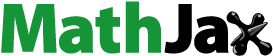
Abstract
Discovering new processing routes to put waste to use in the manufacturing of high-value products is essential to the success of the circular economy. A glass polishing waste containing 16.4% PbO, 0.672% ZnO and 7.296% rare earth elements (REEs) oxides was subjected to this work. The current research looked into the feasibility of adopting a sequential three-stage leaching procedure to selectively recover Pb, Zn, and REEs from hazardous glass polishing waste (HGPW). First-stage leaching with 1 M H2SO4 at 80 °C for 90 min extracted 75–99.9% of contaminant heavy metals such Fe, Ni, Co, and Cu, leaving behind insoluble residue containing the metals of interest. In the second stage of leaching, the sodium hydroxide solution extracted Pb and Zn from the first spend residue. Using shrinking core models, we were able to assess the kinetics of lead and zinc dissolution. The activation energy was determined to be 32.16 kJ/mol for Pb and 34.59 kJ/mol for Zn. In the final step, the REEs were extracted from the dried alkali-treated residue by leaching it with concentrated H2SO4 at higher temperatures. For the technological recovery of Pb, Zn, and REEs from glass polishing waste, a flow chart was created.
1. Introduction
Glass polishing sludge comprises rare earth elements (REEs), lead, zinc, iron, aluminum, sodium, silicon, and other elements (Adhikary, Ashish, & Rudžionis, Citation2021; Graziano et al., Citation2022; Li et al., Citation2023a; Li, Zhang, Luo, & Xie, Citation2023b; Pascual, Tognonvi, & Tagnit-Hamou, Citation2021). In glass surface finishing, metal oxides powders (CeO2, Fe2O3, La2O3, PbO, ZnO, TiO2, Al2O3, etc.) (Bao et al., Citation2020; Borra et al., Citation2019; Borra et al., Citation2021; Kim et al., Citation2011; Wu, Song, Zhang, & Wang, Citation2021) are known as useful polishing agents that achieve the desired surface finish and optical quality of glass products. Lead, zinc, and rare earth elements (REEs) are commonly added as additives due to their specific roles in glass polishing.
Lead oxide (PbO), is widely utilized in glass polishing due to its ability to enhance the polishing performance. Lead acts as a lubricant and abrasive, facilitating the removal of imperfections and irregularities on the glass surface. Its presence in the polishing agent helps to achieve a smoother and more uniform surface finish. While, Zinc oxide (ZnO), also plays a significant role in glass polishing. Zinc functions as a fluxing agent, reducing the melting temperature of glass and promoting the removal of fine scratches and blemishes during the polishing process. Additionally, zinc aids in controlling the viscosity of the polishing slurry, ensuring optimal polishing conditions. On the other hand, REEs, including elements such as cerium (Ce), and lanthanum (La), are employed in glass polishing for their unique properties. REEs exhibit excellent chemical reactivity and catalytic activity, enabling them to participate in the chemical reactions involved in the polishing process. They contribute to the removal of surface defects, smoothing of glass surfaces, and improving the overall optical quality of the glass. However, these polishing powders gradually lose their polishing ability after a finite number of polishing processes, and consequently generate a large amount of waste slurries enriched with cerium, lanthanum, and various elements. After polishing, the leftover slurry is delivered to a flocculation process, where the solids can be separated from the liquid using a filter press. The compacted residue from the filtration process is commonly discarded in dumping sites (Poscher, Luidold, Schnideritsch, & Antrekowitsch, Citation2016). In turn, this causes precious REEs and metals to be lost (Pb and Zn). Moreover, some expenses are associated with the disposal of glass polishing waste. Glass polishing uses rare earth oxides (REOs) as Ce, La, and Y oxides because of their chemical and mechanical qualities (Janos et al., Citation2014). The glass industry uses around 40,000 t of REEs oxides each year, with around 16,000 t going toward polishing (Tercero Espinoza et al., Citation2015). During the polishing process and the subsequent settling operations, the glass polishing powder accumulates SiO2 and other contaminants (Tercero Espinoza et al., 2015). In addition, both lead and zinc are essential elements in several products, including batteries, solder, dielectrics, piezoelectrics, glasses, etc. However, it has been known for a long time that lead is highly poisonous even at very low doses, while Zn are essential micronutrients that are toxic at high concentrations (Hou et al., Citation2022; Li et al., Citation2023a; Li et al., Citation2023b). However, lead glass is widely employed as a shield against radioactive radiation, in cathode ray tubes (CRTs), and as an aesthetic component of buildings.
An effective approach towards a circular economy involves the retrieval of valuable metals such as (REEs, Pb, and Zn) from polishing waste and their utilization in high-value applications or back into the polishing process. This aims to minimize waste, promote the efficient use of resources, and maximize the reuse, recycling, and recovery of materials. The process of extracting valuable metals can reintroduce them into the production cycle. This approach reduces the reliance on virgin resources and minimizes the environmental impact associated with their extraction and processing. The recovered metals can be used as raw materials for various industries, including the manufacturing of new products. By incorporating these metals back into the production cycle, resource efficiency is promoted, reducing the need for primary mining and minimizing waste generation. This strategy ensures that the metals loops are closed, resulting in a sustainable and efficient use of these resources (Bao et al., Citation2020; Lee et al., Citation2019; Yu, Jiang, & Yang, Citation2019). The recycling of polishing waste enables the retrieval of significant metals such as (REEs), lead, and zinc, and promotes the optimal utilization of these valuable resources. The energy required for metal recovery and recycling is typically lower than that of initial production from an ore, and the environmental impact is typically smaller as well (Kumar et al., Citation2014). Furthermore, costs associated with disposal are minimized. Extraction of (REEs) from polishing powder has been the subject of numerous research efforts (Bao et al., Citation2020; Lee et al., Citation2019; Magnago et al., Citation2021; Wu et al., Citation2021). Normally, the procedure involves acid leaching, where ceria necessitates elevated levels of acid concentration and temperature for its dissolution due to its stable nature. Some rare earths, like rare earth fluorides and phosphates, can be recovered from leach waste by employing a recently developed alkali roasting and leaching process (Borra et al., Citation2019). However, HCl was used for leaching the alkali roasted residue and polishing trash, which results in the release of toxic chlorine gas. Recovery of (REEs) from polishing sludge has been the subject of extensive research (Lee et al., Citation2019; Wu et al., Citation2021). Furthermore, the hydrometallurgical approach was the subject of an extensive study with the goal of improving the metals’ recovery rates. These researches (Abdel-Aal, Citation2000; Espiari et al., Citation2006; Ruixiang et al., Citation2008) have employed inorganic acids as leach reagents. In addition, alkaline ore and waste treatment have received considerable study (Chen et al., Citation2009; Ghasemi & Azizi, Citation2018; Santos et al., Citation2010; Zhang et al., Citation2015). In most cases, alkaline leaching is more selective in its effects. Because contaminants, like Fe, Cu, Cd, Co, Ni, etc., are too difficult to leach out in alkaline media, the electrowinning process for zinc and lead is generally regarded as more cost-effective, simpler, and more straightforward to operate and maintain than acidic electrowinning (Cuixiang, Long, Qing, & Youcai, Citation2007; Cuixiang & Youcai, Citation2008; Youcai & Stanforth, Citation2001). Alkaline leaching of lead and zinc from waste materials was the subject of this investigation. In this research, we use shrinking core models to analyze dissolution kinetics and select the most appropriate kinetics model to characterize the leaching of lead and zinc.
New processing methods must be developed to enable the recycling of garbage into high-value products as part of the circular economy. The research team used a three-stage sequential leaching procedure to selectively recover rare earth oxides, zinc, and lead from glass polishing waste. Next, we select the most appropriate kinetics model to characterize the leaching process by analyzing the dissolving kinetics in light of the shrinking core models.
2. Materials and methods
2.1. Materials
Asfour Crystal Company in Cairo, Egypt, supplied us with the hazardous glass polishing waste (HGPW) that is produced during the chemical polishing process in the glass crystal sector. To prepare the sample, it underwent a series of processes including crushing, grinding, and sieving according to the American Society for Testing and Materials (ASTM) standard. The sieving process resulted in a size of −75 μm for the sample. provides a concise overview of the raw materials’ chemical composition, which was verified using an ESEM (Philips XL30 model) and is depicted in . It is worth noting that Y(III), La(III), and Ce(III) were chosen to serve as representatives of the three distinct categories of Rare Earth Elements (REEs), namely light, medium, and heavy REEs.
Table 1. The chemical constitutions of the hazardous glass polishing waste (HGPW) material.
In , the amorphous glassy component of HGPW is seen in the XRD pattern. Phase detection in XRD diffractograms was corroborated by reference codes for quartz (01-086-2339) and mullite (01-079-1276). Sulphuric acid (98%) from Chem-lab, sodium hydroxide (Sigma Aldrich), sodium sulphide (Sigma Aldrich), and oxalic acid (Chem-lab) were all employed in this analysis. Energy dispersive X-ray fluorescence (EDXRF, USA Rigaku model NEX CG) and Inductively Coupled Plasma Optical Emission Spectroscopy (ICP-OES, USA Teledyne technologies) were used for the chemical analysis.
2.2. Sequential leaching studies
To conduct the leaching tests, a vibratory shaker (VWR Thermoshake) was utilized, working at a speed of 300 rpm. Subsequently, the resulting leach solution underwent filtration and was further diluted with distilled water for analysis through (ICP-OES).
2.2.1. First-stage acid leaching
A first round of leaching was performed with diluted H2SO4. Experiments were conducted with varying acid concentrations (0.25, 0.50, 0.75, 1.00 and 1.50), liquid-to-solid ratios (8/1 to 10/1), temperatures (25, 45, 65, 80, and 90 °C), and reaction times (30–150 min). To get ready for ICP-OES analysis, the solution obtained from the leaching process was filtered and thinned out using distilled water. The remaining solid residue from the leaching process was then dehydrated at 105 °C for 3 h.
2.2.2. Second-stage leaching process for Pb and Zn recovery
For the purpose of extracting any lingering heavy metals (Pb and Zn), the initially dried residue from traditional acid leaching was combined with NaOH at a concentration between 0.5 and 5 M to serve as the leaching reagent. The initial dried residue was combined with a NaOH solution, and the blend was warmed gradually from room temperature to designated temperatures (50, 60, 70, and 80 °C) for a specific duration of time (30–90 min). After filtering the mixture to obtain the experimental sample, the concentration of metals present in the filtrate was determined via ICP-OES analysis. The remaining solid residue from the secondary leaching process was then dehydrated at 105 °C for 3 h.
2.2.3. Third-stage leaching process for total REEs (Ce, La, and Y) recovery
Following the alkali treatment, the dehydrated residue underwent a leaching process using concentrated H2SO4, with a liquid-to-solid ratio of 3:1. The leaching process was conducted for a duration of 3 h, within a temperature range of 300 °C to 330 °C. (Borra et al., Citation2021; Mohamed, Guirguis, Orabi, & Khalil, Citation2019; Orabi, Rabia, Elshereafy, & Salem, Citation2017; Orabi, Mohamed, Ismaiel, & Elyan, Citation2021). Following digestion, a thick grey paste was produced, which cooled while water diluted the residue by at least 20 times its weight over the course of at least 2 h. The temperature must be cooled in order not to exceed 17 °C; when the temperature drops, rare earth sulphates become more soluble. To selectively precipitate (REEs), oxalic acid was introduced to the filtrate at a mole ratio of 1:2 (REEs to oxalic acid). The rare earth oxalate, which was formed as a result, was filtered, extensively washed with demineralized water, and then subjected to calcination at 850 °C for a period of 1 h, resulting in the production of rare earth oxides. The proper personal protective equipment was used during the process. Furthermore, the plant design was optimized for worker safety. The design has been optimized by allowing extra space in the equipment to prevent splashing and having sturdy construction materials that can handle high corrosivity levels.
3. Results and discussion
3.1. Sequential leaching studies
The primary chemical components of the hazardous glass polishing waste (HGPW) substance used in the operation are shown in . Metal contents in the research material were determined to be as follows: Pb in HGPW was 152.25 g/Kg, Zn in HGPW was 5.39 g/Kg, and REEs in HGPW was 60.25 g/Kg, with high concentrations of impurity elements such Ni, Co, Cu, and Fe. The waste from the process of polishing glass needs intense conditions (higher concentration of leaching agents and higher temperature) for recycling and recovery of the valuable elements (specially REEs) again and this was agreed with the earlier papers (Bao et al., Citation2020; Lee et al., Citation2019; Magnago et al., Citation2021; Wu et al., Citation2021). Metal oxide powders react with SiO2 surface during polishing process, resulting in the formation of metal–O–Si bonds (Luo et al., Citation2020; Oh, Nho, Cho, Lee, & Singh, Citation2011). Glass polishing powder is accumulated with other impurities during polishing and in subsequent settling processes (Tercero Espinoza et al., 2015) as shown in the analysis of glass polishing waste (). So, the impurity elements must be removed firstly using dilute acid before recovery of valuable elements (REEs, Zn, and Pb) in order not to affect the purity of the studied metals. The components of interest and the unwanted species are both dissolved in the solution when using traditional procedures; hence, another method is needed to separate them as follows.
3.1.1. First-stage acid leaching
Dilute sulphuric acid was used for leaching heavy metal impurities as Ni, Co, Cu, and Fe before recovery of valuable elements (REEs, Zn, and Pb) in order not to affect the purity of the studied metals. Concentrations of 0.25, 0.50, 0.75, 1.00 and 1.50 M of sulphuric acid were tested to see how they affected leaching; the findings are depicted in . The efficiency with which metals were leached increased as the acid concentration rose. A high equivalent concentration (normality) of hydrogen ions (H+) of H2SO4 solution, representing the reactive capacity of H+ for metal dissolution, may account for sulphuric acid’s potency as a leaching agent for heavy metals (Larsson, Ekberg, & Ødegaard-Jensen, Citation2013; Zielinski et al., Citation2020). As the concentration of the solution was increased from 0.25 M to 1.5 M, the leaching efficiency of the heavy metals rose from 20.45% to 99.90% for Ni, 16.68–96% for Co, 55–75% for Fe, and 16.10–96% for Cu. Considering the leaching efficiency and selectivity, 1 M of H2SO4 was chosen for further experiments. The reason for lead and zinc retention in the residue is due to their low solubility, where lead exists as lead sulphate in an acid medium (H2SO4) or in water that hinder its dissolution (Ruşen et al., Citation2008). Similar observations were noted by Liu et al. (Citation2017) and Dimitrijevic, Urosevic, Jankovic, and Milic (Citation2016). Zinc, on the other hand, react with SiO2 surface during polishing process, resulting in the formation of metal–O–Si bonds (Luo et al., Citation2020; Oh et al., Citation2011). So, we have a low-grade zinc oxide with high silicon in the polished waste materials that needs higher acid concentration with higher temperature for Zn recovery (Zhang et al., Citation2016). In the leaching of this type of material such as hemimorphite produce a gel during leaching with sulphuric acid. a gelatinous layer was formed around the reacting core and always covers the reacting core which increases the mass transfer resistance and hinder its dissolution (Safari, Arzpeyma, Rashchi, & Mostoufi, Citation2009). This was agreed with the earlier papers of Zn recovery from a low-grade zinc oxide ore with high silicon by sulphuric acid (Safari et al., Citation2009; Zhang et al., Citation2016). In addition, the studied sample represented low ZnO with a high contamination of other elements. An insoluble spinel phase traps the leaching of zinc in the contaminated zinc oxide samples. 1.32% CaO is found in the studied waste sample and reacts with sulphuric acid to form CaSO4 precipitate in pores and around the edges of Zn grains. Also, REEs were retained in the residue with Zn and Pb, because they need high acid concentration and high temperature. This statement holds particularly true in the case of (REEs) oxides that are produced through annealing at high temperatures, as this process is essential for their application as polishing powders (Bao et al., Citation2020; Lee et al., Citation2019; Magnago et al., Citation2021; Wu et al., Citation2021).
The findings of a study into the effect of L/S using 1 M of H2SO4 at 80 °C, agitated at 100 rpm for 90 min, are displayed in . As the S/L ratio grew from 8/1 to 10/1, the impurity metals leaching efficiency increased from 89.33% to 99% for Ni, 73.5–95% for Co, 65.4–75% for Fe, and 71.5–92% for Cu, and did not alter further with additional increases in S/L ratio. A comparison of the XRF patterns of HGPW before and after leaching with H2SO4 at 80 °C for 90 min reveals both the acid soluble and insoluble elements present in the waste (). The XRF analysis revealed that after the waste was leached, by H2SO4, only the peaks for the metals Pb, Zn, and REEs remained, whereas the peaks for the acid-soluble salts were gone. Leaching studies showed that 1 M H2SO4 was able to remove over 75% of the Fe and over 90% of the Co, Ni, and Cu from glass polishing waste.
Figure 3. XRF spectra of the HGPW before leaching (a) and the leaching residues remaining after sulphuric acid (b) was applied.

Using 1 M H2SO4 and L/S of 10/1 at 80 °C, the leaching reaction rate of metals from HGPW was investigated; the findings are depicted in . Ni, Co, Fe, and Cu leaching efficiency rose throughout time and stabilized at around 120 min. The dissolution of metal complexes, which consumed H+, was responsible for the pH value rising from −0.3 to 1.69 after 120 min.
Dissolution of impurity metals (Ni, Co, Cu and Fe) from HGPW material as a function of temperature (25–95 °C) and 10/1 (L/S) phase ratio using 1 M sulphuric acid are represented in . From 25 °C to 80 °C, the leaching efficiency of impurity metals increased from 49% to 99% for Ni, 73.5–95% for Co, 50.3–75% for Fe, and 50.4–92% for Cu, and then it levelled out. Based on the results of the aforementioned acidic atmospheric agitation leaching investigation, it was determined that the following optimum leaching conditions are sufficient for the dissolution of Ni (99%), Co (95%), Cu (92%), and Fe (75%) from the sample HGPW material: 90 min at 80 °C in sulphuric acid (1 M), and 10/1 L/S. It is worth noting that if the optimum leaching conditions were applied to the study raw material, the impurity metals (Ni, Co, Cu, and Fe) would be separated from the Pb, Zn, and REEs that would remain in the residue. The valuable metals that remained in this residue () would undergo the second leaching process as follows.
Table 2. The chemical constitutions of the first spend residue.
3.1.2. Second-stage leaching process for Pb and Zn recovery
As much as 60.25 g/Kg REEs, 5.39 g/Kg Zn, and 152.25 g/Kg Pb remained in the first wasted residue after treatment with 1 M sulphuric acid. Intense conditions are required since these metals are stable and resistant to leaching with dilute acid, as was previously mentioned. Stage two involved alkaline leaching in caustic soda solution, which is commonly employed for zinc and lead extraction from ores and wastes (Chen et al., Citation2009; Cuixiang et al., Citation2007; Ghasemi & Azizi, Citation2018; Zhang et al., Citation2015). Ba, Ca, Mg, Fe, etc., will not dissolve in the alkaline solution used in this method. We looked at how different amounts of NaOH, leaching times, and temperatures affected the process. The leaching efficiency of zinc and lead from the remaining glass polishing waste was found to be significantly impacted by NaOH concentration (). shows the effects of varying NaOH concentrations (0.5 M to 5 M) on the leaching efficiency of Zn and Pb from residual glass polishing waste as a function of contact time (30 to 90 min) at a liquids-to-solids (L/S) ratio of 20/1 and a temperature of 70 °C. These studies indicate that zinc and lead leaching efficiency can be improved by increasing NaOH content from 0.5 to 4 M. A large proportion of the breakdown occurred during the second time period (60–90 min). It is sufficient to create the more soluble Zn(OH)42− and Pb(OH)42− when the NaOH solution concentration reaches 4 M (Liu, Zhao, & Zhao, Citation2011) and therefore the leaching rate could be over 80% for zinc and 63% for lead. If the NaOH content in the leaching solution is increased to >4 M, the resulting solutions may be too viscous to facilitate phase separation (Zhang et al., Citation2015). First-stage leaching residue can be leached of Pb and Zn using 4 M NaOH.
Figure 4. Effect of the NaOH concentration on the efficiency with which lead (a) and zinc (b) were leached from first residual glass polishing waste at 70 °C and L/S ratio 20/1.

depicts the effect of varying the volume of aqueous NaOH solution used in the leaching process. depicts the variations in L/S ratio (10/1–25/1) as a function of contact duration (30–90 min) at a temperature of 70 °C while using 4 M NaOH to leach Zn and Pb from residual glass polishing waste. These studies indicate that zinc and lead leaching efficiency can be improved by increasing L/S ratio from 10/1 to 20/1. The leached zinc level rises from 60% to 80%, and the leached lead level rises from 48% to 63%, with a rise in L/S ratio from 10/1 to 20/1. Rao et al. (Citation2015) state that increasing the L/S ratio should lessen the slurry’s viscosity by permitting better mixing, hence leading to a decrease in diffusional mass transfer resistance. However, increasing the L/S ratio to 25/1 reduces the leaching rate. Other earlier studies (Dimitrijevic et al., Citation2016; Ghasemi & Azizi, Citation2018) have made similar observations. Thus, the L/S ratio of 20/1 is deemed ideal.
Figure 5. Effect of the S/L ratio on the efficiency with which lead (a) and zinc (b) were leached from first residual glass polishing waste with 4 M NaOH at 70 °C.

displays the temperature dependence of lead and zinc dissolution in alkaline-induced dissolution from first residual glass polishing waste at a phase ratio (L/S) of 20/1. After 90 min, the leached zinc level rises from 16% to 85.5%, and the leached lead level rises from 13% to 72.2%, with a rise in temperature from 25 °C to 80 °C. From these data, it can be concluded that an increase in temperature significantly accelerates the leaching rate of Pb and Zn by NaOH. The increases in temperature result in enhanced diffusivity, reaction constant and mass transfer coefficient (Rao et al., Citation2015). Therefore, it is clear that the reaction of hydroxyl ions with lead and zinc oxides in alkaline leaching is accelerated by heating the reagent to a high enough temperature. The dried alkali treated residue (), after Zn and Pb leaching, would undergo the third leaching process for REEs recovery as follows.
Figure 6. Effect of the temperature on the efficiency with which lead (a) and zinc (b) were leached from first residual glass polishing waste with 4 M NaOH at L/S ratio 20/1.

Table 3. The chemical constitutions of the dried alkali treated residue.
Using sodium sulphide as a precipitant, lead can be separated quantitatively from the filtrate solution of an alkaline solution without causing a loss of zinc (). Both zinc and lead precipitated in the alkaline solution after the addition of sodium sulphide as shown in the following equations:
The selective separation of lead from zinc was possible when proper weight ratios of sodium sulphide to lead were used (Youcai & Stanforth, Citation2001). The solubility of ZnS and PbS is 2 × 10−23 and 1 × 10−28, respectively; This indicates that PbS is significantly less soluble than ZnS, which is one of the reasons why lead can be selectively precipitated before zinc. The sodium sulphide-to-lead weight ratio was maintained between 1.8 and 2.0 (i.e., 209 g of Na2S was added to the alkaline leach liquor with stirring for 60 min.), and the zinc was left in the solution (Youcai & Stanforth, Citation2001); nearly 97% of lead was removed from the alkaline leach solution. Precipitated lead sulphide (PbS) was collected, washed with hot water for two hours, and then dried in an oven set to 110 °C. The content of Pb in the precipitated lead sulphide was 78.8%, while Zn content was 1% in this precipitate. After the lead was removed, the zinc was precipitated quantitatively if the sodium sulphide-to-zinc weight ratio was more than 2.6–2.7 (Youcai & Stanforth, Citation2001) (i.e., 12.2 g of Na2S was added to the alkaline leach liquor with stirring for 60 min.), as depicted in . The removal of Zn from the alkaline leach solution was 99.74%. Similar procedures were used to isolate and process the zinc sulphide precipitate as they were employed before with lead sulphide. The content of Zn in the precipitated zinc sulphide was 74.55%.
3.1.3. An analysis of the Pb and Zn dissolution kinetics
Metal dissolution reaction rates in NaOH solution were calculated using the shrinking core kinetic model. The reaction between the fluid and solid occurs on the solid’s surface, causing the surface to contract and leaving behind a thinner layer of ash, or the inert solid layer, in this kinetic model. This results in a gradual reduction in the size of the unreacted centre of the solid. The kinetics of the leaching process may be described by the following expressions (Apua & Madiba, Citation2021; Ghasemi & Azizi, Citation2018; Kong et al., Citation2022; Orabi, Mohamed, et al., Citation2021):
For solid product diffusion control:
(1)
(1)
For surface chemical reaction control:
(2)
(2)
For a mixed-controlled process (a combination of diffusion and chemical reaction):
(3)
(3)
where X is the dissolved metal fraction and t is the time required for dissolution in minutes. The apparent rates of diffusion and chemical reactions are denoted as K1 (min−1), K2 (min−1) and K3 (min−1), respectively. To explore the effect of temperature and NaOH concentration on the reaction kinetics of lead and zinc, a plot of 1 − (1 − x)1/3, 1 − 2/3(x) + (1 − x)2/3, and [(1 − X)−1/3 − 1] + 1/3 Ln(1 − X) against dissolution time is established and shown in and in Figures S1 and S2 in the Supplementary Material.
Figure 8. Effect of NaOH concentration and temperature on the leaching kinetics, 1 − (1 − X)1/3, of Pb and Zn from the residual glass polishing waste.

The best model was the one that had the highest R2 value when comparing the observed values of X over time. Since the straight line obtained from EquationEq. (2)(2)
(2) had a high correlation coefficient for both Pb and Zn, it was concluded that the rate-determining step was chemically regulated. Plots of 1 − (1 − X)1/3 against dissolution time (t) were constructed for different dissolving circumstances in order to investigate the impact of temperature and NaOH concentration on the kinetics of the dissolution of Pb and Zn from the residual glass polishing waste. depicts these charts for a range of NaOH concentrations and temperatures.
Furthermore, the activation energy (Ea), which can be determined using the Arrhenius equation, is a direct factor in the dissolution ratio during leaching processes. The activation energies were determined by plotting the Arrhenius equation as Ln (k) vs. (1/T) at each temperature () and using the slopes of straight lines with slopes equal to Ea/R to get the activation energies. In the case of lead, the activation energy was determined to be 32.16 kJ/mol; while in the case of zinc, it was 34.59 kJ/mol. Consequently, the results are consistent with the shrinking core model in which the chemical reaction serves as the rate-determining step (Jung & Mishra, Citation2016; Shen et al., Citation2018; Tavakoli, Abdollahy, Ahmadi, & Darban, Citation2017; Yuan, Citation2018). When the apparent energy of activation is low, it suggests that the reaction mechanism involves the adsorption of reactants, followed by the chemical reaction itself (Souza, Pina, Leão, Silva, & Siqueira, Citation2007). It is worth noting that as the iron content in the sample increases, the activation energy decreases (Bobeck & Su, Citation1985; Perez & Dutrizac, Citation1991). These results were agreed with many researches that expressed by chemical reaction (Li et al., Citation2018; Wang et al., Citation2022; Zhou et al., Citation2018).
3.1.4. Third-stage leaching process for total REEs (Ce, La, and Y) recovery
After 4 M NaOH treatment, the dried alkali treated residue still had an undissolved REEs content of 60.25 g/Kg. After filtering the leachate, the residue’s XRF spectra are displayed in . The REEs were discovered to be present in the residue, as predicted. As was previously stated, these components are stable and challenging to extract using conventional techniques. This is particularly true when REEs oxides are created through high-temperature annealing, which is necessary for their usage as polishing powder (Bao et al., Citation2020; Lee et al., Citation2019; Magnago et al., Citation2021; Wu et al., Citation2021). Therefore, more demanding circumstances are needed.
The alkali-treated residue, which had been dried, underwent a leaching process with concentrated H2SO4. This process was carried out using a liquid-to-solid ratio of 3:1 at temperatures ranging from 300 °C to 330 °C, and for a duration of 3 h (Borra et al., Citation2021; Mohamed et al., Citation2019; Orabi et al., Citation2017, Orabi, Mohamed, et al., Citation2021). Following digestion, a thick grey paste was produced, which cooled while water diluted the residue by at least 20 times its weight over the course of at least 2 h. The temperature must be cooled in order not to exceed 17 °C; when the temperature drops, rare earth sulphates become more soluble. The REEs leaching efficiency from the third stage leaching process was 98.8%. shows the XRF spectra of the residue that remained after third stage REEs leaching process. As expected, the majority of REEs were leached and removed from the residue when it was treated with concentrated sulphuric acid compared to the residue before the third stage leaching process (). For selective precipitation of REEs, oxalic acid was added to the filtrate at a mole ratio of 1:2 REEs to oxalic acid. Precipitated rare earth oxalate was filtered, washed many times in demineralized water, and then it was calcined at 850 °C for 1 h to yield rare earth oxides (). The suggested flow sheet for the whole process was shown in .
4. Conclusions
This study explored a three-step sequential leaching process to selectively recover lead, zinc, and rare earth elements from a hazardous glass polishing waste. The first step used sulphuric acid to remove impurities, leaving behind the metals of interest. The second step utilized a sodium hydroxide solution to extract lead and zinc, with maximum leaching efficiencies achieved at 80 °C and 4 M NaOH. Sodium sulphide was used to remove lead and zinc from the filtrate solution, with high removal rates observed. The final step involved leaching the residue with concentrated H2SO4 at 300 °C to recover REEs. Oxalic acid was added to selectively precipitate REEs. The study produced a flow sheet for the technological recovery of Pb, Zn, and REEs from glass polishing waste, which could contribute to a circular economy.
Supplemental Material
Download MS Word (314.6 KB)Acknowledgments
The authors express their gratitude for the support and assistance extended by their institution (Badr University, Jazan University).
Disclosure statement
No potential conflict of interest was reported by the author(s).
Data availability statement
Data available from authors.
References
- Abdel-Aal, E. (2000). Kinetics of sulfuric acid leaching of low-grade zinc silicate ore. Hydrometallurgy, 55(3), 247–254. doi:10.1016/S0304-386X(00)00059-1
- Adhikary, S. K., Ashish, D. K., & Rudžionis, Ž. (2021). Expanded glass as light-weight aggregate in concrete—A review. Journal of Cleaner Production, 313, 127848. doi:10.1016/j.jclepro.2021.127848
- Apua, M., & Madiba, M. (2021). Leaching kinetics and predictive models for elements extraction from copper oxide ore in sulphuric acid. Journal of the Taiwan Institute of Chemical Engineers, 121, 313–320. doi:10.1016/j.jtice.2021.04.005
- Bao, X., Zhang, Z., Luo, T., Wu, X., Xie, Z., Lan, S., … Zhou, D. (2020). Conversion of cerium and lanthanum from rare earth polishing powder wastes to CeO2 and La0.6Ca0.4CoO3. Hydrometallurgy, 193, 105317. doi:10.1016/j.hydromet.2020.105317
- Bobeck, G., & Su, H. (1985). The kinetics of dissolution of sphalerite in ferric chloride solutions. Metallurgical Transactions B, 16(3), 413–424. doi:10.1007/BF02654839
- Borra, C., Vlugt, T., Spooren, J., Nielsen, P., Yang, Y., & Offerman, S. (2019). Characterization and feasibility studies on complete recovery of rare earths from glass polishing waste. Metals, 9(3), 278. doi:10.3390/met9030278
- Borra, C., Vlugt, T., Yang, Y., Spooren, J., Nielsen, P., Amirthalingam, M., & Offerman, E. (2021). Recovery of rare earths from glass polishing waste for the production of aluminium-rare earth alloys. Resources, Conservation & Recycling, 174, 105766. doi:10.1016/j.resconrec.2021.105766
- Chen, A., Zhao, Z., Jia, X., Long, S., Huo, G., & Chen, X. (2009). Alkaline leaching Zn and its concomitant metals from refractory hemimorphite zinc oxide ore. Hydrometallurgy, 97(3-4), 228–232. doi:10.1016/j.hydromet.2009.01.005
- Cuixiang, G., Long, Z., Qing, L., & Youcai, Z. (2007). Leaching of Zn and Pb from Zn and Pb bearing wastes in caustic soda solution. Environmental Pollution Control, 29, 697–700.
- Cuixiang, G., & Youcai, Z. (2008). Recovery of Pb and Zn from smoke dust. Environmental Protection of Chemical Industry, 28, 77–80.
- Dimitrijevic, M., Urosevic, D., Jankovic, Z., & Milic, S. (2016). Recovery of copper from smelting slag by sulphation roasting and water leaching. Physicochemical Problems of Mineral Processing, 52(1), 409–421.
- Espiari, S., Rashchi, F., & Sadrnezhaad, S. (2006). Hydrometallurgical treatment of tailings with high zinc content. Hydrometallurgy, 82(1–2), 54–62. doi:10.1016/j.hydromet.2006.01.005
- Ghasemi, S., & Azizi, A. (2018). Alkaline leaching of lead and zinc by sodium hydroxide: Kinetics modeling. Journal of Materials Research and Technology. 7(2), 118–125. doi:10.1016/j.jmrt.2017.03.005
- Graziano, S., Zanelli, C., Molinari, C., Gennaro, B., Giovinco, G., Correggia, C., … Dondi, M. (2022). Use of screen glass and polishing sludge in waste-based expanded aggregates for resource-saving lightweight concrete. Journal of Cleaner Production, 332, 130089. doi:10.1016/j.jclepro.2021.130089
- Hou, Z., Peng, C., Guangyuan, M., Zhang, X., Zhang, L., & Lu, Z. (2022). Lead leaching behaviors during panel-funnel glass waste separation from cathode-ray tube glass using thermosol, acid etching or mechanical cutting methods. Journal of Saudi Chemical Society, 26(6), 101557. doi:10.1016/j.jscs.2022.101557
- Janos, P., Kuran, P., Kormunda, M., Stengl, V., Grygar, T. M., Dosek, M., … Vrtoch, L. (2014). Cerium dioxide as a new reactive sorbent for fast degradation of parathion methyl and some other organophosphates. Journal of Rare Earths, 32(4), 360–370. doi:10.1016/S1002-0721(14)60079-X
- Jung, M., & Mishra, B. (2016). Kinetic and thermodynamic study of aluminum recovery from the aluminum smelter baghouse dust. Journal of Sustainable Metallurgy, 2(3), 257–264. doi:10.1007/s40831-016-0056-6
- Kim, Y., Kim, U., Byeon, M., Kang, W., Hwang, K., & Cho, W. (2011). Recovery of cerium from glass polishing slurry. Journal of Rare Earths, 29(11), 1075–1078. doi:10.1016/S1002-0721(10)60601-1
- Kong, D., Zhou, Z., Jiang, R., Song, S., Feng, S., & Lian, M. (2022). Extraction of aluminum and iron ions from coal gangue by acid leaching and kinetic analyses. Minerals, 12(2), 215. doi:10.3390/min12020215
- Kumar, K., Jha, M., Kumari, A., Panda, R., Kumar, J., & Lee, J. (2014). Recovery of rare earth metals (REMs) from primary and secondary resources: A review., In Rare Metal Technology, R. Neale, N. R. Neelameggham, S. Alam, H. Oosterhof, A. Jha and S. Wang, Eds.; Wiley: San Diego, CA, pp. 81–88
- Larsson, K., Ekberg, C., & Ødegaard-Jensen, A. (2013). Dissolution and characterization of HEV NiMH batteries. Waste Management (New York, N.Y.), 33(3), 689–698. doi:10.1016/j.wasman.2012.06.001
- Lee, C., Lo, Y., Sandagdorj, N., Gankhuyag, E., Popuri, S., & Hung, C. (2019). Recycling of cerium and lanthanum from glass polishing sludge. Polish Journal of Chemical Technology, 21(4), 26–30. doi:10.2478/pjct-2019-0035
- Li, L., Bian, Y., Zhang, X., Guan, Y., Fan, E., Wu, F., & Chen, R. (2018). Process for recycling mixed-cathode materials from spent lithium-ion batteries and kinetics of leaching. Waste Management (New York, N.Y.), 71, 362–371. doi:10.1016/j.wasman.2017.10.028
- Li, C., Liu, W., Jiao, F., Yang, C., Li, G., Liu, S., & Qin, W. (2023a). Separation and recovery of zinc, lead and iron from electric arc furnace dust by low temperature smelting. Separation and Purification Technology, 312, 123355. doi:10.1016/j.seppur.2023.123355
- Liu, C., Ju, S., Zhang, L., Srinivasakannan, C., Peng, J., Le, T., & Guo, Z. (2017). Recovery of valuable metals from jarosite by sulphuric acid roasting using microwave and water leaching. Canadian Metallurgical Quarterly. 56(1), 1–9. doi:10.1080/00084433.2016.1242972
- Liu, Q., Zhao, Y., & Zhao, G. (2011). Production of zinc and lead concentrates from lean oxidized zinc ores by alkaline leaching followed by two-step precipitation using sulfides. Hydrometallurgy, 110(1–4), 79–84. doi:10.1016/j.hydromet.2011.08.009
- Li, W., Zhang, W., Luo, L., & Xie, X. (2023b). Recycling lead from waste lead-acid batteries by the combination of low temperature alkaline and bath smelting. Separation and Purification Technology, 310, 123156. doi:10.1016/j.seppur.2023.123156
- Luo, T., Wu, X., Bao, X., Wang, Z., Xia, C., Lan, S., … Chen, J. (2020). Research process in recovering and reutilizing of rare earth polishing powder wastes. Chinese Rare Earths, 41(3), 96–104 (in Chinese).
- Magnago, R., Braglia, T., Aguiar, A., Baungarten, P., Mendonça, B., Silva, H., … Parma, G. (2021). Recycling glass‑polishing sludge and aluminum anodising sludge in polyurethane and cement composites: Fire performance and mechanical properties. Journal of Material Cycles and Waste Management, 23(3), 1126–1140. doi:10.1007/s10163-021-01202-x
- Mohamed, B., Guirguis, L., Orabi, A., & Khalil, L. (2019). Extraction of thorium(IV) with N-methyl-N,N,N-trioctylammonium chloride from monazite acidic leach liquor and its use for spectrophotometric determination. Radiochemistry, 61(5), 569–578. doi:10.1134/S1066362219050084
- Oh, M., Nho, J., Cho, S., Lee, J., & Singh, R. K. (2011). Polishing behaviors of ceria abrasives on silicon dioxide and silicon nitride CMP. Powder Technology. 206(3), 239–245. doi:10.1016/j.powtec.2010.09.025
- Orabi, A., Mohamed, B., Ismaiel, D., & Elyan, S. (2021). Sequential separation and selective extraction of uranium and thorium from monazite sulfate leach liquor using dipropylamine extractant. Minerals Engineering. 172, 107151. doi:10.1016/j.mineng.2021.107151
- Orabi, A. H., Rabia, K., Elshereafy, E., & Salem, A. (2017). Application of commercial adsorbent for rare earth elements—Uranium mutual separation and purification. Mediterranean Journal of Chemistry, 6(6), 238–254. doi:10.13171/mjc66/01712211014-orabi
- Orabi, A., Zaki, S., Bayoumi, M., & Ismaiel, D. (2021). Leaching characteristics of uranium from El‑Missikat mineralized granite. Euro-Mediterranean Journal for Environmental Integration, 6(1), 14. doi:10.1007/s41207-020-00214-7
- Pascual, A. B., Tognonvi, T. M., & Tagnit-Hamou, A. (2021). Optimization study of waste glass powder-based alkali activated materials incorporating metakaolin: Activation and curing conditions. Journal of Cleaner Production, 308, 127435. doi:10.1016/j.jclepro.2021.127435
- Perez, I., & Dutrizac, J. (1991). The effect of the iron content of sphalerite on its rate of dissolution in ferric sulphate and ferric chloride media. Hydrometallurgy, 26(2), 211–232. doi:10.1016/0304-386X(91)90032-H
- Poscher, A., Luidold, S., Schnideritsch, H., & Antrekowitsch, H. (2016). Chapter 14-Extraction of lanthanides from spent polishing agent. Rare Earth Industies, 209–222. doi:10.1016/B978-0-12-802328-0.00014-0
- Rao, S., Yang, T., Zhang, D., Liu, W., Chen, L., Hao, Z., … Wen, J. (2015). Leaching of low-grade zinc oxide ores in NH4Cl–NH3 solutions with nitrilotriacetic acid as complexing agent. Hydrometallurgy, 158, 101–106. doi:10.1016/j.hydromet.2015.10.013
- Ruixiang, W., Motang, T., Shenghai, Y., Wenhai, Z., Chaobo, T., Jing, H., & Jian-guang, Y., (2008). Leaching kinetics of low grade zinc oxide ore inNH3–NH4Cl–H2O system. J. Cent. South Univ. Technol., 15, 679–683. doi:10.1007/s11771-008-0126-4
- Ruşen, A., Sunkar, A. S., & Topkaya, Y. A. (2008). Zinc and lead extraction from Cinkur leach residues by using hydrometallurgy method. Hydrometallurgy, 93(1–2), 45–50. doi:10.1016/j.hydromet.2008.02.018
- Safari, V., Arzpeyma, G., Rashchi, F., & Mostoufi, N. (2009). A shrinking particle–shrinking core model for leaching of a zinc ore containing silica. International Journal of Mineral Processing. 93(1), 79–83. doi:10.1016/j.minpro.2009.06.003
- Santos, F. M., Pina, P. S., Porcaro, R., Oliveira, V. A., Silva, C. A., & Leão, V. A. (2010). The kinetics of zinc silicate leaching in sodium hydroxide. Hydrometallurgy, 102(1–4), 43–49. doi:10.1016/j.hydromet.2010.01.010
- Shen, L., Li, X., Zhou, Q., Peng, Z., Liu, G., Qi, T., & Taskinen, P. (2018). Kinetics of scheelite conversion in sulfuric acid. JOM Journal of the Minerals Metals and Materials Society. 70(11), 2499–2504. doi:10.1007/s11837-018-2787-2
- Souza, A. D., Pina, P. S., Leão, V. A., Silva, C. A., & Siqueira, P. F. (2007). The leaching kinetics of a zinc sulphide concentrate in acid ferric sulphate. Hydrometallurgy, 89(1-2), 72–81. doi:10.1016/j.hydromet.2007.05.008
- Tavakoli, H., Abdollahy, M., Ahmadi, S., & Darban, A. (2017). Kinetics of uranium bioleaching in stirred and column reactors. Minerals Engineering. 111, 36–46. doi:10.1016/j.mineng.2017.06.003
- Tercero Espinoza, L., Hummen, T., Brunot, A., Hovestad, A., Peña Garay, I., Velte, D., Smuk, L., Todorovic, J., Van Der Eijk, C., & Joce, C. (2015). Critical raw materials substitution profiles, Karlsruhe, Germany. CRM_InnoNet.
- Wang, P., Tan, K., Li, Y., Liu, Z., Li, C., Tan, W., … Huang, W. (2022). Effect of pyrite on the leaching kinetics of pitchblende in the process of acid in situ leaching of uranium. Minerals, 12(5), 570. doi:10.3390/min12050570
- Wu, Y., Song, M., Zhang, Q., & Wang, W. (2021). Review of rare-earths recovery from polishing powder waste. Resources, Conservation and Recycling, 171, 105660. doi:10.1016/j.resconrec.2021.105660
- Youcai, Z., & Stanforth, R. (2001). Selective separation of lead from alkaline zinc solution by sulfide precipitation. Separation Science and Technology, 36(11), 2561–2570. doi:10.1081/SS-100106110
- Yu, B., Jiang, J., & Yang, C. (2019). Conversion of lanthanum and cerium recovered from hazardous waste polishing powders to hazardous ammonia decomposition catalysts. Journal of Hazardous Materials, 379, 120773. doi:10.1016/j.jhazmat.2019.120773
- Yuan, F. (2018). Study on kinetics of Fe (II) oxidized by air in FeSO4–H2SO4 solutions. Minerals Engineering. 121, 164–168. doi:10.1016/j.mineng.2018.03.013
- Zhang, Y., Hua, Y., Gao, X., Xu, C., Li, J., Li, Y., … Ru, J. (2016). Recovery of zinc from a low-grade zinc oxide ore with high silicon by sulfuric acid curing and water leaching. Hydrometallurgy, 166, 16–21. doi:10.1016/j.hydromet.2016.08.010
- Zhang, C., Zhuang, L., Li, Y., Yuan, W., Wang, J., & Bai, J. (2015). Extraction of lead from spent leaded glass in alkaline solution. Applied Mechanics and Materials, 768, 441–446. doi:10.4028/www.scientific.net/AMM.768.441
- Zhou, X., Chen, Y., Yin, J., Xia, W., Yuan, X., & Xiang, X. (2018). Leaching kinetics of cobalt from the scraps of spent aerospace magnetic materials. Waste Management (New York, N.Y.), 76, 663–670. doi:10.1016/j.wasman.2018.03.051
- Zielinski, M., Cassayre, L., Destrac, P., Coppey, N., Garin, G., & Biscans, B. (2020). Leaching mechanisms of industrial powders of spent nickel metal hydride batteries in a pilot-scale reactor. ChemSusChem, 13(3), 616–628. doi:10.1002/cssc.201902640