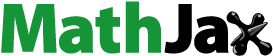
ABSTRACT
Microalgae, especially Chlorella sp., have been used for centuries as food and currently their biotechnological potential is notable for the presence of several compounds relevant to the market, like PHB. Microalgae are appealing because of the increasing demand for biopolymers like PHB. Therefore, the present study is aimed to determine the efficacy of biomass production and yield of PHB producing microalgae isolated and identified by phylogenetic analysis. The parameter was optimized as pH 7, temperature 30°C, under sunlight with 0.2% of sodium bicarbonate for higher biomass and PHB production. The extracted PHB were characterized through SEM, EDAX, XRD and FT-IR. In GC-MS analysis the major peak represents Benzyl butyl phthalate confirming the polymer of PHB. DSC-TGA, was performed and the melting peak of PHB was found as 342.1°C conferring to thermogravimetric analysis, the range of temperature for rapid thermal degradation of PHB was at 418°C to 420°C with the degradation peaking at 418.2°C the total weight loss within this temperature range was 98.5%. Hence, maximum 80% of PHB was produced from Chlorella sp. in addition to sequestering CO2.
Introduction
Algae are the most interesting diverse groups of photosynthetic organisms that play an important role in nature. Algae produce a wide range of bioactive compounds polyunsaturated fatty acids, carotenoids, vitamins, sterols, toxins and polysaccharides [Citation1]. Algae reduces carbon dioxide level in air and maintains neutral CO2 in the environment. The growth rate of microalgae and cyanobacteria is much faster than land plants. Algae are a promising source of biomass for alternative fuel productions accumulating large amounts of neutral lipids that are stored as cytosolic lipid bodies [Citation2]. Microalgae possess an efficient biological system capable of utilizing sunlight for the production of organic compounds, better biomass source for bioplastic production. Micro-algae are more sustainable and contribute to the economy of a nation.. Bioplastics can be used in food packaging, pharmaceuticals and cosmetics [Citation3–8].
There is a worldwide concern regarding the development of the biodegradable plastic materials as a remedy toward harmful effects caused by plastic wastes on the environment and cause pollution. These materials, which are synthesized chemically by polymerization, cause air pollution and waste management problems. The fear of depletion of wood resources has established plastics as the material of choice in many applications [Citation9].
Biopolymers show interesting rheological properties due to high molecular weight and different structural features influenced by intermolecular binding properties. Many biopolymers are applied in the food industries as gelling agent, emulsion, and foam stabilizers and crystallizations inhibitions in frozen foods [Citation1,Citation10]. Biopolymers are generally sustainable and renewable materials as they were made from biological origin and it has a surprising number of responsibilities in our body [Citation11,Citation12]. Biopolymers exhibit important properties viz., biodegradability, biocompatibility, anti-bacterial activity, etc. It can be produced from natural raw materials like starch, sugar, cellulose etc., [Citation13]. Biopolymers have immuno-biological effects, nanostructure properties, and act as a promising model for immuno pharmaco-toxicology and pharmacodynamic aspects. [Citation1,Citation14–16].
Many of these applications can be found in the medical field such as drug delivery system, wound closure and healing properties, surgical implant devices, biodegradable capsules, replacement of human tissue, food containers, waste bags, filtration etc. [Citation17]. Polyhydroxy butyrates are bio-plastics that are produced by many microbial species under carbon-rich and nutrient starvation conditions [Citation18]. There are several polymers naturally occurring in animals, plants, and lower organisms like bacteria, fungi, and algae [Citation12,Citation13,Citation19].
About 140 million tons of plastic is consumed every year worldwide, which necessitates the processing of approximately 150 million tons of fossil fuels and directly causes immense amounts of waste that can take thousands of years to naturally degrade [Citation20]. In addition, the environmental threats caused by these conventional plastics create an urgent need in implementing the usage of biodegradable plastics as a replacement for non-biodegradable plastics [Citation21]. The tolerant negative attribute of revolution to biodegradation, toxicity after incineration of massive waste accumulation in landfills and water bodies, are the problems associated with the synthetic polymer [Citation22].
Biodegradable form of plastic Polyhydroxybutyrate was first characterized in the mid 1920s by French researchers (called PHB). It is aliphatic polyester with thermoplastic properties, which is naturally produced by certain microorganisms as storage compound and is 100% biodegradable [Citation5,Citation23]. PHB is a common biopolymer which is an attractive alternative to common plastics due to its hydrophobicity, complete biodegradability, and biocompatibility [Citation24]. Bacteria such as Alcaligenes eutrophys produce 85% and algae such as Spirulina sp. produce 73% of PHB [Citation25,Citation26]. However, PHB produced by bacteria for industrial applications are limited due to high cost of cultivation when compared to petroleum-derived plastics [Citation27]. Algae can be considered as an alternative source of PHB production due to their photoautotrophic nature, i.e. able to fix CO2 with minimal nutrient consumption [Citation28].
Petroleum-based plastics have been used for more than seventy years in our day-to-day applications owing to their versatility and durability [Citation29,Citation30]. C. Vulgaris is photosynthetic popular microalgae used as SCP, high CO2 fixer and bio-energy production. [Citation31]. Their CO2 fixation efficiencies are approximately 10–50 times higher than plants [Citation32].
The widespread global consumption of plastics has attributed to environmental pollution because of their non-biodegradable nature and thus prevails within the environment. Biopolymers are polymers that occur in nature composed of carbohydrates and lipids. These plastic materials such as PHB have benefits over synthetic plastics due to their biodegradability.
Materials and methods
Isolation and purification
The algae species were isolated and purified from different sources by serial dilution followed by spreading and quadrant streaking on Bold Base medium agar plates. Morphological identification was performed through microscopic observation. Total DNA was extracted from the isolated strain and phylogenetic analysis was done.
Culture condition
Microalgal isolates should be devoid of bacterial and fungal spores. The other microbes were removed by axenization procedure using antibiotics (Meropenum – 2 µg/mL and Nystatin – 5 µg/mL). The purified 5 ml of axenic culture was inoculated in their respective mediums and incubated at 25°C under illumination of yellow fluorescent light. The light and dark conditions were maintained (16 h:8 h) for 21 days.
Microscopic visualization of PHB
1.0 mg was dissolved in dimethyl sulfoxide (1.0 ml) to obtain staining solution. Two drops of the staining solution was added to 200 µl of algal culture which was then incubated at 55°C for 10 min. The cells were transferred to a glass slide and viewed by a fluorescent microscope at excitation wavelength of 450–490 nm under 1000X magnification [Citation29].
Primary cultivation of algae
5.0 ml of axenic culture was inoculated into their respective mediums and incubated at 25°C under illumination of yellow fluorescent light. The light and dark conditions were maintained (16 h: 8 h) for 21 days.
Media optimization
70 ml of the following media Bold basal medium, BG11 medium, Chu 10 modified medium, ASN III medium, Cyanophycean medium, and algal culture medium were prepared. 30 ml of Chlorella sp. and Neochloris sp. was inoculated and the culture was incubated by gentle stirring. Illumination was provided under 37.5 µ mol–1m–1sec–1 intensity 16:8 h photo period for 15 days. The growth was observed regular intervals.
Physicochemical parameters
The concentration of algal growth was based on the earlier optimized results of biomass at which the higher growth was observed from six different media. To determine the effect of pH and temperature on the growth and production of PHB, 70 ml of the microalgae was inoculated in to 30 ml of cyanophycean medium. The varying pH and temperature analyzed in the study was 5–8 and 20°C to 35°C respectively. The effect of different light source viz., normal light, fluorescent light, effect of dark condition and sunlight on the growth of microalgae were tested. Different concentrations of carbon (sodium bicarbonate), 0.2 g, 0.4 g, 0.6 g, 0.8 g, were tested for the increased production of PHB. The growth was measured at 630 nm.
Lab scale cultivation of microalgae under optimized condition
Cyanophycean medium (Potassium nitrate-5.0 gm, Di potassium phosphate 0.20 gm, magnesium sulfate 0.10 gm, pH-7.1/1000 ml) was prepared and microalga was inoculated into it. The cultures were incubated under optimized parameters of pH, temperature, light source, and sodium bicarbonate for the lab scale cultivation (up to 1 L).
The microalgae was harvested and the biomass was measured.
Chlorophyll estimation
The spectrophotometric method [Citation33] was used for estimating the chlorophyll content in the cells. A culture volume corresponding to 5 mg of dry biomass was centrifuged (800 × g, 2 min) and the cells were harvested. The biomass was resuspended in an acetone–water mixture (8 ml, 90% v/v acetone) to extract the pigments. The tube was shaken vigorously; ultra sonicated for 10 min, and allowed to stand in the dark at 4◦C for 48 h. Further processing was carried out in room temperature. Acetone was added to compensate for any evaporation and the sample was centrifuged (800 × g, 5 min). The supernatant was recovered and its optical density was read at 665, 645 and 630 nm.
The following equations were used to calculate the concentrations of chlorophyll:
Where cha, chb and chc are the concentrations (mg l−1) of chlorophyll a, b and c, respectively, and odxxx is the optical density measured at the specified wavelength. The optical density measurements were made in quartz cuvettes of 1 cm light path. If the absorbance value exceeded 0.8 units, the sample was diluted with the solvent (acetone–water) to bring the measurement within range.
Protein estimation
Micro algal biomass protein estimation was done using Lowry’s method [Citation34].
Carbohydrate estimation
The anthrone–sulfuric acid Osborne (1986) method was used to estimate the carbohydrate from the micro-algal biomass [Citation35]. All the tests were performed in duplicates their mean and ± standard values are calculated.
Lipid estimation
The lipid content was estimated using chloroform-methanol mixture [Citation36]. For 400 mg of sample, 5 ml of chloroform-methanol (2:1) mixture was added. The mixture was incubated at room temperature for 24 h. After incubation the mixture was filtered using a filter paper. The filtrate was collected in a 10 ml pre-weighed beaker. The chloroform-methanol mixture was evaporated on a hot plate leaving a residue at the bottom of the beaker. The beaker with the residue and the weight of the empty beaker was calculated to know the weight of the lipid present in the sample.
Extraction of poly hydroxy Butyrate
500 ml of sample was taken and centrifuged at 10,000 rpm for 15 minutes. The supernatant was discarded and the pellet was treated with 10 ml of sodium hypochlorite and the mixture was incubated at 30°C for 2 hours. After incubation, the mixture was centrifuged at 5000 rpm for 15 minutes and the pellet was washed with distilled water and methanol. After washing, it was dissolved in 5 ml of boiling chloroform. The chloroform solution was concentrated to a small volume. A small volume of cold methanol was added and the sample was refrigerated overnight. The precipitated PHB was collected by centrifugation and stored at 4°C [Citation37,Citation38].
SEM with EDX
With the help of an automated SEM with EDX analysis system nonmetallic micro inclusions in samples can be detected in a metallo graphically prepared surface area. Here, SEM with EDX were used to determine positions, size, shape and composition of the particles [Citation38].
Fourier transform infra-red (FT-IR) analysis
2 mg of PHB was mixed with KBr and dried. The sample was analyzed using FT-IR spectrophotometer (Instrument model Perkin Elmer model spectrum-I Pc). The spectral range attained was matched with the control (commercial PHB) [Citation39].
XRD analysis
X-Ray diffraction measurements were analyzed using Ni-filtered Cu K radiation (bruker d8 advance diffracto meter). The patterns were documented in the 2 range 20◦C-65◦C at a scan rate of 10°C for 1 min at room temperature [Citation39].
GC-MS analysis
The extracted PHB was analyzed using GC-MS. The spectrums of the components were compared with the database of spectrum of known components stored in the GC-MS NIST library.
Thermal properties analysis
Thermo gravimetric differential thermal analysis (TG-DTA) was done under a nitrogen atmosphere at a heating rate of 20°C/min to observe the degradation pattern of PHB [Citation39].
Result
Sample collection
Water samples were collected from Gomuki River in Kallakurichi by random sampling technique (). Geographical location is shown in . The water sample properties viz., pH and temperature are the deciding factors of algal diversity. Hence, the physical parameters of the water samples were analyzed at the time of collection. The pH of the water samples ranged from pH 7.0 to pH 7.5. The physicochemical parameters of the collected water samples are listed in .
Table 1. Physical characteristics of water quality of selected localities of Gomuki River, Kallakurichi (DT), India
Isolation of microalgae
Based on the microscopic observation 27 types of microalgae were observed (Plate 1). The samples were categorized and listed for possible algal strains that are included in . Among the 27 algae only 2 strains were selected based on growth/biomass and PHB production.
Table 2. Microalgal biodiversity of the collected water sample
Micro algae strain
The water samples were inoculated in Bold Basal medium agar plate and screened for unialgal strains. Plate 2 (a & b) shows the growth of unialgal strains on BBM agar plate. Further the isolates were confirmed microscopically for their monoculture state. Two isolates have been identified as Chlorella sp. and Neochloris sp. subcultured in BBM and examined microscopically at 400X magnification for their purity (Plate 3). The axenic microalgal strains were scaled up from test tubes up to 100 ml volume in BBM medium.
Screening and staining of PHB
Screening of PHB production was done using Nile red-staining method on both Chlorella sp. and Neochloris sp. The Chlorella sp. showed better PHB accumulation Plate 11 (a). Based on the morphological features and the intensity of fluorescence maximum producers of PHB were screened. The microalgae Chlorella sp were identified as Chlorella vulgaris by molecular identification. (GenBank accession number - MZ045839).
Media optimization
Among the tested six different media viz., BBM, BG11, Chu10, ACM, ASNIII medium, the Cyanophycean medium showed increased growth rate and higher production of PHB Plate 4 (a & b).
Effect of pH on algae Growth
Algae were grown in pH range of 5 to 7 showed a significant effect. At pH 7.0 Chlorella sp. and Neochloris sp. showed maximum intensity measured in terms of absorbance Plate 5 (aChlorella sp & b Neochloris sp).
Effect of temperature on algae culture
Microalgae when grown at different temperature showed significant effect. From the results, it is evident that growth was influenced by the temperature. The results indicate that optimum temperature was 30°C for Chlorella sp., and Neochloris sp., Plate 6 (a Chlorella sp., & b Neochloris sp.).
Effect of light source on microalgae
Microalgae when grown in different light source show significant effect on the growth. The effect of light source on algal growth revealed that growth was influenced by subjecting to different light sources. The results indicate that Chlorella sp. and Neochloris sp. showed higher growth rate in biomass when grown in sun light Plate 7 (a & b).
Effect of carbon source on microalgae
Microalgae when grown in different concentrations of carbon showed significant effect on it. The results for effect of sodium bicarbonate on algal growth revealed that sodium bicarbonate at 0.6 gm and 0.2 gm was found to be optimum and increased the growth of Chlorella sp. and Neochloris sp.--. The effects of different concentrations of carbon source were presented in Plate 8 (a Chlorella sp. & b).
Lab scale cultivation of microalgae strain in optimized condition
With the optimized parameters the microalgae were grown and biomass estimation studies were carried out Plate 9 (a & b). The amount of dry weight, chlorophyll, carbohydrate, protein and lipid produced from these algae are listed in . The amount of dry weight produced by Chlorella sp. was 0.619 ± 0.02 g/100 ml higher than the Neochloris sp. 0.21 ± 0.01 g/100 ml. The Chlorella sp. was chosen for the PHB production.
Table 3. Biomass, protein, lipid, carbohydrate and pigment estimation from the microalgal isolates
Chlorophyll estimation
The chlorophyll a (16.57510 mg l−1), b (6.18414 mg l−1) and c (4.04568, mg l−1) produce by Chlorella sp. shows high concentration in the cyanophycin. .
Extraction of poly hydroxy butyrate
The PHB extraction was performed using solvent extraction method. The PHB dry weight extracted was 2.15 gram/500 ml Plate 10.
SEM with EDAX analysis of PHB
The PHB granules extracted from Chlorella sp. were observed by SEM with EDAX. The shape and size of the PHB granules appeared spherical and intact in shape. The granules were either single or in budding groups Plate 11 (b, c & d).
Fourier transform infra red (FT-IR) analysis
The FTIR spectrums of the extracted polymer recorded large absorption peak at 3416.10 cm−1 – 3198.22 cm−1 of OH stretching and C-H was between 2955.50 cm−1 – 2582.94 cm−1. The absorption band at 1712.52 cm−1 attributed to the stretching vibration of the carboxyl bond (C = O). The band at 2395 cm−1 was assigned to the C ≡ C stretching of alkynes. Absorption peaks between 1537.95 cm−1 and 1655.59 cm−1 indicate the presence of nitro compounds. The bands between 1578 cm−1 arise from N-H vibration of amines. Intense bands centered at 1043.80 cm−1 – 1255.47 cm−1 were assigned to C-N vibrations of amine group. From the above details it is concluded that the extracted compound is PHB .
XRD analysis
There were distinct diffraction peaks at 25°C to 30°C in X-RD analysis. The crystalline size was found to be 528.27 Å ().
GC-MS of PHB
The Gas Chromatogram of the isolated polymer showed a major peak with retention time of 11.750 min (Peak 1) with other minor peaks at 9.992 to 3.354 indicate the presence of PHB. The major peak represents Benzylbutyl phthalate confirming the polymer of PHB , .
Table 4. GC-MS analysis of PHB extracted from Chlorella sp.
TGA analysis of PHB
PHB extracted from Chlorella sp. were analyzed for thermal properties. Based on the results obtained from DSC-TGA, the melting peak of PHB was at 342.1°C conferring to thermogravimetric analysis, the range of temperature for rapid thermal degradation of PHB was 418°C to 420°C with the degradation peaking at 418.2°C the total weight loss within this temperature range is 98.5% ( a & b).
Discussion
Five samples were collected from Gomuki River and 27 types of phytoplankton were observed. Among 27 phytoplankton only 2 microalgal strains, belonging to Chlorophyceae family were identified for PHB production. In the present study green algae chlorophyta was identified as PHB producers since they accumulate 20%–50% of their dry cell weight in the form of lipid, primarily triaglycerides under unfavorable conditions[Citation40].
The microalgae stained with Nile red was observed under fluorescent microscope showed the presence of PHB. Based on the morphology and intensity of fluorescence PHB were screened. Nile red stain appeared to be a more specific dye for poly-b-hydroxybutyrate compared to Sudan black B stain [Citation41].
In this study the growth of microalgae was optimized in six different medium among them Cyanophycean medium showed maximum level of biomass when compared to other medium.
The effect of pH on microalgae growth 7.5 to 8.5 was found for maximum PHB production and 28°C temperature under light and light photoperiod are better for PHB accumulation [Citation32]. In this present study pH 7 was showed to be optimum production, maximum PHB was produced at 30°C. Growth in sunlight was optimum for maximum biomass production.
In previous reports the presence of sodium bicarbonate as a carbon source enhanced the PHB production [Citation41], since sodium bicarbonate is found to be one of the key nutrition for the production of PHB [Citation42]. In a recent study, it was reported that 60% (W/V) of NaHCO3 resulted in high biomass accumulation of PHB [Citation39]. PHB levels reached up to 16.4% from CO2 after 14 days of cultivation under nitrogen and phosphorus conditions [Citation43]. In the present study 0.2% percent of sodium bicarbonate showed maximum biomass production.
In the present study maximum biomass yield of 2.01 g/L−1 and 20% of PHB content under sunlight at 30 ± 1°C temperature was observed. Dayanand [Citation44–51] study showed the biomass of 30% of PHB content under sunlight in open raceway pond process.
In the FTIR analysis the large absorption peak was OH stretching and C-H. The stretching vibration of the carboxyl bond (C = O) was assigned to the C ≡ C stretching of alkynes. Absorption peaks indicate the presence of nitro compounds, and amines groups.
The major peak reflected in GC-MS analysis was Benzyl butyl phthalate [Citation52–87] with the peak area at 11.7 min retention time and other group confirming the presence of PHB.
In DSC-TGA, the melting peak of PHB was found to be 342.1°C and the total weight loss within this temperature range was 98.5%. The crystallinity of the PHB had been determined for standard PHB in previous reported [Citation32].
Conclusion
The microalgae strains isolated in the present study showed PHB accumulation, proving Chlorella sp. as a good source of PHB. The optimum physicochemical conditions for PHB accumulation were; pH 7, 30°C, sunlight with 0.2% of sodium bicarbonate (carbon source) Chlorella sp. accumulated 80% at of PHB under these optimized condition, FTIR, XRD, GCMS analyses confirmed the isolated polymer as PHB. Thermal properties and mechanical properties of the isolated PHB were similar to standard PHB. Chlorella sp. as PHB producers have the benefit of altering waste carbon dioxide, greenhouse gas to eco friendly plastics by utilizing the energy of sunlight. Hence it may be concluded that Chlorella sp. can be exploited for PHB production at a larger scale.
Highlights
Among 27 phytoplanktons’s, Chlorella sp. Neochloris sp. was isolated from freshwater.
The optimization studies on Chlorella sp. showed maximum PHB production.
Characterized through SEM, EDAX, XRD, FT-IR.
The presence of Benzyl butyl phthalate confirming the PHB through GC-MS analysis.
TGA & DSC confirmed the presence of PHB.
Maximum (80%) PHB was produced from Chlorella vulgaris.
Disclosure statement
No potential conflict of interest was reported by the author(s).
Additional information
Funding
Notes on contributors
Kanaga Selvaraj
Kanaga Selvaraj, Research Scholar, Fermentation research Laboratory, Department of Microbiology, Periyar University, Salem-636011, India. [email protected]
Nandhini Vishvanathan
Nandhini Visvanathan, MSc., Student, Fermentation research Laboratory, Department of Microbiology, Periyar University, Salem-636011, India. [email protected]
Ramamurthy Dhandapani
Dhandapanij RamamurthyAssistant Professor, Fermentation research Laboratory, Department of Microbiology, Periyar University, Salem-636011, India. [email protected]
References
- Halaj M, Maria M, Martina S, et al. Chemico-physical and pharmacodynamic properties of extracellular Dictyosphaerium chlorelloides biopolymer. Carbohydr Polym. 2018;198:215–224.
- Liu A, Chen W, Zheng L, et al. Identification of high-lipid producers for biodiesel production from forty-three green algal isolates in China progress in natural science. Mater Int. 2011;21:269−276.
- Muhammad R, Ghulam M, Sheraz AM, et al. Exploring the potential of microalgae for new biotechnology applications and beyond, a review. Renewable Sustainable Energy. 2018;92:394–404.
- Chisti Y. Biodiesel from microalgae. Biotechnol Adv. 2007;25(3):294–306.
- Hempel F, Bozarth AS, Lindenkamp N, et al. Microalgae as bioreactors for bioplastic production. Microb Cell Factories. 2011;10(1):81.
- Rahman A, Miller CD. Microalgae as a source of bioplastics. Edited by, Rastogi RP, Madamwar D, Pandey A, et al. editors Algal green chemistry, recent progress in biotechnology. Amsterdam. The Netherlands: Elsevier; 2017. 121–138.
- Mohan SV, Hemalatha M, Chakraborty D, et al. Algal biorefinery models with self-sustainable closed loop approach: trends and prospective for blue-bioeconomy. Bioresour Technol. 2019;295:122–128.
- Moreno GL, Adjalle K, Barnabe S, et al. Microalgae biomass production for a biorefinery system recent advances and the way towards sustainability. Renewable Sustainable Energy Rev. 2017;76:493–506.
- Sasikala S, Santhosh S, Hemalatha V, et al. A new method for the production of polyhydroxyalkanoates by Bacillus sp. and detect the presence of PHA. synthase. Smart Sci. 2018; 6:2, 105-116.
- Mishra A, Kavita K, Jha B. Characterization of extracellular polymeric substances produced by micro-algae Dunaliella salina. Carbohydr Polym. 2011;83(2):852–857.
- Babu RP, Connor KO, Seeram R. Current progress on bio-based polymers and their future trends. Prog Biomater. 2013;2(1):1–16.
- Gopi S, Amalraj A, Thomas S. Effective drug delivery system of biopolymers based on nanomaterials and hydrogels a review. Drug Des. 2016;5:1–7.
- Nandini AP, Geetha BH, Mahadevappa YK. Smart biopolymers and their biomedical applications. Procedia Manuf. 2017;12:263–279.
- Van De V, Kiekens P. Biopolymers, overview of several properties and consequences on their applications. Polym Test. 2002;21(4):433–442.
- Mishra A, Jha B. Isolation and characterization of extracellular polymeric substances from micro-algae Dunaliella salina under salt stress. Biores Techno. 2009;100(13):3382–3386.
- Cybulska J, Halaj M, Cepak V, et al. Nanostructure features of microalgae biopolymer. Starch Starke. 2016;68(7–8):7–8.
- Ehret P. Biodegradable nonwovens, ITB Nonwovens industrial textiles. Deposa Nonwovens: Deposable Disposables’, INSIGHT 96 San Antonio. Vol. 3, 1996. p. 29–30.
- Kritika S, Pragya R. Study of polyhydroxybutyrate producing bacillus sp. isolated from soil. Res J Recent Sci. 2015; (4): 61–69.
- Salerno A, Pascual CD. Bio-based polymers, supercritical fluids and tissue engineering. Process Biochem. 2015;50(5):826–838.
- Suriyamongkol P, Weselake R, Narine S, et al. Biotechnological approaches for the production of polyhydroxyalkanoates in microorganisms and plant a review. Biotechnol Adv. 2007;25(2):148–175.
- Abeed FMBD, Reddy PMRM, Khan HA. Biosynthesis of poly(3-hydroxybutyrate) (PHB) by Cupriavidus necator H16 from jatropha oil as carbon source. Bioprocess Biosyst Eng. 2014;37(5):943–951.
- Veeramanikandan V, Mrudula S, Yuvaraj R, et al. Optimization of poly b-hydroxy butyrate production by Alcaligenes latus MTCC2311 using central composite design. Int J Comput Appl. 2013;68:0975–8887.
- Madison LL, Huisman GW. Metabolic engineering of poly(β)hydroxyalkanoates, from DNA to plastic. Microbiol Mol Biol Rev. 1999;63:21–53.
- Vincenzini M, Sili C, Philippis R, et al. Occurrence of poly-beta-hydroxybutyrate in Spirulina species. J Bacteriol. 1990;172(5):2791–2792.
- Valappil SP, Misra SK, Boccaccini AR, et al. Large-scale production and efficient recovery of PHB with desirable material properties, from the newly characterised bacillus cereus SPV. J Biotechnol. 2007;132(3):251–258.
- Balaji S, Gopi K, Muthuvelan B. A review on production of poly hydroxybutyrates from cyanobacteria for the production of bioplastics. Algal Res. 2013;2(3):278–285.
- Khetkorn W, Aran I, Peter L, et al. Enhancement of poly(β)hydroxybutyrate production in Synechocystis sp. PCC 6803 by overexpression of its native biosynthetic genes. Bioresour Technol. 2016;214:761–768.
- Zinn M, Witholtb B, Eglia T. Occurrence, synthesis and medical application of bacterial polyhydroxyalkanote. Adv Drug Delive Rev. 2001;53(1):5–21.
- Ojumu TV, Yu J, Solomon BO. Production of polyhydroxyalkanoates, a bacterial biodegradable polymer. Afr J Biotechnol. 2004;3(1):18–24.
- Saratale RG, Chandrasekar K, Ackmez M, et al. Bioelectrochemical systems using microalgae A concise research update. Chemosphere. 2017;177:35–43.
- Ming C, Chong X, Xie Y. Photocatalytic nitrogen fixation, the role of defects in photocatalysts. J Mater Chem A. 2019;7(34):19616–19633.
- Ansari S, Fatma T. Cyanobacterial polyhydroxybutyrate (PHB), screening, optimization and characterization. PLoS ONE. 2016;11(6):158–168.
- Hansmann E. Pigment analysis in handbook of phycological methods, culture methods and growth measurements. Stein JR, Edited by. New York: Cambridge University Press; 1973. p. 359–368.
- Lowry OH, Rosenbrough NJ, Farr AL, et al. Protein measurement with the folin phenol reagent. J Biol Chem. 1951;193(1):265–275.
- Osborne B, Raven JA. Growth light level and photon absorption by cells of Chlamydomonas rheinhardii, Dunaliella tertiolecta (Chlorophyceae, Volvocales), Scenedesmus obliquus (Chlorophyceae, Chlorococcales) and Euglena viridis (Euglenophyceae, Euglenales). Br Phycol J. 1986;21(3):303–313.
- Folch J, Lees M, Stanley GHS. A simple method for the isolation and purification of total lipids from animal tissues. J Biol Chem. 1957;226(1):497–509.
- Rebecca R, Iyer PR. Isolation and optimization of PHB (Poly-β-hydroxybutyrate) based biodegradable plastics from Chlorella vulgaris. J Bioremediat Biodegrad. 2018;9(2):433.
- Sayeda MA, Gamila HA. Analysis of polyhydroxybutrate and bioplastic production from microalgae. Bull National Res Centre. 2019;43(1):97.
- Kavitha G, Chidambaram K, Krishnan S, et al. Mass cultivation of UV-B adapted Arthrospira platensis RRGK under open raceway pond for the production of Poly-hydroxyl butyrate. Int J Biol Macromol. 2016;93:1304–1316.
- DOE (U.S. Department of Energy) (2016) National algal biofuels technology review. U.S. department of energy, office of energy efficiency and renewable energy, bioenergy technologies office. Bioenergy energy gov.
- Nishioka M, Nakai K, Miyake M, et al. Production of poly(β)hydroxybutyrate by thermophilic cyanobacteria Synechococcus sp. MA19, under phosphate limitation. Biotechnol Lett. 2001;23(14):1095–1099.
- Arun A, Murrugappan R, Ravindran ADD, et al. Utilization of various industrial wastes for the production of poly(β)hydroxy butyrate (PHB) by Alcaligenes eutrophus. Afr J Biotechnol. 2006;5:1524–1527.
- Donya K, Pflügl S, Nischkauer W, et al. Photosynthetic poly-β-hydroxybutyrate accumulation in unicellular cyanobacterium Synechocystis sp. PCC 6714. AMB Expr. 2017;7(1):143.
- Dayananda C, Sarada R, Rani U, et al. Autotrophic cultivation of Botryococcus braunii for the production of hydrocarbons and exopolysaccharides in various media. Biomass Bioenerg. 2007;31(1):87–93.
- Nakamura Y, Tanaka K, Matsumura Y, et al. Flow electrosynthesis and molecular weight control of polyphenylene deriving from 1,4-Bis(trimethylsilyl)benzene: effect of a silyl substituent on the coupling position, the electrochemical society of Japan. electrochemistry. in press; 2020. https://doi.org/https://doi.org/10.5796/electrochemistry.20-00060
- Trofimov BA, Myachina GF, Korzhova SA, et al. New electrochemically active highly sulfurized polyconjugated polymers. Russ J Electrochem. 2002;38(2):188–192.
- Revell JD, Dörner B, White PD, et al. PS-COD and PS-9-BBN: polymer-supported reagents for solution-phase parallel synthesis Sigma Aldrich. Org Lett. 2005;-2-25(PMID):15727452.
- Ganesan A. Solid-phase synthesis in the twenty-first century. Sigma Aldrich Mini Reviews in Medicinal Chemistry. 2006;-2-7(PMID):16457628.
- Ohno H, Takeoka Y, Miyamura K, et al. Novel synthesis of 3-Azabicyclo[3.1.0]hexanes by unusual Palladium(0)-catalyzed cyclopropanation of allenenes. Org Lett. 2003;5(25):4763–4766.
- Uehara K, Olson JM. Aggregation of bacteriochlorophyll c homologs to dimers, tetramers, and polymers in water-saturated carbon tetrachloride. Photosynth Res. 1992;33(3):251–257.
- Orf GS, Collins AM, Niedzwiedzki DM, et al. Polymer-chlorosome nanocomposites consisting of nonnative combinations of self-assembling bacteriochlorophylls Langmuir. 2017; 27; 33(25): 6427-6438.
- Herrero O, Planello R, Morcillo G. The plasticizer benzyl butyl phthalate (BBP) alters the ecdysone hormone pathway, the cellular response to stress, the energy metabolism, and several detoxication mechanisms in Chironomus riparius larvae. Chemosphere. 2015;128:266–277.
- Cerhr. Monograph on the potential human reproductive and developmental effects of Butyl Benzyl Phthalate (BBP). Bethesda: National Institutes of Health; 2003.
- CPSC. Toxicity review for Benzyl-n-Butyl Phthalate (Benzyl Butyl Phthalate or BBP). Bethesda: United States Consumer Product Safety Commission; 2010.
- EFSA (European Food Safety Authority). Opinion of the scientific panel on food additives, flavourings, processing aids and materials in contact with food (AFC) on a request from the commission related to Butylbenzylphthalate (BBP) for use in food contact materials. EFSA J. 2005b;241:1–14. Question No. EFSA-Q-2003-190.
- EFSA (European Food Safety Authority). Opinion of the scientific panel on food additives, flavourings, processing aids and materials in contact with food (AFC) on a request from the Commission related to Di-Butylphthalate (DBP) for use in food contact materials. EFSA J. 2005a;242:1–17. Question No. EFSA-Q-2003-192.
- Aylward LL, Hays SM, Gagne M, et al. Derivation of biomonitoring equivalents for di-n-butyl phthalate (DBP), benzylbutyl phthalate (BzBP) and diethyl phthalate (DEP). Regul Toxicol Pharmacol. 2009;55(3):259–267.
- Wormuth M, Scheringer M, Vollenweider M, et al. What are the sources of exposure to eight frequently used phthalic acid esters in Europeans? Risk Anal. 2006;26(3):803–824.
- Keipert S, Siebenbrodt I. Preparations of preoral sustained-release preparations with a biological polymer base. 5. Pharmazie. 1990 July;45(8):594–595. PMID: 2080206.
- Nter KGU, Lohmar E, Rupprich N, et al. Chloroacetic acids. Ullmann’s fine chemicals Wiley-VCH Verlag GmbH & Co. KGaA, Boschstr. 12, 69469. Weinheim Germany. 2014;2:473–488.
- SAAPedia 2011-2021. Cyclotrisiloxane, hexamethyl- SAAPedia all rights reserved. Update 20140828 (1);20200918 (2). https://www.surfactant.top/en/saa/?type=detail&id=1966
- Chojnowski J, Cypryk M, Kazmierski KO. Cationic polymerization of a model cyclotrisiloxane with mixed siloxane units initiated by a protic acid. mechanism of polymer chain formation. Macromolecules. 2002;35(27):9904–9912.
- Kendrick Bhukan TC, Parbhoo M, White JW. 25 - polymerization of cyclosiloxanes. Comprehensive Polymer Sci Supplements. 1989;4:459–523.
- Sukeshinia AM, Kulkarnia AR, Sharmab A. PEO based solid polymer electrolyte plasticized by dibutyl Phthalate. Solid State Ion. 1998;113–115(1–2):179–186.
- Sukeshini AM, Nishimoto A, Watanabe M. Transport and electrochemical characterization of plasticized poly(vinyl chloride) solid electrolytes. Solid State Ionics. 1996; 86-88(Part 1):385–393.
- Juan H, Ruihe L, Zhu J, et al. Selective solid-phase extraction of dibutyl phthalate from soybean milk using molecular imprinted polymers. Anal Chim Acta. 2010;661(2):215–221.
- Kang Y, Zhang L, Lai Q, et al. Molecularly imprinted polymer based on metal-organic frameworks: synthesis and application on determination of dibutyl phthalate. Polymer-Plastics Technol and Mater. 2020, 1-10. https://doi.org/https://doi.org/10.1080/25740881.2020.1786582
- Ramesh S, Yahaya AH, Arof AK. Dielectric behaviour of PVC-based polymer electrolytes. Solid State Ion. 2002;152– 153:291–294.
- Okada M, Fqjimoto K, Nose, et al. Nose phase separation induced by polymerization of 2-Chlorostyrene in a PolystyreneAIibutyl Phthalate mixture. Macromolecules. 1995;28(6):1795–1800.
- Xiaojiao L, Wang LL, Duan H, et al. Electro chemical sens or based on magnetic graphene oxide @ gold nanoparticles-molecular imprinted polymers for determination of dibutyl phthalate. Talanta. 2015, 131: 354–360.
- Kalberer M, Paulsen D, Sax M, et al. Identification of polymers as major components of atmospheric organic aerosols reports. Science. 2004;303(5664):1659–1662. www.sciencemag.org
- Xie F, Hu W, Ding L, et al. Synthesis of microporous organic polymers via radical polymerization of fumaronitrile with divinylbenzene. Polym Chem. 2017;8(39):6106–6111.
- De Oliveira DR, Mazzetto SE, Lomonaco D. Synthesis and polymerization of Naphthoxazines containing furan groups: an approach to novel biobased and flame-resistant thermosets Hindawi. Int J Polym Sci. 2018:1–13.
- Sheftel VO. 1-Hexadecanol indirect food additives and polymers. migration and toxicology. Boca Raton, FL: Lewis Publishers; 2000; 486.
- Wojcik A, Kubiak M, Rotsztejn H. Influence of azelaic and mandelic acid peels on sebum secretion in ageing women. Postep Derm Alergol. 2013;3:140–145.
- CAS RN. Screening assessment for the challenge Phenol, 2,6-bis(1,1-dimethylethyl)-4-(1-methylpropyl)- chemical abstracts service registry number. Environ Canada Health Canada. 2010; 17540-75-9.
- Godswill AC, Godspel AC. Physiological effects of plastic wastes on the endocrine system (Bisphenol A, Phthalates, Bisphenol S, PBDEs, TBBPA). Int J Bioinformatics Computational Biol. 2019;4(2):11–29.
- Kaller M, Basf SE, Koch M. Process for the production of carboxylic esters and use of these as plasticizers. United States patent. 2017;A1(9):776–949 B2.
- Lorz PM, Towae FK, Enke W, et al. Phthalic acid and derivatives. Wiley-VCH Verlag GmbH & Co. KGaA. Weinheim 2012; 12: 131–180. https://doi.org/https://doi.org/10.1002/14356007.a20_181.pub2
- APA Pyridine, 2-ethenyl-, polymer with 1,3-butadiene and ethenylbenzene National Center for Biotechnology Information. PubChem Compound Summary for CID 168262. 2021 Apr 12 from https://pubchem.ncbi.nlm.nih.gov/compound/168262.
- Hou C, Rongjun Q, Wang C, et al. Copolymerization kinetics of poly(acrylonitrile-ran-2- ethenyl-pyridine) and its degradation apparent activation energy. J Appl Polym Sci. 2008;107(4):2651–2655.
- Greehberg S, Inclair D. The polymerization of silicic acid., 1955. Vol. 59(5): 435–440.
- Goto K. Effect of pH on polymerization of silicic acid. J. Phys. Chem. Faculty of Engineering, Hokkaido University, Sappor, Japan, 1956; 60: 1007–1008.
- Bergman I, Sybil Nelson E. The polymerization of silicic acid and its subsequent interaction with proteins and other hydrogen-bonding agents. J Colloid Sci. 1962;17(9):823–837.
- Harold Wittcoff WC, Ferneuus. Benzene—the polymer former. J Chem Educ. 1981;58(3):270–272.
- Britannica 2013, The editors of Encyclopaedia. Aniline Encyclopedia Britannica, https://www.britannica.com/science/aniline. Accessed 9 April 2021
- Rohde A, Urland W. Synthesis crystal structure and magnetic behaviour of dimeric and polymeric gadolinium carboxylates with pentafluoropropionic acid. Inorganica Chim Acta. 2006;359(8):2448–2454.