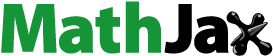
Abstract
Recently, the extrusion process has been applied to the production of snacks, cereals, and pasta due to the advantages it offers but processing temperature is a critical factor that affects the retention of nutrients in biofortified crops. This study examined the effect of extrusion cooking on the proximate, antinutritional, and carotenoid properties of biofortified maize and soybean flour blends. Samples were prepared by blending maize and soybeans flours in varied proportions (100:0, 90:10, 85:10, 80:20, 70:30) and were extruded at a feed rate of 1.5 kg/h with different temperatures and screw speeds. The extrudates were subjected to proximate, antinutritional, and carotenoid analyses using standard laboratory methods. The moisture content, crude protein, crude fibre, crude fat, and ash contents of the extrudates ranged from 8.89 to 12.91%, 8.21 to 20.61%, 2.08 to 4.64%, 3.81 to 5.90% and 1.62 to 2.37%, respectively. The comparative percentage composition of carotenoids of the flour blends indicated that lutein, zeaxanthin, β- cryptoxanthin, α-carotene, 13-cis β-carotene, 9-cis β-carotene, total β-carotene, total xanthophylls, provitamin A were higher when extruded at lower processing speed and temperature. The antinutrient composition shows a significant reduction in the levels of oxalate, tannins and phytate compared with previous related work. Sample ABM (90:10 biofortified Maize and soybean flours) showed high contents of carotenoid properties and low antinutritional properties and made it better than other samples. The extruded samples are nutritious, and further processing (addition of ingredients) will help derive a new product with increased nutritional quality.
PUBLIC INTEREST STATEMENT
The extrusion cooking is a process in which processing of food material is achieved under the combined effect of temperature, pressure and shear. The extrusion cooking method is preferable to other food processing techniques because of its high productivity and better nutrient retention. This study examined the effect of extrusion cooking on the proximate, antinutritional, and carotenoid properties of biofortified maize and soybean flour blends. The results of this research showed that the extrusion cooking technique could produce products with good nutrients retention from a mix of orange maize and soybean flours that could be used for further product developments. The crop-to crop fortification could be a vehicle to curb the deficiency of essential nutrients and improve diet in developing countries.
Competing Interests
The authors declares no competing interests.
1. Introduction
Over the years, food scientist and other researchers have consistently sought for a more efficient and effective way of processing food with little or no loss to the nutritional composition of the food. One of such food processing method in recent times is extrusion cooking. According to Castells et al. (Citation2005), extrusion cooking is a process in which processing of feed material is achieved under the combined effect of temperature, pressure and shear. It is preferable to other food processing techniques in terms of the continuous process with high productivity and significant nutrient retention, due to the short processing and temperature required. Still, the effects of these factors have not been adequately established for the nutrients in biofortified crops, most especially maize. Maize, Zea mays L (corn) is one of the most widely cultivated cereals in the world. There are several types of genotypes with varying colours. Biofortified maize has received increased attention from a nutraceutical perspective because it contains several bioactive photochemical such as carotenoids, tocopherols, phytic acid and phenolic compounds (Hu & Xu, Citation2011; Zilić et al., Citation2012). Although most of these compounds are considered to have little or no nutrition values, their antioxidant and bioactive properties have these compounds to have substantial potential health benefits. In fact, it has been proved that several plant phytochemicals are bioavailable, they are absorbed from the intestine and exert several physiological roles as demonstrated in animal models and human studies. Besides their high antioxidant and antiradical activities, the beneficial health properties of these plant metabolites will also be attributed to many other mechanisms such as anti-inflammatory properties, inhibition of enzymes, and induction of detoxification enzymes (Ferretti et al., Citation2010; Wang & Stoner, Citation2008).
Carotenoids are vital for human nutrition and health (Zhou et al., Citation2011). The increased interest in dietary carotenoids could be due to the association between carotenoid deficiencies and many chronic human diseases. The breeding to increase β-carotene levels (biofortification) in cereal grains has been identified to be the best economical approach to address dietary vitamin A deficiency in the developing world (Owens et al., Citation2014).
Aside from the abundant amount of carbohydrate present in biofortified maize, it is also considered to be a source of carotenoids. These substances are essential for human health, given the capability as an antioxidant and the activity as vitamin A precursor (Rodriguez-Amaya, Citation2001). It was reported that the main carotenoids found in the chromoplasts of orange maize endosperm are xanthophylls (lutein, zeaxanthin and β-cryptoxanthin) and carotenes (β-carotene, α-carotene, and β-zeacarotene). The lutein and zeaxanthin were dominant carotenoid species in milled maize fractions, accounting for 70% of total carotenoid content (Kean et al., Citation2008).
An important legume selected for this study is Soybean (Glycine max (L.) Merr.). Soy proteins have been widely used as a functional ingredient in many processed foods because of their ability to form gels with high nutritional, sensory and physiological qualities (Mesa et al., Citation2008). The use of plant proteins, such as soy protein, instead of animal proteins is an economical and more viable intervention strategy to reduce the risk of coronary heart disease (Girgih et al., Citation2013).
Food extrusion is a high temperature-short time process as barrel temperatures are adjusted to not to exceed 180°C, and residence time in extruder usually changes in the range of 20–200 seconds (Martínez-Bustos et al., Citation2012). This time-temperature combination is ample to the physical changes or chemical reactions like starch gelatinization, protein denaturation, enzyme inactivation and destruction of microorganisms(Wolf, Citation2010). In contrast, the formation of flavors or color change reactions may not take place mainly as a result of limited time (Chessari & Sellahewa, Citation2001; Martínez-Bustos et al., Citation2012).
In recent years, the extrusion process draws interest in the food industry, especially in the production of snacks, cereals and pasta due to the advantages it offers. While short residence time provides the quality of extrudates; opportunity to form variously shaped products creates appetizing foods. Besides; too little waste produced during extrusion makes it possible to overcome the environmental or government restrictions and lowers the cost of the final product (Riaz & Rokey, Citation2011). It has been reported that food- to- food fortification of cereals and legumes has been reported to be an effective strategy for alleviating malnutrition in developing countries (Alamu et al., Citation2018; Lartey et al., Citation1999). In Nigeria, some biofortified maize and improved soybean varieties were recently released, most notably the improved maize variety SAMMAZ 39 (with yellow -orange grain colour) and improved soybean TGx 1989–19 F. These varieties need to be evaluated for end-use and product development, and there is scanty information on the effects of the extrusion process on the nutrients and bioactive compounds of composite flours of biofortified maize and soybean varieties that have recently released in Nigeria. This study, therefore, aimed at evaluating the effect of extrusion cooking on the proximate, antinutritional, and carotenoid properties of the blend of biofortified maize and soybean flours.
2. Materials and methods
2.1. Sources of materials
The biofortified Maize (SAMMAZ 39) and soybean (TGx 1989–19 F) were purchased from the Farm office of International Institute of Tropical Agriculture (IITA) Ibadan, Nigeria. It was packed in a dark paper bag and kept away from light to prevent the loss of carotenoids.
2.2. Sample preparation
2.2.1. Preparation of maize flour
The maize was cleaned from extraneous particles, and 3.55 kg of biofortified maize was weighed using a Fuji FTS- 15 kg Mechanical Table scale. The maize was dry milled using a Tigmax grinding machine (GX160) with less penetration of sunlight. The maize flour was packaged in a Ziploc bag and stored in a cold and darkroom for further processing and analysis (Edema et al., Citation2005).
2.2.2. Preparation of soybeans flour
Soybean seeds were cleaned from extraneous particles, and 1 kg of soybean seed was weighed using a Fuji FTS- 15 kg Mechanical Table scale. The grinding machine was properly clean to avoid errors or cross-contamination. The maize was dry milled using a Tigmax grinding machine (GX160) and packaged in a Ziploc bag. It was stored at room temperature (Ajibola & Filani, Citation2015).
2.2.3. Preparation of composite flours
Samples of different composite (Table ) were prepared and weighed using an electronic balance/scale with 500 g calibration weight. Each bag of flour was weighed separately and adequately mixed in a clean plastic bowl before packaged in a Ziploc bag.
Table 1. Formulation of flour and composite
2.3. The ratio of mixing flours
The formulation below was used to produce the samples and were coded as LMN, ABM, CBM, FBM and ZBM as % of soybean flour in the mixture increases from 0, 10,15,20 and 30% respectively (Table ).
2.3.1. Extrusion cooking process
A total of 150 ml of distilled water was added to 200 g of each blended flour sample and mixed properly. The blended samples were extruded using a single screw extruder from Cosmic Controls India Pvt Ltd, powered by Motor Generator (1 HP DC Motor Model). The material was fed at a rate of 1.5 kg/h during extrusion of all the samples. Each sample was run at a temperature and screw speed of 90°C and 100 rpm; and 100°C and 120rpm, respectively. Extruded samples were collected at steady state extrusion conditions. All the samples produced a flat thread-like chip after extrusion cooking. The collected samples were held on a tray at ambient conditions (29 ± 1°C) for five (Ferretti et al., Citation2010) minutes. The extrudates were dried in the oven at a low temperature of 60° C for 12 hours. All extrudates were milled immediately to 0.5 mm sieve size using laboratory milling machine as described by Alamu et al. (Citation2015). The products were sealed in a dark ziploc bag and stored in the freezer before analysis. However, all analyses were done within 48 hours after processing to avoid degradation of carotenoids.
2.4. Determination of the proximate composition of the extrudates
The protein, ash, moisture content, crude fat, crude fiber and carbohydrate contents of the extrudates were determined using AOAC (Citation2006) methods. The total carbohydrate was calculated by difference.
2.5. Determination of tannin and oxalates
Tannin and oxalate were analyzed spectrophotometrically at 440–500 nm, as described by Leyva et al. (Citation1990) and Doss et al. (Citation2011). Tannins were extracted by shaking 1 g of the sample in 10 ml acidified methanol in centrifuge tubes at 25 °C for 20 minutes. The mixture was centrifuged at 10,000 rpm for 15 minutes before pipetting 1 ml into a test tube. The vanillin-hydrochloric acid reagent (5 ml) was added to the aliquot, and absorbance read in 1 cm cuvettes using an ultrospec 1000 spectrophotometer at 500 nm after 20 minutes against vanillin-hydrochloric acid reagent as blank. A standard curve was prepared by adding 1 g of tannic acid to 100 ml acidified methanol (Makkar et al., Citation1993).
2.6. Determination of phytates
Phytate contents were determined as described by Mbaeyi and Onweluzo (Citation2010). Phytates were extracted by adding 0.1 g sample to 100 ml of 0.2 mol/L hydrochloric acid and shaken for 1 hour before centrifuging at 5000 rpm for 15 minutes. The supernatant (0.5 ml) was pipette into a test tube fitted with a ground glass stopper before adding 1 ml acidic ammonium iron (iii) sulfate dodecahydrate (0.2 g NH4 Fe (SO4)2. 12H2O in 100 ml 2 mol/l hydrochloric acid and made up to 1000 ml with distilled water). Absorbance was read after 1 minute using ultrospec 1000 spectrophotometer at 519 nm against distilled water. A standard curve was prepared by adding 125 mg sodium phytate to 100 ml 0.2 mol/l hydrochloric acid (Makkar et al., Citation1993).
2.7. Carotenoids determination of the extrudate
The carotenoid analysis was carried out using the method described by Alamu et al. (2015). 0.6 g of the ground extrudate was weighed in a screw cap tube. 6 ml of ethanol containing 0.1% butylhydroxytoluene (BHT) was added, followed by addition of 0.5 ml of 80% KOH. It was vortex for 30 seconds and stood for 5 minutes in a water bath at 85°C. The tube was removed from the water bath and was put into ice. 3 ml of cold H20 and 3 ml of Hexane was added. It was centrifuged for 10 seconds at 1000 rpm. The upper phase was pipette into a 50 ml concentrator tube. The extract was concentrated in the evaporator at 40°C using N2 gas for 25 minutes (Kimura et al., Citation2007). Immediately before injection, the sample was dissolved in 1 ml of 50:50 DCE: MeOH and vortex for 10secs. It was transferred into HPLC vial and ran for major carotenoids using the HPLC machine. The chromatogram was extracted, major carotenoid was identified, and the value of each carotenoid was calculated (Kimura et al., Citation2007).
2.8. Calculation of carotenoids
As × sample weight (g)
Where Cx = concentration of carotenoid X;
Ax = peak area of carotenoid X;
Cs = concentration of the standard;
As = peak area of the standard.
2.9. Data analysis
The data generated from this experiment were analyzed using IBM SPSS statistical software. The analysis of variance (ANOVA) was carried out, and the means were separated using Duncan’s multiple range test, compared by the least significant difference (LSD) with a mean square error of 5% probability.
3. Results and discussion
3.1. Proximate composition of the extrudates
Figures and presented the proximate composition of the extruded samples at High temperature and speed; 100°C and 120 rpm and Low temperature and speed: 90 oC and 100rpm respectively. The moisture content (MC) of the extrudate from biofortified maize (BMF) and soybean (SF) flours ranged from 8.89 to 12.91%. Sample ZBM (70% BMF, and 30% SB at High temperature of 120 °C) has the least MC when compared with others. Sample LMN of 100% BMF (Low temperature of 90 °C and speed 100rpm) had the highest MC. This shows that sample from 100% biofortified maize extruded at low temperature with low speed had the highest moisture. The moisture content of a sample reflects the amount of solid matter in the sample. The higher the moisture content, the higher the rate of spoilage.
Adebowale et al. (Citation2005) stated that moisture content is a measure of the water content and an indicator of shelf stability. An increase in moisture content can enhance microbial growth, which leads to deterioration in foods. Food and Agriculture Organization of the United Nations [FAO] (Citation2009) recommended a safe level of 12 to 14% moisture content for flour or powdered food. The MC of all the extrudate was below the FAO recommended limit, and thus, the samples could be stored for an extended period without being showing the microbial growth. However, the MC of the samples was higher than the range reported by Edema et al. (Citation2005) for maize-soybean flour, although their samples were not extruded. The higher moisture content observed for extruded samples in this study may be due to the initial MC of the biofortified maize and soybean varieties used and the volume of water added before extrusion. Also, it can be observed that the moisture content increases as the biofortified maize flour increases. However, the moisture contents were significantly (P < 0.05) different from each other. Extrusion cooking at a high temperature helps to reduce the moisture content of the extrudates, hence ensuring a longer shelf life than the extrusion at the lower temperature. This was further justified by exposing both samples to open air for a few days. The preliminary result showed that the mould growth appeared first on extrudate samples with low temperature and low speed.
The protein content values for the extruded samples ranged from 8.21 to 20.61% (Figures and ). The variations in the blend ratio revealed that maximum protein (20.61%) was obtained at the blend ratio of BMF 70% to SB 30% (Low temperature), i.e. ZBM (low). In comparison, the least protein content was obtained for sample LMN (100% BMF, High temperature). Protein is essential in the human diet for growth. The protein content of the extruded samples was observed to increase with a progressive increase in the soybean flour, indicating that incorporation of biofortified maize with soybean flour into extrusion would significantly improve the protein content of the extruded sample. This agreed with Obatolu (Citation2002) and Iwe (Citation2003) that there were marginal increases in protein content of extrudates from malted or unmalted millet/soybean mixture. The extrudates protein contents produced under high temperature were higher than low-temperature extrudates. This could be due to the dilution effect because of the higher MC observed for the extrudates from the low temperature and low-speed extrusion process. The values of protein content obtained in this study for all the extrudates were lower to the values reported by Annonye et al. (Citation2007), but their extrudates were from Acha and soybean flours.
The crude fiber contents of the extruded samples ranged from 2.08 (ZBM) to 4.64% (LMN). The crude fiber content decreases as the % of soybean increases, and thus, ZBM (70:30 BMF: SF) had the lowest crude fibre content. Crude fiber is the insoluble polymeric material of plant cell walls such as cellulose, hemicelluloses, pectin and lignin that constitute the major part of dietary fiber (Johnston & Oliver, Citation1982). High fiber intake has been linked with decreased chances of colon cancer and associated with reducing constipation. The fiber content of the extruded sample was higher than maize-soybean flour reported by Edema et al. (Citation2005). Fiber is said to have a beneficial effect on preventing cancer. It was observed that increasing soybean flour decreases fiber content while increasing biofortified maize flour increases fiber content. It was seen that there is only a slight difference in fiber content between the extrudates from the high temperature with high speed and that of low temperature with low speed. It implies that extrusion cooking at the conditions used in this study did not affect the crude fiber content.
From Figures and , fat content ranged from 3.89 (LMN) to 5.22% (ZBM) for biofortified maize (SAMMAZ 39) and soybean extrudate produced under high temperature and 3.81 (LMN) to 5.90 % (ZBM) under low temperature. The results showed that the extruded sample of biofortified maize (SAMMAZ 39) and soybean flour resulted in the increased fat content of the blend relative to the raw biofortified maize (SAMMAZ 39). The more soybean flour added to the sample, the increase in the fat content of the extrudates sample. Biofortified maize (SAMMAZ 39) and soybean flour had higher values of fat content produced under high and low temperatures, which indicated that blending improved the proximate composition of the extrudates. There was a significant difference between the extruded sample of biofortified maize (SAMMAZ 39) and soybean flour produced under high and low temperatures.
Ash content reflects mineral status, even though contamination can indicate a high concentration in a sample. The ash content ranged from 1.64 (LMN) to 2.37 (ZBM) % for high-temperature extrusion cooking and 1.62 to 2.21% for LMN and ZBM respectively for low-temperature extrusion cooking. There was an increase in the amount of ash content. The high ash content of the extruded sample is indicative of being a good source of minerals. It was observed that increasing soybean flour increases ash content. However, sample LMN and ABM (both High and low temperature) recorded no significant differences between them.
Figure 1. Proximate Composition of Extruded Samples (High Temperature and Speed; 100 °C and 120 rpm)
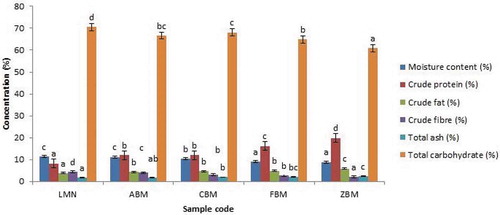
Figure 2. Proximate Composition of Extruded Samples (Low Temperature and Speed; 90 °C and 100 rpm)
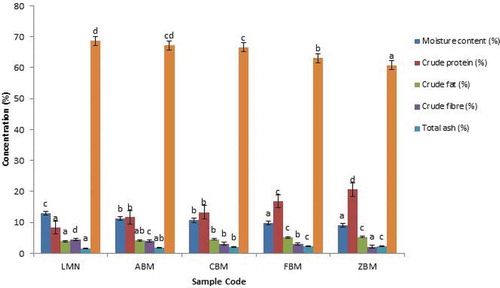
3.2. Antinutritional factors of the extruded sample of biofortified maize and soybean flour
Figures and show the antinutritional factors of the extruded samples of biofortified maize and soybean flours. The tannin composition varied from 2.05 mg/g (ABM) to 2.76 mg/g (LMN) in high temperature extrudates and 2.37 mg/g (ABM) to 2.89 mg/g (ZBM) (low temperature). Sample ABM had the lowest value (2.05 mg/g) in both high and low temperatures while sample (ZBM) had the highest value in low and second-highest value in high-temperature extrudates. There was no significant difference in the tannin content of the extruded samples for both low and high temperatures extrudates. However, when compared the two sets of the extrudates, the set from higher temperature showed a bit lower values but not significant (p > 0.05). It means that extrusion process conditions did not significantly influence the tannin content of the extrudates studied. However, the tannin content increases as the percentage of soybean flour increases and that were why high tannin content was observed for sample ZBM irrespective of the temperature of the extrusion. The tannin-protein complexes could cause inactivation of digestive enzymes and reduce protein digestibility by the interaction of protein substrate with ionizable iron. It has been established that tannins in food could decrease the iron absorption, damage the mucosal lining of the gastrointestinal tract, alter excretion of cations, and increase excretion of proteins and essential amino acids (Tchinmegni & Djeukam Citation2016).
The phytate composition ranged from 0.83 mg/g (ABM) to 0.98 mg/g (ZBM) in high temperature extrudates and 0.75 mg/g (ABM) to 0.83 mg/g (ZBM) (low temperature). The phytate content increases as the soybean flour ratio increases, and there was no significant difference between the extrudates of high temperature and that of low temperature. However, sample ABM showed the least phytate values in both high and low extrusion cooking temperatures, while ZBM had the highest values for both temperatures. Phytic acid in foods binds trace elements and macro-elements such as zinc, calcium, magnesium, and iron in the gastrointestinal tract and making dietary minerals unavailable for absorption and utilization by the body (Melaku et al., Citation2005; Sandberg, Citation2002). It can also form complexes with proteins, proteases, and amylases of the intestinal tract, thus inhibiting proteolysis. Phytic acid is the main phosphorus store in mature seeds. It also renders several minerals biologically unavailable to animals and humans. Phytate occurs as a mineral complex, which is insoluble at the physiological pH of the intestine. It is considered antinutritional, causing reduced uptake in the human intestine of essential dietary minerals such as Fe and Zn. According to Weaver and Kannan (Weaver & Kannan, Citation2002), zinc forms the most stable complex with phytate. Phytic acid is better reduced by soaking. However, extrusion processing could not eliminate the antinutritional components evaluated; a considerable reduction can be observed depending upon the extruded samples and temperature of the extrusion process.
The oxalate composition ranged from 10.89 mg/g (ABM) to 15.96 mg/g (ZBM) in high temperature extrudates and 12.09 mg/g (LMN) to 15.63 mg/g (ZBM) in low temperature respectively. Sample ABM maintains the least value in high extrusion cooking and second-highest value in low extrusion cooking, while ZBM has the highest values for both high and low extrusion cooking. The levels of the tannin and phytate present in the extrudate samples are within the safe limit according to FAO/WHO, Codex Alimentarius Commission, and Food Standards. They can be further eliminated by processing such as cooking and boiling. The oxalate content significantly (P < 0.05) changed in some extrudates (Figures and ). From the results, it was observed that oxalate content increases with an increase in soybean supplementation.
According to Tiwari and Jha (Citation2017), extrusion cooking also improves the nutritional quality of foods by destroying many natural toxins and antinutrients. A dilemma exists as to whether it is desirable to remove these compounds because of their bioactive activity. Enzyme inhibitors, hormone-like compounds, saponins, oxalates, and other compounds could impair growth and development in children, but these same compounds may offer protection against chronic diseases in adulthood.
In this study, the values of tannin and phytic contents at high-temperature extrusion cooking were marginally lower than that of the low-temperature extrusion process. This may be as a result of the reduction in the antinutritional properties of the extrudates with an increase in temperature. However, this does not apply to the oxalate content from the high and low-temperature extrusion process in this study. The high values of oxalate observed for all of the extrudates agreed with what Weiwen and Michael (Citation2004) reported. They indicated that the oxalate contents (mg/100 g wet weight) of soy and cornmeal are 187 and 55, respectively, before further cooking or processing. The higher value of oxalate in soybean flour explains the increase in the oxalate content with an increase in soybean flour. However, sample ABM (90:10, Maize and soybean flours) had the lowest antinutritional factors at both low temperature with low speed and high temperature with high speed.
Figure 3. Antinutritional Factors of Extruded Sample of Biofortified Maize and Soybean Flour (High Temperature and Speed; 100 °C and 120 rpm)
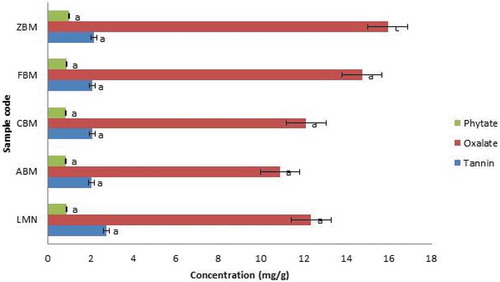
Figure 4. Antinutritional Factors of Extruded Sample of Biofortified Maize and Soybean Flour (Low Temperature and Speed; 90°C and 100 rpm)
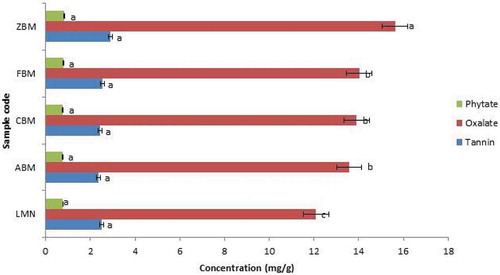
3.3. Carotenoid composition of the extruded samples under high and low temperatures
Tables and show the carotenoid composition of the extruded samples produced from bio-fortified maize (SAMMAZ 39) and soybean flours at high and low temperatures, respectively. Extrusion cooking is known to improve the nutritional quality of the food. There is excellent retention of nutrients due to the short residence time of the food material in the barrel as well as inactivation of antinutritional components (Singh et al., Citation2007). From all the samples, CBM-(85% maize and 15% soybean) had the highest value (4.57 µg/g) of lutein, and FBM-(80% maize and 20% soybean) had the lowest value (2.49 µg/g). The Zeaxanthin contents of the samples ranged from 3.49 (FBM) to 7.09 µg/g (ABM). There were significant differences (p < 0.05) in the variables, which might be due to the different concentrations of the flours in the mixtures.
Table 2. Carotenoid Composition (µg/g) of Extruded Sample of Biofortified Maize and Soybean Flour under High Temperature
Table 3. Carotenoid composition (µg/g) of the extruded sample of bio-fortified maize and soybean flour under low temperature (90 °C, 100rpm)
For β-Cryptoxanthin, contents ranged from 2.35 µg/g (ZBM) to 2.69 µg/g (CBM), significant differences (p < 0.05) were observed within the values. Cryptoxanthin has been identified as the most abundant carotenoids; whilst-carotene is present in smaller concentrations (Pillay, Citation2011).
The α-carotene contents ranged from 0.51 µg/g (ABM) to 0.71 µg/g (CBM). There was no significant difference (p > 0.05) in the variables. The 13-cis β-carotene content of the samples revealed that the result ranged between 0.31 µg/g (FBM) and 0.58 µg/g (ABM). The addition of soybean might be responsible for the decrease in the level of 13-cis β-carotene content observed.
Also, the trans-β-carotene contents of the samples range between the value of 0.63 µg/g and 1.42 µg/g. The result further revealed that FBM had the lowest value, while CBM had the most moderate trans-β-carotene content. More so, the 9-cis β-carotene content of the samples ranged from 0.58 to 0.86 µg/g. There was no significant difference (p > 0.05) observed in the variables. In diet, β-carotene and other carotenoids provide most of the vitamin A. The food labelling regulations require that vitamin A is calculated as micrograms of retinol or retinol equivalent (RE) on the basis that 6 μg of β-carotene equals 1 μg of retinol equivalent (FAO, Citation2009).
The Total β-carotene contents show a significant difference between the variables as the values ranged from 1.80 µg/g to 2.62 µg/g. Sample CBM had the lowest total Β-carotene, while sample ABM had the highest amount of total β-carotene. There was a significant difference (p < 0.05) in the variables. The most abundant provitamin A carotenoids in plant-based foods are β-carotene (two retinyl groups), β-cryptoxanthin (one retinyl group), and α-carotene (one retinyl group), but in most plant tissues they are substrates for hydroxylation reactions that produce the di-hydroxy- xanthophylls, lutein, and zeaxanthin, the most prevalent carotenoids in vegetative and seed tissues (Cazzonelli & Pogson, Citation2010; Howitt & Pogson, Citation2006).
The total xanthophylls of the samples ranged between 5.98 and 11.36 µg/g. Also, CBM (85% Maize 15% Soy) had the lowest total β-carotene, while ABM (90% Maize 10% Soy) had the highest value of total xanthophylls contents of carotenoids. Xanthophylls and carotenes result in the carotenoid pigments found in yellow and orange maize are responsible for the endosperm color (yellow or orange). The total xanthophylls values were higher than Provitamin A (PVA) values for all the samples and this corroborated with what had been reported that the lutein and zeaxanthin were major the carotenoids found in biofortified maize, and accounted for 70% of the total carotenoid content (Kean et al., Citation2008).
TheProvitamin A content of the samples ranged from 3.26 µg/g (FBM) to 4.30 µg/g (CBM). There was a significant difference (p < 0.05) in the mean values of PVA among the samples. The decrease in provitamin A carotenoid content of the extrudates with increasing soybean flour indicates that the soybean flour significantly influenced the content of provitamin A carotenoids in the extruded flour blends. There was no significant difference between the total xanthophylls and PVA values of the extrudates from both high and low temperatures. The marginal decrease in PVA content seen when extruded at the high temperature could probably be due to thermal degradation of the carotenoids, pigments which are known to be heat-sensitive. It is known that carotenoids in different food matrices are highly degraded by oxygen, temperature, and light during storage (Rodriguez-Amaya, Citation2015). In matrixes with high exposure to oxygen, such as flour, it was found that the oxygen level had more considerable influence on carotenoid degradation than temperature (Bechoff et al., Citation2010). However, the reasonably high content of total xanthophylls and PVA of the extrudate indicates the extruded maize and soybean flour could be used in addressing vitamin A deficiency (Beswa et al., Citation2016). Another advantage is that it is cheaper compared to other vitamins A supplementations).
4. Conclusions
Extrusion technology provides a new exciting opportunity to processed foods, and the extrusion process enhanced the nutritional qualities, as well as reduced the antinutritional components of the products. However, it is pertinent to pay attention to processing speed and temperature of foods containing carotene. Excess heat and longer time for processing carotenoid foods will result in loss of carotene. Sample ABM (90:10 biofortified Maize and soybean flours) showed high contents of carotenoid properties and low antinutritional properties and made it better than other samples. Existing results on levels of carotenoids retention suggest that bio-fortification can benefit nutritionally vulnerable population groups who traditionally cultivate staple foods such as maize and others or who have access to them.
The extrusion process enabled the reduction of antinutritional components present in the samples. It was observed that the increase in oxalate is as a result of the addition of soybean. It has been established that legumes contain a high amount of oxalate. Excess intake of food containing oxalate has been linked to an increased risk of kidney stones and other health problems. Fortification and supplementation of staple food is a vehicle to curb the deficiency of essential nutrients and improve diet in developing countries. Hence, further research into this should be conducted.
Additional information
Funding
Notes on contributors
M. O Adegunwa
M. O. Adegunwa (PhD) is a Senior Lecturer in the Department of Hospitality and Tourism, Federal University of Agriculture, Abeokuta, Nigeria.
E-mail: [email protected]
J. E Ayanlowo
J. E. Ayanlowo is a Graduate Student in the Department of Hospitality and Tourism, Federal University of Agriculture, Abeokuta, Nigeria.
E-mail: [email protected]
G. O Olatunde
G. O. Olatunde (PhD) is a Senior Lecturer in the Department of Food Science and Technology, Federal University of Agriculture, Abeokuta, Nigeria.
E-mail: [email protected]
L. A Adebanjo
L. A. Adebanjo is an Assistant Lecturer in the Department of Hospitality and Tourism, Federal
University of Agriculture, Abeokuta, Nigeria.
E-mail: [email protected]
E. O Alamu
E. O. Alamu (PhD) is an Associate Scientist at the Food and Nutrition Sciences Laboratory, International Institute of Tropical Agriculture (IITA), Southern Africa Research and Administration Hub (SARAH), P.O. Box 310142, Chelston, Lusaka, Zambia.
E-mail: [email protected].
References
- Adebowale, A. A., Sanni, L. O., & Awonarin, S. O. (2005). Effect of Texture modifies on the physicochemical and sensory properties of dried fufu. Food Science and Technology International, 11(5), 373–15. https://doi.org/10.1177/1082013205058531
- Ajibola, C. F., & Filani, A. (2015). Storage stability of deep-fried cowpea products (AKARA) incorporated with soy-flour and Aframomumdanielli. British Journal of Applied Science & Technology, 8(2), 204–212. https://doi.org/10.9734/BJAST/2015/16478
- Alamu, E. O., Maziya-Dixon, B., Menkir, A. ;., & Olaofe, O. (2015). Effects of husk and harvesting time on provitamin A activity and sensory properties of boiled fresh orange maize hybrids. Journal of Food Quality, 38(6), 387–395. https://doi.org/http://dx.doi.10.1111/jfq.12158
- Alamu, E. O., Popoola, I., & Maziya-Dixon, B. (2018). Effect of soybean (Glycine max (L.) Merr.) flour inclusion on the nutritional properties and consumer preference of fritters for improved household nutrition. Food Science & Nutrition, 6, 1–6. https://doi.org/10.1002/fsn3.751
- Annonye, J. C., Badifu, G. I. O., Inyang, C. U., Akpapunam, M. A., Odumudu, C. U., & Mbajika, V. I. (2007). Protein dispersibility index and trypsin inhibitor actiVitaminy of extruded blends of Acha/soybean: A response surface analysis. American Journal of Food Technology, 2(6), 502–511. https://doi.org/10.3923/ajft.2007.502.511
- AOAC. (2006). Official method of analysis of Association of Official Analytical Chemists (18th ed.). Association of Official Analytical Chemists, Gaithersburgs, MD.
- Bechoff, A., Westby, A., Owori, C., & Menya, G. (2010). Effect of drying and storage on the degradation of total carotenoids in orange-fleshed sweet potato cultivars. Journal of the Science of Food and Agriculture, 90(4), 622–629. DOI: 10.1002/jsfa.3859.
- Beswa, D., Dlamini, N., Amonsou, E., Siwela, M., & Derera, J. (2016). Effects of amaranth addition on the provitamin A content, and physical and antioxidant properties of extruded pro vitamin A-biofortified maize snacks. Journal of the Science of Food and Agriculture, 96(1), 28794. https://doi.org/10.1002/jsfa.7092
- Castells, M., Marín, S., Sanchis, V. & Ramos, A.J. (2005) Fate of mycotoxins in cereals during extrusion cooking: A review, Food Additives & Contaminants, 22:2, 150-157, DOI: 10.1080/02652030500037969
- Cazzonelli, C. I., & Pogson, B. J. (2010). Source to sink: Regulation of carotenoid biosynthesis in plants. Trends in Plant Science, 15(5), 266–274. https://doi.org/10.1016/j.tplants.2010.02.003
- Chessari, C. J., & Sellahewa, J. N. (2001). Effective process control. In R. Guy (Ed.), Extrusion cooking: Technologies and applications (pp. 83–107). Woodhead publishing.
- Doss, A., Pugalenthi, M., Vadivel, V. G., Subhashini, G., & Subash, R. A. (2011). Effects of processing technique on the nutritional composition and anti-nutrients content of under Utilized food legume Canavaliaensiformis L. DC. International Food Research Journal, 18(3), 965–970.
- Edema, M. O., Sanni, L. A., & Sanni, A. I. (2005). Evaluation of maize-soybean flour blends for sour maize bread production in Nigeria. African Journal of Biotechnology, 4(9), 911–918.
- Ferretti, G., Bacchetti, T., Belleggia, A., & Neri, D. (2010). Cherry antioxidants: From farm to table. Molecules, 15(10), 6993–7005. https://doi.org/10.3390/molecules15106993
- Food and Agriculture Organization of the United Nations (FAO). (2009). Agriculture data. Food and Agriculture Organization of United Nations.
- Girgih, A. T., Myrie, S. B., Aluko, R. E., & Jones, P. J. (2013). Is category A status assigned to soy protein and coronary heart disease risk reduction health claim by the United States food and drug administration still justifiable? Trends in Food Science and Technology, 30(2), 121–132. https://doi.org/10.1016/j.tifs.2012.12.003
- (Howitt, C. A., & Pogson, B. J. (2006). Carotenoid accumulation and function in seeds and non- green tissues. CSIRO Plant Industry. GPO Box 1600, Canberra, ACT 2601.
- Hu, Q. P., & Xu, J. G. (2011). Profiles of carotenoids, anthocyanins, phenolics, and antioxidant activity of selected colour waxy corn grains during maturation. Journal of Agricultural and Food Chemistry, 59(5), 2026–2033. https://doi.org/10.1021/jf104149q
- Iwe, M. O. (2003). The science and technology of soybean: chemistry nutrition processing and utilization (1st ed.). Rejoint Communication Services Ltd.
- Johnston, D. E., & Oliver, W. T. (1982). The influence of cooking technique on dietary fiber ofboiled potato. International Journal of Food Science & Technology, 17(1), 99–107. First published: February 1982. https://doi.org/10.1111/j.1365-2621.1982.tb00164.x
- Kean, E. G., Hamaker, B. R., & Ferruzzi, M. G. (2008). Carotenoid bioaccessibilty from whole grain and degermed maize meal products. Journal of Agricultural and Food Chemistry, 56(21), 9918–9926. https://doi.org/10.1021/jf8018613
- Kimura, M., Kobori, C. N., Rodriguez-Amaya, D. B., & Nestel, P. (2007). Screening and HPLC methods for carotenoids in sweetpotato, cassava and maize for plant breeding trials. Food Chemistry, 100(4), 1734–1746. https://doi.org/10.1016/j.foodchem.2005.10.020
- Lartey, A., Manu, A., Brown, K. H., Peerson, J. M., & Dewey, K. G. (1999). A randomized community-based trial of the effects of improved, centrally processed complementary foods on growth and micronutrient status of Ghanaian infants from 6 to 12 mo of age. The American Journal of Clinical Nutrition, 70(3), 391–404. https://doi.org/10.1093/ajcn/70.3.391
- Leyva, J. A., Artiga, M. P., Aragon, M., & Perez, J. J. (1990). Atomic absorption of UV-VIS absorption spectrophotometric determination of oxalate in urine by ligand exchange extraction. Clinica Chimica Acta, 195(1–2), 47–56. https://doi.org/10.1016/0009-8981(90)90193-V
- Makkar, H. P. S., Blummel, M., Borowy, N. K., & Becker, K. (1993). Gravimetricdetermination of tannins and their correlations with chemical and protein precipitationmethods. Journal of the Science of Food and Agriculture, 61(2), 161–165. https://doi.org/10.1002/jsfa.2740610205
- Martínez-Bustos, F., Aguilar-Palazuelos, E., & Galicia-García, T. (2012). Thermoplastic behavior of biopolymers during extrusion. In V. R. N. Telis (Ed.), Biopolymer engineering in food processing (pp. 245–278). CRC Press.
- Mbaeyi, I. E., & Onweluzo, J. C. (2010). Effect of sprouting and pre-gelatinization on the physicochemical properties of sorghum-pigeon pea composite blend used for the production of breakfast cereal. Journal of Tropical Agriculture, Food, Environment and Extension, 9(1), 8–17.DOI: 10.4314/as.v9i1.57448
- Melaku, S., Peter, K. J., & Tegegne, A. (2005). Intake, digestibility and passage rate in Menz sheep fed tef (Eragrostistef) straw supplemented with dried leaves of selected multipurpose trees, their mixture of wheat bran. Small Ruminant Research, 56(1–3), 139 149. https://doi.org/10.1016/j.smallrumres.2004.06.008
- Mesa, D. M., Silvan, J. M., Olza, J., Gil, A., & Del Castillo, M. D. (2008). Antioxidant properties of soy protein-fructooligosaccharideglycation systems and its hydrolyzates. Food Research International, 41(6), 606–615. https://doi.org/10.1016/j.foodres.2008.03.010
- Obatolu, V. A. (2002). Nutrient and sensory qualities of Extruded malted or unmalted millet/soybean mixture. Food Chemistry, 76(2), 129–133. https://doi.org/10.1016/s03088146(01)00188-1
- Owens, B. F., Lipka, A. E., Magallanes-Lundback, M., Tiede, T., Diepenbrock, C. H., Kandianis, C. B., Kim, E., Cepela, J., Mateos- Hernandez, M., Buell, C. R., Buckler, E. S., DellaPenna, D., Gore, M. A., & Rocheford, T. (2014). A foundation for provitamin A biofortification of maize: A genome-wide association and genomic prediction models of carotenoid levels. Genetics, 198(4), 1699–1716. https://doi.org/10.1534/genetics.114.169979 PMid:25258377
- Pillay, K. Nutritional quality and consumer acceptability of provitamin A-biofortified maize. Thesis (Ph.D.) University of KwaZulu-Natal, Pietermaritzburg, 2011. http://www.hdl.handle.net/10413/8458.
- Riaz, M. N., & Rokey, G. J. (2011). An introduction to food and feed extrusion and associated terminology. In Extrusion problems solved: Food, pet food and feed (pp. 1–9). Cambridge, UK: Woodhead Publishing. ISBN 978-1-84569-664-1, Hardcover,184 pages.
- Rodriguez-Amaya, D. B. (2001). A guide to carotenoid analysis in foods. ILSI Human Nutrition Institute.
- Rodriguez-Amaya, D. B. (2015). Carotenes and xanthophylls as antioxidants. Book: Handbook of antioxidants for food preservation (pp. 17–50), Editors: Fereidoon Shahidi. Woodhead Publishing. ISBN: 9781782420897. https://doi.org/10.1016/B978-178242-089-7.0000-6
- Sandberg, A. S. (2002). Bioavailability of minerals in legumes. British Journal of Nutrition, 88(S3), 281–285. https://doi.org/10.1079/BJN/2002718
- Singh, R. P., Dhania, G., Sharma, A., & Jaiwal, P. K. (2007). Biotechnological approach to improvephytoremediation efficiency for environmental contaminants. In S. N. Singh & R. D. Tripahti (Eds.), Environmental bioremediation technologies. Springer (pp. 223–258).
- Tchinmegni, F. I, & Djeukam, P. S. V. (2016). Study of fermentation and extrusion on the antinutritional composition and digestibility of millet and soybean flour blends.international. Journal Of Research in Pharmacy and Biosciences, 3(7), 14-22.
- Tiwari, A., & Jha, S. K. (2017). Extrusion cooking technology: Principal mechanism andeffect on direct expanded snacks - An overview. International Journal of Food Studies, 6(1), 113–138. https://doi.org/10.7455/ijfs/6.1.2017.a10
- Wang, L. S., & Stoner, G. D. (2008). Anthocyanins and their role in cancer prevention. Cancer Letters, 269,(2), 281–290. doi: 10.1016/j.canlet.2008.05.020.
- Weaver, C. M., & Kannan, S. (2002). Phytate and mineral bioavailability. In N. R. Reddy & S. K. Sathe (Eds.), Food phytates Florida (pp. 211–223). CRC.
- Weiwen, C., & Liebman, M. (2004). Oxalate content of legumes, nuts, and grain-based Flours. Journal of Food Composition and Analysis, 18(7), 723–729. https://doi.org/10.1016/j.jfca.2004.07.001
- Wolf, B. (2010). Polysaccharide functionality through extrusion processing. Current OpinioninColloid & Interface Science, 15(1), 50–54. https://doi.org/10.1016/j.cocis.2009.11.011
- Zhou, X., McQuinn, R., Fei, Z., Wolters, A. M., Van Eck, J., Brown, C., Giovannoni, J. J., & Li, L. (2011). Regulatory control of high levels of carotenoid accumulation in potato tubers. Plant, Cell & Environment, 34(6), 1020–1030. https://doi.org/10.1111/j.1365-3040.2011.02301.x
- Zilić, S., Serpen, A., Akıllıoğlu, G., Gökmen, V., & Vančetović, J. (2012). Phenolic compounds, carotenoids, anthocyanins, and antioxidant capacity of coloured maize (Zea mays L.) kernels. Journal of Agricultural and Food Chemistry, 60(5), 1224–1231. https://doi.org/10.1021/jf204367z