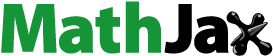
Abstract
This study utilized the Taguchi method to optimize the conditions for the hydrothermal extraction of γ-aminobutyric acid (GABA) from fermented mulberry leaves (FMLs) and evaluated the antioxidant capacity of FML extract powder (EP). Four extraction factors—the leaves-to-water ratio, temperature, duration, and pH—were considered, and a series of experiments were conducted in accordance with a Taguchi design. The results indicated that the optimal hydrothermal extraction conditions were a leaves-to-water ratio of 1:10, temperature of 100 °C, duration of 15 min, and pH of 4. Under these conditions, the GABA content of an FML infusion was 75.04 mg GABA/g EP. Further analysis revealed that the pH had the strongest impact on GABA extraction, with the corresponding signal/noise ratio ranging from 27.36 to 31.60. Additionally, experimental results for antioxidant capacity showed that the total phenolic and flavonoid content of the EP were 12.94 mg gallic acid equivalent/g EP and 81.77 mg quercetin equivalent/g EP, respectively. The IC50 values for ABTS and DPPH free-radical-scavenging ability were 0.58 mg/mL and 0.20 mg/mL, respectively. The reducing power of the EP was 81.69 mg vitamin C equivalent/g EP. These findings provide an important foundation for further applications of FMLs.
Reviewing Editor:
Introduction
Health and nutrition have become paramount concerns for certain individuals, and substances that contribute to maintaining physical and mental well-being are receiving increasing attention. γ-Aminobutyric acid (GABA) has emerged as a noteworthy topic of interest. GABA is a nonprotein amino acid and is distinct from the amino acids typically found in proteins (Ramos-Ruiz et al., Citation2018), because it does not participate in protein synthesis. It plays a completely different role in the biological system. GABA is primarily present in the nervous system (Chen et al., Citation2019), where it acts as a neurotransmitter that regulates various physiological functions, including emotions, sleep, and stress (Sarasa et al., Citation2020). Despite not being involved in protein synthesis, the significance of GABA’s regulation of physiological and neural functions cannot be overstated.
GABA not only plays important roles in mammals but also is widely distributed in the plant kingdom, and it is detectable in various organs of plants (Khan et al., Citation2021). The widespread distribution of GABA in plants indicates its diverse functions across various plant species and emphasizes the potential value of GABA in plants (Fromm, Citation2020). Notably, the GABA content of mulberry leaves is significantly higher than that of other foods, such as green tea, oolong tea, black tea (Bae et al., Citation2011), melon, tomato, potato, grape (Horie, Citation2015), etc. Mulberry leaves are not only rich in protein, vitamins, minerals, gallic acid, catechins, caffeic acid, rutin, resveratrol, and quercetin but have also been identified to have the highest GABA content among numerous foods (Li et al., Citation2022; Park et al., Citation2013).
GABA has noteworthy antioxidant properties, and antioxidants are crucial for maintaining overall health. Antioxidant capacity denotes a substance’s ability to counteract free radicals, which are unstable molecules produced in the body that can damage cells and tissues. The accumulation of excessive free radicals can lead to the development of various chronic diseases, including heart disease, diabetes, and cancer (Lobo et al., Citation2010; Phaniendra et al., Citation2015). The robust antioxidant effect of GABA contributes to the neutralization of free radicals in the body, a protective action that is vital for delaying the aging process and mitigating the aging of organs and tissues (Tu et al., Citation2022). Additionally, the antioxidant properties of GABA mean that it can reduce the risk of chronic inflammation, thereby enhancing the overall function of the immune system (Ngo & Vo, Citation2019; Phaniendra et al., Citation2015).
Mulberry leaves, with their inherently high GABA content (Bae et al., Citation2011), are a fascinating subject of research. However, to fully harness the potential of these leaves, in-depth research is needed into how to enhance their GABA levels. Anaerobic fermentation is a possible method. Anaerobic fermentation is a process in which the production of GABA in tissue is stimulated by placing the tissue in a low-oxygen or no-oxygen environment (Bae et al., Citation2011). In one study on tea leaves, this process was found to result in a tenfold increase in GABA content (Wang et al., Citation2006). Nevertheless, research on the optimal GABA extraction conditions for fermented mulberry leaves (FMLs) is insufficient.
The primary objective of this study was to optimize the conditions for extracting GABA from FMLs, thereby ensuring an efficient and sustainable extraction process. Given the multitude of extraction conditions, a mere trial-and-error approach was not practical. Therefore, the Taguchi method was employed for implementing a systematic experimental design to identify the optimal extraction conditions while saving time and resources (Fratila & Caizar, Citation2011). Once the optimal extraction conditions had been determined, the antioxidant properties of the extract—its total phenolic content (TPC), total flavonoid content (TFC), 2,2′-azino-bis(3-ethylbenzothiazoline-6-sulfonic acid) (ABTS) free radical scavenging capacity, 2,2-diphenyl-1-picrylhydrazyl (DPPH) free radical scavenging capacity, and reducing power—were evaluated. These assessments provided insights into the potential antioxidant benefits of the FML extract and its applicability in nutrition and health.
Experimental section
FMLs
In this study, FMLs were obtained through anaerobic fermentation. In brief, freshly harvested mulberry leaves were immediately transferred to stainless steel drums, where the air inside the drums was first evacuated and then replaced with nitrogen gas, creating an anaerobic environment. The leaves remained in this anaerobic environment for 8–12 h for deoxygenation. After deoxygenation and nitrogen filling were completed, the leaves were transferred to a frying pan for the panning process to heat the leaves to 200 °C for 5 min. Subsequently, the leaves were moved to a rolling table for rolling, which lasted for 5 min to form them into strips. After rolling, the leaves were placed in a dryer and dried for 1 h to get the final FMLs.
Hydrothermal extraction
This study employed a hydrothermal extraction method to extract GABA from FMLs. In Teflon cups, powdered FMLs were mixed with deionized water at leaves (powdered dried fermented leaves)-to-water ratios of 1:10, 1:20, 1:30, and 1:40. The initial pH of the solutions was then adjusted to a certain value, namely 4, 5, 6, or 7, with a hydrochloric acid solution (Pereira et al., Citation2016; Ramos-Ruiz et al., Citation2018). Each set of samples was sealed within a stainless steel sample chamber. The sample chambers were placed inside a high-temperature furnace, and the temperature was increased at a rate of 3 °C/min from room temperature to 100 °C, 120 °C, 140 °C, or 160 °C. Once the target temperature had been reached, it was maintained, and the extraction process was performed for various durations—15, 30, 45, or 60 min—to investigate the effects of the extraction time on substance exchange and extraction.
Subsequently, the sample chambers were retrieved, and each extraction liquid was filtered and subjected to freeze-drying to obtain an extract powder (EP). The hydrothermal extraction yield was defined as the percentage of EP mass relative to FML mass:
Taguchi method
During hydrothermal extraction, excessively high temperature and prolonged exposure to heat may lead to the degradation of GABA (Toyoizumi et al., Citation2021). The Taguchi method was employed to optimize the conditions of FML hydrothermal extraction (Fernández et al., Citation2014), with the aim of maximizing the GABA content of the extract. The Taguchi method is a multifactorial experimental design approach that considers variations in different parameters, which, in the present context, were the leaves-to-water ratio, temperature, duration, and pH. This approach allows us to identify the optimal hydrothermal extraction conditions to maximize the efficiency of GABA extraction while minimizing factors that could have adverse effects on GABA. Additionally, the Taguchi method reduces the number of experimental iterations, saving both time and resources (Fratila & Caizar, Citation2011).
Specifically, an L16 Taguchi orthogonal array () was designed in this study, including various combinations of leaves-to-water ratios, temperature, duration, and pH. These combinations were utilized in practical hydrothermal extraction experiments. GABA content analysis was conducted on the FML infusions obtained under different conditions to determine the efficiency of GABA extraction in each scenario. The signal-to-noise (S/N) ratio was employed to assess the performance of each combination and identify which factor had the greatest positive impact on GABA content on the basis of the ‘the larger the better’ characteristic (Yeh & Tsai, Citation2014). Through this methodology, this study identified the optimal hydrothermal extraction conditions that maximized GABA content while ensuring the quality and applicability of mulberry leaves.
Table 1. Taguchi orthogonal array for obtaining FML extracts.
Analysis of GABA content
A previously reported method (Henderson et al., Citation2000) was employed to quantitatively determine the GABA content of the extract. This method involved high-performance liquid chromatography (HPLC) performed on an Agilent 1100 series HPLC system equipped with an Eclipse–AAA column (150 mm, 5 μm). Two mobile phases were utilized; mobile phase A, NaH2PO4, and mobile phase B, a mixture of methanol, acetonitrile, and water in the ratio 45:45:10 (v/v/v). During the experiment, 10 µL of the sample solution was injected at a flow rate at 2 mL/min, and detection was conducted at a wavelength of 338 nm. The optimal analysis conditions were assessed for their appropriateness, which was characterized by the reproducibility of all variable values, a relative standard deviation (RSD) of <3%, a recovery rate of 100% ± 10%, and detection and quantification limits reaching S/N ratios of 3/1 and 10/1, respectively.
Analysis of TPC
The experimental method was adapted from the literature (Huang et al., Citation2013; Wu et al., Citation2019; Wu et al., Citation2021) with slight modifications. Initially, 200 μL of an FML infusion was mixed uniformly with 200 μL of 0.3 M Folin–Ciocalteu phenol reagent, and the mixture was then allowed to stand for 5 min. Subsequently, 200 μL of 10% sodium carbonate solution was added to the mixture, which was vigorously vortexed for 1 min to ensure thorough mixing. Subsequently, 400 μL of deionized water was introduced, and the reaction was allowed to proceed at room temperature in the dark for 1 h.
After the 1-h reaction period, the liquid was left undisturbed for 10 min. Then, the mixture was centrifuged at 3000 rpm for 10 min, after which 200 μL of the supernatant was carefully extracted. Finally, a spectrophotometer was employed to measure absorbance at a wavelength of 700 nm. For quantification, gallic acid was used as the standard in the calculations of the TPC of the EP samples; thus, TPC is expressed in terms of the gallic acid equivalent (GAE) per gram of EP.
Analysis of TFC
This study followed a previously reported method (Chlopicka et al., Citation2012; Chan et al., Citation2018; Lin et al., Citation2020). Initially, 50 μL of FML infusion was mixed with 40 μL of 99.9% methanol and 50 μL of 5% w/v NaNO2, and for the reaction, the mixture was allowed to stand for 5 min. Then, 10% w/v AlCl3 was added, and the solution was thoroughly mixed, after which it was incubated for 6 min. Subsequently, 100 μL of 1 M NaOH was added, and the mixture was allowed to stand for 1 h. Afterward, absorbance was measured at a wavelength of 510 nm using a spectrophotometer. For quantification, quercetin was used as the standard. The quercetin standard curve was prepared for the calculation of the TFC of the EP samples, which is expressed in terms of the quercetin equivalent (QE) per gram of EP.
Analysis of ABTS free-radical-scavenging activity
The experimental procedure followed a previously reported method (Huang et al., Citation2014; Huang et al., Citation2020). Initially, 250 μL of 7 mM ABTS and 250 μL of 2.45 mM potassium persulfate were mixed uniformly. Then, the mixture was allowed to react in the dark at 4 °C for 16 h. Then, the reaction mixture was diluted with deionized water to achieve an absorbance reading of 0.7 ± 0.05 at a wavelength of 734 nm.
Subsequently, 180 μL of the adjusted ABTS solution was mixed with 180 μL of the FML infusion, and the reaction was allowed to proceed at room temperature in the dark for 10 min. Subsequently, absorbance was measured at 734 nm. A lower absorbance value indicated a stronger ability to inhibit ABTS free radicals. Deionized water was used as a control in the experiment. The ABTS radical scavenging capacity of an FML infusion was calculated as follows:
Analysis of DPPH free-radical-scavenging activity
The experimental procedure followed a previously reported method (Chang et al., Citation2020; Ruiz-Ciau et al., Citation2017; Tsai et al., Citation2013). Initially, 180 μL of FML infusion was uniformly mixed with 180 μL of 198 µM DPPH solution and allowed to stand in the dark for 30 min. Absorbance was then measured at 517 nm using a spectrophotometer. Deionized water was used as a control in the experiment. The DPPH free-radical-scavenging capacity of an FML infusion was calculated as follows:
Analysis of reducing power
The experimental procedure followed a previously reported method (Chen et al., Citation2021; Wang et al., Citation2021). Initially, 200 μL of FML infusion was uniformly mixed with 100 μL of 0.2 M phosphate buffer solution (pH 6.6) and 100 μL of 1% K3(FeCN)6 solution. The mixture was then placed in a 50 °C water bath for 20 min, after which it was cooled at room temperature for 3 min. Subsequently, 100 μL of 10% trichloroacetic acid was added, and the mixture was vigorously shaken for 10 min; its absorbance was then detected at a wavelength of 700 nm. Higher absorbance indicated higher reducing power. The standard curve of vitamin C was generated to calculate the reducing power of the EP samples, which is expressed in terms of the vitamin C equivalent (VCE) per gram of EP.
Results and discussion
presents the extraction yields for the EP extracted from FML infusions; these yields ranged from 5.5% to 30.9%. The highest extraction yield, achieved in Group 4, was obtained for a leaves-to-water ratio of 1:10, temperature of 160 °C, extraction duration of 60 min, and pH of 7. The lowest extraction yield, recorded in Group 14, was obtained for a leaves-to-water ratio of 1:40, temperature of 120 °C, extraction duration of 45 min, and pH of 4.
Table 2. GABA content for groups in L16 Taguchi orthogonal array.
Regarding GABA content (), significant differences in GABA content were found between the different sample groups. The highest was 75.04 mg GABA/g EP (Group 1), whereas the lowest was 20.88 mg GABA/g EP (Group 9). The variation was thus substantial at 54.16 mg GABA/g EP. Compared to the leaves-derived sources of GABA like green tea, oolong tea, and black tea (Bae et al., Citation2011), a significantly higher GABA content was observed in this study, possibly attributed to differences in the fermentation process.
As revealed by the factor response table presented in , Factor D (pH) had the strongest main effect on GABA content, with S/N ratios ranging from 27.36 to 31.60. This observation indicates that pH is the primary factor influencing GABA extraction. Factor C (duration) was the second most important influencing parameter, with S/N ratios ranging from 28.37 to 30.60 and the second-largest magnitude from level 1 to level 4. GABA content gradually decreased with duration from 15 to 45 min but slightly increased for a duration of 60 min.
Table 3. Response table for the S/N ratio in GABA extraction.
A similar pattern was obtained for Factor B (temperature), with S/N ratios ranging from 28.79 to 30.68. The GABA extraction rate gradually decreased as the temperature was increased from 100 °C to 140 °C, a result similar to a previous finding (Toyoizumi et al., Citation2021) due to huge degradation of GABA (Jirasatid & Nopharatana, Citation2021), but the rate increased slightly when the temperature was raised to 160 °C. Factor A (leaves-to-water ratio) had the weakest impact, with S/N ratios ranging from 28.92 to 30.01. GABA extraction gradually decreased as the leaves-to-water ratio was changed from 1:10 to 1:20 and was stabilized between 1:20 and 1:40.
In summary, these results highlight the influence of various factors on GABA content, the differences in GABA content between different sample groups, and the strong impact of pH on GABA content.
TPC and TFC play important roles in the antioxidant capacity of a substance. In this study, gallic acid was used as the standard to measure the TPC of samples. Higher absorbance indicates higher TPC. The absorbance value of the EP was compared with the calibration curve of gallic acid, and the TPC of the EP was thereby calculated to be 12.94 mg GAE/g EP (). According to a study (Panyatip et al., Citation2022), the phenolic compounds present in the EP may include gallic acid, caffeic acid, gentisic acid, ellagic acid, and catechin. A similar procedure was employed to determine the TFC of the EP, and quercetin was chosen as the standard. The results () revealed that the TFC of the EP was 81.77 mg QE/g EP, which is similar to the finding in another study (Hao et al., Citation2018).
The ABTS cation radical is commonly used to assess the antioxidant capacity of samples (Re et al., Citation1999). ABTS cation radicals appear blue-green, but they lose their color when they are reduced by antioxidants. By measuring the reduction of ABTS cation radicals for various EP concentrations, a calibration curve was obtained. The IC50 value is the concentration at which a sample can achieve a 50% reduction in the number of free radicals. A lower IC50 value indicates that a sample has a higher antioxidant capacity. The IC50 value of the EP for scavenging of ABTS free radicals was calculated as being 0.58 mg/mL ().
DPPH was used as a free-radical-scavenging indicator. DPPH appears deep purple but turns pale yellow after it has reacted with antioxidants. In this study, different concentrations of the EP were tested, and a calibration curve was thereby obtained for calculating the IC50 value of the EP. According to the calculation result in , the DPPH free-radical-scavenging capacity IC50 of the EP was 0.20 mg/mL.
This study evaluated the reducing power of the EP and compared it with vitamin C as a standard. The results showed that with increasing EP concentrations, the reducing power of the EP increased. The reducing power of the EP was found to be 81.69 mg VCE/g EP ().
summarizes the phytochemical properties of EP in the study. The results indicated that EP has excellent antioxidant capabilities in terms of its TPC, TFC, ABTS free-radical-scavenging capacity, DPPH free-radical-scavenging capacity, and reducing power. These findings are consistent and confirm the antioxidant properties of EP. Specifically, EP contains abundant phenolic and flavonoid compounds that effectively neutralize and eliminate ABTS and DPPH free radicals, and EP thus exhibits considerable reducing power. Phenolic and flavonoid compounds play crucial protective roles in combating oxidative damage. In summary, this study determined the optimal conditions for the hydrothermal extraction of EP, such that its antioxidant abilities are maximized. This discovery can contribute to further research and the applications of FML infusions for their natural antioxidant content.
Table 4. Phytochemical properties of optimal EP in the study.
Conclusions
This study investigated the optimal conditions for GABA extraction from FMLs, and the results revealed that under conditions of a leaves-to-water ratio of 1:10, temperature of 100 °C, duration of 15 min, and pH of 4, the highest GABA content of 75.04 mg GABA/g EP could be obtained. An appropriate pH value was found to be crucial to high GABA extraction efficiency. Further antioxidant experiments revealed that EP from FMLs is rich in phenolic and flavonoid compounds and exhibits considerable antioxidant activity. These findings indicate that EP has potential applications in areas such as cosmetics, skincare products, and health supplements. Overall, this study conducted an in-depth analysis of the optimal extraction conditions and antioxidant properties of EP, offering valuable insights for the further development and utilization of EP from FMLs.
Authors contributions
Conceptualization: Yung-Sheng Lin and Chia-Hung Shih; investigation: Yung-Sheng Lin and Chia-Hung Shih; data curation: Ji-Hong Zheng, Cheng-You Chen, and Chiu-Hsun Liao; funding acquisition and supervision: Yung-Sheng Lin and Chia-Hung Shih; writing – original draft: Yung-Sheng Lin and Chia-Hung Shih; writing – review and editing: Yung-Sheng Lin and Chia-Hung Shih. All authors have read and agreed to the published version of the manuscript.
Ethical approval
Ethical approval was not required for the study.
Disclosure statement
No potential conflict of interest was reported by the author(s).
Data availability statement
Data and materials are available on request.
Additional information
Funding
Notes on contributors
Yung-Sheng Lin
Dr. Yung-Sheng Lin is a professor in Department of Chemical Engineering, National United University, Taiwan. He specializes in biomaterials and functional analysis in plant.
Ji-Hong Zheng
Ji-Hong Zheng holds a master’s degree in Department of Chemical Engineering, National United University, Taiwan.
Cheng-You Chen
Dr. Cheng-You Chen is a postdoctoral researcher and an adjunct assistant professor in Department of Chemical Engineering, National United University, Taiwan. He specializes in natural product extraction and detection technique.
Chiu-Hsun Liao
Chiu-Hsun Liao is an associate researcher in Miaoli District Agricultural Research and Extension Station, Ministry of Agriculture, Taiwan. She also holds a doctor’s degree in Ph.D. Program in Materials and Chemical Engineering, National United University, Taiwan.
Chia-Hung Shih
Dr. Chia-Hung Shih is the researcher and deputy director in Miaoli District Agricultural Research and Extension Station, Ministry of Agriculture, Taiwan. He is also an adjunct assistant professor in Department of Chemical Engineering, National United University, Taiwan. His area of research interest is agronomic and horticulture crops cultivation improvement, postharvest treatment and management.
References
- Bae, H., Baek, H., Park, H.-. I., Choung, M.-G., Sohn, E.-H., Kim, S.-H., Kim, D.-S., Chung, I.-M., Seong, E.-S., Yu, C.-Y., & Lim, J. D. (2011). Effect of fermentation time on the chemical composition of mulberry (Morus alba L.) leaf teas. Korean Journal of Medicinal Crop Science, 19(4), 1–9. https://doi.org/10.7783/KJMCS.2011.19.4.276
- Chan, C.-F., Wu, C.-T., Huang, W.-Y., Lin, W.-S., Wu, H.-W., Huang, T.-K., Chang, M.-Y., & Lin, Y.-S. (2018). Antioxidation and melanogenesis inhibition of various Dendrobium tosaense extracts. Molecules, 23(7), 1810. https://doi.org/10.3390/molecules23071810
- Chang, M.-Y., Lin, Y.-Y., Chang, Y.-C., Huang, W.-Y., Lin, W.-S., Chen, C.-Y., Huang, S.-L., & Lin, Y.-S. (2020). Effects of infusion and storage on antioxidant activity and total phenolic content of black tea. Applied Sciences, 10(8), 2685. https://doi.org/10.3390/app10082685
- Chen, C., Zhou, X., He, J., Xie, Z., Xia, S., & Lu, G. (2019). The roles of GABA in ischemia-reperfusion injury in the central nervous system and peripheral organs. Oxidative Medicine and Cellular Longevity, 2019, 4028394. https://doi.org/10.1155/2019/4028394
- Chen, C.-Y., Shih, C.-H., Lin, T.-C., Zheng, J.-H., Hsu, C.-C., Chen, K.-M., Lin, Y.-S., & Wu, C.-T. (2021). Antioxidation and tyrosinase inhibitory ability of coffee pulp extract by ethanol. Journal of Chemistry, 2021, 8649618. https://doi.org/10.1155/2021/8649618
- Chlopicka, J., Pasko, P., Gorinstein, S., Jedryas, A., & Zagrodzki, P. (2012). Total phenolic and total flavonoid content, antioxidant activity and sensory evaluation of pseudocereal breads. LWT-Food Science and Technology, 46(2), 548–555. https://doi.org/10.1016/j.lwt.2011.11.009
- Fernández, M. B., Burnet, M. A., Perez, E. E., Crapiste, G. H., & Nolasco, S. M. (2014). Taguchi’s methodology for determining optimum operating conditions in hydrothermal pretreatments applied to canola seeds. The Canadian Journal of Chemical Engineering, 92(7), 1239–1246. https://doi.org/10.1002/cjce.21995
- Fratila, D., & Caizar, C. (2011). Application of Taguchi method to selection of optimal lubrication and cutting conditions in face milling of AlMg3. Journal of Cleaner Production, 19(6-7), 640–645. https://doi.org/10.1016/j.jclepro.2010.12.007
- Fromm, H. (2020). GABA signaling in plants: Targeting the missing pieces of the puzzle. Journal of Experimental Botany, 71(20), 6238–6245. https://doi.org/10.1093/jxb/eraa358
- Hao, J.-Y., Wan, Y., Yao, X.-H., Zhao, W.-G., Hu, R.-Z., Chen, C., Li, L., Zhang, D.-Y., & Wu, G.-H. (2018). Effect of different planting areas on the chemical compositions and hypoglycemic and antioxidant activities of mulberry leaf extracts in Southern China. PLoS One, 13(6), e0198072. https://doi.org/10.1371/journal.pone.0198072
- Henderson, J. W., Ricker, R. D., Bidlingmeyer, B. A., & Woodward, C. (2000). Rapid, accurate, sensitive, and reproducible HPLC analysis of amino acids: Amino acid analysis using Zorbax Eclipse-AAA columns and the Agilent 1100 HPLC. (Part No. 5980–1193E). Agilent Technologies.
- Horie, N. (2015). GRAS exemption claim for gamma-aminobutyric acid (GABA) for use as a food ingredient in the United States (U.S.), Pharma Foods International, GRAS Notice No. 595.
- Huang, S.-L., Wang, W.-H., Zhong, X.-Y., Lin, C.-T., Lin, W.-S., Chang, M.-Y., & Lin, Y.-S. (2020). Antioxidant properties of Jatropha curcas L. seed shell and kernel extracts. Applied Sciences, 10(9), 3279. https://doi.org/10.3390/app10093279
- Huang, W. Y., Lin, Y. R., Ho, R. F., Liu, H. Y., & Lin, Y. S. (2013). Effects of water solutions on extracting green tea leaves. The Scientific World Journal, 2013, 368350.https://doi.org/10.1155/2013/368350
- Huang, W.-Y., Lee, P.-C., Hsu, J.-C., Lin, Y.-R., Chen, H.-J., & Lin, Y.-S. (2014). Effects of water quality on dissolution of yerba mate extract powders. The Scientific World Journal, 2014, 768742. https://doi.org/10.1155/2014/768742
- Jirasatid, S., & Nopharatana, M. (2021). Thermal kinetics of gamma–aminobutyric acid and antioxidant activity in germinated red jasmine rice milk using Arrhenius, Eyring-Polanyi and Ball models. Current Research in Nutrition and Food Science Journal, 9(2), 700–711. https://doi.org/10.12944/CRNFSJ.9.2.33
- Khan, M. I. R., Jalil, S. U., Chopra, P., Chhillar, H., Ferrante, A., Khan, N. A., & Ansari, M. I. (2021). Role of GABA in plant growth, development and senescence. Plant Gene, 26, 100283. https://doi.org/10.1016/j.plgene.2021.100283
- Li, R., Zhu, Q., Wang, X., & Wang, H. (2022). Mulberry leaf polyphenols alleviated high-fat diet-induced obesity in mice. Frontiers in Nutrition, 9, 979058. https://doi.org/10.3389/fnut.2022.979058
- Lin, Y.-S., Lin, W.-S., Tung, J.-W., Cheng, Y.-C., Chang, M.-Y., Chen, C.-Y., & Huang, S.-L. (2020). Antioxidant capacities of jujube fruit seeds and peel pulp. Applied Sciences, 10(17), 6007. https://doi.org/10.3390/app10176007
- Lobo, V., Patil, A., Phatak, A., & Chandra, N. (2010). Free radicals, antioxidants and functional foods: Impact on human health. Pharmacognosy Reviews, 4(8), 118–126. https://doi.org/10.4103/0973-7847.70902
- Ngo, D. H., & Vo, T. S. (2019). An updated review on pharmaceutical properties of gamma-aminobutyric acid. Molecules, 24(15), 2678. https://doi.org/10.3390/molecules24152678
- Panyatip, P., Padumanonda, T., Yongram, C., Kasikorn, T., Sungthong, B., & Puthongking, P. (2022). Impact of tea processing on tryptophan, melatonin, phenolic and flavonoid contents in mulberry (Morus alba L.) leaves: Quantitative analysis by LC-MS/MS. Molecules, 27(15), 4979. https://doi.org/10.3390/molecules27154979
- Park, E., Lee, S. M., Lee, J., & Kim, J. H. (2013). Anti-inflammatory activity of mulberry leaf extract through inhibition of NF-κB. Journal of Functional Foods, 5(1), 178–186. https://doi.org/10.1016/j.jff.2012.10.002
- Pereira, P. H. F., Oliveira, T. Í. S., Rosa, M. F., Cavalcante, F. L., Moates, G. K., Wellner, N., Waldron, K. W., & Azeredo, H. M. C. (2016). Pectin extraction from pomegranate peels with citric acid. International Journal of Biological Macromolecules, 88, 373–379. https://doi.org/10.1016/j.ijbiomac.2016.03.074
- Phaniendra, A., Jestadi, D. B., & Periyasamy, L. (2015). Free radicals: Properties, sources, targets, and their implication in various diseases. Indian Journal of Clinical Biochemistry, 30(1), 11–26. https://doi.org/10.1007/s12291-014-0446-0
- Ramos-Ruiz, R., Poirot, E., & Flores-Mosquera, M. (2018). GABA a non-protein amino acid ubiquitous in food matrices. Cogent Food & Agriculture, 4(1), 1534323. https://doi.org/10.1080/23311932.2018.1534323
- Re, R., Pellegrini, N., Proteggente, A., Pannala, A., Yang, M., & Rice-Evans, C. (1999). Antioxidant activity applying an improved ABTS radical cation decolorization assay. Free Radical Biology & Medicine, 26(9-10), 1231–1237. https://doi.org/10.1016/s0891-5849(98)00315-3
- Ruiz-Ciau, D., Cuevas-Glory, L., Quijano, L., & Sauri-Duch, E. (2017). Chemical composition and antioxidant dpph activity of the floral and leaves essential oils of Cmontanoa speciosa DC. American Journal of Plant Sciences, 8(4), 745–753. https://doi.org/10.4236/ajps.2017.84052
- Sarasa, S. B., Mahendran, R., Muthusamy, G., Thankappan, B., Selta, D. R. F., & Angayarkanni, J. (2020). A brief review on the non-protein amino acid, gamma-amino butyric acid (GABA): Its production and role in microbes. Current Microbiology, 77(4), 534–544. https://doi.org/10.1007/s00284-019-01839-w
- Toyoizumi, T., Kosugi, T., Toyama, Y., & Nakajima, T. (2021). Effects of high-temperature cooking on the gamma-aminobutyric acid content and antioxidant capacity of germinated brown rice (Oryza sativa L.). CyTA – Journal of Food, 19(1), 360–369. https://doi.org/10.1080/19476337.2021.1905721
- Tsai, C.-C., Chan, C.-F., Huang, W.-Y., Lin, J.-S., Chan, P., Liu, H.-Y., & Lin, Y.-S. (2013). Applications of Lactobacillus rhamnosus spent culture supernatant in cosmetic antioxidation, whitening and moisture retention applications. Molecules, 18(11), 14161–14171. https://doi.org/10.3390/molecules181114161
- Tu, J., Jin, Y., Zhuo, J., Cao, X., Liu, G., Du, H., Liu, L., Wang, J., & Xiao, H. (2022). Exogenous GABA improves the antioxidant and anti-aging ability of silkworm (Bombyx mori). Food Chemistry, 383, 132400. https://doi.org/10.1016/j.foodchem.2022.132400
- Wang, H. F., Tsai, Y. S., Lin, M. L., & Ou, A. S. M. (2006). Comparison of bioactive components in GABA tea and green tea produced in Taiwan. Food Chemistry, 96(4), 648–653. https://doi.org/10.1016/j.foodchem.2005.02.046
- Wang, W.-H., Lin, W.-S., Shih, C.-H., Chen, C.-Y., Kuo, S.-H., Li, W.-L., & Lin, Y.-S. (2021). Functionality of silk cocoon (Bombyx mori L.) sericin extracts obtained through high-temperature hydrothermal method. Materials, 14(18), 5314. https://doi.org/10.3390/ma14185314
- Wu, C.-T., Agrawal, D. C., Huang, W.-Y., Hsu, H.-C., Yang, S.-J., Huang, S.-L., & Lin, Y.-S. (2019). Functionality analysis of spent coffee ground extracts obtained by the hydrothermal method. Journal of Chemistry, 2019, 4671438. https://doi.org/10.1155/2019/4671438
- Wu, C.-T., Wang, W.-H., Lin, W.-S., Hu, S.-Y., Chen, C.-Y., Chang, M.-Y., Lin, Y.-S., & Li, C.-P. (2021). Effects of different Chenopodium formosanum parts on antioxidant capacity and optimal extraction analysis by Taguchi method. Materials, 14(16), 4679. https://doi.org/10.3390/ma14164679
- Yeh, J. H., & Tsai, T. N. (2014). Optimizing the fine-pitch copper wire bonding process with multiple quality characteristics using a grey-fuzzy Taguchi method. Microelectronics Reliability, 54(1), 287–296. https://doi.org/10.1016/j.microrel.2013.09.001