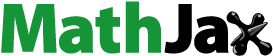
Abstract
Adsorption performance of maize cob-derived activated carbon (MCAC) for the removal of nickel (II) from solution was investigated in the present study. The MCAC was prepared by adsorbent chemical activation method. Afterwards, the MCAC was characterized and utilized as the adsorbent for batch adsorption process. Initial adsorbate concentration, contact time and adsorbent dosage were considered as the independent variables for the adsorption process. The experiments were designed based upon central composite design (CCD) of response surface methodology with the aid of Design Expert. The experimental data were fitted into a model using MATLAB regression analysis, and the developed model was later simulated to obtain the operation parameters that will give the optimum removal efficiency. The analysis of variance (ANOVA) revealed that all the considered independent process variables had significant effect on the adsorption process. The simulated response from MATLAB gave 97.113% removal efficiency of nickel (II) on experimental validation at optimum conditions of 9.75 mg/L initial nickel (II) concentration, 120 min contact time and 0.803 g adsorbent dosage. This result correlates with CCD that predicted 97.6154% nickel (II) removal efficiency. The experimental data were observed to be best fitted to pseudo-second-order kinetic model which showed that the process is chemisorption controlled. Hence, it may be concluded from the present data that MCAC is highly effective for the removal of Ni(II) from aqueous solution and the highly generated agricultural waste (Maize cob) can the easily modified as a adsorbent.
1. Introduction
Anthropogenic activities resulting from high rate of urbanization and industrialization have been considered as important contributors to environmental pollution (Bradl, Citation2002). The increasing load of heavy metals in water bodies can be attributed to the vast use of heavy metal containing compounds in domestic, agricultural and industrial applications (Yusuff, Popoola, & Babatunde, Citation2019). Studies have shown that operations such as mining, smelting, metal galvanizations, burning of coal in power plants, processing of metal in refineries, wood preservation activities and some agricultural products processing releases unacceptable amount of toxic metals to environment (Ahmed, Yasser, Norhan, & Farida, Citation2019; Tchounwou, Yedjou, Patlolla, & Sutton, Citation2014). Heavy metals are relatively high density (usually 3.5 to 7 g cm−3) metallic or metalloid elements, capable of inducing toxicity to human and the environment at some level of exposure (Tchounwou et al., Citation2014). Heavy metals can be considered as either essential or non-essential. Essential heavy metals help with metabolism activities in human body and also help with some functions (e.g. physiological and biochemical) in animals and plants when they exist in trace quantities; otherwise they induce serious concern (Gautam, Sharma, Mahiyab, & Chattopadhyaya, Citation2014). The non-essential heavy metals are those with little or no recognized biological functions, and their excessive exposure was seen to cause diverse side effects such as cellular and tissue damage (Lala, Kawu, Adesina, & Sonibare, Citation2022).
Nickel, a very common and predominant metal occurs naturally in the crust of the earth and it is also present in cigarettes as nickel carbonyl. Water-soluble salts of nickel are highly applicable in electroplating, aircraft parts, automobile parts and spark plugs. Also, they are useful in making coins, stainless steel, cosmetics, nickel–cadmium batteries, etc., and they constitute the main aquatic body pollution problem (Gautam, Sharma, Mahiyab, & Chattopadhyaya, Citation2014). It has been shown that soluble micron nickels are more toxic compared to nickel nanoparticles (Zhou et al., Citation2016). Nickel gains access to water bodies through mining, fuel combustion, waste incineration, rock weathering, and discharges from paint formulation and enamelling industries, etc (Buxton et al., Citation2019). Nickel helps in red blood cells synthesis if present in very trace amount, but become toxic when exposed to higher doses for long period. Nickel can lead to liver damage, nervous system damage, heart failure and loss in body weight. Also, nickel causes cell growth reduction and are highly carcinogenic (Gautam, Sharma, Mahiyab, & Chattopadhyaya, Citation2014). Nickel thresholds level corresponding to some human health and environmental compartment are; 0.44 µg Ni/cm2 skin/day for human allergic contact dermatitis (Fischer, Menné, & Johansen, Citation2005), 0.1 mg Ni/m3 inhalable aerosol for human respiratory cancer (Oller, Oberdörster, & Seilkop, Citation2014), 0.5 µg Ni/m3 ng/m3 PM10 aerosol for human respiratory cancer (Buekers et al., Citation2015) and 4 µg Ni/L bioavailability-based for freshwater (European Union (EU), Citation2013). Hence, it is highly important to treat nickel-contaminated waste water before their discharge into water bodies.
Studies have shown the effectiveness of removing nickel and some other heavy metals from wastewater through reverse osmosis, ion exchange, electrocoagulation, adsorption, electrochemical, membrane filtration, bioremediation and heterogeneous photocatalysts (Yusuff et al., Citation2019; Osasona, Aiyedatiwa, Johnson, & Faboya, Citation2018). Adsorption among other methods is widely accepted due to its effectiveness, simplicity, possibility of using different low cost materials such as bentonite as adsorbent (Caglar, Afsin, Tabak, & Eren, Citation2009), and also because the method is highly environmentally friendly (Osasona et al., Citation2018; Yusuff et al., Citation2019). Adsorption process is a unit operation where fluid concentration is altered by passing it through a porous material called adsorbent. Adsorbents such as activated carbon (Osasona et al., Citation2018), zeolite (Kussainova et al., Citation2018), etc have been utilised for removing trace metals in wastewater. In recent times, different industrial wastes and biomass have been used in preparing activated carbon (Reza et al., Citation2020; Heidarinejad et al., Citation2020). Carbonization and activation are the 2 stages involved in activation carbon preparation. Activation of the carbonized material can be carried out through; physical (using heat with gas such as N2, CO2 and H2O), chemical (using H3PO4, KOH, NaOH, ZnCl2, etc), physiochemical (by chemical and heat), and also microwave activation (Reza et al., Citation2020). Among all, chemical activation is more economical as it requires shorter processing time, lower activation temperature and higher carbon efficiency (Heidarinejad et al., Citation2020). Adsorption of Ni(II) ion from aqueous solution using low-cost wastes is possible and these include raw bentonite (Caglar et al., Citation2009), chemically modified biosorbent from rice bran (Zafar et al., Citation2015) and acid-treated lignin obtained from black liquor in pulp and paper industry (Pérez, Citation2006). Also, maize cob (Arunkumar, Perumal, Lakshmi, & Arunkumar, Citation2014) and bagasse fly ash (Garg, Kaur, Garg, & Sud, Citation2008) were also considered by some researchers.
The effectiveness of an adsorbent depends on its method of preparation and the functional groups present within (Herrera-Barros, Tejada-Tovar, Villabona-Ortiz, Gonzalez-Delgado, & Alvarez-Calderon, Citation2018). Studies have shown that carboxyl, hydroxyl and amide groups which are in favour of the heavy metal adsorption are present maize cob (Herrera-Barros et al., Citation2018). Also, adsorbent modification influences the surface area and adsorption capacities. The study of Al2O3 nanoparticles modified maize cob (Herrera-Barros et al., Citation2018) showed 86% removal of nickel from aqueous solution. This is higher than those obtained using maize cob particles.
Maize is a staple agricultural product in Nigeria. It serves as a major ingredient for the production of various types of food and can also be consumed directly when boiled or roasted. Maize cob on the other hand is one of the natural wastes from maize plant and may cause high level of environmental pollution if not properly handled. The ratio of maize grain to the cob is given as 100:18 (Hartmann, Khalil, & Bernet, Citation2012). This may result in useless accumulation of the cobs worldwide and particularly in Nigeria in case of not being properly utilized. Therefore, the present work has been carried out to the possibility of using on NaOH-treated activated carbon produced from maize cob for removing nickel (II) ion from aqueous solutions. Statistical and adsorption kinetics analyses were carried out to develop models that could describe the process in detail.
2. Materials and methods
2.1. Materials
Maize cobs used in this research work were collected from a local farm close to Afe Babalola University, Ado-Ekiti, Ekiti State, Nigeria. Sodium hydroxide (NaOH), nickel nitrate hexa-hydrate () and all other chemical used in this work were purchased from Topjay Chemical Enterprise, Ado-Ekiti, Nigeria, and they were all analytical grade. A stock solution of 1000 mg/L Ni(II) was prepared by dissolving 5.82 g of the salt in 1000 mL deionized water. Various solutions of needed initial Ni(II) concentrations were obtained by diluting the stock solution with deionized water ().
2.2. Preparation of the adsorbent (MCAC)
The collected maize cobs were thoroughly washed with clean water so that all unwanted materials can be removed. Subsequently, distilled water was used to re-wash so as to avoid the alteration of the composition of the material, and then dried by heating up to 105°C for 3 hr in an oven. The dried maize cobs were crushed with mortar and pestle. Subsequently, the maize cob size was further reduced with the aid of a laboratory grinding mill. The sample thus obtained was then screened using a laboratory sieve-shaker to yield 300 µm uniform-sized particles. Afterwards, 300 g of the pulverized maize cob was poured into a beaker containing 1000 cm3 of 1.0 M sodium hydroxide (NaOH) solution, and left for 24 hr to ensure proper impregnation. After the impregnation, deioni zed water was used to wash the resulting activated maize cob, filtered and dried in an oven at 105°C for 3 h. Finally, the activated maize cob was carbonized at 240°C for 5 min using a furnace (muffle). The derived maize cob activated carbon was screened again using the laboratory sieve-shaker to reach 300 µm uniform-sized particles labelled MCAC.
2.3. Characterization of the maize cob based activated carbon (MCAC)
C, H, O, N and S amounts of maize cob were determined based on standard procedures (García, Pizarro, Lavín, & Bueno, Citation2012). Also, proximate analysis was performed on the MCAC to determine its volatile matter (VM) (% w/w), moisture content (MC) (% w/w), fixed carbon (FC) (% w/w) and ash content (AC) (% w/w) as described by Dada, Inyinbor, and Oluyori (Citation2012). Bulk density (g/cm3) and pH of the MCAC were determined using the method adopted by Dada et al. (Citation2012). Here, the bulk density was determined by drying some MCAC for 1 h in an oven at 105°C. The ratio of weight of powder taken in 25 cm3 bottle to 25 gives the bulk density. Also, pH of the adsorbent was determined by introducing 2 g of MCAC into a beaker containing 20 mL of distilled water and the mixture heated for 15 min under reflux. Moreover, morphology of the prepared MCAC was determined with the aid of scanning electron microscope (SEM-JEOL-JSM 7600 F) before and after adsorption.
2.4. Adsorption studies
2.4.1. Experimental design and analysis
The influence of three process variables on the adsorption of Ni(II) on MCAC was considered using Response Surface Methodology (RSM). Central Composite Design (CCD), an important RSM tool was used to design experiment for the independent process variables namely, initial concentration of Ni(II), C mg/L; MCAC dosage, d g and contact time, t min. In the present study, the effects of these three variables on the adsorption of Ni(II) on MCAC were investigated and each numeric factor was varied over 5 levels. The CCD was characterized by 6 axial points, factorial points of 8 and 1 central replicate accounting for a total of 15 experiments. Levels generated by the CCD tool embedded in Design Expert 12.0 for the three factors studied are shown in . The response i.e removal efficiency of Ni(II) was determined and develop into a mathematical model. The model showed the relationship between the dependent variable (R) and the three independent variables as represented by EquationEquation (1)(1)
(1) , where
and
are the coefficients for the model. The variables C, t and d are the actual values of the experimental independent variables.
(1)
(1)
Table 1. Experimental process parameters and levels.
2.4.2. Experimental procedures
The stock adsorbate solution for the experiment was prepared from nickel nitrate hexa-hydrate (). Adsorption experiments onto MCAC were performed by batch tests to see the effects of contact time, initial Ni(II) concentration and MCAC dosage on Ni(II) removal from aqueous solutions. The batch experiments were carried out in 250 mL beakers containing 50 mL aqueous solution of nickel ions of various concentrations unto which activated carbon was added. At room temperature, the suspensions were shaken with a mechanical shaker at 120 rpm for a certain time. The solutions were filtered off using whatman 42 filter paper and the absorbance of the solutions was determined afterwards. The absorbance was determined using Buck Scientific 211 VGP Atomic Absorption Spectrophotometer (AAS) in Afe-Babalola University (ABUAD), Nigeria. The amount of Ni(II) remained in equilibrium and the removal efficiency of Ni(II) in the solution was calculated using EquationEqs. (2)
(2)
(2) and Equation(3)
(3)
(3) . R% is the removal efficiency of Ni(II) in the aqueous solution and qt is the adsorption capacity at any time t. C0 is the initial concentration of the metal ion (mg/L), Ce is the equilibrium concentration of the metal ion (mg/L), Ct is the metal concentration at any time t (mg/L), V is the volume of the adsorbate solution (mL) and m is the mass of MCAC (g) used.
(2)
(2)
(3)
(3)
2.4.3. Kinetic modelling
Intra-particle diffusion (IpD), pseudo-first order (PFO) and pseudo-second (PSO) order kinetic models were used to determination Ni(II) adsorption mechanism on the prepared MCAC surface. Non-linear equations of the three stated models are given in EquationEqs. (5)
(5) Equation(4)
(4)
(4) , Equation(5)
(5)
(5) and Equation(6)
(6)
(6) respectively, where ke (mg.g-1.min−1/2), k1 (min−1) and k2 (g−1 min−1) are the rate constant for IpD, PFO and PSO model respectively. Adsorbate present in equilibrium and over time t (mg/g) is given as qe and qt respectively.
(4)
(4)
(5)
(5)
(6)
(6)
3. Results and discussion
3.1. Adsorbent characterization
Presented in are the physical and chemical parameters of MCAC. The elemental composition showed lower values of nitrogen (0.35%) and sulphur (0.23%) compared to oxygen (44.62%), carbon (49.50%) and hydrogen (5.32%). According to the literature, biomass with high hydrogen, oxygen and carbon content performs will in adsorption process; hence the prepared MCAC has a good tendency towards the removal of Ni(II) ion from aqueous solution. Moreover, the obtained bulk density (0.482) is similar to those obtained by some researchers as materials suitable for adsorbent (Igwegbe, Onukwuli, Onyechi, & Ahmadi, Citation2020, Dada Citation2012). The particle size (300 µm) preferred of the prepared MCAC seems suitable as it is being an effective adsorbent. The significant surface area increase of the smaller sized adsorbents induced by the development of microporous structure contributes to the adsorption efficiency (Tejada-Tovar, Ortiz, Gonzalez, Cantillo, & Montero, Citation2018, Regti, Laamari, Stiriba, & E-Haddad, Citation2017, Koksal, Afsin, Tabak, & Caglar, Citation2020). The reported moisture content of MCAC sample (7.8%) implies that the MCAC will dilute the carbon action and will necessitate utilizing more of the carbon (Thangamani et al., Citation2007). Also, the low ash content (9.2%) and high fixed carbon content (36.7%) showed that the MCAC is of good quality. Carbons with low ash content possess more sorption and high fixed carbon signifies high adsorption capacity (Dada et al., Citation2012). The reported pH (7.5) of MCAC shows that this material can be used as a good adsorbent for treating water since the value is close to neutral pH value (Igwegbe, Onukwuli, et al., Citation2020; Igwegbe, Onukwuli, et al., Citation2020). There were changes in the undefined, clouds-like and rough structure of the micrograph in compared to . This is due to the adsorption of Ni(II) on the MCAC surface. presents the micrograph image of the fresh MCAC (i.e before adsorption) while presents the micrograph images of the MCAC after the adsorption. The MCAC structure became more defined and clear after the adsorption due to the observed blocked pores and agglomeration of the adsorbent particles.
Table 2. Physicochemical properties MCAC.
3.2. Contact time effect
Contact time effect on Ni(II) adsorption onto MCAC was studied for 15 min to 150 min with the intervals of 15 min. 1 g dosage of MCAC was considered and the experiment was performed at room temperature. Ni(II) initial concentration and speed of agitation were maintained at 10.67 mg/L and 120 rpm. respectively. shows that Ni(II) adsorption on MCAC increased with increasing the contact time up to 120 min and there is no removal at 0 min. After 15 min and 30 min of adsorption periods, the removal percentage of Ni(II) is shown to be less dependent on the presence of available active sites on the surface that were not in properly contact with the aqueous solution. Furthermore, at a contact time of 150 min, lower removal efficiency is attained which may be explained by the absence of free sites on the adsorbent surface in contact. Thus, the contact time of 120 min can be suggested for optimum removal (85%) of Ni(II) from the aqueous solution.
3.3. Dosage effect
Dosage is another parameter that strongly influences the adsorption process. MCAC quantity effect on the adsorption of Ni(II) was studied for 0.2 g up to 1 g. The experiments were carried out for 120 min at room temperature. The initial concentration of nickel solution and the agitation speed was maintained at 9.85 mg/L and 120 rpm, respectively. shows a progressive increase in Ni(II) percentage removal with increase in the amount of adsorbent up to 0.8 g. This may be attributed to the increase of adsorbent surface area and also to the generation of new empty adsorption sites following successive contact. However, the removal percentage decreased when the adsorbent dosage was increased from 0.8 to 1.0 g. This may be link to agglomeration of the MCAC particles and resulting into their adsorption sites overlapping. Thus, 0.8 g of MCAC may be suggested for optimum removal of Ni(II) from the aqueous solution.
3.4. Central composite design (CCD) model and data analysis
presented the CCD design comprising the adsorption variables and their corresponding response. A 2FI model was suggested by the design and was adopted for the removal response, and also used to develop the correlation between MCAC and uptake of Ni(II). The model developed in actual units for the efficiency of Ni(II) removal is represented by EquationEquation 7(7)
(7) . The two variables included in the equation imply a double factor effect.
(7)
(7)
Table 3. Experimental design removal efficiency.
The experimental response from a set of data is known to be well predicted by a model when the values of the correlation coefficient is close to unity and when the standard deviation is considerably small (Tan, Ahmad, & Hameed, Citation2008). The current experimental result shows good correlation between the predicted and experimental values. The experimental and the predicted values for the adsorption process were presented in and . It was indicated that there was a good capture of relation between the adsorption parameters and the responses by the developed model.
Furthermore, the developed model authenticity was examined based on the values for correlation coefficient (R2) and standard deviation as given in . The model equation R2 value was obtained as 0.9984 which may be considered to be close to unity. The standard deviation of the model was calculated as 0.7456 and this value is agreeably small, indicating that the predicted responses have accurate values and are similar to those of the actual response. It may also suggest that the selected 2FI model was suitable in predicting the response variables. Moreover, as given in (ANOVA table), the significance of the fitted model was also justified by F-value i.e fisher’s test value, p-value i.e probability of occurrence, adjusted R2 and predicted R2. The F-value (834.88) implies that the model is significant and that the probability of F-value this much could occur due to noise is 0.01%. Also, the p-value (< 0.0001) indicated that the model is adequate since it is below 0.05. Moreover, all the independent variables present in the model (C, t, d, tC, dC and td) were significant since there p-value is below than 0.05. The pred-R2 and the Adj. R2 values of 0.9653 and 0.9972 respectively agreed perfectly well and therefore, confirmed the predictable model. Percentage contributions of the significant process parameters were calculated from the mean square as 12.14%, 70.85%, 11.24%, 0.94%, 0.34% and 4.50% for C, t, d, tC, dC and td respectively. Time, t (min) has the more percentage contribution (70.85%) compared to the other process parameters. This points out that the adsorption of Ni(II) depends more on the contact time of MCAC with the adsorbate solution. Considering the interactive effect of parameters on the removal efficiency of Ni(II) ions from aqueous solution, td has the highest percentage contribution (4.50%) compared to tC (0.94%) and dC (0.34%).
Table 4. ANOVA for the efficiency of Ni(II) adsorption on MCAC.
presents the surface plot for the removal efficiency of Ni(II) ion. It can be observed that increasing the dosage of MCAC and contact time favour the removal of Ni(II) ion from solution. This can be attributed to the fact that the number of active sites of MCAC increases when dosage is increased. The higher number of active sites favours more interaction time in parallel with the longer contact time, thus improving the removal efficiency of Ni(II) until the equilibrium is reached. Also, the highly Ni(II) removal efficiencies at higher MCAC dosages could be attributed to possibilities of unsaturated MCAC adsorption sites during Ni(II) removal (Gorzin & Abadi, Citation2017).
3.5. Process optimization
3.5.1. Central Composite Design (CCD) optimization
The goal of the optimization process was to maximize the percentage removal of Ni(II) within the experimental range of the independent variables studied in using CCD of design expert 12.0 software. shows the process parameter optimization cube plot for Ni(II) adsorption on MCAC. The optimum values of the process parameters for the maximum Ni(II) removal efficiency were determined to be 9.850 mg/L initial Ni(II) concentration (C), 118.693 min contact time (t) and 1.00 g MCAC dosage (d). The optimization was based on the highest desirability i.e its closeness to 1. At the predicted optimum conditions, the removal efficiency was given as 97.6154% (). This was validated experimentally referring to the predicted optimum conditions, and 96.9993% maximum experimental Ni(II) removal efficiency was achieved. These results show a reasonable agreement between the predicted and experimental Ni(II) removal efficiencies, and suggest a successful RSM.
3.5.2. Matlab optimization
It is important to simulate the response and behaviour of a system based on reliant input parameters. In this study the initial Ni(II) concentration, MCAC dosage and contact time were considered as the input parameters for the system modelling. Regression analysis was carried out to fit model for the experimental data. Correlation coefficient (R2) of the model developed was obtained as 0.9840 which implies a reasonably agreement of the data with the model, thus suitable for describing the process. Matlab code was used to develop the model shown in and the regression equation developed is given in EquationEquation 8(8)
(8) , where x1, x2 and x3 represent the initial Ni(II) concentration, the contact time and the MCAC dosage, respectively.
Optimization was carried out on the developed model to obtain the optimal conditions of the three variables studied within a desired range and initial conditions. The initial conditions set were x1 = 9.85, x2 = 120 and x3 = 0.8. The initial set point was set based on the highest response obtained from the experimental data that was fitted into the model. From the simulated response, the optimum adsorption conditions were obtained as: 9.75 mg/L initial Ni(II) concentration, 120 min contact time and 0.803 g MCAC dosage, these conditions gave 100% Ni(II) removal.
(8)
(8)
When validated experimentally under the predicted optimum conditions above, 97.113% maximum removal of Ni(II) was achieved. When compared with the result obtained from CCD, it can be inferred that there is a good correlation between the optimum process variables.
3.6. Adsorption kinetics
As a combination of kinetic model plot for Ni(II) adsorption, experimental, PFO, PSO and IpD data are shown in . Also, the parameters obtained from the non-linear model plots are given in . The most suitable model was selected based on the fitted coefficient of determination, R2. The higher R2 value, 0.9704 for PSO model than 0.9156 for PFO and 0.9191 for IpD signified that PSO model fitted better to the experimental data. Also, the evaluated qe value for PSO is the closest to that of experimental data. Hence, it can be inferred that Ni(II) adsorption on MCAC is chemisorption controlled (Oladipo, Ahaka, & Gazi, Citation2019).
Table 5. Ni(II) adsorption on MCAC kinetic models parameters.
4. Conclusion
This work has been carried out to study the adsorption of Ni(II) on an activated carbon prepared from maize cob. The results obtained indicated that the developed maize cob-derived activated carbon (MCAC) could be used as a highly effective adsorbent in removing Ni(II) ion from aqueous solution as an effective adsorbent. It was also discovered that contact time contributed more to the adsorption process (70.85%). Considering parameter interaction, the interaction between MCAC dosage and contact time gives the highest contribution (4.50%). Furthermore, 9.75 mg/L initial Ni(II) concentration, 120 mins contact time and 0.803 g adsorbent dosage were found to give complete removal of Ni(II) from the solution. The experimental data fitted better to pseudo-second-order kinetic and a chemisorption controlled adsorption process occurs. Therefore, the current study shows that maize cob which is an abundant agricultural waste is highly effective for the removal of toxic and carcinogenic Ni(II) from aqueous solution when activated with NaOH.
Interest declaration
No form of competing interest is related to this work.
Disclosure statement
No potential conflict of interest was reported by the author(s).
Correction Statement
This article has been corrected with minor changes. These changes do not impact the academic content of the article.
References
- Ahmed, A. Y., Yasser, A. M. A., Norhan, S. S., & Farida, M. S. E. (2019). Removal of cadmium ions from wastewaters using corn cobs supporting nano-zero valent iron. Separation Science and Technology, 56(1), 1–13. doi:10.1080/01496395.2019.1708109
- Arunkumar, C., Perumal, R., Lakshmi, N. S., & Arunkumar, J. (2014). Use of corn cob as low cost adsorbent for the removal of Nickel (II) from aqueous solution. International Journal of Advanced Biotechnology and Research(IJBR), 5(3), 325–330. http://www.bipublication.com.
- Bradl, H. (2002). Heavy metals in the environment: Origin, interaction and remediation. Vol. 6. London: Academic Press.
- Buekers, J., De Brouwere, K., Lefebvre, W., Willems, H., Vandenbroele, M., Van Sprang, P., … Oller, A. R. (2015). Assessment of human exposure to environmental sources of nickel in Europe: Inhalation exposure. The Science of the Total Environment, 521–522, 359–371. doi:10.1016/j.scitotenv.2015.02.092
- Buxton, S., Garman, E., Heim, K. E., Lyons-Darden, T., Schlekat, C. E., Taylor, M. D., & Oller, A. R. (2019). Concise review of Nickel human health toxicology and ecotoxicology. Inorganics, 7(7), 89. doi:10.3390/inorganics7070089
- Caglar, B., Afsin, B., Tabak, A., & Eren, E. (2009). Characterization of the cation-exchanged bentonites by XRPD, ATR, DTA/TG analyses and BET measurement. Chemical Engineering Journal and the Biochemical Engineering Journal, 149(1–3), 242–248. doi:10.1016/j.cej.2008.10.028
- Dada, A., Inyinbor, A., & Oluyori, A. (2012). Comparative adsorption of dyes unto activated carbon prepared from maize stems and sugar cane stems. Journal of Applied Chemistry, 2(3), 38–43. doi:10.9790/5736-0233843
- Dada, A. O., Ojediran, J. O., & Olalekan, A. P. (2013). Sorption of Pb2+ from aqueous solution unto modified rice husk: Isotherms studies, Hindawi Publishing Corporation. Advances in Physical Chemistry, 2013, 1–6. doi:10.1155/2013/842425
- European Union (EU). (2013). Directive 2013/39/EU of the European Parliament and of the Council. Off. J. Eur. Union, 226, 338–436.
- Fischer, L. A., Menné, T., & Johansen, J. D. (2005). Experimental nickel elicitation thresholds—A review focusing on occluded nickel exposure. Contact Dermatitis, 52(2), 57–64. doi:10.1111/j.0105-1873.2005.00523.x
- García, R., Pizarro, C., Lavín, A. G., & Bueno, J. L. (2012). Characterization of Spanish biomass wastes for energy use. Bioresource Technology, 103(1), 249–258. doi:10.1016/j.biortech.2011.10.004
- Garg, U. K., Kaur, M. P., Garg, V. K., & Sud, D. (2008). Removal of nickel (II) from aqueous solution by adsorption on agricultural waste biomass using a response surface methodological approach. Bioresource Technology, 99(5), 1325–1331. doi:10.1016/j.biortech.2007.02.011
- Gautam, R. K., Sharma, S. K., Mahiyab, S., & Chattopadhyaya, M. C. (2014). Heavy metals in water: Presence, removal and safety, royal society of chemistry. http://pubs.rsc.org. | doi:10.1039/9781782620174-00001
- Gorzin, F., & Abadi, M. M. B. R. (2017). Adsorption of Cr(VI) from aqueous solution by adsorbent prepared from paper mill sludge: Kinetics and thermodynamic studies. Adsorption Science & Technology, 0(0), 1–21.
- Hartmann, M., Khalil, S., & Bernet, T. (2012). Organic agriculture in Saudi Arabia. Section Study, 10, 1–48.
- Heidarinejad, Z., Dehghani, M. H., Heidari, M., Javedan, G., Ali, I., & Sillanpää, M. (2020). Methods for preparation and activation of activated carbon: A review. Environmental Chemistry Letters, 18(2), 393–415. doi:10.1007/s10311-019-00955-0
- Herrera-Barros, A., Tejada-Tovar, C., Villabona-Ortiz, A., Gonzalez-Delgado, A. D., & Alvarez-Calderon, J. (2018). Adsorption of Nickel and Cadmium by corn cob biomass chemically modified with alumina nanoparticles. Indian Journal of Science and Technology, 11(22), 1–11. doi:10.17485/ijst/2018/v11i22/126125
- Igwegbe, C. A., Onukwuli, O. D., Onyechi, K. K., & Ahmadi, S. (2020). Equilibrium and kinetics analysis on Vat Yellow 4 uptake from aqueous environment by modified rubber seed shells: Nonlinear modelling. Journal of Materials and Environmental Science, 11(9), 1424–1444.
- Igwegbe, C. A., Umembamalu, C. J., Osuagwu, E. U., Oba, S. N., & Emembolu, L. N. (2020). Studies on adsorption characteristics of corn cobs activated carbon for the removal of oil and grease from oil refinery desalter effluent in a downflow fixed bed adsorption equipment. European Journal of Sustainable Development Research, 5(1), em0145. doi:10.29333/ejosdr/9285
- Koksal, E., Afsin, B., Tabak, A., & Caglar, B. (2020). Butylamine-resadiye bentonite composite characterization. Spectroscopy Letters. 53(10), 745–750. doi:10.1080/00387010.2020.1832530
- Kussainova, M. Z., Chernyakova, R. M., Jussiphekov, U. Z., Temel, H., Pasa, S., Kaiybayeva, R. A., & Agatayeva, A. A. (2018). Sorption removal of Pb2+, Cd2+, Cu2+ from diluted acid solution by chitosan modified zeolite. Journal of Chemical Technology and Metallurgy, 53(1), 94–100.
- Lala, M. A., Kawu, S., Adesina, O. A., & Sonibare, J. A. (2022). Assessment of heavy metal pollution status in surface soil of a Nigerian University. Journal of the Nigerian Society of Physical Sciences, 4, 887. doi:10.46481/jnsps.2022.887
- Oladipo, A. A., Ahaka, E. O., & Gazi, M. (2019). High adsorptive potential of calcined magnetic biochar derived from banana peels for Cu 2 +, Hg 2 + and Zn 2 + ions removal in single and ternary systems. Environmental Science and Pollution Research International, 26(31), 31887–31899. doi:10.1007/s11356-019-06321-5
- Oller, A. R., Oberdörster, G., & Seilkop, S. K. (2014). Derivation of PM10 size-selected human equivalent concentrations of inhaled nickel based on cancer and non-cancer effects on the respiratory tract. Inhalation Toxicology, 26(9), 559–578. doi:10.3109/08958378.2014.932034
- Osasona, I., Aiyedatiwa, K., Johnson, J. A., & Faboya, O. L. (2018). Activated carbon from spent brewery barley husks for cadmium ion adsorption from aqueous solution. Indonesian Journal of Chemistry, 18(1), 145–152. doi:10.22146/ijc.22422
- Pérez, N. A., Rincón, G., Delgado, L. A., & González, N. (2006). Use of biopolymers for the removal of heavy metals produced by the oil industry –a feasibility study. Adsorption, 12(4), 279–286. doi:10.1007/s10450-006-0504-x
- Regti, A., Laamari, M. R., Stiriba, S. E., & E-Haddad, M. (2017). Use of response factorial design for process optimization of basic dye adsorption onto activated carbon derived from Perseaspecies. Microchemical Journal, 130, 129–136. doi:10.1016/j.microc.2016.08.012
- Reza, M. S., Yun, C. S., Afroze, S., Radenahmad, N., Bakar, M. S. A., Saidur, R., … Azad, A. K. (2020). Preparation of activated carbon from biomass and its’ applications in water and gas purification, a review. Arab Journal of Basic and Applied Sciences, 27(1), 208–238. doi:10.1080/25765299.2020.1766799
- Tan, I. A. W., Ahmad, A. L., & Hameed, B. H. (2008). Preparation of activated carbon from coconut husk: Optimization study on removal of 2,4,6-trichlorophenol using response surface methodology. Journal of Hazardous Materials, 153(1–2), 709–717. doi:10.1016/j.jhazmat.2007.09.014
- Tchounwou, P. B., Yedjou, C. G., Patlolla, A. K., & Sutton, D. J. (2014). Heavy metals toxicity and the environment. NIH-RCMI Center for Environmental Health, College of Science, Engineering and Technology, EXS, 101, 133–164. doi:10.1007/978-3-7643-8340-4_6
- Tejada-Tovar, C., Ortiz, A. V., Gonzalez, A. D., Cantillo, E. M., & Montero, M. P. (2018). Kinetics and adsorption equilibrium of linear alkylbenzene sulfonate in aqueous solution using corn residues in batch system. Indian Journal of Science and Technology, 11(13), 1–10. doi:10.17485/ijst/2018/v11i13/121426
- Thangamani, K. S., Sathishkumar, M., Sameena, Y., Vennilamani, N., Kadirvelu, K., Pattabhi, S., & Yun, S. E. (2007). Utilisation of modified silk cotton hull waste as an adsorbent for the removal of textile dye (Reactive Blue MR) from aqueous solution, Bioresour. Bioresource Technology, 98(6), 1265–1269. doi:10.1016/j.biortech.2006.05.010
- Yusuff, A. S., Popoola, L. T., & Babatunde, E. O. (2019). Adsorption of cadmium ion from aqueous solutions by copper‑based. Applied Water Science, 9(4), 106. doi:10.1007/s13201-019-0991-z
- Zafar, M. N., Aslam, I., Nadeem, R., Munir, S., Rana, U. A., & Khan, S. U. D. (2015). Characterization of chemically modified biosorbents from rice bran for biosorption of Ni(II). Journal of the Taiwan Institute of Chemical Engineers, 46, 82–88. doi:10.1016/j.jtice.2014.08.034
- Zhou, C., Vitiello, V., Casals, E., Puntes, V. F., Iamunno, F., Pellegrini, D., … Buttino, I. (2016). Toxicity of nickel in the marine calanoid copepod Acartia tonsa: Nickel chloride versus nanoparticles. Aquatic Toxicology, 170, 1–12. doi:10.1016/j.aquatox.2015.11.003