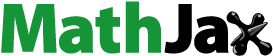
ABSTRACT
We collected tailings samples to assess and analyze the concentrations of heavy metals, pollution levels, and the risks posed by heavy metals in the tailings of the Zuoxiguo antimony mine to ecological and human health. The samples were selected by employing the serpentine sampling method, which were treated heavy-metal digestion method. Cu, Cr, Cd, and Pb were determined via inductively coupled plasma mass spectrometry, As and Sb were identified through atomic fluorescence, the average concentration of heavy metals in the tailings is: Sb (5902.77 mg/kg)>As (412.53 mg/kg)>Zn (155.42 mg/kg)>Cr (143.32 mg/kg)>Cu (92.68 mg/kg)>Pb (48.49 mg/kg)>Cd (3.29 mg/kg). The average Nemerow pollution index (NPI) was 553.06, indicating a high level of pollution. The average integrated ecological risk index (RI) result was 5395.94, indicating a serious threat to the study area’s ecology. The Non-carcinogenic health-risk index (HQ) suggested that all heavy metals posed a higher risk to children than to adults under different modes of exposure, with oral intake of Sb and As posing a non-carcinogenic risk to both adults and children, For adults, HQing value of Sb and As are 22.07 and 2.13, respectively; for child, HQing value of Sb and As are 178 and 16.29, which are exceeding limitation of 1. Dermal exposure posing a non-carcinogenic risk to child, for child, HQderm value of Sb and As are 14.96 and 3.32, which are also exceeding limitation of 1. The findings suggest that Sb and As pose serious threats to both the ecological environment and human health and that the local government should focus on Sb and As management for regions.
Introduction
Exploration, exploitation, and utilization of mineral resources have driven China’s rapid economic growth in recent decades [Citation1]. Although mining has been advantaged for economic development, this industrial activity has caused severe environmental pollution, especially in terms of heavy metals [Citation2–4]. Heavy metals are metals and metalloids with densities ≥ 5 g/cm3 [Citation2,Citation5]. However metalloids such as As are classified as heavy metals due to chemical similarities and environmental behavior [Citation6]. Heavy-metal pollution in the environment is a persistent phenomenon, difficult to degrade, and irreversible in nature [Citation7]. A nation-wide assessment of 1,672 soil sites in 70 mining locations was undertaken by the State Environmental Protection Administration in 2012, revealing that 33.4% of the sites exceeded the allowable limit [Citation8]. With the primary pollutants as cadmium, lead, arsenic, and polycyclic aromatic hydrocarbons. Among them, Cd, Pb and As are the substantial metals near the non-ferrous metal mining sites [Citation9]. Particularly, if heavy-metal concentrations are higher than safe levels in a particular location, then the ecosystem of the mining region and the quality and production of nearby crops are considerably degraded. Moreover, increased concentrations of these pollutants pose a serious threat to human health owing to bioaccumulation through the food chain [Citation10,Citation11].
Heavy metals are elements that are non-essential to the human body; these elements influence human health directly or indirectly through media such as water and soil. In this regard, studies have discovered that approximately 94 to 220 million people across the globe are potentially at risk from high concentrations of arsenic in groundwater [Citation12]. Citation13,investigated gold-mining regions in the eastern Amazon, revealing that the mining environment was highly contaminated owing to high levels of As enrichment and contamination. Reportedly, the non-carcinogenic risk-index results were as high as 17.8, well above the acceptable limit of 1, including high carcinogenic risk to children and adults. In addition, certain concentrations of Sb were found in the hair and nails of residents in close proximity to an active antimony mine in Hunan Province, China; these high concentrations were mainly attributed to mining activities [Citation14]. Notably, mining activities are a major source of heavy metal pollution [Citation15]. Numerous studies have shown that pollution by heavy metals is widespread, especially in the regions featuring mining activities [Citation16]. For example, studies focusing on soils surrounding a Pb-Zn mine in Yunnan Province, China, found that the main sources of As, Cd, and Pb were mining activities, airborne particles from the smelter, and weathering of tailings [Citation17]. Considering this aspect, by extensively investigating heavy metals in soils around mining areas and evaluating the risk assessment of the heavy metals to the population and property in the area, pertinent management strategies related to mining areas can be suggested to the local government. These measures are essential for supporting the environmental and ecological health of the area.
Karst landforms are widely distributed in Yunnan Province, and the scarce soil resources in Yunnan Province exhibit significant spatial heterogeneity, yielding a vulnerable ecosystem [Citation18,Citation19]. Considering this aspect, we selected the Zuoxiguo antimony mine in Yunnan Province as the study area (1) to survey the total concentration of heavy metals; (2) the contamination level of each heavy metal in the study area was analyzed by using the Nemerow index method. Moreover, (3) the potential risk posed by heavy metals to the mining environment was evaluated via the potential ecological risk-index; the non-carcinogenic risk as well as the carcinogenic risk to adults and children were evaluated for different exposure scenarios.
Materials and methods
Study area
Zuoxiguo antimony mine (23°39′20′′N, 103°34′11′′ E) is located in Zuoxiguo village, Beige township, Kaiyuan City. Honghe Hani and Yi Autonomous Prefecture, Yunnan Province. This location is in close proximity to the tropic of Cancer, 81.5 km from downtown and 13.5 km from National Highway 323. The sampling site of Zuoxiguo antimony mine was established, with water flowing in the tailing direction of the mine ().
Sample collection and treatment
The tailings samples were selected by employing the serpentine sampling method, with every sampling point featuring a sample square measuring 100 cm×100 cm. Surface tailings weighting at least 1000 g and measuring 0–30 cm in length were collected from each sample square using a stainless-steel shovel via the double diagonal 5-point mixing method. Subsequently, information regarding the sampling point and the surrounding environment was recorded and labeled onto the samples, which were transferred to the laboratory for testing. The resulting tailings were left to dry in the air, and the sample pieces (0.05 g) were accurately weighed and dissolved in a 3-mL acid mixture comprising a concentrated HNO3 and concentrated HF (volumetric ratio: 2:1). The samples, mixed in this HNO3-HF solution, were placed into a digestion tank, heated for 48 hours at 180°C, and left undisturbed overnight for digestion. After digestion, the dissolved liquid was diluted to 50 ml in a volumeter bottle for machine analysis. The Cu, Cr, Cd, and Pb concentrations were measured through inductively coupled plasma mass spectrometry (ICP-MS, iCAP-RQ) and As and Sb were determined by atomic fluorescence (AFS, AFS-9700). A PHS-3c pH meter was leveraged to continuously measure the tailing supernatant for three iterations, and the average value was recorded as the pH value of the tailing sample [Citation20]. In accordance with the standard for determining heavy metals in soil (HJ680-[Citation21]2013), a total of 6 samples to be analyzed were inserted using a blank sample, in accordance with the 10% standard; thus, a total of one blank sample was inserted. During the analysis, procedural gaps, parallel experiments, and soil samples (obtained as per national standard GSS-4) were considered for quality control. The calibration curves of Sb and As standard solutions possessed determination coefficients exceeding 0.999, and the recoveries rates were between 80.0 and 105.0%. Ultrapure water was used as the experimental water, and all reagents utilized were guaranteed reagents. The container was soaked in 10% HNO3 solution for more than 24 hours, rinsed with ultra-pure water, and dried for use.
Assessment Methods
Nemerow Pollution Index Method
The Nemerow pollution index represents the pollution level of a single heavy-metal element present in the tailings and can also be used to evaluate the combined pollution level of multiple heavy metals [Citation22], The calculation formula is expressed as follows:
In the given formulae, Pi denotes the pollution index of the heavy metal element in tailings, Ci represents the measured value of the heavy metal in soil or crop (mg·kg−1), Si expresses the evaluation standard of heavy metal in tailings (mg·kg−1), NPI indicates the Nemerow integrated pollution index, Pave symbolizes the average value of single pollution index corresponding to a heavy metal, and Pmax represents the maximum value of single pollution index corresponding to a heavy metal [Citation15]. The grading criteria of the Nemerow comprehensive pollution index are listed in .
Table 1. Classification criteria of the pollution index method.
Potential Ecological Risk Index Method
The potential ecological risk index based on the interaction between the physicochemical properties of heavy metals and the environment to evaluate the degree of heavy metal pollution and its potential ecological hazards [Citation24]. The calculation formula is expressed follows:
Where RI denotes the comprehensive ecological risk index, ERI indicates the potential ecological risk index of heavy metals, Ti represents the toxicity response coefficient of heavy metals, is the measured value of the heavy metal (mg·kg−1),
symbolizes the background value of the heavy metal (mg·kg−1). The toxicity response coefficients of heavy metals Cu, As, Cd, Cr, Zn, Sb, and Pb are 5, 10, 30, 2, 5, 10, and 5, respectively, and the evaluation criteria of the potential ecological risk index are shown in .
Table 2. Classification criteria of potential ecological hazards.
Health risk Index Method
The health-risk evaluation model, recommended by the U.S. Environmental Protection Agency, was employed to evaluate the carcinogenic and non-carcinogenic health risks posed to adults and children in the study area [Citation26]. The average daily intake of heavy metals for children and adults via the three exposure routes considered were calculated using the following equations.
The non-carcinogenic-risk and carcinogenic-risk indices of heavy metals to humans are calculated as follows:
In the formulae, ADD represents the average daily intake of heavy metals (mg·kg·d −1), and RfD indicates the reference daily dose via ingestion (mg kg −1, day −1). The representations and values of the parameters utilized in the formulae are presented in and .
Table 3. Health risk assessment exposure parameters.
Table 4. Model parameters RfD and SF values.
HQ is the possible non-carcinogenic risk caused by a single toxic element to human health, while HI is the possible comprehensive non-carcinogenic risk caused by all toxic elements to human health. If the values of HQ or HI < 1, it indicates no significant risk of non-carcinogenic effects. If the values of HQ or HI > 1, it indicates a great chance of non-carcinogenic effects [Citation27].
Data analysis
ArcGIS 10.8 software was employed to generate the distribution of the study area, and software-based plots were drawn using Excel 2013 and Origin 2021.
Result and discussion
Heavy-metal concentrations in tailings
The concentrations of heavy metals in the tailings obtained from the Zuoxiguo antimony mine are listed in , the average concentration of heavy metals in the tailings is: Sb (5902.77 mg/kg)>As (412.53 mg/kg)>Zn (155.42 mg/kg)>Cr (143.32 mg/kg)>Cu (92.68 mg/kg)>Pb (48.49 mg/kg)>Cd (3.29 mg/kg). The average concentration of all heavy metals is higher than the background value for Yunnan Province, among which the average exceedance rates of Sb, As, and Cd are 99.87%, 96.39%, and 97.57% respectively. This trend mainly results from the complex and chaotic management mode employed in the Zuoxiguo antimony mine; group mining is commonly practiced, and the average concentrations of Sb, As, and Cd in the tailings are extremely high owing to the influence of large-scale mining activities. Additionally, the study area is located in the karst region of southwest China, which experiences a warm and rainy climate; the rainfall type is primarily acid rain, with a fragile ecological environment [Citation34]. The average pH of the tailing sand is 3.44, and the low pH environment also contributes to the migration and transformation of heavy metals, causing severe pollution in the study area [Citation17].
Table 5. Concentrations distribution of heavy metals in tailings obtained from Zuoxiguo antimony mine (mg/kg).
As listed in , the coefficients of variation of heavy metals in the tailing sands are in the following order: Pb (105%) > Sb (73%) > As (64%) > Cd (26%) ≥ Cu (26%) > Zn (16%) > Cr (13%). The coefficient of variation of Pb in the tailing sands is 105%, exhibiting anomalously strong variability. Moreover, the coefficients of variation of Sb and As are 73% and 64%, which represent strong variability, indicating that As, Sb, and Pb concentrations in the tailing samples of the study area are strongly influenced by human activities [Citation35]. Presumably, the frequent mining activities conducted in the study area and the combination of the open topography of the study area with the influence of acid rain led to the severe exceedance of Sb, As, and Cd in the tailing samples of the study area [Citation36]. In contrast, the coefficients of variation for Cr (13%), Zn (16%), Cu (26%), and Cd (26%) are low, indicating limited influence of human activities and probably greater influences of natural factors such as ore weathering.
Principal Component Analysis (PCA)
The sources of heavy metals in tailings are influenced by both anthropogenic and natural factors, and a concomitant or homologous relationship is generally observed between heavy metals, which may result from the similarities in their sources [Citation37]. Therefore, a principal component analysis was performed for heavy metals in tailings to explore the sources of heavy-metal pollution in the study area. The results in demonstrate that the cumulative contribution of principal component 1 and principal component 2 reached 78%. This result indicates that the extracted principal components exhibit a high degree of explanation for the original variables; specifically, these two principal components maximally the logical representation and information contained within the total data. As illustrated in , the contents of As, Zn and Pb in the tailings are affected by human activities, whereas those of Sb, Cr, Cu, Cd, and pH are affected by natural factors. However, this result does not imply that the contents of As, Zn, and Pb in tailings are not affected by natural factors and that the contents of Sb, Cr, Cu, and Cd as well as pH in tailings are not affected by human activities. Moreover, the average pH of the tailings is 3.44, and this low pH value is conducive to the dissolution, migration, and transformation of heavy metals. Therefore, the sources and influencing factors of heavy metals in the tailings of Zuoxiguo are an important aspect to be considered in the subsequent research focusing on this region.
Nemerow pollution index method
illustrates the results of the single-factor pollution mean index (PI) and the Nemerow pollution index (NPI) corresponding to the study area. The PI values of all heavy metals in the tailings of the Zuoxiguo antimony mine indicate different levels of exceedances of heavy metals in the region. The average PI values of heavy metals in the tailings are in the following order: Sb (772.61) > Cd (41.10) > As (30.00) > Cu (2.46) > Cr (2.06) > Zn (1.75) > Pb (1.33), of which those corresponding to Sb, As, and Cd are severe, suggesting that the pollution level is extremely serious. In addition, Zn, Cr, Pb, and Cu exhibit minor amounts of exceedance. The average NPI value of Zuo Xiguo antimony mine tailings is 553.06, of which the share of Sb, As, and Cd contributions to the NPI value are 93%, 2.9%, and 3.4%, respectively, and the overall pollution level is extremely grave. Considering the forementioned results, the local government and environmental protection agencies should devote more attention to the treatment of Sb, As, and Cd pollution. In particular, the Sb pollution in the environment around the antimony mine should be addressed immediately. Although the contribution of other heavy metals to the NPI value does not exceed 1%, the PI values clearly indicate that Zn, Cr, Pb, and Cu exhibit different degrees of exceedance and cannot be ignored. Overall, as revealed by the resulting PI and NPI values, the heavy metals in the tailings of the Zuoxiguo antimony mine have polluted the mine environment to different degrees, and among these pollutants, the pollution caused by Sb, Cd, and As requires urgent remediation.
Potential ecological risk assessment
The tailings contain a large number of toxic or persistently toxic hazardous metals, which considerably harmful to humans. A total of seven toxic elements: Sb, As, Zn, Cr, Pb, Cu, and Cd, were evaluated for their potential ecological risk. As demonstrated in , average ERI values of heavy metals in the tailings of the Zuoxiguo antimony mine are: Sb (3861.11)> Cd (1233.13)> As (276.87)> Cu (12.33)> Pb (6.64)> Cr (4.12)> Zn (1.75). In particular, Sb and Cd exhibit serious potential risks to the mine environment (both >320). The ERI values of Sb and Cd are 3861.11 and 1233.13, and their contributions to the RI values are 71.6% and 22.9%; this trend may be related to the toxicity coefficients and bioavailability of Sb and Cd, especially those of Cd, which is more likely to be enriched in the ecosystem, thereby posing a relatively higher potential ecological risk to the ecosystem [Citation38]. In addition, As exhibited a high potential ecological risk to the mine environment, featuring an ERI value of 276.87. In contrast, the potential ecological risk values of Zn, Cr, Pb, and Cu were under 40, representing a low potential risk that could manifest as a small environmental risk with a negligible contribution to the RI value. Moreover, the RI value of Zuoxiguo antimony mine tailings is 5359.93, which is much larger than highest limitation of 1200 (). These results suggest that the heavy metals in the study area pose an extremely high integrated ecological risk to the mine environment. Moreover, considering the similarities and differences in the toxicity index of heavy metals, certain differences were observed between the potential ecological risk and the Nemerow pollution-index assessor. Nevertheless, among the heavy metals present in the study, Sb, Cd, and As require urgent attention; these elements not only cause serious pollution but also pose an extremely high risk to the mining environment.
Health risk assessment
The Individual non-carcinogenic health risk index (HQ) and total non-carcinogenic risk index (HI) of heavy metals for adults and children exposed via oral intake, dermal contact, and inhalation are summarized in and . The risks posed by different uptake mechanisms are arranged as follows: Ingestion > dermal contact > inhalation. For adults, HQing value of Sb and As are 22.07 and 2.13, respectively. For child, HQing value of Sb and As are 178 and 16.29, respectively, which are exceeding limit of 1 (). In particular, the HQing values of Sb and As for children are higher than those for adults, indicating that the Sb and As in tailings pose a greater threat to children than to adults. Moreover, the HQ values of Sb and As for adults, characterizing uptake through oral ingestion, are 22.07 and 2.13, respectively, both are higher than unity, indicating that Sb and As pose a non-carcinogenic risk for adults when ingested orally. Furthermore, the HQing and HQderm values of Sb are 178 and 14.96, respectively, indicating that Sb pose a non-carcinogenic risk to children through oral ingestion and dermal contact. In contrast, for adults and children at risk through oral ingestion, respiratory inhalation, and dermal exposure to Zn, Cr, Pb, Cu, and Cd, the HQ values are less than 1, which are within the acceptable range and do not pose a non-carcinogenic risk. The HI values for adults are greater than 1. Sb contributed 91% to the HI values, indicating that Sb is the primary heavy metal posing the highest non-carcinogenic risk to adults via oral intake. In cases of oral and dermal exposures in children, the HI values are 195.1 and 18.37, respectively, both of which are greater than 1. Moreover, Sb contributed to 91% and 81% of the HI values for oral and dermal exposures, respectively, whereas As contributed to 7% and 18% of the HI values upon oral and dermal exposures, respectively. These results validate that through oral and dermal exposures, Sb and As are the main heavy metals that maximally contribute to the non-carcinogenic risk posed to children. The HI values for all heavy metals via inhalation and dermal exposure are less than 1 for both adults and children, which are within acceptable limits. Judging by the result, these heavy metals did not pose a non-carcinogenic risk for either adults or children via inhalation and dermal exposure.
Table 6. Risk of non-carcinogenic exposure to heavy metals in Zuoxiguo antimony mine tailings.
The individual carcinogenic health-risk indices (CR) and total carcinogenic health-risk indices (TCR) of heavy metals are presented in and . The severity of carcinogenic risk posed by heavy metals in the tailings of the Zuoxiguo antimony mine to adults and children through three exposure routes are in the following order: oral intake > dermal contact > inhalation (). Particularly, the CR values of different exposure modes are higher for children than those for adults, indicating that the carcinogenic risk posed by heavy metals to children is higher than that posed to adults. The CR values of As for both adults and children after oral ingestion exceed 10 −4, indicating the carcinogenic risk posed by As to both adults and children after oral ingestion. Moreover, upon dermal exposure to As, the CR values for both adults and children lie between 10 −6 and 10 −4, clearly suggesting the potential carcinogenic risk. In contrast, the CR values of Pb for adults and children after oral ingestion and the CR values of As, Cd, and Cr after respiratory inhalation are less than 10 −6, which lie within the acceptable range for these values. Furthermore, the TCR after oral ingestion is greater than 10 −4 for both adults and children, and the TCR after inhalation is less than 10 −6 for both adults and children. Observably, the contributions of As to the TCR values upon oral ingestion by adults and children are 96% and 94%, respectively. This result clearly demonstrates that As is the main pollutant presenting the carcinogenic health risk.
Table 7. Risk of carcinogenic exposure to heavy metals in Zuoxiguo antimony mine tailings.
Conclusion
In this research, tailings were obtained from the Zuoxiguo antimony mine and analyzed to determine the concentration of the heavy metals in the tailings. Following the relevant risk assessment, the following main conclusions were drawn: (1) heavy metals in the tailings of Zuoxiguo antimony mine exceeded the background value by varying degrees. The average concentration of heavy metals in the tailings is Sb (5902.77 mg/kg)>As (412.53 mg/kg)>Zn (155.42 mg/kg)>Cr (143.32 mg/kg)>Cu (92.68 mg/kg)>Pb (48.49 mg/kg)>Cd (3.29 mg/kg). Among these heavy metals, the average exceedance rates of Sb, As, and Cd were 99.87%, 96.39%, and 97.57% respectively. (2) The average single-factor index results revealed that the pollution levels of Sb (772.61), As (30.00), and Cd (41.10) in the study area were gravely serious. Moreover, the average Nemerow pollution index result was 553.07, which showed serious pollution-levels. In this regard, Sb contributed to 93% of the NPI value, which was determined to be the main polluting heavy metal in the studied area. (3) As indicated in the average ERI-value results, Sb (3861.11), As (276.87), and Cd (1233.13) posed a high risk to the ecology of the mining area. The average potential ecological integrated-risk index (RI) result was 5395.94, which indicated an extreme risk to the ecology of the study area. In this perspective, Sb and Cd, as the highest risk-posing heavy metals in the region, contributed to 71.6% and 22.9% of the overall RI value, respectively. (4) The HQ results showed that the threat of heavy metals to children was significantly higher than that to adults under different modes of exposure. For adults, HQing value of Sb and As are 22.07 and 2.13, respectively; for child, HQing value of Sb and As are 178 and 16.29, respectively, which are exceeding limit of 1; for child, HQderm value of Sb and As are 14.96 and 3.32, which are also exceeding limitation of 1. Moreover, the HI results revealed Sb value to be 24.31, which was the elements posing the major non-carcinogenic risk. Furthermore, CR results suggested that a carcinogenic risk for adults and children under oral ingestion exposure for As, for adult and child, CRing value of As was 3.30E-04 and 3.70E-04; a potential carcinogenic risk to adults and children under oral ingestion exposure for Cd, for adult and child, CRing value of As was 1.10E-05 and 2.06E-05; and a potential carcinogenic risk to children only under oral ingestion exposure for Cr (1.25E-06).In addition, potential carcinogenic risk to both adults and children was identified for dermal-contact exposure to As. Overall, the TCR results indicated that As was the major carcinogenic contaminant via oral exposure.
Contributions
All authors provided support and contributions to the conception and design of this article. Sample collection and data collection were conducted by Ziyou Bai and Yinping He. Manuscript writing was completed by Ziyou Bai, and constructive comments from Zhiwei Han and Fuzhong Wu guided the research process. All authors read and approved the final manuscript.
Ethics approval and consent to participate
Not applicable.
Consent for publication
All authors agree to publish this research (including any individual details, images, or videos) in Environmental Pollutants and Bioavailability.
Data availability
The datasets supporting the relevant research findings in this article are available from the corresponding author (Zhiwei Han) through reasonable request.
Disclosure statement
The authors declare that they have no known competing financial interests or personal relationships that could have appeared to influence the work reported in this paper.
Additional information
Funding
References
- Sun X, Liu Y, Guo S, et al. Interregional supply chains of Chinese mineral resource requirements. J Clean Prod. 2021;279(2–4):123514.
- Herath I, Vithanage M, Bundschuh J. Antimony as a global dilemma: geochemistry, mobility, fate and transport. Environ Pollut. 2017;223:545–11.
- Huang Y, Wang L, Wang W, et al. Current status of agricultural soil pollution by heavy metals in China: a meta-analysis. SciTotal Environ. 2019;651(2):3034–3042. (PT.2.
- Yang H, He M. Distribution and speciation of selenium, antimony, and arsenic in soils and sediments around the area of xikuangshan (China). CLEAN-Soil Air Water. 2016;44(11):1538–1546
- Azimi A, Azari A, Rezakazemi M, et al. Removal of heavy metals from industrial wastewaters: a review. Chem Bio Eng Rev. 2017;4(1):37–59.
- Yudovich YE, Ketris MP. Arsenic in coal: a review. Int J Coal Geol. 2005;61(3–4):141–196.
- Yang Q, Li Z, Lu X, et al. A review of soil heavy metal pollution from industrial and agricultural regions in China: pollution and risk assessment. SciTotal Environ. 2018 nov 15;642:690–700.
- Fei X, Lou Z, Xiao R, et al. Contamination assessment and source apportionment of heavy metals in agricultural soil through the synthesis of PMF and GeogDetector models. SciTotal Environ. 2020;747:141293.
- W P L 2013. Ministry of environmental protection of the people’s republic of China.
- Xu DM, Fu RB, Liu HQ, et al. Current knowledge from heavy metal pollution in Chinese smelter contaminated soils, health risk implications and associated remediation progress in recent decades: A critical review. J Clean Prod. 2021;286:124989.
- Yan J, Qu Z, Li F, et al. Heavy metals in the water environment of Yangtze River Economic Belt: Status, fuzzy environmental risk assessment and management. Urban Climate. 2021;40:100981.
- Podgorski J, Berg M. and Berg M.Global threat of arsenic in groundwater. Science. 2020;368(6493):845–850.
- Neto H, Pereira W, Yan ND, et al. Environmental and human health risks of arsenic in gold mining areas in the eastern Amazon. Environ Pollut. 2020;265(B):114969.
- Cui H, Wen J, Yang L, Wang, Q . Spatial distribution of heavy metals in rice grains and human health risk assessment in Hunan Province, China. Environmental Science and Pollution Research. 2022;29(55):83126–83137.
- Liu X, Bai Z, Shi H, et al. Heavy metal pollution of soils from coal mines in China. Nat Hazards. 2019;99(2):1163–1177.
- Zehang S, Xiande X, Ping W, et al. Heavy metal pollution caused by small-scale metal ore mining activities: a case study from a polymetallic mine in South China. SciTotal Environ. 2018 oct 15;639:217–227.
- Cheng X, Danek T, Drozdova J, et al. Soil heavy metal pollution and risk assessment associated with the Zn-Pb mining region in Yunnan, Southwest China. Environ Monit Assess. 2018;190(4):194.
- Chen W, Zhang X, Huang Y. Spatial and temporal changes in ecosystem service values in karst areas in southwestern China based on land use changes. Environ Sci Pollut Res. 2021;28(33):45724–45738.
- Guo B, Zang W, Luo W. Spatial-temporal shifts of ecological vulnerability of Karst Mountain ecosystem-impacts of global change and anthropogenic interference. SciTotal Environ. 2020;741:140256.
- Luo G, Han Z, Xiong J, et al. Heavy metal pollution and ecological risk assessment of tailings in the Qinglong Dachang antimony mine, China. Environ Sci Pollut Res. 2021;28(25):33491–33504.
- HJ 680-2013 Soil and Sedimen-Determination of mercury,arsenic,selenium,bismuth,antimony-Microwave dissolution/Atomic Fluorescence Spectrometry.
- Li L, Wu J, Lu J, et al. Distribution, pollution, bioaccumulation, and ecological risks of trace elements in soils of the northeastern Qinghai-Tibet Plateau. Ecotoxicol Environ Saf. 2018a DEC;166:345–353.
- Zhu G, Guo Q, Xiao H, et al. Multivariate statistical and lead isotopic analyses approach to identify heavy metal sources in topsoil from the industrial zone of Beijing Capital Iron and steel Factory. Environ Sci Pollut Res. 2017;24(17):14877–14888.
- Wang N, Wang A, Kong L, et al. Calculation and application of Sb toxicity coefficient for potential ecological risk assessment. SciTotal Environ. 2018 jan 1;610-611:167–174.
- Mamut A, Eziz M, Mohammad A, et al. The spatial distribution, contamination, and ecological risk assessment of heavy metals of farmland soils in Karashahar–Baghrash oasis, northwest China. Hum Ecol Risk Assess. 2017;23(6):1300–1314.
- Li Z, Ma Z, van der Kuijp TJ, et al. A review of soil heavy metal pollution from mines in China: pollution and health risk assessment. Sci Total Environ. 2014;468:843–853.
- Ma JJ, Yan Y, Chen XJ, et al. Incorporating bioaccessibility and source apportionment into human health risk assessment of heavy metals in urban dust of Xiamen, China. Ecotoxicol Environ Saf. 2021;228:112985.
- Yu G, Chen F, Zhang H, et al. Pollution and health risk assessment of heavy metals in soils of Guizhou, China. Ecosyst Health Sustain. 2021;7(1):1859948.
- Zhaoyong Z, Xiaodong Y, Shengtian Y. Heavy metal pollution assessment, source identification, and health risk evaluation in Aibi Lake of northwest China. Environ Monit Assess. 2018;190(2):69.
- Gao J, Wang L. Ecological and human health risk assessments in the context of soil heavy metal pollution in a typical industrial area of Shanghai, China. Environ Sci Pollut Res. 2018;25(27):27090–27105.
- Baltas H, Sirin M, Gökbayrak E, et al. A case study on pollution and a human health risk assessment of heavy metals in agricultural soils around Sinop Province, Turkey. Chemosphere. 2020;241:125015.
- Sawut R, Kasim N, Maihemuti B, et al. Pollution characteristics and health risk assessment of heavy metals in the vegetable bases of northwest China. SciTotal Environ. 2018;642:864–878.
- Mamat A, Zhang Z, Mamat Z, et al. Pollution assessment and health risk evaluation of eight (metalloid) heavy metals in farmland soil of 146 cities in China. Environ Geochem Health. 2020;42(11):3949–3963.
- Zhang Q, Wang C. Natural and human factors affect the distribution of soil heavy metal pollution: a review. Water Air Soil Pollut. 2020;231(7):1–13.
- Chakraborty B, Bera B, Roy SH, et al. Assessment of non-carcinogenic health risk of heavy metal pollution: evidences from coal mining region of eastern India. Environ Sci Pollut Res. 2021;28(34):47275–47293.
- Tepanosyan G, Sahakyan L, Belyaeva O, et al. Continuous impact of mining activities on soil heavy metals levels and human health. SciTotal Environ. 2018 oct 15;639:900–909.
- Sawut R, Tiyip T, Abliz A, et al. Using regression model to identify and evaluate heavy metal pollution sources in an open pit coal mine area, Eastern Junggar, China. Environ Earth Sci. 2017;76(24):822.
- Hua C, Zhou G, Yin X, et al. Assessment of heavy metal in coal gangue: distribution, leaching characteristic and potential ecological risk. Environ Sci Pollut Res. 2018;25(32):32321–32331.