhibited an upward trend as the amplitude increased from 60% to 80%, while the temperature ranged from 25 to 45 °C, and the extraction time varied between 20 to 40 minutes. The highest extraction yield, reaching 23.28%, was achieved at 30 minutes of extraction time duration. The recovery yield might have improved at a higher amplitude due to the penetration of powerful sonic waves in the solvent media through the leaf samples causing shear or rupturing the plant cell wall with the rise in a temperature gradient to solubilize the biomolecules (Ma et al., Citation2008, Chavan et al., Citation2013, Zhang et al., Citation2017, Wen et al., Citation2018). However, the extraction yield decreased when the amplitude increased beyond 70%, as it may degrade the biomolecules at higher cavitation process in the extract. According to , extraction yield also increased at a temperature of 35 °C and an increase in temperature of more than 35 °C dramatically decreased the extraction yield to ∼15%. Comparable findings have been documented in studies conducted by Pan et al., Citation2003, Ma et al., Citation2008, Karabegović et al., Citation2013.
3.2. The impact of amplitude, temperature and time on totalphenoliccontent
The experimental and projected values for the total phenol content in the moringa leaf extract obtained through ultrasound-assisted extraction, encompassing various combinations of independent variables were presented in . The projected values are juxtaposed with the actual experimental results, revealing a high degree of concordance. delineats the statistical analysis and its respective interpretation.
Table 5. Analysis of Variance (ANOVA) pertaining to the total phenol content.
The significance of the model was signified based on the F-value of 52.00. In the experimental results of ultrasound-assisted extraction, the TPC spans from 100.12 mg GAE/g to 149.22 mg GAE/g. Considering the P-values (<0.05), it is evident that amplitude, temperature, and time exert a notable and statistically significant influence on the recovery of total phenolic content from moringa leaves. From the experimental design, increase in amplitude from 20% to 70% with the extraction temperature of 35 °C for 30 min yielded maximum phenolic compounds. The increased efficiency in extracting total phenol content could be attributed to the higher shear rate achieved within a shorter duration by rupturingplant cells of moringa leaf, thereby enhancing the leaching of phenolic compounds (Santos et al., Citation2018). The p-value for lack of fit is 0.0713, which means that p > 0.05, indicating non-significance. This suggests that the proposed model is a good fit for the experimental data, and the independent variables or parameters significantly influence the response.
The F-values in the experimental trial surpass the P-values, indicating that RSM design is well-suited for the experimental model. The coded equations representing the prophesied second-order polynomial response model derived from the CCD for extracting moringa leaf extract yield are as follows:
In the ANOVA table, the quadratic regression model presents both the adjusted R2and R2values. The predicted R2 = 0.8659 demonstartes reasonable close match with adjusted R2 = 0.9603 with a less difference (< 0.2). Increased amplitude, elevated temperature, and prolonged extraction duration, combined with hydro-ethanolic solvent, result in a increased recovery of phenoliccompounds from plant sample. These findings align with prior research findings of Alves et al., Citation2016, Desta et al., Citation2016, Xie et al., Citation2018. 2D and 3D plots of total phenol content for different ranges of amplitude and temperature were depicted in . According to the P values in the ANOVA results (), the amplitude is the most important factor having an impact on the phenolic content in moringa leaf extract (p < 0.05) followed by temperature and time on phenolic compounds as the P-value is lesser than 0.05. Consistent findings were observed in the previous literatures and research conducted by Wang et al. (Citation2018) and Ghafoor et al. (Citation2009), where wheat bran and grape seeds, respectively, yielded increased amounts of phenolic compounds through ultrasound-assisted extraction (UAE). represents the relationship between the factors like amplitude and time of extraction under ultrasound conditions are expressed in 2D and 3D plots to understand easily.
3.3. The impact of amplitude, temperature and time on total flavonoid content
The quadratic curve derived from the Multivariate regression analysis utilizing response surface methodology (RSM) of total flavonoid content, considering the independent factors of Amplitude, Temperature, and Time, is represented as follows:
To assess the significance of the experimental model, an analysis of variance (ANOVA) was conducted. The outcomes of the ANOVA are presented in for reference.
Table 6. Analysis of Variance (ANOVA) pertaining to the total flavonoid content.
displays the F-values and P-values corresponding to each independent variable. The Model F-value, which stands at 52.02, signifies the model’s significance. There is merely a 0.01% likelihood that such a substantial F-value could occur purely by chance. Additionally, since the P-values are all below 0.0500, it confirms the model’s significance. Within this experimental model, both individual independent variables and their combinations, including A, B, AB, AC, A2, B2, and C2, exhibit statistical significance. In of the ANOVA, values exceeding 0.1000 indicate the lack of significance for the respective factor. If numerous model terms are found to be insignificant, it may be beneficial to consider model reduction to enhance the model’s performance. The Lack of Fit of F-value and its related P-value, which are 3.60 and 0.092, respectively, indicate that it is not statistically significant. This suggests that the proposed experimental model aligns well with the observed data. To determine the optimal values of the independent variables affecting the experimental model for flavonoid extraction, one can readily explore the relationship between these factors and the response using 2D and 3D graphs, as shown in .
Figure 3. 2D and 3D graphs depicting the relationship between Amplitude and temperature for total flavonoid content.

In , it shows that total flavonoid content ranges from 14.43 to 20.19 QE mg/g, similarly the flavonoid content increased at the first phase with an increase in amplitude and temperature. Subsequently, the flavonoid content exhibited a decrease when subjected to higher levels of ultrasonication power (Amplitude > 70%), elevated temperatures exceeding 35 °C, and extended extraction periods exceeding 30 minutes. Consistent findings were documented in previous studies of Wang et al. (Citation2017) and Daghaghele et al. (Citation2021), indicating an increase in flavonoid content with prolonged extraction times, spanning from 1.5 to 2 hours and from 5 to 25 minutes, respectively. Similarly, a remarkable decrease was observed above 2.5 hours and 25 min during the extraction process in moringa samples respectively.
3.4. The impact of amplitude, temperature and time on scavenging activity
The free radical scavenging activity for the moringa leaf extract obtained from ultrasonication ranged from 58.87% to 85.08%. The DPPH activity results obtained from the experimental model show a slightly higher range compared to those reported by Pollini et al. (Citation2020) for drumstick leaves, which ranged from 13.7% to 40.6%. The peak antioxidant activity of 85% was noted in experimental run 17, characterized by 70% amplitude, 30 °C of extraction temperature with 35 minutes of duration. According to the findings in of the ANOVA, the P-values for the variables amplitude and temperature were both below 0.05. This indicates that the independent variables, amplitude and extraction temperature, showed a significant influence on the recovery of antioxidant compounds from moringa leaves. In contrast, extraction time did not have a prominent impact on the extraction of antioxidant compounds. These factors exhibited P-values below 0.05, signifying the significance of model terms A, B, AB, AC, A2, B2, and C2. Moreover, the Model F-value of 79.28 confirms the experimental model’s significance at a 95% confidence level. The Lack of Fit P-value is 0.4982 infers its non-significance with considerable effect of independent variables on the responses.
Table 7. Analysis of variance (ANOVA) pertaining to the radical scavenging activity (antioxidant activity).
At 95% confidence level, both R2 and adjusted R2 values were greater than 0.95, respectively. The coefficient of variance is lesser than 10% in the RSM model of optimization supporting significance of the propsed model. The lower value of CV (1.73%) represents the precision of the prediction, especially in laboratory studies. The relationship between the X1, X2, and X3 for free radical scavenging activity (RSA %) is expressed as follows:
The antioxidant activity (RSA%)of the moringa leaf extract, has been investigated using DPPH method and moringa leaves were reported to have maximum antioxidant potential (Wang et al., Citation2017, Zhao et al., Citation2019). The scavenging activity ranges from 58.87 to 85.08%. Based on the various factors in the experimental model, amplitude and temperature at optimal level increased the antioxidant compound recovery from leaves. Similarly, Castro-López et al. (Citation2017) and Xu et al. (Citation2019) reported high antioxidant activity and IC50 values in moringa leaf extract obtained through UAE method. Similarly, the results of the current experimental model corroborate with the results of Bozinou et al. (Citation2019) with 64.82 RSA % (DPPH assay) in ultrasonicated moringa leaf extracts. The graphical depiction of the connection between the independent and dependent variables is presented in 2D and 3D graphs ().
Figure 4. 2D and 3D graphs depicting the relationship between Amplitude and temperature for radical scavenging activity.

The graphs illustrate that amplitude and temperature are critical factors in the recovery of free radical scavenging compounds. Antioxidant activityincreases with an increase in amplitude along with the desired temperature. Beyond the amplitude of 70% and extraction temperature above 30 °C degrades the biomolecules related to antioxidant activity. From the above graphs, it is also found that lower amplitude power and less extraction temperature didn’t provide an impact on the leaf samples in extracting antioxidant compounds. This might be attributed mostly to cavitation during the ultrasonication process, which could have ruptured the cell wall and improved solvent penetration into the damaged plant cells (Dolatowski et al., Citation2007 and Chemat et al., Citation2017). This impact of sonication energy results in leaching the majority of biomolecules into the dispersed solvent phase during extraction.
3.5. RSM experimental confirmation of optimal conditions
To graphically evaluate the impact of each factor on the responses, the perturbation plots was plotted (). The graphical plot show that increases in the factors amplitude (A) from 60% to 72.13%, temperature (B) from 25 to 34.33 °C and time (C) from 20 min to 31.19 min. The dependent variables (EY, TPC, TFC and RSA) decreases with increase in amplitude (A) from 72.13 to 80%, extraction temperature (B) from 34.33 to 45 °C and extraction time (C) from 31.19 min to 40 min. Under, predicted extraction conditions higher yield in Moringa oleifera biomolecules were extracted with optimized factors. Similarly, the results coincide with the research outcomes of (Dadi et al., Citation2019, Setyaningsih et al., Citation2019, Pollini et al., Citation2020, Albarri & Şahin, Citation2021, Daghaghele et al., Citation2021, Yerena-Prieto et al., Citation2022) were the moringa biomolecules are extracted by adopting optimized amplitude, temperature and time between the predicted values.
Figure 5. Perturbation plot indicating (Left to Right), EY, TPC, TFC and RSA produced with reference to each variable.

A desirability function methodology was utilized to optimize the extraction process for achieving the highest possible yield while maximizing phenol content, flavonoid content, and antioxidant compounds. With RSM generated experimental model, the optimum experimental extraction condition required for the maximum recovery of four responses (EY, TPC, TFC and RSA) was validated with 72.13% of amplitube power, 34.33 °C of temperature and 31.19 min of extraction time. The optimization of UAE method for dried leaves of moringa using RSM demonstrates that regression models can reliably forecast the extraction yield, total phenol content, total flavonoid content, and radical scavenging activity across various combinations of independent factors such as amplitude, extraction temperature, and time.The comparison between experimental responses and predicted values on the plot revealed negligible discrepancies between the corresponding data points (). At the ideal extraction conditions, the extraction yield, total phenol content, total flavonoid content, and DPPH antioxidant activity were measured at 22.27%, 145.71 GA mg/g, 19.90 QE mg/g, and 83.52%, respectively, demonstrating a remarkable proximity to the predicted values. The findings indicate that the model is highly appropriate for the extraction of the four mentioned responses.
3.6. Validation experiment and analysis of the moringa leaf extract using GC-MS
The extraction parameters of the equipment were simulated with 95% confidence based on the findings of the design experiment software and extracted for its stability based on the optimisation results of the projected points from the RSM design. The quantitative values of the moringa leaf extract through the optimized parameters were represented in .
Table 8. Qualitative data of the extracted moringa leaf extract with optimized parameters.
3.7. Chemical profile of moringa leaf extract from optimized UAE protocol using GC-MS
GC-MS analysis was used to profile several unknown volatile biomolecules and identify phytoconstituents of the hydroethanolic extract from the optimised UAE process. These biomolecules identified using the novel extraction method was compared to previous studies on their biological activities. The results of GC-MS were determined using NIST database library and respective chromatogram () is mentioned. The mass spectrum of unidentified phyto-compounds was interpreted against the reference spectrum in the NIST database. The moringa extract was found to contain a variety of phytoconstituents, including alkaloids, flavonoids, phenols, and tannins. The identified phytocompounds through GC-MS analysis were 2-Amino-4,4,6,6-tetramethyl-4,6-dihydrothieno-[2,3-c]furan-3-carbonitrile,Corynan-17-ol, 18,19-didehydro-10-methoxy-, 1,4-Dihydroxybenzene, 2-amino-4,6-dihydro-4,4,6,6-tetramethyl-, 2,6-di-tert-butyl-; 2,6-Bis(tert-butyl)phenol and 1-Linolenoylglycerol, 2TMS derivative, were found to possess anticancer properties, as reported by Ren et al. (Citation2019), Divyalakshmi and Thoppil (Citation2022), and Ibrahim and Abdul-Hafeez (Citation2023). Additionally, antimicrobial components like Propanoic acid, 2-(3-acetoxy-4,4,14-trimethylandrost-8-en-17-yl)-, 1,2,4-Cyclopentanetriol, 1-Monolinoleoylglycerol trimethylsilyl ether, and 2,5-Furandione, 3-methyl were identified in previous literatures of Abed et al. (Citation2023) and Singh et al. (Citation2009). In a similar vein, compounds such as 1-Monolinoleoylglycerol trimethylsilyl ether, Hydroquinone, 2,6-bis(1,1-dimethyl ethyl)-, 1,2,4-Cyclopentanetriol, 1,1,3,3,5,5,7,7,9,9,11,11,13,13-tetradecamethyl, Distearylthiodipropionate, and aarotene were found to exhibit antioxidant activities in the results were informed by Senthil et al. (Citation2016), Ren et al. (Citation2019), Yassin et al. (Citation2022), and Ezez et al. (Citation2023), respectively. Other biological activities like Hydroxylase inhibitors were identified from n-Hexadecanoic acid (Mohammad et al., Citation2019), GABAB neural receptors in Succinic acid, (4-methoxyphenyl) ester (Kowalczyk & Kulig, Citation2014), Antifungal activities in, 2,6-bis(1,1-dimethyl ethyl), Hexasiloxane,1,1,3,3,5,5,7,7,9,9,11,11- dodecamethyl-, 1,4-Benzenediol and Adulticidal Activity in 2-Acetylamino-2-cyano-acetamide (Rajalakshmi et al., Citation2022). Other important identified compounds with their respective biological activities are listed in under supplementary data. The profiling of phytochemicals with various biological activities might be due to the shearing effect caused by cavitation during sonication on the cellular structure of the moringa leaves in the hydroethanolic solvent causing increased recovery through leaching of listed biomolecules into the solvent phase.
Table 9. List of identified compounds using GC-MS with optimized extraction parameters.
4. Conclusion
This study utilized the Response Surface Methodology (RSM) experimental design to concentrate on optimizing Ultrasound-Assisted Extraction (UAE) with 70% ethanol. The objective was to attain maximum recovery of extract, total phenolic content, total flavonoid content, and free radical scavenging activity (RSA%) from Moringa oleifera var. PKM 1. Ultrasound-Assisted Extraction stands out as an effective approach within a relatively brief timeframe and this extraction technique reduces both processing time and temperature, making it a valuable method for extracting thermolabile biomolecules found in moringa leaves. Based on the outcome of the optimization model, at 35 °C and 30 min of extraction time under 70% hydroethanolic solvent phase system maximum biomolecules from Moringa oleifera leaves can be extracted in a very short span with higher yield and quality. These products could be a boon to various food based industries to fortify moringa biomolecules in wide range of food products along with its nutraceutical properties by adapting micro or nano encapsulation techniques.
Author contribution
AT: Methodology, Supervision, Project administration, Resources, Data curation; SG: Conceptualization, Writing original draft, Visualization, Investigation; VKR: Resources, Investigation, Review & Editing, Fromal analysis; RA: Writing, Visualization, Validation. MSA: Validation and Funding; SD: Validation, RR: Validation. TM: Editing, Fromal analysis, Validation.
Abbreviation | ||
UAE | = | Ultrasound Assisted Extraction |
RSA | = | Radical Scavenging Activity |
CCD | = | Central Composite Design |
RSM | = | Response Surface Methodology |
EY | = | Extraction Yield |
TPC | = | Total Phenol Content |
TFC | = | Total Flavonoid Content |
Revised Supplementary.docx
Download MS Word (37.3 KB)Acknowledgement
Additionally, we would like to thank the Department of Vegetable Science of HC & RI, TNAU, Periyakulam East, Theni for their assistance in organic moringa leaf cultivation and procurement of leaf samples. We would like to express our gratitude to the Department of Spices, Plantation, Medicinal and Aromatic Crops (PSMA), and the Centre for Agricultural Nanotechnology for granting us access to their well-equipped laboratory facilities. The authors express their sincere appreciation to the Researchers Supporting Project Number (RSP2024R398) King Saud University, Riyadh, Saudi Arabia.
Disclosure statement
No potential conflict of interest was reported by the author(s).
Availability of data and material
The datasets generated and/or analyzed during the research are made available upon a reasonable request from the corresponding author.
Additional information
Funding
References
- Abed, S. S., Kiranmayi, P., Imran, K., Lateef, S. S., & Abed Sr, S. S. (2023). Gas chromatography-mass spectrometry (GC-MS) metabolite profiling of Citrus limon (L.) osbeck juice extract evaluated for its antimicrobial activity against. Streptococcus mutans. Cureus, 15(1), 1. https://doi.org/10.7759/cureus.33585
- Aggarwal, K., Singh, M., & Zalpouri, R. (2021). Effect of treatment and drying method (Solar and convective) on physico-chemical quality of dried moringa leaves. International Journal of Agricultural Sciences, 17(2), 228–18. https://doi.org/10.15740/HAS/IJAS/17.2/228-233
- Ahmad, N., Zuo, Y., Lu, X., Anwar, F., & Hameed, S. (2016). Characterization of free and conjugated phenolic compounds in fruits of selected wild plants. Food Chemistry, 190, 80–89. https://doi.org/10.1016/j.foodchem.2015.05.077
- Albarri, R., & Şahin, S. (2021). Kinetics, thermodynamics, and mass transfer mechanism of the ultrasound-assisted extraction of bioactive molecules from Moringa oleifera leaves. Biomass Conversion and Biorefinery, 13(9), 7919–7926. https://doi.org/10.1007/s13399-021-01686-5
- Ali, M. A., Yusof, Y. A., Chin, N. L., Ibrahim, M. N., & Basra, S. M. A. (2014). Drying kinetics and colour analysis of Moringa oleifera leaves. Agriculture and Agricultural Science Procedia, 2, 394–400. https://doi.org/10.1016/j.aaspro.2014.11.055
- Alkafafy, M. E., Sayed, S. M., El-Shehawi, A. M., El-Shazly, S., Farouk, S., Alotaibi, S. S., Madkour, D. A., Orabi, S. H., Elbaz, H. T., & Ahmed, M. M. (2021). Moringa oleifera ethanolic extract ameliorates the testicular dysfunction resulted from HFD-induced obesity rat model. Andrologia, 53(8), e14126. https://doi.org/10.1111/and.14126
- Alves, G. H., Ferreira, C. D., Vivian, P. G., Monks, J. L. F., Elias, M. C., Vanier, N. L., & de Oliveira, M. (2016). The revisited levels of free and bound phenolics in rice: Effects of the extraction procedure. Food Chemistry, 208, 116–123. https://doi.org/10.1016/j.foodchem.2016.03.107
- Alvin, A., Miller, K. I., & Neilan, B. A. (2014). Exploring the potential of endophytes from medicinal plants as sources of antimycobacterial compounds. Microbiological Research, 169(7–8), 483–495. https://doi.org/10.1016/j.micres.2013.12.009
- Aryal, S., Baniya, M. K., Danekhu, K., Kunwar, P., Gurung, R., & Koirala, N. (2019). Total phenolic content, flavonoid content and antioxidant potential of wild vegetables from Western Nepal. Plants, 8(4), 96. https://doi.org/10.3390/plants8040096
- Baothman, O. A., Altayb, H. N., Zeyadi, M. A., Hosawi, S. B., & Abo-Golayel, M. K. (2023). Phytochemical analysis and nephroprotective potential of Ajwa date in doxorubicin-induced nephrotoxicity rats: Biochemical and molecular docking approaches. Food Science & Nutrition, 11(3), 1584–1598. https://doi.org/10.1002/fsn3.3199
- Bharali, R., Tabassum, J., & Azad, M. R. H. (2003). Chemomodulatory effect of Moringa oleifera, Lam, on hepatic carcinogen metabolising enzymes, antioxidant parameters and skin papillomagenesis in mice. Asian Pacific Journal of Cancer Prevention, 4(2), 131–140.
- Bozinou, E., Karageorgou, I., Batra, G., G. Dourtoglou, V., & I. Lalas, S. (2019). Pulsed electric field extraction and antioxidant activity determination of Moringa oleifera dry leaves: A comparative study with other extraction techniques. Beverages, 5(1), 8. https://doi.org/10.3390/beverages5010008
- Brintha, S., Rajesh, S., Renuka, R., Santhanakrishnan, V., & Gnanam, R. (2017). Phytochemical analysis and bioactivity prediction of compounds in methanolic extracts of Curculigo orchioides Gaertn. Journal of Pharmacognosy and Phytochemistry, 6(4), 192–197.
- Castillo-Lopez, R. I., Leon-Felix, J., Angulo-Escalante, M., Gutierrez-Dorado, R., Muy-Rangel, M. D., & Heredia, J. B. (2017). Nutritional and phenolic characterization of Moringa oleifera leaves grown in Sinaloa, Mexico. Pakistan Journal of Botany, 49(1), 161–168.
- Castro-López, C., Ventura-Sobrevilla, J. M., González-Hernández, M. D., Rojas, R., Ascacio-Valdés, J. A., Aguilar, C. N., & Martínez-Ávila, G. C. (2017). Impact of extraction techniques on antioxidant capacities and phytochemical composition of polyphenol-rich extracts. Food Chemistry, 237, 1139–1148. https://doi.org/10.1016/j.foodchem.2017.06.032
- Chavan, J. J., Gaikwad, N. B., Kshirsagar, P. R., & Dixit, G. B. (2013). Total phenolics, flavonoids and antioxidant properties of three Ceropegia species from Western Ghats of India. South African Journal of Botany, 88, 273–277. https://doi.org/10.1016/j.sajb.2013.08.007
- Chemat, F., Rombaut, N., Sicaire, A.-G., Meullemiestre, A., Fabiano-Tixier, A.-S., & Abert-Vian, M. (2017). Ultrasound assisted extraction of food and natural products. Mechanisms, techniques, combinations, protocols and applications. A review. Ultrasonics Sonochemistry, 34, 540–560. https://doi.org/10.1016/j.ultsonch.2016.06.035
- Cho, W.-Y., Kim, D.-H., Lee, H.-J., Yeon, S.-J., & Lee, C.-H. (2020). Journal of food quality evaluation of effect of extraction solvent on selected properties of olive leaf extract. Journal of Food Quality, 2020, 1–7. https://doi.org/10.1155/2020/3013649
- Dadi, D. W., Emire, S. A., Hagos, A. D., & Eun, J. B. (2019). Effect of ultrasound-assisted extraction of Moringa stenopetala leaves on bioactive compounds and their antioxidant activity. Food Technology and Biotechnology, 57(1), 77–86. https://doi.org/10.17113/ftb.57.01.19.5877
- Daghaghele, S., Kiasat, A. R., Safieddin Ardebili, S. M., & Mirzajani, R. (2021). Intensification of extraction of antioxidant compounds from Moringa oleifera leaves using ultrasound-assisted approach: BBD-RSM design. International Journal of Fruit Science, 21(1), 693–705. https://doi.org/10.1080/15538362.2021.1926396
- Desta, K. T., Kim, G. S., Kim, Y. H., Lee, W. S., Lee, S. J., Jin, J. S., Abd El-Aty, A., Shin, H. C., Shim, J. H., & Shin, S. C. (2016). The polyphenolic profiles and antioxidant effects of Agastache rugosa Kuntze (Banga) flower, leaf, stem and root. Biomedical Chromatography: BMC, 30(2), 225–231. https://doi.org/10.1002/bmc.3539
- Divyalakshmi, M., & Thoppil, J. (2022). Molecular docking of phytochemicals against breast cancer: a review. Plant Archives (09725210), 22(2), 163–173. https://doi.org/10.51470/PLANTARCHIVES.2022.v22.no2.029
- Dolatowski, Z. J., Stadnik, J., & Stasiak, D. (2007). Applications of ultrasound in food technology. Acta Scientiarum Polonorum Technologia Alimentaria, 6(3), 88–99.
- Evans, J. R., & Lawrenson, J. G., Cochrane Eyes and Vision Group. (2017). Antioxidant vitamin and mineral supplements for preventing age-related macular degeneration. Cochrane Database of Systematic Reviews, 2017(7), 1–52. Art. No.: CD00025. https://doi.org/10.1002/14651858.CD000253.pub4
- Ezez, D., Mekonnen, N., & Tefera, M. (2023). Phytochemical analysis of Withania somnifera leaf extracts by GC-MS and evaluating antioxidants and antibacterial activities. International Journal of Food Properties, 26(1), 581–590. https://doi.org/10.1080/10942912.2023.2173229
- Geankoplis, C. J., Hersel, A. A., & Lepek, D. H. (2018). Transport processes and separation process principles (5th ed.). Prentice Hall. ISBN 9780134181028.
- Ghafoor, K., Choi, Y. H., Jeon, J. Y., & Jo, I. H. (2009). Optimization of ultrasound-assisted extraction of phenolic compounds, antioxidants, and anthocyanins from grape (Vitis vinifera) seeds. Journal of Agricultural and Food Chemistry, 57(11), 4988–4994. https://doi.org/10.1021/jf9001439
- Ibrahim, O. H., & Abdul-Hafeez, E. Y. (2023). The acetone extract of albizia lebbeck stem bark and its in vitro cytotoxic and antimicrobial activities. Horticulturae, 9(3), 385. https://doi.org/10.3390/horticulturae9030385
- Jin, W. B., Xu, C., Cheung, Q., Gao, W., Zeng, P., Liu, J., Chan, E. W. C., Leung, Y.-C., Chan, T. H., Wong, K.-Y., Chen, S., & Chan, K.-F. (2020). Bioisosteric investigation of ebselen: Synthesis and in vitro characterization of 1, 2-benzisothiazol-3 (2H)-one derivatives as potent New Delhi metallo-β-lactamase inhibitors. Bioorganic Chemistry, 100, 103873. https://doi.org/10.1016/j.bioorg.2020.103873
- Kaderides, K., Papaoikonomou, L., Serafim, M., & Goula, A. M. (2019). Microwave-assisted extraction of phenolics from pomegranate peels: Optimization, kinetics, and comparison with ultrasounds extraction. Chemical Engineering and Processing - Process Intensification, 137, 1–11. https://doi.org/10.1016/j.cep.2019.01.006
- Karabegović, I. T., Stojičević, S. S., Veličković, D. T., Nikolić, N. Č., & Lazić, M. L. (2013). Optimization of microwave-assisted extraction and characterization of phenolic compounds in cherry laurel (Prunus laurocerasus) leaves. Separation and Purification Technology, 120, 429–436. https://doi.org/10.1016/j.seppur.2013.10.021
- Kilany, O. E., Abdelrazek, H. M. A., Aldayel, T. S., Abdo, S., & Mahmoud, M. M. A. (2020). Anti-obesity potential of Moringa olifera seed extract and lycopene on high fat diet induced obesity in male Sprauge Dawely rats. Saudi Journal of Biological Sciences, 27(10), 2733–2746. https://doi.org/10.1016/j.sjbs.2020.06.026
- Kowalczyk, P., & Kulig, K. (2014). GABA system as a target for new drugs. Current Medicinal Chemistry, 21(28), 3294–3309. https://doi.org/10.2174/0929867321666140601202158
- Lasta, H. F. B., Lentz, L., Rodrigues, L. G. G., Mezzomo, N., Vitali, L., & Ferreira, S. R. S. (2019). Pressurized liquid extraction applied for the recovery of phenolic compounds from beetroot waste. Biocatalysis and Agricultural Biotechnology, 21, 101353. https://doi.org/10.1016/j.bcab.2019.101353
- Ma, Y., Ye, X., Hao, Y., Xu, G., Xu, G., & Liu, D. (2008). Ultrasound-assisted extraction of hesperidin from Penggan (Citrus reticulata) peel. Ultrasonics Sonochemistry, 15(3), 227–232. https://doi.org/10.1016/j.ultsonch.2007.03.006
- Majumder, R., Dhara, M., Adhikari, L., Ghosh, G., & Pattnaik, S. (2019). Evaluation of in vitro antibacterial and antioxidant activity of aqueous extracts of Olax psittacorum. Indian Journal of Pharmaceutical Sciences, 81(1), 99–109. https://doi.org/10.4172/pharmaceutical-sciences.1000484
- Mason, T. J., Paniwnyk, L., & Lorimer, J. (1996). The uses of ultrasound in food technology. Ultrasonics Sonochemistry, 3(3), S253–S260. https://doi.org/10.1016/S1350-4177(96)00034-X
- Mehta, K., Balaraman, R., Amin, A., Bafna, P., & Gulati, O. (2003). Effect of fruits of Moringa oleifera on the lipid profile of normal and hypercholesterolaemic rabbits. Journal of Ethnopharmacology, 86(2–3), 191–195. https://doi.org/10.1016/s0378-8741(03)00075-8
- Michel, S., Baraka, L. F., Ibañez, A. J., & Mansurova, M. (2021). Mass spectrometry-based flavor monitoring of Peruvian chocolate fabrication process. Metabolites, 11(2), 71. https://doi.org/10.3390/metabo11020071
- Mohammad, H., Prabhu, K., Rao, M. R. K., Sundaram, R. L., Shil, S., & Vijayalakshmi, N. (2019). The GC MS studies of one Ayurvedic medicine, Amritarishtam. Research Journal of Pharmacy and Technology, 12(1), 351–356. https://doi.org/10.5958/0974-360X.2019.00064.7
- Olson, M. E., & Fahey, J. W. (2011). Moringa oleifera: a multipurpose tree for the dry tropics. Revista Mexicana de Biodiversidad, 82(4), 1071–1082. https://doi.org/10.22201/ib.20078706e.2011.4.678
- Pan, X., Niu, G., & Liu, H. (2003). Microwave-assisted extraction of tea polyphenols and tea caffeine from green tea leaves. Chemical Engineering and Processing: Process Intensification, 42(2), 129–133. https://doi.org/10.1016/S0255-2701(02)00037-5
- Pollini, L., Tringaniello, C., Ianni, F., Blasi, F., Manes, J., & Cossignani, L. (2020). Impact of ultrasound extraction parameters on the antioxidant properties of Moringa oleifera leaves. Antioxidants, 9(4), 277. https://doi.org/10.3390/antiox9040277
- Rajalakshmi, R., Vaidehi, J., & Krishnappa, K. (2022). The naturally available phyto-products of Indian medicinal plants against adulticidal activity of human vector mosquitoes. Uttar Pradesh Journal of Zoology, 43(24), 80–87. https://doi.org/10.56557/upjoz/2022/v43i243298
- Rakesh, B., Bindu, K. H., & Praveen, N. (2021). Variations in the L-DOPA content, phytochemical constituents and antioxidant activity of different germlines of Mucuna pruriens (L.) DC. Asian Journal of Chemistry, 33(8), 1881–1890. https://doi.org/10.14233/ajchem.2021.23293
- Ramić, M., Vidović, S., Zeković, Z., Vladić, J., Cvejin, A., & Pavlić, B. (2015). Modeling and optimization of ultrasound-assisted extraction of polyphenolic compounds from Aronia melanocarpa by-products from filter-tea factory. Ultrasonics Sonochemistry, 23, 360–368. https://doi.org/10.1016/j.ultsonch.2014.10.002
- Ren, J., Wang, J., Karthikeyan, S., Liu, H., & Cai, J. (2019). Natural anti-phytopathogenic fungi compound phenol, 2, 4-bis (1, 1-dimethylethyl) from Pseudomonas fluorescens TL-1. Indian Journal of Biochemistry & Biophysics, 56(2), 162–168.
- Rodríguez-Pérez, C., Gilbert-López, B., Mendiola, J. A., Quirantes-Piné, R., Segura-Carretero, A., & Ibáñez, E. (2016). Optimization of microwave-assisted extraction and pressurized liquid extraction of phenolic compounds from Moringa oleifera leaves by multiresponse surface methodology. Electrophoresis, 37(13), 1938–1946. https://doi.org/10.1002/elps.201600071
- Şahin, S. (2015). A novel technology for extraction of phenolic antioxidants from mandarin (Citrus deliciosa Tenore) leaves: Solvent-free microwave extraction. Korean Journal of Chemical Engineering, 32(5), 950–957. https://doi.org/10.1007/s11814-014-0293-y
- Santos, C. H. K., Baqueta, M. R., Coqueiro, A., Dias, M. I., Barros, L., Barreiro, M. F., Ferreira, I. C. F. R., Gonçalves, O. H., Bona, E., da Silva, M. V., & Leimann, F. V. (2018). Systematic study on the extraction of antioxidants from pinhão (araucaria angustifolia (bertol.) Kuntze) coat. Food Chemistry, 261, 216–223. https://doi.org/10.1016/j.foodchem.2018.04.057
- Sbayou, H., Oubrim, N., Bouchrif, B., Ababou, B., Boukachabine, K., & Amghar, S. (2014). Chemical composition and antibacterial activity of essential oil of Origanum compactum against foodborne bacteria. International Journal of Engineering Research and Technology, 3(1), 3562–3567.
- Senthil, J., Rameashkannan, M., & Mani, P. (2016). Phytochemical profiling of ethanolic leaves extract of Ipomoea sepiaria (Koenig Ex. Roxb). International Journal of Innovative Research in Science, Engineering and Technology, 5(3), 3140–3147.
- Setyaningsih, W., Saputro, I. E., Carrera, C. A., & Palma, M. (2019). Optimisation of an ultrasound-assisted extraction method for the simultaneous determination of phenolics in rice grains. Food Chemistry, 288, 221–227. https://doi.org/10.1016/j.foodchem.2019.02.107
- Setyani, W., Murwanti, R., Sulaiman, T. N. S., & Hertiani, T. (2023). Application of Response Surface Methodology (RSM) for the optimization of ultrasound-assisted extraction (UAE) of Moringa oleifera: Extraction yield, content of bioactive compounds, and biological effects in vitro. Plants, 12(13), 2455. https://doi.org/10.3390/plants12132455
- Sheik, A., Kim, E., Adepelly, U., Alhammadi, M., & Huh, Y. S. (2023). Antioxidant and antiproliferative activity of Basella alba against colorectal cancer. Saudi Journal of Biological Sciences, 30(4), 103609. https://doi.org/10.1016/j.sjbs.2023.103609
- Siddhuraju, P., & Becker, K. (2003). Antioxidant properties of various solvent extracts of total phenolic constituents from three different agroclimatic origins of drumstick tree (Moringa oleifera Lam.) leaves. Journal of Agricultural and Food Chemistry, 51(8), 2144–2155. https://doi.org/10.1021/jf020444+
- Singh, B. N., Singh, B., Singh, R., Prakash, D., Dhakarey, R., Upadhyay, G., & Singh, H. (2009). Oxidative DNA damage protective activity, antioxidant and anti-quorum sensing potentials of Moringa oleifera. Food and Chemical Toxicology: An International Journal Published for the British Industrial Biological Research Association, 47(6), 1109–1116. https://doi.org/10.1016/j.fct.2009.01.034
- Suslick, K. S., Hammerton, D. A., & Cline, R. E. (1986). Sonochemical hot spot. Journal of the American Chemical Society, 108(18), 5641–5642. https://doi.org/10.1021/ja00278a055
- Utharalakshmi, N., Kumar, A. G., & Narendrakumar, G. (2015). Optimization of cellulase producing Aspergillus flavus SB4 by solid state fermentation using response surface methodology (RSM)-CCD. Research Journal of Pharmacy and Technology, 8(4), 349. https://doi.org/10.5958/0974-360X.2015.00058.X
- Uysal, S., Cvetanović, A., Zengin, G., Zeković, Z., Mahomoodally, M. F., & Bera, O. (2019). Optimization of maceration conditions for improving the extraction of phenolic compounds and antioxidant effects of Momordica charantia L. leaves through response surface methodology (RSM) and artificial neural networks (ANNs). Analytical Letters, 52(13), 2150–2163. https://doi.org/10.1080/00032719.2019.1599007
- Vaishnav, A., Chandy, A., Jhade, D., & Rai, S. (2011). Pharmacognostical and Preliminary Phytochemical Studies on Moringa olifera Leaves. Research J. Pharmacognosy and Phytochemistry, 3(6), 272–274.
- Van, H. T., Tran, M. D., Tran, T. K. N., Nguyen, H. T.-D., Nguyen, N.-A., Huynh, N. T. A., Le, V. S., Nguyen, Q. H., Le, T. T., Nguyen Phi, N., & Pham, T.-V. (2023). Chemical profiles and antibacterial activity of acetone extract of two Curcuma species from Vietnam. Plant Science Today, 10(2), 83–89. https://doi.org/10.14719/pst.1993
- Vázquez-Espinosa, M., V. González-de-Peredo, A., Espada-Bellido, E., Ferreiro-González, M., Toledo-Domínguez, J. J., Carrera, C., Palma, M., & F. Barbero, G. (2019). Ultrasound-assisted extraction of two types of antioxidant compounds (TPC and TA) from black chokeberry (Aronia Melanocarpa L.): Optimization of the individual and simultaneous extraction methods. Agronomy, 9(8), 456. https://doi.org/10.3390/agronomy9080456
- Vinatoru, M. (2001). An overview of the ultrasonically assisted extraction of bioactive principles from herbs. Ultrasonics Sonochemistry, 8(3), 303–313. https://doi.org/10.1016/s1350-4177(01)00071-2
- Vongsak, B., Sithisarn, P., Mangmool, S., Thongpraditchote, S., Wongkrajang, Y., & Gritsanapan, W. (2013). Maximizing total phenolics, total flavonoids contents and antioxidant activity of Moringa oleifera leaf extract by the appropriate extraction method. Industrial Crops and Products, 44, 566–571. https://doi.org/10.1016/j.indcrop.2012.09.021
- Wang, T. Y., Li, Q., & Bi, K. S. (2018). Bioactive flavonoids in medicinal plants: Structure, activity and biological fate. Asian Journal of Pharmaceutical Sciences, 13(1), 12–23. https://doi.org/10.1016/j.ajps.2017.08.004
- Wang, Y., Gao, Y., Ding, H., Liu, S., Han, X., Gui, J., & Liu, D. (2017). Subcritical ethanol extraction of flavonoids from Moringa oleifera leaf and evaluation of antioxidant activity. Food Chemistry, 218, 152–158. https://doi.org/10.1016/j.foodchem.2016.09.058
- Wen, C., Zhang, J., Zhang, H., Dzah, C. S., Zandile, M., Duan, Y., Ma, H., & Luo, X. (2018). Advances in ultrasound assisted extraction of bioactive compounds from cash crops–A review. Ultrasonics Sonochemistry, 48, 538–549. https://doi.org/10.1016/j.ultsonch.2018.07.018
- Xie, P. J., Huang, L. X., Zhang, C. H., Ding, S. S., Deng, Y. J., & Wang, X. J. (2018). Skin-care effects of dandelion leaf extract and stem extract: Antioxidant properties, tyrosinase inhibitory and molecular docking simulations. Industrial Crops and Products, 111, 238–246. https://doi.org/10.1016/j.indcrop.2017.10.017
- Xu, Y.-B., Chen, G. L., & Guo, M. Q. (2019). Antioxidant and anti-inflammatory activities of the crude extracts of Moringa oleifera from Kenya and their correlations with flavonoids. Antioxidants, 8(8), 296. https://doi.org/10.3390/antiox8080296
- Yassin, S., Abubker, M., Mohamed, A., Omer, S., Humeada, S., Ahmed, E. M., & Alrahman, M. A. (2022). Antibacterial, Antioxidant Activities and GC-MS Analysis of Dichrostachys cinera (L.) Ethanolic Leaves Extract. Pharmacology & Pharmacy, 13(12), 545–557. https://doi.org/10.4236/pp.2022.1312039
- Yerena-Prieto, B. J., Gonzalez-Gonzalez, M., Vázquez-Espinosa, M., González-de-Peredo, A. V., García-Alvarado, M. Á., Palma, M., Rodríguez-Jimenes, G. d C., & Barbero, G. F. (2022). Optimization of an ultrasound-assisted extraction method applied to the extraction of flavonoids from Moringa leaves (Moringa oleífera Lam.). Agronomy, 12(2), 261. https://doi.org/10.3390/agronomy12020261
- Zhang, L., Zhou, C., Wang, B., Yagoub, A. E.-G A., Ma, H., Zhang, X., & Wu, M. (2017). Study of ultrasonic cavitation during extraction of the peanut oil at varying frequencies. Ultrasonics Sonochemistry, 37, 106–113. https://doi.org/10.1016/j.ultsonch.2016.12.034
- Zhao, B., Deng, J., Li, H., He, Y., Lan, T., Wu, D., Gong, H., Zhang, Y., & Chen, Z. (2019). Optimization of phenolic compound extraction from Chinese Moringa oleifera leaves and antioxidant activities. Journal of Food Quality, 2019, 1–13. https://doi.org/10.1155/2019/5346279