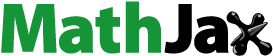
Abstract
In accordance with the State Program of the Russian Federation «Development of Industry and Its Competitiveness», as well as the absence of balance deposits of rare earth metals on the territory of the Russian Federation, it is urgent to study methods for the extraction and separation of rare earth metals from technological solutions obtained during the processing of apatite concentrates. The article is devoted to the study of the kinetic features of solid-phase extraction of samarium (III) and gadolinium (III) from simulated industrial phosphoric acid solutions. Levextrel resine (co-polymerization product of styrene and divinylbenzene) containing Di-2-ethylhexylphosphoric acid (D2EHPA) – was used as a solid-phase extraction agent. Significant dependence of samarium (III) and gadolinium (III) extraction rate constant on the stirring rate was revealed using the formal first-order kinetic equation. Based on the linear dependences of the logarithm of the reaction rate constant on the reciprocal temperature, the activation energies for the extraction of Gd and Sm from phosphate model solutions were established, which, respectively, were 42.47 and 28.48 kJ/mol. The obtained values of the activation energies indicate that the limiting stage of the extraction process on a solid extractant can be considered a chemical reaction between ions of rare-earth metals and functional groups of the extractant. Separation of elements at the extraction stage is possible at temperature of 333 K under non-equilibrium conditions with separation coefficient up to 1.8 in 5 min.
1. Introduction
Rare earth metals form a group of 17 elements, including scandium, yttrium, lanthanum, and the lanthanides (cerium, praseodymium, neodymium, promethium, samarium, europium, gadolinium, terbium, dysprosium, holmium, erbium, tulium, ytterbium, lutetium), while possessing similar chemical and physical properties (Binnemans, Jones, Muller, & Yurramendi, Citation2018). Rare earths metals (REM) are widely used in various high-tech industries due to their unique optical, electrical, and magnetic properties. Due to the development of industry, the share of the use of rare earth metals is increasing every year (Tunsu & Petranikova, Citation2018). In the Russian Federation, rare earth metals are extracted exclusively as a by-product during the processing of ores and the extraction of the main components from them due to their low content in the extracted ores. In terms of reserves of rare earth metals, Russia is firmly in second place in the world, giving priority to the People’s Republic of China (Guimei, Yong, Wendong, Raimund, & Zewen, Citation2023). In the Russian Federation, the state balance includes 26.9 tons of the amount of rare earth metals, with 70% of the reserves concentrated in the deposits of the Northwestern Federal District, 17% in the Far Eastern Federal District and 13% in the Siberian Federal District.
Mineral ores are still the main source of rare earth metals. In the Russian Federation, the state balance of mineral deposits includes 17 primary deposits, where rare earth metals can be isolated as a by-product during the processing of raw materials. Currently, only the Lovozero deposit of loparite ores is engaged in the associated extraction of rare earth metals, their concentration and further separation with the release of oxides of rare earth metals and their compounds, since this deposit contains about 25% of the reserves of rare earth metals in the Russian Federation.
An important aspect of the development of any technology is the choice of a sequence of optimal technological processes that reduce the economic costs of an enterprise when introducing stages for the extraction of rare earth metals and their further separation into the scheme (Litvinova, Citation2015; Wang et al., Citation2010).
The Khibinskoye apatite deposit located on the Kola Peninsula can be a promising source of rare earth metals. During the decomposition of apatite raw materials with concentrated sulfuric acid, two products are formed containing rare earth metals—phosphoric acid and a solid residue of phosphogypsum—the calcium salt of sulfuric acid.
Extractive phosphoric acid (EPA) contains about 20% of the total of rare earth metals and is a promising source for the extraction of rare earth metals. Attractive is the fact that the use of extraction phosphoric acid does not require a significant increase in production stages and associated capital costs. shows the relative content of oxides of rare earth metals in solutions of extraction phosphoric acids.
Table 1. The relative content of rare earth metal oxides in EPA solutions.
When using the volumes of extraction phosphoric acids existing in the Russian Federation, it is possible to obtain up to 4-5 thousand tons of rare earth metal oxides per year, which will help to significantly reduce the need for the purchase of rare earth metals from companies in other countries.
Taking into account the production of such high volumes of extraction phosphoric acids, one of the promising technologies for the extraction of Sm and Gd is the hydrometallurgical method, which includes the stages of the stage-by-stage separation of the sum of light, medium and heavy rare earth elements from the extraction phosphoric acid, followed by their isolation and separation using solid extractants. based on D2EHPA (Abu Elgoud, Ismail, El-Nadi, & Aly, Citation2020; Artiushenko, Ávila, Nazarkovsky, & Zaitsev, Citation2020).
In industrial practice, during the hydrometallurgical processing of raw materials, the isolation and concentration of rare earth metals occurs using the following methods:
Extraction extraction and separation of rare earth metals (main method) (Voropanova & Pukhova, Citation2018);
Separation of rare earth metals by the ion exchange method (Zhang, Ye, & Chen, Citation2012; Zheng, Bao, Zhang, & Chen, Citation2018).
A well-known factor complicating the implementation of solvent extraction is the use of flammable, volatile, and toxic organic solvents. The formation of interfacial emulsion can also be attributed to undesirable side effects of the solvent extraction process. The use of solid-phase extraction has a number of advantages compared to solvent extraction during the process of extracting the target component, such as compatibility with a continuous stream of acidic saline solutions, which can provide scalability of the process (Artiushenko et al., Citation2020). The disadvantage of this technology is the need for additional space and equipment to ensure the regeneration of materials used as solid carriers for reuse (Akkaya, Citation2014).
Currently, solid extractants systems (SES) are promising products for the separation of rare earth metals (Babu, Binnemans, & Roosen, Citation2018; Bao, Tang, Zhang, & Liang, Citation2016). Solid state extractants are a product of suspension copolymerization of styrene and divinylbenzene and an extractant (TBP, D2EHPA, as well as any other organophosphorus extractants in a total volume of up to 70%) (Razieh, Rezvan, & Mehdi, Citation2022). Compared to liquid extraction in SES, the extractant is in a droplet-liquid state and has improved kinetic characteristics (Hérès et al., Citation2018; Kabay, Cortina, Trochimczuk, & Streat, Citation2010; Valenzuela et al., Citation2012).
The absence of physicochemical characteristics of solid-phase extraction of REE by D2EHPA-containing extraction resins from concentrated phosphoric acid solutions and the necessity to confirm technological advantages of their use determined the subject of this study. The study of the kinetic features of solvent and solid-phase extraction of Gd (III) and Sm (III) using Levextrel resin containing groups of di-2-ethylhexylphosphoric acid, which is a selective extractant with relation to REE, is the subject of this research (Kaibo et al., Citation2022).
2. Research methods
Experimental studies on the sorption extraction of Sm and Gd were carried out from phosphate solutions simulating in their composition the solutions released after the leaching of apatite concentrate. Model acid leach solution consisted of: orthophosphoric acid (CH3PO4 = 0.5 M); sulfuric acid (CH2SO4 = 0.19 M); gadolinium nitrate (CGd = 0.00704 M) and samarium nitrate (CSm = 0.00989 M) (Wang et al., Citation2010).
To study the processes of extraction of Gd and Sm, SES—D2EHPA was used as a solid extractant (Kabay et al., Citation2010). This solid extractant is a macroporous resin with an extractant fixed by adsorption. This sorbent is used to extract metal ions from acidic solutions. The working temperature of the sorbent is no more than 80 0C, the bulk density is 600 g/dm3, the working range of pH values is 1.0–4.0.
The process of solid-phase extraction was studied under static conditions at a phase ratio L: S = 1:10. The process was carried out in a thermostatic system with an oscillation rate of 75 rpm. The mass of the solid extractant was 1.0000 ± 0.0005 g. The stirring time for each sample was 5, 15, 30, 45, 60, and 120 minutes. Experimental dependences were obtained at 298 K, 308 K, 318 K, and 333 K (Callura et al., Citation2019; Cheremisina, Schenk, Cheremisina, & Ponomareva, Citation2019). After reaching the specified contact time, the aqueous phase was separated from the solid extractant using a Schott filter with a porosity of 160 μm. The extractant was sent for regeneration, and the equilibrium solutions were analyzed for the content of metal ions by an X-ray fluorescence method using an energy-dispersive X-ray fluorescence spectrometer PANalytical Epsilon 3 (Cheremisina, Sergeev, Fedorov, & Iliyna, Citation2019; Praveenkumar, Citation2021).
From the point of view of formal kinetics, such extraction processes can be represented as a first-order kinetic reaction (Li, Citation2019; Lutskiy, Ignatovich, & Sulimova, Citation2019). In first-order reactions, the reaction rate is related to the rate of change in the concentration of substance A by EquationEquation (1)(1)
(1) :
(1)
(1)
where C0 is the initial concentration of the starting material, mol/l; C is the concentration of the starting substance, mol/l by the time τ, min, k is the reaction rate constant, min−1.
To characterize the reaction rate, along with the rate constant, the half-transformation time (τ1/2) is often used—the time during which half of the initial amount of the substance will react. For first-order reactions, the half-life is defined as:
(2)
(2)
To determine the half-extraction time and rate constants of the processes of extraction of Sm and Gd ions using the solid-phase extractant SES—D2EHPA, kinetic dependences were obtained, presented in and and in and .
Figure 1. Kinetic dependence of the degree of extraction of Gd in a solid extractant at different temperatures.

Figure 2. Kinetic dependence of the degree of extraction of samarium in a solid extractant at different temperatures.

Table 2. The degree of extraction of gadolinium into a solid extractant at different temperatures.
Table 3. Sm recovery into solid extractant at different temperatures.
3. Results and discussion
The experimental results showing the degree of gadolinium extraction in SES-D2EHPA at temperatures of 298 K, 308 K, 318 K, 333 K, depending on the time of contact of the phases, are presented in and in .
shows the dependences of the degree of extraction of Gd into SES—D2EHPA.
As can be seen from the data obtained, the dependence of the effect of temperature on the extraction of metal in SES is traced, reaching its maximum value by 120 minutes. However, an increase in temperature from 318 to 333 K gives a very insignificant increase in the degree of extraction of gadolinium into the sorbent, while increasing the power consumption required to maintain a constant temperature of the solution. The optimal process can be considered as taking place at 318 K and 120 minutes.
The experimental results showing the degree of samarium extraction in SES—D2EHPA at 298 K, 308 K, 318 K, 333 K depending on the time of contact of the phases are presented in .
shows the dependences of the degree of extraction of Sm into SES—D2EHPA.
As can be seen from the data presented in , the phase contact time also affects the degree of Sm extraction into the sorbent, and an increase in temperature from 318 K to 333 K with a phase contact time of 120 minutes is insignificant, although at a temperature of 333 K and a phase contact time of 60 minutes we obtain the same degree of Sm extraction as at 318 K and the phase contact time of 120 minutes.
To obtain the kinetic characteristics of the process for the isotherms presented in and , linear forms of the kinetic dependences shown in and were constructed.
Figure 3. Dependence of the logarithm of the concentration of Gd ions in the aqueous phase on the time of the process when using the solid extractant SES—D2EHPA at different temperatures.

Figure 4. Dependence of the logarithm of the concentration of Sm ions in the aqueous phase on the time of the process when using the solid extractant SES—D2EHPA at different temperatures.

The linear forms of the equations for approximating the extraction isotherms presented in and are presented in .
Table 4. Linear forms of equations for approximation of isotherms for extraction of Gd ions using solid extractant SES—D2EHPA.
From the linear forms of the kinetic dependencies presented in , the values of the rate constants of liquid extraction (k) and the half-extraction time (τ1/2) presented in were obtained.
Table 5. Values of the rate constants for liquid extraction (k) and the half-extraction time (τ1/2) of gadolinium and samarium using the solid extractant SES—D2EHPA.
The processing of the dependences of the reaction rate constant on temperature, shown in , makes it possible to determine the activation energies of the reaction for the extraction of Sm and Gd.
Figure 5. Linear forms of the dependences of the reaction rate constant on temperature during the extraction of Gd (a) and Sm (b) using a solid extractant SES—D2EHPA.

The linear dependences of the logarithm of the reaction rate constant on the reciprocal temperature is described by empirical equations for the slope coefficients of which the values of activation energies are set, presented in .
Table 6. Equations of linear dependences of the logarithm of the reaction rate constant on the reciprocal temperature and activation energy of the reactions of extraction of Gd and Sm using the solid extractant SES—D2EHPA.
The obtained values of the activation energies indicate that the limiting stage of the extraction process on a solid extractant can be considered a chemical reaction between ions of rare-earth metals and functional groups of the extractant.
4. Conclusions
In this work, the kinetic dependences of the extraction of Gd and Sm from phosphate solutions were established, simulating in their composition the solutions released after leaching the apatite concentrate using the solid extractant SES-D2EHPA. The efficiency of this extractant for the extraction of Gd and Sm from phosphate media is shown. Isotherms of extraction of Gd and Sm from a model phosphate solution from PJSC PhosAgro (Balakovo Branch of Apatit, Saratov Region, Balakovo District, Russia) as a product of apatite processing were obtained.
The effect of temperature on the degree of extraction of Gd and Sm from model solutions is shown. For a number of temperatures, the values of the extraction rate constants (k) and the half-extraction time of Gd and Sm (τ1/2) were determined.
Experimental data obtained indicate differences of Gd and Sm extraction kinetics from a phosphoric acid medium. In case of Gd, the process is limited by a chemical reaction with an activation energy of 42.47 kJ/mol. In case of Sm, diffusion becomes the limiting stage, characterized by a decrease in activation energy to 28.4 kJ/mol. The diffusion process determines the rate Sm extraction over a wide temperature range. Based on the revealed differences in the element extraction kinetics, it is possible to carry out their separation at the extraction stage. Separation coefficient of 1.8 between Gd and Sm is ensured by the short phase contact time (up to 5 min). Solid extractants have an undoubted technological advantage. Such resins are characterized by high mechanical strength, high chemical stability in aqueous solutions of acids and alkalis, and can be used in coarse systems and bulk filters. The absence of significant amounts of flammable solvents, which accompany liquid extraction, complies with the principles of green chemistry.
According to the values of the rate constants of ion-exchange adsorption and the half-exchange time, it is shown that the use of solid extractant SES-D2EHPA for the extraction of Gd and Sm ions from phosphate solutions is promising in comparison with its analogues.
Disclosure statement
The authors declare no conflict of interest.
Data availability statement
The datasets generated during and/or analyzed during the current study are available from the corresponding author upon reasonable request.
References
- Abu Elgoud, E. M., Ismail, Z. H., El-Nadi, Y. A., & Aly, H. F. (2020). Separation of Cerium (IV) and Yttrium (III) from citrate medium by solvent extraction using D2EHPA in kerosene. Chemical Papers, 74(8), 2461–2469. doi:https://doi.org/10.1007/s11696-020-01083-8
- Akkaya, R. (2014). Terbium adsorption onto polyhydroxyethylmethacrylate–hydroxyapatite composite and its modified composition by phytic acid. Desalination and Water Treatment. 52(7-9), 1440–1447. doi:10.1080/19443994.2013.793922
- Artiushenko, O., Ávila, E. P., Nazarkovsky, M., & Zaitsev, V. (2020). Reusable hydroxamate immobilized silica adsorbent for dispersive solid phase extraction and separation of rare earth metal ions. Separation and Purification Technology. 231, 115934. doi:10.1016/j.seppur.2019.115934
- Babu, C. M., Binnemans, K., & Roosen, J. (2018). Ethylenediaminetriacetic acid-functionalized activated carbon for the adsorption of rare earths from aqueous solutions. Industrial & Engineering Chemistry Research, 57(5), 1487–1497. doi:10.1021/acs.iecr.7b04274
- Bao, S., Tang, Y., Zhang, Y., & Liang, L. (2016). Recovery and separation of metal ions from aqueous solutions by solvent-impregnated resins. Chemical Engineering & Technology, 39(8), 1377–1392. doi:10.1002/ceat.201500324
- Binnemans, K., Jones, P., Muller, T., & Yurramendi, L. (2018). Rare earths and the balance problem: How to deal with changing markets? Journal of Sustainable Metallurgy, 4(1), 126–146. doi:10.1007/s40831-018-0162-8
- Callura, J. C., Perkins, K. M., Baltrus, J. P., Washburn, N. R., Dzombak, D. A., & Karamalidis, A. K. (2019). Adsorption kinetics, thermodynamics, and isotherm studies for functionalized lanthanide-chelating resins. Journal of Colloid and Interface Science, 557, 465–477. doi:10.1016/j.jcis.2019.08.097
- Cheremisina, O. V., Schenk, J., Cheremisina, E. A., & Ponomareva, M. A. (2019). Thermodynamic model of ion-exchange process as exemplified by cerium sorption from multisalt solutions. Journal of Mining Institute, 237(3), 307–316. doi:10.31897/pmi.2019.3.307
- Cheremisina, O., Sergeev, V., Fedorov, A., & Iliyna, A. (2019). Specific features of solvent extraction of REM from phosphoric acid solutions with DEHPA. Mineral Processing and Extractive Metallurgy Review, 130, 233–239. doi:10.1080/25726641.2019.1626658
- Guimei, Z., Yong, G., Wendong, W., Raimund, B., & Zewen, G. (2023). Assessing gadolinium resource efficiency and criticality in China. Resources Policy, 80, 103137. doi:10.1016/j.resourpol.2022.103137
- Hérès, X., Blet, V., Di Natale, P., Ouaattou, A., Mazouz, H., Dhiba, D., & Cuer, F. (2018). Selective extraction of rare earth elements from phosphoric acid by ion exchange resins. Metals, 8(9), 682. doi:10.3390/met8090682
- Kabay, N., Cortina, J. L., Trochimczuk, A., & Streat, M. (2010). Solvent-impregnated resins (SIRs)—Methods of preparation and their applications. Reactive and Functional Polymers. 70(8), 484–496. doi:10.1016/j.reactfunctpolym.2010.01.005
- Kaibo, H., Lu, X., Yi, N., Xuewei, L., Haifeng, D., & Hongshuai, G. (2022). Removal of aluminum to obtain high purity gadolinium with pyridinium-based ionic liquids. Hydrometallurgy, 213, 105930. doi:10.1016/j.hydromet.2022.105930
- Li, D. (2019). Development course of separating rare earths with acid phosphorus extractants: A critical review. J. Rare Earths, 37(5), 468–486. doi:10.1016/j.jre.2018.07.016
- Litvinova, T. (2015). Separation of the heavy and light rare earth metals concentrate after sulfuric acid eudialyte concentrate treatment. FOG Freiberg Online Geoscience, 40, 159–166.
- Lutskiy, D. S., Ignatovich, A. S., & Sulimova, M. A. (2019). Determination of the sorption characteristics of ammonium perrenate ions on anion exchange resin AV-17-8. Journal of Physics: Conference Series, 1399(5), 055069. doi:10.1088/1742-6596/1399/5/055069
- Praveenkumar, S. (2021). Molecular simulation of separation of gadolinium ions from aqueous waste using directional solvent extraction. Journal of Molecular Liquids, 341, 117330. doi:10.1016/j.molliq.2021.117330
- Razieh, S. A., Rezvan, T., & Mehdi, A. (2022). Evaluation of effective parameters on the non-aqueous solvent extraction of samarium and gadolinium to n-dodecane/D2EHPA. Progress in Nuclear Energy, 144, 104072. doi:10.1016/j.pnucene.2021.104072
- Tunsu, C., & Petranikova, M. (2018). Perspectives for the recovery of critical elements from future energy-efficient refrigeration materials. Journal of Cleaner Production, 197, 232–241. doi:10.1016/j.jclepro.2018.06.185
- Valenzuela, F., Valdés, A., Ide, V., Basualto, C., Sapag, J., & Araneda, C. (2012). Equilibrium, kinetic, and thermodynamic analysis of Cd (II) sorption from aqueous solutions using polymeric microcapsules containing an acidic organophosphonic extractant. Solvent Extraction and Ion Exchange. 30(4), 422–430. doi:10.1080/07366299.2012.687180
- Voropanova, L. A., & Pukhova, V. P. (2018). Extraction of copper, cobalt and nickel ions from aqueous solutions by extractant Cyanex 272. Journal of Mining Institute, 233, 498–505. doi:10.31897/PMI.2018.5.498
- Wang, L., Long, Z., Huang, X., Yu, Y., Cui, D., & Zhang, G. (2010). Recovery of rare earths from wet-process phosphoric acid. Hydrometallurgy, 101(1-2), 41–47. doi:10.1016/j.hydromet.2009.11.017
- Zhang, W., Ye, G., & Chen, J. (2012). TRPO impregnated levextrel resin: Synthesis and extraction behavior of Zr (IV) and Nd (III) ions. Separation Science and Technology, 48(2), 263–271. doi:10.1080/01496395.2012.675002
- Zheng, R., Bao, S., Zhang, Y., & Chen, B. (2018). Synthesis of di-(2-ethylhexyl) phosphoric acid (D2EHPA)-tributyl phosphate (TBP) impregnated resin and application in adsorption of vanadium (IV). Minerals, 8(5), 206. doi:10.3390/min8050206