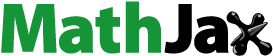
Abstract
The discharge of untreated wastewater become a major threat to the environment and posed health risk to human. Thus, in this study a low-cost and eco-friendly bio-sorbent produced from cheap and locally available material of Eucalyptus camaldulensi was used for removing toxic heavy metals from wastewater. The bio-sorbent was studied for its capacity of removing total Pb, Cd and Cr from synthetic and industrial wastewater of textile and leather industries. Parameters such as the effect of particle size, contact time, pH, and amount of adsorbent and initial concentration of metal ions were examined using the batch method. The removal of metal ions was mainly dependent on the physico-chemical nature of the adsorbent and concentration of the adsorbate. Maximum removal for Pb (II), Cd (II) and Cr (III) were observed at pH 10.0 for Pb and Cr and pH 12.0 for Cd, respectively. The experimental data were analyzed based on Langmuir and Freundlich isotherm models and Langmuir was found to be best suited for the studied bio-sorbent. At the optimum pH and particle size studied, the adsorption capacity (mg/L) of the bio-sorbent was obtained to be 9.259, 6.173 and 47.619 for Pb, Cd and Cr. Thus, the finding suggests that the Eucalyptus camaldulensis bio-sorbent can be employed as an alternative low-cost adsorbent in the removal of Pb, Cd and Cr from wastewater.
PUBLIC INTEREST STATEMENT
An increase in the industrialization and urbanization in the world posed a major problem of water contamination through the release of poisonous heavy metals from chemical industries such as tannery, textile, chemical industries and others. Thus, it is common to obtain high levels of heavy metals from the discharge of untreated industrial wastewater which pollutes the ground and surface water once released to the environment. Therefore, several methods have been used for the treatment of wastewater effluents. Most of the methods employed were associated with their high costs, less efficiency, requiring large amounts of reagent and solvent and generation of toxic sludge. Thus, the researchers were interested and worked on the development of cheaper, environment-friendly and efficient heavy metal treatment techniques to minimize the amount of toxic heavy metals discharged from industrial effluents.
Competing Interests
The authors declares no competing interests.
1. Introduction
Environmental pollution due to heavy metal is severe and complex that has been received attention due to its toxicological effects on the environment and human health, even at very low concentrations. An increase in the industrialization and urbanization rate of the world posed a major problem of water contamination through the release of poisonous heavy metals which are often found in chemical industries such as tannery, textile, cement, metallurgy, photographic material and corrosive paints (Malkoc, Nuhoglu, & Abali, Citation2006). Thus, high levels of heavy metals were obtained in untreated industrial wastewater which pollutes the ground and surface water once it is discharged to the environment. Among these toxic metals; arsenic (As), chromium (Cr), copper (Cu), zinc (Zn), aluminum (Al), cadmium (Cd), lead (Pb), nickel (Ni), mercury(Hg), and silver (Ag) are the major ones (El Nemr, Khaled, Abdelwahab, & El-Sikaily, Citation2008). The non-biodegradability nature of heavy metals and their tendency to bioaccumulate in living organisms causes several diseases, disorders and fatality (Galal-Gorchev, Ozolins, & Bonnefoy, Citation1993). Therefore, the removal of toxic heavy metals from industrial wastewater is primary importance in terms of the protection of public health and environment.
Currently, several methods are used for the treatment of wastewater effluents. Among these includes precipitation with coagulation and flocculation, ion exchange (Eltayeb & Khan, Citation2019; Khan et al., Citation2019; Khan, Khan, Asiri, & Sirajuddin, Citation2018; Khan, Khan, Rahman, & Asiri, Citation2016; Rangreez, Inamuddin, Mu, & Ali, Citation2015; Khan et al., Citation2014, Citation2013, Khan and khan, Citation2010; Khan Ali, Habiba, & Khan, Citation2009), complexation of dry biomass, filtration and adsorption (Çalımlı et al., Citation2019, Demirbaş et al. Citation2019a and Demirbaş et al. Citation2019b; Metcalf and Eddy, Citation2003). But, some of these methods such as precipitation and ion exchange have significant drawbacks which are associated with their high costs, less efficiency, requiring large amounts of reagent and solvent and generation of toxic sludge. Therefore, there is a great demand for developing cheaper, environment-friendly and efficient heavy metal treatment procedures to minimize the amount of toxic heavy metals from industrial effluents (Isloor et al., Citation2019; Kolangare, Isloor, Asiri, & Ismail, Citation2019; Kumar et al., Citation2019; Rangreez et al., Citation2015; Tchobanoglous, Burton, & Stensel, Citation2003).
Adsorption using activated carbon is the most widely used technique for the removal of toxic heavy metals (Amin et al., Citation2006; Ahmed, Yamin, Ansari, & Hasany, Citation2006; Aloko & Afolabi, Citation2007). The primary significant characteristic of this magnificent adsorbent is its high porosity nature which results in larger surface areas to bind the toxic heavy metals. Thus, adsorption is the most prevalent and effective physical working method for the treatment of toxic heavy metal from contaminated waters. But, the effectiveness and economic feasibility of the treatment process lies largely on the cost of bio-sorbent (Ghodbane & Hamdaoui, Citation2008). Moreover, the use of activated carbon is not chosen because of its high-cost values (Fu and Wang, Citation2011). Therefore, there is a need in the designing and preparing of low-cost adsorbents using locally available bio-sorbent materials.
Eucalyptus camaldulensis (E. camaldulensis), which is readily available and used as a major energy source by the local communities, can be used in the removal of toxic heavy metals from industrial wastewaters. Therefore, the objective of this study was to investigate the bio-sorbent prepared from E. camaldulensis as an alternative choice of commercial activated carbon in the removal of Pb, Cd and Cr from aqueous solution and industrial wastewater in batch mode studies; study the influence of various parameters such as pH, initial concentration of metal ion, contact time and adsorbent dose on the removal efficiency of the bio-sorbent and determine the best-fit isotherm equation, the experimental equilibrium adsorption data using Langmuir and Freundlich isotherm models.
2. Materials and methods
2.1. Preparation of activated E. camaldulensis carbon
The granulated activated carbons were selected from two raw materials, namely E. camaldulensis activated carbon (ECAC) and commercial activated carbon (CAC) where the CAC from literature was used for comparative study. The local activated carbon, ECAC was obtained from local village Dangla, near Bahr Dar, Amhara region, Ethiopia, where it is mainly used as an energy source and prepared traditionally by the local community. The local activated carbon was made from dry wood logs of E. camaldulensis. Before the application of the charcoal to biosorption, it was washed several times with distilled water to remove dust and some other residuals. The washed sample was dried at room temperature until it reached a constant weight and then activated with 2 M H2SO4 at room temperature for 24 hours. The activated carbon was washed repeatedly with distilled water until the pH reaches 7. Finally, the washed ECAC was oven-dried for about five hours at 120 °C and stored in an airtight reagent bottle and used for adsorption studies.
2.2. Chemicals
The chemicals used were: 70% HNO3 (BDH, England), 37% HCl (Riedel-de Hä en, Germany), NaOH (Rankem, Indian), K2Cr2O7(Himedia, Mumbai, India), H2SO4 (Himedia, Mumbai, India) and calibration standards SPECTROSCAN, (Industrial Analytical (pty) Ltd, South Africa) for the metals Pb, Cr and Cd. De-ionized water obtained from a Millipore® Milli-Q system was used throughout this work. All the chemicals and reagents used were of analytical grades.
2.3. Preparation of metal solutions
Single element working solutions containing 0.25 ppm for each Pb, Cr and Cd were prepared by transferring 0.25 mL of 1000 mg/L stock solutions into 1000 mL volumetric flask and diluting to content to the final volume with distilled and deionized water. Aqueous solutions of the standards were further prepared by serial dilution with distilled and deionized water.
2.4. Instruments
A drying oven with forced air and timer (FED 53, USA) was used for drying samples and the pH of the solutions was measured with a pH meter (HANNAHI99) and calibrated with buffers of pH 2, 4, and 7. The solutions were shaken with a B_Hler Horizontal Shaker (Fisher Scientific), which was a thermostatic electronic shaker. Scanning electron microscope (SEM XL30 FEG, Belgium,) was used to study the surface physical morphology of the adsorbent material.
A Varian AA240 FS Fast Sequential Atomic Absorption Photometry, which is fully automated PC-controlled true double-beam Atomic Absorption spectrometer with the Fast Sequential operation for fast multi-element flame AA determinations, features 4 lamp positions and automatic lamp selection, Operated with SpectrAA Base and PRO software versions were used during the experiment.
2.5. Adsorption studies
2.5.1. Batch adsorption experiments
The adsorption of Cr, Cd and Pb were investigated in batch equilibrium experiments. Stock solutions of metal ions were prepared from the standard solutions using the de-ionized water. The pH was adjusted with 0.1M HCl or NaOH to range from 2 to 12 before using the batch experiment. The experiments were carried out in 250 mL conical flasks containing 100 mL of metal ions solutions of different concentrations and adsorbent dose shaking for 2 h at 200 rpm in a Horizontal shaker. After the adsorption process, the sample was settled for certain minutes then the bio-sorbent was separated from the samples by filtering using Watchman No 42 filter paper and the filtrate collected was used for analysis. The filtrate was kept in a refrigerator at 4 °C until analysis. The effects on adsorption of the heavy metals (Cd, Cr and Pb) onto eucalyptus activate carbon for different parameters such as particle size (0.063, 0.1, 0.25, 0.5, 1, 2 mm), bio-sorbent dose (0, 0.5, 1, 2, 3 and 4 g), initial metal concentrations (0, 0.25, 0.5, 1, 1.5 and 2 mg/L), contact time (10, 30, 60, 90 and 120 min) was studied at different pH in a batch mode of operation. The amount of heavy metals adsorbed per weight unit of bio-sorbent was calculated using the equation given below:
Where Co and Ce are the heavy metal concentration measured before and after adsorption (mg/L), V is the volume of aqueous solution (L) and W is the dry weight of the adsorbent (g). Two replicates per sample were done and the average result was used for analysis. By quantifying the heavy metals concentration before and after adsorption, the efficiency of adsorption of heavy metals by eucalyptus carbon was calculated using the equation given below:
2.5.2. Adsorption isotherms
The adsorption isotherms of the studied heavy metals on the activated carbons used in this study were determined based on the batch experiments. In this study, Langmuir and Freundlich isotherm models were used to investigate the adsorption equilibrium between the heavy metal from solutions and an activated carbon phase. The Langmuir adsorption isotherm quantitatively describes for the formation of monolayer coverage of adsorbate onto the surface of adsorbent which also determines the maximum capacity of the adsorbent from complete monolayer coverage of the adsorbent surface. Langmuir’s isotherm linear equation is given as follows (Langmuir, Citation1918, Citation1916):
Where qe represents the amount adsorbed at equilibrium time (mg/g), qmax and b are Langmuir constants that depend on the maximum monolayer adsorption capacity and adsorption equilibrium constant that relates to the energy of adsorption and Ce represents the equilibrium concentration (mg/L), respectively.
Freundlich isotherm is often used for heterogeneous surface energy systems. It represents initial surface adsorption followed by a condensation effect resulting from strong adsorbent-adsorbate interaction. A linear form of the Freundlich equation is also given as follows (Freundlich, Citation1906):
Where KF is an intercept that roughly indicated the adsorption capacity and 1/n the slope is also known as the heterogeneous factor and ranges between 0 and 1 (Ayranci & Hoda, Citation2005); the more heterogeneous the surface, the closer 1/n is to 0 (Al Duri, Citation1995). Evaluation of the coefficient of KF and n can be accomplished using the linearized form of the above equation which is given as follows:
Thus, an isotherm study was performed by mixing adsorbent to the heavy metal spiked solutions at different dosages while keeping all other parameters constant. The sample was withdrawn for analysis at the equilibrium time chosen based on the experiments.
2.5.3. Column application
The column experiment was carried out using filter funnel columns with an internal diameter of 20 mm and a bottom with a pore size less than 0.25 mm not to lose any adsorbent material. The column was made of transparent glass and had a height of 210 mm. The column was filled with varied doses of 0.25, 0.5, 1 and 2 mm size ECAC as an adsorbent. In these experiments, a substantially lower concentration of heavy metals 0.0248–0.084 mg/L of each component was applied in order to approach realistic concentrations in wastewaters. Industrial effluent through the column and the amount of heavy metals from the effluents were determined.
2.5.4. Desorption
Desorption study was also carried out from the exhausted adsorbent after the column study. The sorbent which was removed from filtration was collected and washed with distilled water three times to remove residual Cr, Cd and Pb from the surface. To this 100 mL of (0.05, 0.1, 0.15 and 0.2) M of HCl solution was added. The mixtures were shaken for 120 min at 150 rpm using a horizontal shaker. The sorbent was removed by filtration. The concentrations of Cr, Cd and Pb in the aqueous solutions were determined by AAS method. Desorption tests were performed to evaluate the ability of the adsorbent to recover the metals which allows for the re-use of the metals.
3. Result and discussions
3.1. Characterization of E. camaldulensis activated carbon
The surface physical morphology of the adsorbent materials was studied by scanning electron microscopy (SEM). The SEM photograph of E. camaldulensis activated carbon (ECAC) is presented in Figure . The SEM micrograph of the ECAC showed the presence of a thick wall structure along with a well-developed wider porosity, which is promising for the ionic adsorption of the cationic form of metal ions. Thus, this very large surface area of the studied adsorbent, ECAC, is expressive of high adsorption capacity for heavy metal pollutants such as Cr, Cd and Pb.
3.2. Effect of particle size
The effect of particle size on adsorption of the heavy metals onto ECAC was studied by conducting different sets of experiments by varying the particle size of the adsorbent in increasing order from 0.063, 0.1, 0.25, 0.5, 1, 2 mm (Figure ). The finding showed that the percentage removal of the metals under investigation decreased from 70% to 24%, 60% to 13.6% and 97.2% to 20% for Cr, Cd and Pb, respectively. Thus, the experimental finding showed that the biosorption of these metal ions on ECAC was strongly dependent on the particle size of the adsorbent. This could probably be due to the fact that the smaller the particle size, the higher adsorption take place by increasing surface area during adsorbent-adsorbate interactions.
3.3. Effect of adsorbent dosage
Adsorption was carried out by varying the adsorbent dose to find out its effect on the removal of Cr, Cd and Pb using ECAC (Figure ). When the adsorbent dosage was increased from 0.1 to 4 g, keeping constant for adsorbate concentration at 0.25 mg/L and pH 10.0 for Cr and Pb, pH 12.0 for Cd, respectively, the percentage removal of metal ion increased. This is due to the greater availability of the exchangeable sites or surface area at a higher dose of the adsorbent. The removal of Pb, Cr and Cd attained maximum removal at a lower adsorbent dosage with 100 % removal at the adsorbent mass of 1 g. The removal percentage of heavy metals increased rapidly with increased in the adsorbent dosage was related to the increased in surface area and by extension the increased number of ion-exchangeable sites available for interaction with Cd, Cr and Pb ions. Therefore, this maximums dose was considered for further adsorption processes. The observed trend of percentage removal of metal ions was also recorded in the order of Cd > Cr > Pb.
3.4. Effect of contact time
The effect of contact time on the adsorption of Cr, Cd and Pb with an initial concentration of 0.25 mg/L for EACA adsorbents is shown in Figure . From this findings, it has been observed that most of Cr, Cd and Pb ions uptake takes place during 120 minutes of contact indicating the adsorption of Cr, Cd and Pb increased from 32 % to 69.36%, 45.36 % to 82.8% and10.68 % to 80%, respectively with an increased time from 10 to 120 minutes. This is due to the fact that the higher contact time between the sorbent surface and the metal solutions, the more complete adsorption by activating the equilibrium. Therefore, the optimum contact time for ECAC adsorbent was found to be 120 min. The very fast adsorption kinetics observed for the studied toxic metals showed an advantage for designing water treatment systems for chemical industries. Therefore, this maximums time was considered for further adsorption processes.
3.5. Effect of initial ph
The effect of pH on Cr (III), Cd (II) and Pb (II) adsorptions were studied in the pH range of 2.0–12.0, where chemical precipitation is expected to be avoided so that the metal removal could be related to that of the adsorption process, keeping initial Cr (III), Cd (II) and Pb (II) concentrations to be 0.25 mg/L and adsorbent dose = 1g in 100 mL of solution with a stirring speed of 180 rpm at room temperature for a contact period of 120 min. The effect of pH onto the studied adsorbent for Cr (III), Cd (II) and Pb (II) adsorption is shown in Figure . The obtained experimental results showed that the adsorption capacity increased as the solution pH increased. Accordingly, the observed trend of increase percentage adsorption of the metal ions were in the order of Cr > Pb > Cd. This higher adsorption at higher pH value for all metal ions could be due to the competing of H+ ions with that of the metal ions for adsorption sites which reduces their adsorption. Similar trends of adsorption of heavy metals at higher pH values were also observed by other researchers (Agbozu & Emoruwa, Citation2014). Keeping this in view, further adsorption studies were undertaken at pH 10 for Cr (III) and Pb (II), and pH 12 for Cd (II).
3.6. Effect of metal concentration
The effect of initial metal concentration on sorption of Cr, Cd and Pb was investigated with initial concentrations of 0.25, 0.5, 1, 1.5 and 2 mg/L. The adsorption efficiency increased with increasing initial metal ion concentration (Figure ). The removal percentage of total Cr, Cd and Pb generally increased with an increased in the initial concentration of the metal ions. Thus, the removal percentage of total metal ion increased from 80 to 95%, 55 to 100% and 95 to 100 % for Pb, Cr and Cd, respectively. This is because an increase in the initial metal ion concentration also increases the number of collisions between the metal ion and the bio-sorbent and hence increases the metal uptake. Thus, it could be concluded that the highest metal uptake would occur at the highest metal concentration, which is 2 mg/L, at optimal conditions. This result was similar to the work of (Okieimen & Onyenkpa, Citation1989). Increasing metal ion concentration increased the biosorption capacity for each bio-sorbent, which could be attributed to an increased rate of the mass transfer due to increased diffusivity of the driving force (Bansal, Singh, & Garg, Citation2009).
3.7. Adsorption isotherms
The analysis of equilibrium data for the adsorption of Cd, Cr and Pb on EACA was done using Langmuir and Freundlich isotherm model. The adsorption isotherm constants determined using Langmuir from the respective plots of Ce/qe Vs Ce for each metal and regression values (R2) are shown in Table . The extremely high value of R2 provided Langmuir isotherm model indicated that the adsorption data best fitted for the Langmuir isotherm given by the equation (Langmuir, Citation1918, Citation1916):
Table 1. Adsorption isotherms constants for Pb, Cr and Cd
The adsorption capacity of the adsorbent,
The feasibility of Langmuir isotherm was also expressed by a dimensionless constant separation factor or the equilibrium parameter RL which is defined as:
Indicating where RL> 1 unfavorable; RL = 1 linear, 0 < RL< 1 favorable and RL = 0 indicating for irreversible adsorption (McKay, Blair, & Gardner, Citation1982). Thus, the separation factor (RL) value also indicated the adsorption of Cr, Cd and Pb were in a favourable condition with that of the E. camaldulensis carbon adsorbent.
The adsorption isotherm constants determined using Freundlich from the respective plots of lnqe Vs lnCe for each metal and regression values (R2) are also shown in Table . The low value of R2 provided by Freundlich isotherm model indicated the adsorption data not fitted for the Freundlich isotherm given by the equation (Freundlich, Citation1906):
where KF is an intercept that roughly indicate the adsorption capacity and n the slope of the line that describes adsorption intensity, respectively (Okieimen & Onyenkpa, Citation1989). According to the KF values (see Table ), the adsorption capacities of the heavy metals studied was higher for Cd than Pb and Cr which could be associated with the physico-chemical properties of the metal. The slope (1/n) value for Cr and Pb in the studied adsorbent was <1, suggesting nonlinear adsorption isotherms. A slope >1 for Cd in the studied bio-sorbent indicates the saturation was not attained, which are characterized by an increase in the adsorption at a higher aqueous concentration of compounds and favourable adsorptions. Thus, the adsorption data (see Table ) indicate the adsorption for Pb, Cr and Cd metal ion removal fitted well with the Langmuir isotherm.
3.8. Comparison of sorption performance of E. camaldulensis with other selected bio-sorbents
The maximum capacity and removal efficiency of the E. camaldulensis activated carbon for the removal of the metal ions of Pb, Cd and Cr was also compared with other commercial activated carbon (CAC) studied by other scholars. The comparison was made from literature and using the data obtained from biosorption experiments conducted after all the operating parameters (i.e. bio-sorbent dose, contact time and pH) were optimized for 2 mg/L initial concentrations of Cd, Pb and Cr, respectively. Thus, it can be seen from Table that the adsorption capacity of the activated carbon from E. camaldulensis wood was comparable in removing the selected metal ions. Therefore, the activated carbon prepared from E. camldulensis can be used as an alternative for the removal of Cd, Cr and Pb from wastewater effluents of chemical industries such as MAA Garment and Textile Industry, Almeda Textile Industry and Sheba Leather Industry with low cost and high efficiency.
Table 2. Comparison of the maximum adsorption capacity of Eucalyptus activated carbon with different studied commercial activated carbon as adsorbents
3.9. The removal efficiency of E. camaldulensis on real samples
An efficient bio-sorbent not only adsorb the intended heavy metal ions from the synthetic solution but also removes heavy metals from the real wastewater samples collected from the industrial effluents. But it is known that effluents collected from industrial wastewater contain many metal ions, with each of them computing to the bio-sorbent in different ways and different capacity and thus compete for binding sites of the sorbent. Accordingly, the locally available bio-sorbent was tested for its effectiveness in removing the three heavy metals collected from different industrial wastewaters, i.e, after treatment Adwa textile industry (ATA), before treatment Adwa textile industry (BTA), MAA Garment after treatment (MAT), Wukro tannery after treatment (WAT) and Wukro tannery before treatment (WBT). Thus, the results of the preliminary experiments presented in Table indicated that higher sorption activity of Pb, Cr and Cd were shown in MAT, ATA and BTA. However, preliminary experiments performed for Cr using in WBT showed the removal efficiency of the studied bio-sorbent for chromium was lower due to the high presence of Cr from the wastewater of tannery and the presence of other ions. Hence, an increase in the amount of the bio-sorbent for tannery wastewaters is required to increase its efficiency for the removal of Cr from the tannery wastewater. In general, the presence of other ions also influences the biosorption of the studied heavy metals from the real wastewater samples. But, the experimental findings revealed that the sorption efficiency of the studied bio-sorbent (ECAC) was relatively good for the removal of the studied heavy metal ions from wastewater and can be used as an alternate and cost effective bio-sorbent for the removal of toxic pollutants from chemical industries.
Table 3. Results of preliminary biosorption experiments for total Pb, Cd and Cr (bio-sorbent dose = 1 g, pH (Pb and Cd = 10: Cr = 12), contact time = 2h) from real sample
3.10. Columns filtration
The removal of toxic heavy metals using column filtration is also presented in Table . The result showed that the column operation is more efficient at a low level of heavy metal concentrations for the fact that a column fully utilizes the carbon adsorption capacity due to a large concentration gradient existing opposite to the direction of flow in the column. As the flow rate increase, there is no enough time for adsorption equilibrium to be reached which results in low column utilization and the adsorbate solution leaves the column before equilibrium. Thus, the faster flow rate was attributed for the fast movement of the adsorption zone along the column, resulting in reducing the contact time between the effluent (Cr, Cd and Pb) and the adsorbent. From this, a flow rate of 5 mL/min was suggested longer residence time of effluent in the column which increases the chance of adsorption of heavy metals with the studied adsorbent filled in the column. A similar result has been also reported by other scholars using activated rice husk adsorbent to remove Cu (II) from solution (Yahaya, Abustan, Muhamed, Bello, & Ahmad, Citation2011).
Table 4. Column adsorption of Cr, Pb, and Cd at 11g, pH of 10, 2 h wastewater at 5 and10 ml/min of flow rate
3.11. Desorption
Desorption studies were also conducted using HCl solutions of various strengths ranging from 0.05 to 0.20 M. Maximum removal with 80 %, 81% and 75 % were shown for Cr, Cd and Pb, respectively indicating that desorption was possible from the studied bio-sorbent (Figure ). During desorption study, results showed that with an increase in the concentration of HCl the desorption also increased and thus 0.2 M of HCl solution was obtained enough for desorption of more than 80% the adsorbed Cr, Cd and Pb. From this, it may be concluded that in the acid medium protons compete for the sorption sites with the metal ions and displace the maximum amount of adsorbed Pb, Cr, and Cd. Hence, it can be stated that an ion-exchange mechanism is important in connection with the sorption process for the studied bio-sorbent.
4. Conclusions
This study was carried out to evaluate the efficiency of ECAC on the removal of metal ions from synthetic solution and industrial effluents. The biosorption was found to be strongly dependent on the size of the particle, pH, adsorbent dose, contact time and initial concentration of the metal ions. Generally, the result showed that activated carbon which was traditionally prepared from eucalyptus wood has a high capacity for the removal of metal ions from wastewater and the adsorption isotherm was well fitted to that of Langmuir isotherm models. The obtained result suggests monolayer coverage of the surface of adsorbent by Cr, Cd and Pb ions as the Langmuir isotherm assumes that the surface is homogeneous. The experimental findings also indicated that the column test was incomparable with the batch mode removal of the heavy metals and desorption tests using HCl was comparable with other findings. Thus, the present finding revealed the efficiency of ECAC for the removal of toxic heavy metal pollutants of Cr, Cd and Pb with the percentage removal of 82 to 100. The SEM image also supported the potential of the studied activated carbon for the removal of toxic heavy metals. Therefore, E. camaldulensis is cheap material with its high adsorptive capacity and thus it would be convenient to use it in industrial wastewater treatment plants.
Acknowledgements
This study was conducted at Mekelle University, Ethiopia and thus all resources used are acknowledged. The authors also thank Mr Alem Halefom, Chemistry Department, Mekelle University for his kind help in sample preparation; Prof. Bart Van Der Bruggen for adsorbent SEM analysis, KU Leuven, Belgium and Ezana Analytical Laboratory, Ezana Mining Development PLC, Mekelle, Tigray, Ethiopia, for heavy metal analysis.
Additional information
Funding
Notes on contributors

Abraha Gebrekidan
G. Hirut holds her MSc degree in analytical chemistry from Mekelle University. Her MSc thesis supervised by Asgedom and Demlie, focused on the removal of Cr, Cd and Pb from wastewater using Eucalyptus camaldulensis activated carbon. Thus, the results of her findings are presented in this paper.
Libargachew Demlie
A. G. Asgedom (PhD) is working as analytical and environmental chemist at the Department of Chemistry, Mekelle University, Ethiopia. He has more than 28 articles published in reputable local and international journals. He is mainly engaged in both basic and applied researches which are all mainly focused on environmental issues.
References
- Agbozu, I. E., & Emoruwa, F. O. (2014). Batch adsorption of heavy metals (Cu, Pb, Fe, Cr and Cd) from aqueous solutions using coconut husk. African Journal of Environmental Sciences and Technology, 8(4), 239–16.
- Ahmed, R., Yamin, T., Ansari, M. S., & Hasany, S. M. (2006). Sorption behavior of lead (II) ions from aqueous solution onto Haro river sand. Adsorption Science Technology, 24(6), 475–486. doi:10.1260/026361706780154400
- Al Duri, B. (1995). Adsorption modeling and mass transfer, use of adsorbents for the removal of pollutants from wastewaters. Boca Raton, FL: CRC Press.
- Aloko, D. F., & Afolabi, E. A. (2007). Titanium dioxide as a cathode material in a dry cell. Leonardo Electronic Journal of Practices and Technology, 11, 97–108.
- Amin, N. M., Kaneco, S., Kitagawa, T., Begum, A., Katsumata, H., Suzuki, T., & Ohta, K. (2006). Removal of arsenic in aqueous solutions by adsorption onto waste rice husk. Indian Journal of Environmental Protection, 45(24), 8105–8110.
- Ayranci, E., & Hoda, N. (2005). Adsorption Kinetics and Isotherms of Pesticides onto Activated Carbon-Cloth. Journal of Chemosphere, 60(11), 1600–1607. doi:10.1016/j.chemosphere.2005.02.040
- Bansal, M., Singh, D., & Garg, V. K. (2009). A comparative study for the removal of hexavalent chromium from aqueous solution by agriculture wastes’ carbons. Journal of Hazardous. Materials, 171(1–3), 83–92. doi:10.1016/j.jhazmat.2009.05.124
- Barkat, M., Nibou, D., Chegrouche, S., & Mellah, A. (2009). Kinetics and thermodynamics studies of chromium (VI) ions adsorption onto activated carbon from aqueous solutions. Chemical Engineering and Processing, 48(1), 38–47. doi:10.1016/j.cep.2007.10.004
- Çalımlı, M. H., Demirbaş, Ö., Aygün, A., Alma, M. H., Nas, M. S., Khan, A., … Şen, F. (2019). Equilibrium, kinetics and thermodynamics of bovine serum albumin from carbon-based materials obtained from food wastes. BioNanoScience, 9(3), 692–701. doi:10.1007/s12668-019-00633-z
- Demirbaş, Ö., Çalımlı, M., Demirkan, B., Alma, M., Salih Nas, M., Khan, A., … Şen, F. (2019a). The kinetic parameters of adsorption of enzymes using carbon-based materials obtained from different food wastes. BioNanoScience, 9(3), 749–757. doi:10.1007/s12668-019-00635-x
- Demirbaş, Ö., Çalımlı, M. H., Demirkan, B., Alma, M. H., Nas, M. S., Khan, A., … Şen, F. (2019b). Thermodynamics, kinetics, and adsorption properties of biomolecules onto carbon-based materials obtained from food wastes. BioNanoScience, 9, 672–682. doi:10.1007/s12668-019-00628-w
- El Nemr, A., Khaled, A., Abdelwahab, O., & El-Sikaily, A. (2008). Treatment of wastewater containing toxic chromium using new activated carbon developed from date palm seed. Journal of Hazardous Material, 152(1), 263–275. doi:10.1016/j.jhazmat.2007.06.091
- Eltayeb, N., & Khan, A. (2019). Design and preparation of a new and novel nanocomposite with CNTs and its sensor applications. Journal of Materials Research and Technology, 8(2), 2238–2246. doi:10.1016/j.jmrt.2019.03.002
- Freundlich, H. (1906). Over the adsorption in solution. Journal of Physical Chemistry, 57, 385–471.
- Fu, F., & Wang, Q. (2011). Removal of heavy metal ions from wastewaters: A review. Journal of Environmental Management, 92(3), 407–418. doi:10.1016/j.jenvman.2010.11.011
- Galal-Gorchev, H., Ozolins, G., & Bonnefoy, X. (1993). Revision of the WHO guidelines for drinking water quality. Annali dell’Istituto Superiore Di Sanità, 29(2), 335–345. ISSN: 00212571.
- Ghodbane, I., & Hamdaoui, O. (2008). Removal of mercury (II) from aqueous media using eucalyptus bark: Kinetic and equilibrium studies. Journal of Hazardous Materials, 160(2–3), 309–310. doi:10.1016/j.jhazmat.2008.02.116
- Isloor, A. M., Chandrashekhar Nayak, M., Inamuddin, Prabhu, B., Ismail, N., Ismail, A. F., & Asiri, A. M. (2019). Novel polyphenylsulfone (PPSU)/nano tin oxide (SnO2) mixed matrix ultrafiltration hollow fiber membranes: Fabrication, characterization and toxic dyes removal from aqueous solutions. Reactive and Functional Polymers, 139, 170–180. doi:10.1016/j.reactfunctpolym.2019.02.015
- Khan, A., Asiri, A., Khan, A., Abdul Rub, M., Azum, N., Rahman, M., … Ab Ghani, S. (2013). Sol-gel synthesis and characterization of conducting polythiophene/tin phosphate nano tetrapod composite cation-exchanger and its application as Hg(II) selective membrane electrode. Journal of Sol-Gel Science and Technology, 65(2), 160–169. doi:10.1007/s10971-012-2920-6
- Khan, A., Asiri, A., ParwazKhan, A., AbdulRub, M., Azum, N., Rahman, M., … Qusti, A. (2014). Dual nature, self oxidized poly(o-anisidine) functionalized multiwall carbon nanotubes composite: Preparation, thermal and electrical studies. Composites Part B: Engineering, 58, 451–456. doi:10.1016/j.compositesb.2013.10.059
- Khan, A., Khan, A., Rahman, M., & Asiri, A. (2016). High-performance polyaniline/vanadyl phosphate (PANI–VOPO4) nanocomposite sheets prepared by the exfoliation/intercalation method for sensing applications. European Polymer Journal, 75, 388–398. doi:10.1016/j.eurpolymj.2016.01.003
- Khan, A., Parwaz Khan, A., Khan, I., Oves, M., Khan, S., Asiri, A., … Facchetti, A. (2019). Facial synthesis of highly active polymer vanadium molybdate nanocomposite: Improved thermoelectric and antimicrobial studies. Journal of Physics and Chemistry of Solids, 13, 148–155. doi:10.1016/j.jpcs.2019.03.022
- Khan, A. A., & Khan, A. (2010). Ion-exchange studies on poly-o-anisidine Sn(IV) phosphate nanocomposite and its application as Cd(II) ion-selective membrane electrode. Central European Journal of Chemistry, 8, 396–408.
- Khan Ali, A., Habiba, U., & Khan, A. (2009). Synthesis, characterization and electrical conductivity measurement studies of poly-o-anisidine Sn(IV) phosphate [POASn(IV)P] nano-composite cation-exchange material. Materials Science and Engineering: B, 158(1–3), 92–97. doi:10.1016/j.mseb.2009.01.024
- Khan, M., Khan, A., Asiri, A., & Sirajuddin, V. (2018). Transport and surface charge density of univalent ion of polyvinyl chloride-based barium tungstate ion-exchange composite membrane for industrial separation of wastewater. Journal of Industrial Textiles, 49(5), 584–596. doi:10.1177/1528083718791344
- Kolangare, I. M., Isloor, A. M., Asiri, A. M., & Ismail, A. F. (2019). Improved desalination by polyamide membranes containing hydrophilic glutamine and glycine. Environmental Chemistry Letter, 17, 1053–1059. doi:10.1007/s10311-018-00825-1
- Kumar, M., RaoT, S., Isloor, A. M., Ibrahim, G. P. S., Inamuddin, Ismail, N., … Asiri, A. M. (2019). Use of cellulose acetate/polyphenylsulfone derivatives to fabricate ultrafiltration hollow fiber membranes for the removal of arsenic from drinking water. International Journal of Biological Macromolecules, 129, 715–727. doi:10.1016/j.ijbiomac.2019.02.017
- Langmuir, I. (1916). The constitution and fundamental properties of solids and liquids. Journal of American Chemical Society, 38(11), 2221–2295. doi:10.1021/ja02268a002
- Langmuir, I. (1918). the adsorption of gases on plane surfaces of glass, mica and platinum. Journal of American Chemical Society, 40(9), 1361–1403. doi:10.1021/ja02242a004
- Malkoc, E., Nuhoglu, Y., & Abali, Y. (2006). Cr (VI) adsorption by waste acorn of Quercus Ithaburensis in fixed beds: Prediction of breakthrough curves. Chemical Engineering Journal, 119(1), 61–68. doi:10.1016/j.cej.2006.01.019
- McKay, G., Blair, H. S., & Gardner, J. R. (1982). Adsorption of dyes on chitin I Equilibrium studies. Journal of Applied Polymer Science, 27(8), 3043–3057. doi:10.1002/app.1982.070270827
- Metcalf and Eddy. (2003). Wastewater engineering: Treatment and reuse. New York, NY: McGraw-Hill.
- Mohanah, D., Pittmanjr, C. U., Bricka, M., Smith, F., Yancey, B., Mohammad, J., Steele, P. H., … Gong, H. (2007). Sorption of arsenic, cadmium, and lead by chars produced from fast pyrolysis of wood and bark during bio-oil production. Journal of Colloid Interface Sciences, 310(1), 57–73. doi:10.1016/j.jcis.2007.01.020
- Okieimen, F. E., & Onyenkpa, V. U. (1989). Removal of heavy metal ions from aqueous solutions with melon (Citrullus vulgaris) seed husks. Biological Wastes, 29(1), 11–16. doi:10.1016/0269-7483(89)90099-2
- Rangreez, T., Inamuddin, Mu, N., & Ali, H. (2015). Synthesis and characterisation of poly (3,4-ethylenedioxythiophene)-poly(styrenesulfonate) (PEDOT: PSS) Zr(IV) monothiophosphate composite cation exchanger: Analytical application in the selective separation of lead metal ions. International Journal of Environmental Analytical Chemistry, 95(6), 556–568. doi:10.1080/03067319.2015.1036863
- Rawat, N. S., Ranjana, P. K., & Singh, D. (1993). Characteristic adsorption of aqueous lead (II) on bituminous coal. Indian Journal of Environmental Protection, 13(3), 193–197.
- Tchobanoglous, G., Burton, F. L., & Stensel, H. D. (2003). Wastewater engineering: Treatment and reuse. New York, NY: Metcalf and Eddy Inc., McGraw-Hill.
- Yahaya, N. K. E. M., Abustan, I., Muhamed, P. M., Bello, O. S., & Ahmad, M. A. (2011). Fixed-bed column study for Cu (II) removal from aqueous solutions using rice husk based activated carbon. International Journal of Engineering & Technology, 11(1), 248–252.