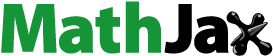
ABSTRACT
The indoor simulation remediation of chromium-contaminated soil in semi-arid areas with chemical reduction-stabilization method was conducted to study the effects of remediation agent types and dosage, water (mL)-soil (g) ratio and remediation time on reduction and stabilization efficiency of the chromium-contaminated soil. The results showed that FeSO4 was the most effective reduction agent for reduction of Cr(VI) in soil. The optimally combined chemical reduction-stabilization remediation conditions were 2.5 times theoretical reaction dosage FeSO4 and 8% of modified corn stover biochar (KBC), 50% of water-soil ratio, and 7 days remediation time. The total Cr and Cr(VI) leaching concentrations and Cr(VI) content in soil were reduced from 65.65 mg L-1, 61.98 mg L-1 and 1000.00 mg kg-1 without treatment to 0.114 mg L-1, 0.125 mg L-1 and 18.909 mg kg-1 after treatment, respectively. They were according with comprehensive wastewater discharge standards and construction land soil contamination risk control standards, respectively.
Graphical abstract
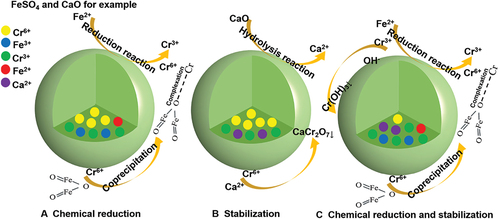
1. Introduction
Chromium (Cr) is a common heavy metal element in the natural environment and an important raw material in industrial processes. Cr and its compounds are widely used in electroplating, tanning, printing and dyeing, metallurgy, metal processing, pigment manufacturing, chemical pharmaceutical, and other industries [Citation1–4]. However, the emission of Cr-containing waste liquid, gas, and sludge produced by these industries during the production process may lead to soil and groundwater contamination in the surrounding areas [Citation5]. The study showed that the Cr content in Chinese arable soils ranged from 0.05 to 3353.60 mg kg−1, with an average Cr content of 78.94 mg kg−1, which was significantly higher than its background value (57.30 mg kg−1), indicating that human activities have introduced it into the soil environment [Citation6]. According to the National Soil Pollution Investigation Bulletin in 2014, of which chromium pollution in China exceeds the risk value in the national soil sampling points by 1.1% [Citation7]. In the soil environment, Cr usually exists in two dominant states: trivalent chromium (Cr(III)) and hexavalent chromium (Cr(VI)). Cr(III) usually exists in the form of hydroxides and oxides or is adsorbed onto clay particles, soil organic matter, metal oxyhydroxides, and so on, hindering its migration into soil and groundwater [Citation8]. On the contrary, Cr(VI) exists mainly as relatively soluble oxygen-containing anions such as HCrO-4, CrO2-4, and Cr2O2-7. Compared with Cr(III), Cr(VI) has greater solubility, stronger activity, and is easy to migrate in soil, so it is more toxic [Citation9]. Many studies [Citation10] have reported that Cr(VI) is 100 times more toxic than Cr(III), and Cr(VI) is easily absorbed by the human body and accumulates in the body. Long-term exposure to Cr(VI) may cause cancer, and the U.S. Environmental Protection Agency identified Cr(VI) as a Class A carcinogen [Citation11]. Therefore, high priority must be given to the remediation of chromium-contaminated soil.
At present, there are two main methods to treat chromium-contaminated soil: one is to remove chromium directly from the contaminated soil, and the other is to change the form of chromium present in the soil. Reduction of Cr(VI) with high toxicity and high migration capacity to Cr(III) with low toxicity and low migration capacity, and Cr(III) was precipitated to reduce the toxicity, bioavailability, and migration ability of Cr(VI) in the natural environment. Based on the above treatment methods, researchers have proposed a variety of remediation techniques for chromium-contaminated soil, such as chemical reduction technology, solidification/stabilization technology, chemical leaching technology, electrokinetic remediation technology, and bioremediation technology [Citation12–16]. Among them, chemical reduction technology is the most widely used treatment method for chromium-contaminated soil at home and abroad due to its advantages of simple operation, obvious treatment effect, and low cost [Citation17]. Yang et al. [Citation18] provided a detailed classification of reducing agent types, and common reducing agents include iron-based reducing agents (e.g. zero-valent iron and ferrous salts), sulfur-based reducing agents (e.g. polysulfide compounds and thiosulfates, etc.), and organic materials (e.g. biochar and humic acid). Lu et al. [Citation19] were able to achieve 70% and 87% stabilization ratios for industrial chromium slag using FeSO4 and Na2S as reducing agents, respectively. Li et al. [Citation20] found experimentally that Fe(NH4)2(SO4)2 effectively remediated soil contaminated with Cr (VI) and, acted as a fertilizer by supplying nitrogen because it contains ammonium.
Chemical reduction technology is usually used in combination with stabilization technology to remediate chromium-contaminated soil by combining reducing agents and stabilizers [Citation21–24], so as to achieve the purpose of green remediation such as reducing the dosage of reducing agents and reducing the influence of reducing agents on the soil. Biochar is produced from carbon-rich biomass by pyrolysis under oxygen-limited conditions [Citation25]. Some studies have reported that due to its highly developed porosity, high degree of surface reactivity, and excellent adsorption capacity [Citation26–28], biochar has been increasingly recognized as the great potential to remove heavy metals [Citation29,Citation30], especially for Cr(VI) with highly redox activity [Citation31–33] in soil. Raw biochar has a limited adsorption capacity for a high concentration of Cr(VI), and modification of biochar is needed to improve the adsorption capacity. Among the various modification methods [Citation34–37], alkali-modification biochar can increase the specific surface area, change pH, introduce more oxygen-containing functional groups, and can facilitate the removal of anionic Cr(VI) by increasing the positive charge on the biochar surface [Citation38]. At present, the mechanism of long-term stability of chromium-contaminated soil after remediation is still under study. It is the key to effectively improve the stability of remediation soil and ensure the optimal chromium endowment speciation in soil. With the rapid development of agriculture and industry, chromium contamination in the soil of the semi-arid northwest areas to deepen. However, there have been no reports on chemical reduction agents combined with stabilizers for remediation of chromium-contaminated soil in semi-arid areas.
This study compared the effects of four reduction agents, FeSO4, Fe(NH4)2SO4, Na2S and Na2SO3, on the leaching concentration of total Cr and Cr(VI) under the conditions of different dosages, water (mL)-soil (g) ratio and remediation time through indoor simulation experiment, which determined the best reduction agent type and reduction conditions. On this basis, three stabilizers, calcium magnesium phosphate (CMP), quicklime (CaO) and modified corn stover biochar (KBC), were combined to screen the stabilizer type and dosage, and select the combination of reduction agent and stabilizer that is economical, efficient, environmentally friendly and stable in long-term effect. And the stabilization effectiveness before and after soil remediation was compared, so as to evaluate the practicality and feasibility of the combined chemical reduction and stabilization technology, with a view to providing theoretical and management basis for the remediation of chromium-contaminated soil in semi-arid areas, and feasible technical solution for its remediation and treatment.
2. Materials and methods
2.1 Cr(VI) contaminated soil preparation
The indoor simulated restoration experiment was conducted in September 2021 at Lanzhou City in northwestern China. This area belongs to a semi-arid region with low precipitation (180–450 mm annually), high evapotranspiration (1500–1600 mm annually), and high temperatures in the summer (16–28°C). The raw soil used is gray calcium soil, excavated from unpolluted soil at 0–20 cm depth with a spade in the campus greenery of Northwest Minzu University at Lanzhou city, and sub-samples collected were mixed to obtain a composite sample in accordance with the standard method [Citation39]. The soil sample was transferred to the laboratory in plastic bags, naturally air-dried and milled, and then passed through a 2 mm sieve to remove large stones and grass clippings. The chemical and physical properties of raw soil are listed in . The chromium-contaminated soil used in the experiment was simulated soil. The soil with Cr(Ⅵ) content of about 1000 mg kg−1 was prepared with potassium dichromate (K2Cr2O7) solution. After mixing well with a glass rod, the mouth of the beaker was sealed with plastic film. The soil was cured under natural conditions for 7 days, dried in an oven at 60°C, and then milled to pass through a 0.149 mm sieve until ready for use.
Table 1. Chemical and physical properties of raw soil.
2.2 Preparation and modification of biochar
The corn straw collected from the suburbs of Lanzhou City was washed repeatedly with tap water, dried in an oven at 60°C, and then milled to pass through a 0.25 mm sieve until ready for use. The corn straw powder was compressed in a crucible, and then pyrolyzed in a muffle furnace at 500°C for 2 h under constant temperature and oxygen limitation. After cooling to room temperature, milled to pass through a 0.149 mm sieve to obtain the corn stover biochar (BC), which was sealed and stored until ready for use.
Biochar (50 g) was added to 1000 mL of 2 mol L−1 KOH solution which was fully soaked at a ratio of 1:10. The suspension was dispersed with an ultrasonic cleaner for 30 min, and then oscillated at a constant temperature of 25°C for 24 h. The resulting product was washed with deionized water until the pH of the waste liquid reached 7.0, and then dried in an oven at 60°C. The sample was milled to pass through a 0.149 mm sieve to obtain KBC which was sealed and stored until ready for use.
2.3 Experiment design
2.3.1 Screening of optimal reducing agent and dosage
Chromium-contaminated soil (100.0 g) was added to a 500 mL plastic beaker. The reducing agents ferrous sulfate (FeSO4), ammonium iron(II) sulfate (Fe(NH4)2SO4), sodium sulfide (Na2S), and sodium sulfite (Na2SO3) were dissolved in 40 mL deionized water at 1, 1.5, 2, 2.5, and 3 times theoretical reaction dosage for the chemical reaction with chromium-contaminated soil. The samples maintained the water (mL)-soil (g) ratio at 40%. After stirring well with a glass rod, sealed the beakers with plastic film and reduced at room temperature for 7 days. At the same time, the soil without adding any reducing agent was used for the blank test. After reducing, the soil dried in an oven at 60°C, and then milled to pass through a 0.149 mm sieve, and put into a plastic bottle. The total Cr and Cr(VI) leaching concentrations in soil were measured to determine the optimal reducing agent and dosage. Three parallel experiments were performed for each test condition.
2.3.2 Screening of optimal reduction conditions for chromium-contaminated soil
According to the results of Section 2.3.1, the optimal reduction agent and dosage were selected, and the single-factor experiment was performed. The stabilization effectiveness of total Cr and Cr(VI) in Chromium-contaminated soil under different water (mL)-soil (g) ratios (40%, 50%, 60%, 80%, and 100%) and remediation times (3, 7, 14, 21, 28, and 30 d) was examined to determine the optimal reduction conditions. The test methods are the same described in Section 2.3.1.
2.3.3 Chromium-contaminated soil stabilization experiment
Chromium-contaminated soil (100.0 g) was added to a 500 mL plastic beaker. The stabilizers CMP, CaO, and KBC were added to the beaker at 5%, 10%, 15%, 20%, 25%, and 30% of the soil mass, respectively, and the total Cr and Cr(VI) leaching concentrations in soil were measured. The test methods are the same described in Section 2.3.1.
2.3.4 Remediation experiment of chromium-contaminated soil by combined chemical reduction and stabilization
Chromium-contaminated soil (100.0 g) treated with the optimal reduction agent and dosage for 3 days was added to a 500 mL plastic beaker. The stabilizers CMP, CaO, and KBC were added to the beaker at 0.5%, 1%, 1.5%, 2%, 2.5%, 3%, 5%, and 8% of the soil mass, respectively. The samples maintained the water (mL)-soil (g) ratio at 50%. After stirring well with a glass rod, sealed the beakers with plastic film, reduced at room temperature for 4 days. The total Cr and Cr(VI) leaching concentrations in soil and Cr(VI) content in soil after remediation were measured. The test methods are the same described in Section 2.3.1.
2.3.5 Chemical speciation analysis
Chromium was extracted by MgCl2 solution in exchangeable (EX); by HAc-NaAc solution in carbonate-bound (CB); by NH2OH•HCl solution in Fe-Mn oxide-bound (OX); by HNO3-H2O2-NH4Ac solution in organic matter-bound (OM); and by HNO3-HClO4-HF solution in residual (RS) (). Chromium in soil obtained from Tessier’s sequential extraction procedure [Citation40] was also determined by an ultraviolet-visible spectrophotometer (UV-Vis, P2PC, Meproda Instruments Corporation, China).
Table 2. Tessier’s sequential extraction procedures.
The bioavailability coefficient was introduced in the paper to study the distribution and bioavailability of each speciation of chromium in soil. The bioavailability coefficient is the sum of the EX and OX fractions content divided by the amount of the total fraction.
2.3.6 Determination of effective speciation Cr(VI) in soil
DTPA and EDTA were used to extract and determine the effective speciation Cr(VI) in chromium-contaminated soil before and after the combined chemical reduction and stabilization remediation, to verify whether the biological effective speciation Cr(VI) content in chromium-contaminated soil before and after the remediation was decreased, and correlation and regression analysis were performed.
DTPA extraction method: Chromium-contaminated soil (25.0 g) treated with the optimal reduction agent and dosage for 3 days was added to 50 mL 0.005 mol L−1 DTPA-0.01 mol L−1 CaCl2-0.1 mol L−1 TEA (pH 7.30) solution.
EDTA extraction method: Chromium-contaminated soil (5.0 g) treated with the optimal reduction agent and dosage for 3 days was added to 50 mL 0.05 mol L−1 EDTA (pH 9.0) solution.
The above-treated samples oscillated in a water bath oscillator at 25°C and 180 r min−1 for 2 h, and then centrifuged at 3000 r min−1 for 10 min in a centrifuge. The supernatant was filtered through qualitative filter paper to measure the effective speciation Cr(VI) content.
2.4 Experiment methods
The determination of pH, water content, conductivity, cation exchange capacity, and organic matter content of raw soil was based on the ‘Soil Agrochemical Analysis Method’ [Citation41]. The Cr(VI) content in soil was determined by the alkaline digestion method (HJ 1082–2019, Chinese National Standards) [Citation42]; and 1,5-diphenylcarbohydrazide spectrophotometric method (GB/T 15555.4–1995, Chinese National Standards). The chromium leaching solutions were collected using an extraction agent containing sulfuric acid and nitric acid (HJ/T 300–2007, Chinese National Standards) [Citation43]. The pH of the extraction agent used was 3.20 ± 0.05. Total Cr and Cr(VI) leaching concentrations were measured spectrophotometrically using 1,5-diphenylcarbohydrazide as the chromogenic reagent (GB/T 15555.5–1995 and GB/T 15555.4–1995, Chinese National Standards) [Citation44]. The comprehensive wastewater discharge standards and soil control standards are listed in . The stabilization efficiency (η) of pharmaceuticals for total Cr and Cr (VI) in soil was calculated using the equation
Table 3. Comprehensive wastewater discharge standards and soil control standards.
where and
are the total Cr and Cr (VI) concentrations (in mg L−1) leached from the unstabilized and stabilized soil, respectively.
3. Results and discussion
3.1 Stabilization effectiveness of reducing agents on total Cr and Cr(VI) in soil
The changes in total Cr and Cr(VI) leaching concentrations with dosage during the treatment of chromium-contaminated soil with different reducing agents are shown in . The total Cr and Cr(VI) leaching concentrations in soil decreased gradually with the increase of reducing agent dosage. Under the same dosage of reducing agents, the reduction effect of iron-based reducing agents (Fe(NH4)2(SO4)2, FeSO4) is better than that of sulfur-based reducing agents (Na2SO3, Na2S). This is because due to the reduction reaction of Cr(VI) occurring at the liquid–liquid interface, ferrous salts can reduce Cr(VI) to Cr(III). At the same time, Fe2+ in soil reacts with water to form hydrated iron oxide, which adsorbs Cr2O2-7 in soil to form insoluble composite precipitates FexCr1-x(OH)3 or Fe2Cr2-xO3. Therefore, total Cr and Cr(VI) leaching concentrations decreased significantly under the dual effects of chemical reduction and colloid adsorption. Comparing FeSO4 with Fe(NH4)2(SO4)2 at 3 times theoretical reaction dosage, the stabilization effect of FeSO4 is better than Fe(NH4)2(SO4)2. This is because the stabilization efficiency of FeSO4 is greater than that of Fe(NH4)2(SO4)2 for the same dosage from the point of view of electron transfer for the role of reducing agent, so FeSO4 was chosen as the optimal reducing agent in this study. The stability of FeSO4 as the most effective reducing agent lies in the fact that Cr(VI) is rapidly reduced to Cr(III) under the reduction of Fe2+, and while toxicity is reduced, the resulting Fe3+ and Cr(III) exists in the form of precipitation. Due to the co-precipitation of Fe(OH)3 and Cr(OH)3, the reoxidation risk of Cr(III) is reduced after reduction. The results of Yin et al. [Citation45] found that FeSO4 had the best stabilization effect on chromium-contaminated soil and effectively reduced the total Cr and Cr(VI) contents in the leachate. Total Cr and Cr(VI) leaching concentrations in soil met the requirements of the discharge standards when the FeSO4 dosage was the theoretical reaction dosage (2.5 times and 3 times), while the stabilization efficiency improvement was less obvious when the FeSO4 dosage was 3 times theoretical reaction dosage. The main reason for this phenomenon is that chromium in soil exists partly on the outer surface of soil particles and partly wrapped in the inner part [Citation46]. Cr(VI) attached to the outer surface of particles is stabilized by the reduction of FeSO4 to Cr(III), while Cr(VI) stored in the inner part of soil particles is difficult to react with FeSO4 effectively, making it difficult to further improve the stabilization efficiency, and the excessive FeSO4 will lead to soil acidification and secondary pollution. Therefore, 2.5 times theoretical reaction dosage of FeSO4 was chosen as the optimal dosage in this study.
Table 4. Effect of reductant types and dosage on the leaching concentration of total Cr and Cr(VI) in soil.
3.2 Water (mL)--soil (g) ratio and remediation time on stabilization effectiveness of total Cr and Cr(VI) in soil with FeSO4 as reducing agent
The water (mL)-soil (g) ratio is one of the most important factors affecting the reduction of heavy metals in soil. To ensure adequate reaction between the reducing agent and the heavy metals, it is usually necessary to add appropriate deionized water for treatment. From the screening experiment results of reducing agent and dosage, the effect of different water (mL)-soil (g) ratios on total Cr and Cr(VI) leaching concentrations in soil is shown in ). When the water (mL)-soil (g) ratio increased from 40% to 50%, 60%, 80%, and 100%, total Cr and Cr(VI) leaching concentrations in soil were 0.724 mg L−1, 0.674 mg L−1, 0.759 mg L−1, 0.814 mg L−1, 0.850 mg L−1 and 0.485 mg L−1,0.471 mg L−1, 0.504 mg L−1, 0.522 mg L−1, 0.544 mg L−1, respectively. Therefore, when the water (mL)-soil (g) ratio is 50%, the stabilization effectiveness was obvious; at water (mL)-soil (g) ratio less than or greater than 50%, the stabilization effectiveness was less optimal. The analysis shows that when the water (mL)-soil (g) ratio was low, the slurry was thicker, Fe2+ and Cr2O2-7 could not react completely, and stabilization effectiveness was poor. With the increase of water (mL)-soil (g) ratio, the migration ability of Cr2O2-7 in soil was enhanced, and the reducing agent dissociated more reducing ions, which accelerated the reaction between Fe2+ and Cr2O2-7, and improved the stabilization efficiency of Cr2O2-7. However, when water (mL)-soil (g) ratio from 50% to 100%, the reducing agent concentration was continuously diluted, and its stabilization ability of total Cr and Cr(VI) decreased gradually, resulting in increased total Cr and Cr(VI) leaching concentrations in soil with increased water (mL)-soil (g) ratio. Therefore, the 50% of water (mL)-soil (g) ratio was selected as the best according to the soil remediation target value.
Figure 1. Effect of water -soil ratio (a) and remediation time (b) on the leaching concentration of total Cr and Cr(VI) in soil with FeSO4 as reducing agent.

Based on the above experimental results, the effect of different remediation times on total Cr and Cr(VI) leaching concentrations in soil is shown in ). With the extension of remediation time, total Cr and Cr(VI) leaching concentrations in soil decreased gradually. When the remediation time was 3 days, total Cr and Cr(VI) leaching concentrations in soil were 0.763 mg L−1 and 0.591 mg L−1, respectively, while Cr(VI) leaching concentration in soil exceeded the requirements of the discharge standards. When the remediation time was increased from 7 days to 30 days, total Cr and Cr(VI) leaching concentrations in soil decreased from 0.674 mg L−1 and 0.471 mg L−1 to 0.371 mg L−1 and 0.214 mg L−1, respectively, and the stabilization efficiency of total Cr and Cr(VI) only increased by 0.46% and 0.41%, respectively. The stabilization effectiveness was not obvious, and the remediation time of 7 days most of the total Cr and Cr(VI) in soil had been stabilized and met the discharge standards. Therefore, the remediation time of 7 days was selected as the best according to the soil remediation target value.
3.3 Stabilization effectiveness of stabilizers on total Cr and Cr(VI) in soil
The changes in total Cr and Cr(VI) leaching concentrations with dosage during the treatment of chromium-contaminated soil with different stabilizers are shown in . The total Cr and Cr(Ⅵ) leaching concentrations in soil decreased gradually with the increase of stabilizer dosage, which the stabilization effectiveness of KBC on Cr2O2-7 in soil was the best. The total Cr and Cr(VI) leaching concentrations of untreated contaminated soil were 65.65 mg L−1 and 61.98 mg L−1. For the CMP-stabilized soil, the total Cr and Cr(VI) leaching concentrations decreased from 54.315 mg L−1 and 53.114 mg L−1 to 32.147 mg L−1 and 30.497 mg L−1, respectively, when the dosage of CMP was increased from 5% to 30%. Their stabilization efficiency increased from 17.27% and 14.30% to 51.03% and 50.80%, respectively. This is because CMP belongs to phosphate stabilizers, whose main components include Ca3(PO4)2, CaSiO3, and MgSiO3 [Citation47]. After CMP was incorporated into chromium-contaminated soil, the slowly released Ca2+ can combine with Cr2O2-7 to generate stable CaCr2O7 precipitate, which reduces the migration activity of Cr(VI). For the CaO-stabilized soil, the total Cr and Cr(VI) leaching concentrations decreased from 27.206 mg L−1 and 27.771 mg L−1 to 11.658 mg L−1 and 10.839 mg L−1, respectively, when the dosage of CaO was increased from 5% to 30%. Their stabilization efficiency increased from 58.56% and 55.19% to 82.24% and 82.51%, respectively. This may be due to the hydrolysis reaction of CaO to generate Ca(OH)2, which raises the pH of the soil, and the free Ca2+ in solution reacts with Cr2O2-7 to generate CaCr2O7 precipitate. CaCr2O7 can exist stably in alkaline and neutral environments. For the KBC-stabilized soil, the total Cr and Cr(VI) leaching concentrations decreased from 53.709 mg L−1 and 52.909 mg L−1 to 31.982 mg L−1 and 32.727 mg L−1, respectively, when the dosage of KBC was increased from 5% to 30%. Their stabilization efficiency increased from 18.19% and 14.64% to 51.28% and 47.20%, respectively. This is because KBC, with its excellent porous structure and abundant oxygen-containing functional groups [Citation7], adsorbs Cr(VI) on the surface and reductively transforms it into Cr(III), after which Cr(III) is stabilized by KBC through mechanisms such as surface precipitation, surface coordination, and ion exchange.
Figure 2. Effect of CMP (a), CaO (b) and KBC (c) on the leaching concentration of total Cr and Cr(VI) in soil.

In conclusion, the stabilization experiments showed that the total Cr and Cr(VI) leaching concentrations after stabilization with CMP, CaO, and KBC were still higher than the requirements of the emission standards. Therefore, further treatment is needed to enhance the stability of chromium in soil and ensure environmental safety.
3.3 Stabilization effectiveness of combined chemical reduction and stabilization remediation on chromium-contaminated soil
The effects of FeSO4 combined with different dosages of stabilizers on the total Cr and Cr(VI) leaching concentrations and Cr(VI) content in soil are shown in . From the perspective of stabilization effectiveness, compared with FeSO4 alone, the stabilization effectiveness of FeSO4 combined with three kinds of stabilizers showed different degrees of change, and the overall stabilization effectiveness was KBC, CaO, and CMP in order from strong to weak. The total Cr and Cr(VI) leaching concentrations and Cr(VI) content of KBC at each dosage met the requirements of the emission standard, and the 8% dosage of KBC had the best stabilization effectiveness in remediating chromium-contaminated soil. At this time, the total Cr and Cr(VI) leaching concentrations and Cr(VI) content in soil were 0.125 mg L−1, 0.114 mg L−1, and 18.909 mg/kg, respectively. Their stabilization efficiency was 99.81%, 99.82%, and 98.11%, respectively. This is because, on the one hand, oxygen-containing functional groups such as C = O, -OH, -COOH, and C-O on the surface of KBC are involved in the adsorption of Cr(VI) and occur in a specialized adsorption behavior [Citation48]; on the other hand, the pH of soil decreases after FeSO4 treatment, and Fe3+ is adsorbed on KBC surface under acidic conditions and further reduced to Fe2+. Fe2+ can directly reduce Cr(VI) to Cr(III) [Citation31]. In the reduction process, Fe2+ is electronically oxidized to Fe3+. Fe3+ can be re-adsorbed to the surface of KBC and act as an electron shuttle again, thus improving the stability effectiveness. Cr(III) is easily electrostatically adsorbed to form Cr(OH)3 precipitate by the negatively charged KBC on the surface. At the same time, Cr(III) can form a poorly soluble FexCr1−x(OH)3 or Fe2Cr2−xO3 composite precipitate with Fe2+ and Fe3+, thus improving the removal rate of Cr(VI). The stabilization effectiveness of 8% dosage of CaO was taken second place. At this time, the total Cr and Cr(VI) leaching concentrations and Cr(VI) content in soil were 0.210 mg L−1, 0.183 mg L−1, and 24.000 mg kg−1, respectively. Their stabilization efficiency was 99.66%, 99.70%, and 97.60%, respectively. This is because CaO has a strong chromium adsorption capacity, raises the pH of the soil, ionizes under alkaline conditions to produce OH−, and forms more stable Cr(OH)3 precipitate with amorphous Cr(III). At the same time, Cr(III) forms a poorly soluble FexCr1−x(OH)3 or Fe2Cr2−xO3 composite precipitate [Citation49] under the joint action of Fe2+. Compared with KBC and CaO, FeSO4 combined with CMP has poor stability effectiveness.
3.5 Chemical speciation analysis
The biotoxicity and stability of chromium in soil are more largely determined by the bound morphology of chromium. The morphology of chromium and its transformation are important for the study of the environmental effects of heavy metals, the treatment, and remediation of contaminated soils, and their stability. The effect of FeSO4 combined with different stabilizers on the morphological transformation of chromium in soil was studied by Tessier continuous extraction method. As shows, chromium species in chromium-contaminated soil were exchangeable (EX, 55.7%), carbonates-bound (CB, 1.9%), Fe-Mn oxides-bound (OX, 14.5%), organic matter-bound (OM, 11.3%) and the residual (RS, 16.5%), and the bioavailability coefficient of Cr(VI) was 0. 5763. Compared with the chromium-contaminated soil, the EX and CB fraction were almost completely converted to OX, OM, and RS when FeSO4 alone treats chromium-contaminated soil. Especially, both OM and RS fractions increased by 22.5%, possibly because chromium in soil was mainly in the form of Cr(OH)3 or Cr(III)/Fe(III) oxides/hydroxides [Citation49] after FeSO4 treatment, which accounted for the reduced total Cr and Cr(VI) leaching concentrations in the above remediation tests.
Figure 5. Effect of combined remediation with reductor of FeSO4 and stabilizers of CMP (a), CaO (b) and KBC (c) on chromium speciation in soil. (Unstable-with FeSO4 only, CK-blank control without remediation).

When 0.5% to 8% of CMP was added as stabilizer, the EX fraction was slightly higher than that in FeSO4 treatment. Accordingly, the total Cr and Cr(VI) leaching concentrations in soil were also higher, which was consistent with the results of previous studies. The OX and OM fraction gradually decreased with the increase of the dosage, while the RS fraction showed a gradually increasing trend. This was because, CMP was a silica-aluminate complex containing phosphate radical (PO3-4), and the aqueous solution was alkaline. CMP could slowly release PO3-4 when added to chromium-contaminated soil, and free Cr(III) could react with PO3-4 to form the chemically extremely stable CrPO4 precipitate. As the solubility of CrPO4 was lower than that of Cr(OH)3, more chromium occurred in the RS fraction. When 0.5% to 8% of Cao and KBC were added as stabilizers, the EX and CB fractions were basically consistent with that of FeSO4 treatment. The OX and OM fractions gradually decreased with the increase of the dosage, while the RS fraction showed a gradually increasing trend. Among the three stabilizers, KBC has the best stabilization effectiveness. This was because, after the reduction of Cr(VI) to Cr(III) by Fe2+, Cr(III) had a similar ionic radius to Fe3+ and was easily adsorbed on the surface of Fe(OH)3, while Cr(III) in the presence of KBC undergoes ion exchange and complexation reactions with the oxygen-containing functional groups in KBC, resulting in the morphology transformation of chromium to a more stable form. When the dosage of KBC was 8%, the RS fraction reached 90.4%, which was 2.3 times higher than that of chromium-contaminated soil and 5.5 times higher than that of FeSO4 when treated alone, and the bioavailability coefficient of Cr(VI) was reduced to 0.0029, which was a remarkable stabilization effectiveness.
In summary, it was determined that the decreasing trend of total Cr and Cr(VI) leaching concentrations and Cr(VI) content in soil were significantly better than that of CaO and CMP when KBC was used as stabilizer, and the RS fraction was most superior. The optimally combined chemical reduction and stabilization remediation condition for stabilizing chromium in soil were 2.5 times the theoretical reaction amount FeSO4 and 8% KBC.
3.6 Changes in effective speciation Cr(VI) in soil
Two chelating agents (DTPA and EDTA) were used to extract the effective speciation Cr(VI) in chromium-contaminated soil. The effect of different dosages of stabilizers combined with FeSO4 on the effective speciation Cr(VI) content in chromium-contaminated soil was explored, as shown in . With the increase of CMP, CaO, and KBC, the effective state Cr(VI) contents in soil gradually decreased, and KBC had the best stabilization effect. The effective speciation Cr(VI) content of DTPA extraction was significantly lower than that of EDTA, which may be due to the different types of chelating agents with different effects on the chelation of Cr(VI) in chromium-contaminated soil. Many studies had shown that EDTA, HCI, Mehlich3, DTPA, NH4OAc, CaCl2, and other extractants extracted the largest amount of soil heavy metals was EDTA [Citation50], which was consistent with the results of this experimental study. The effective speciation Cr(VI) contents extracted by DTPA and EDTA were 0.098 mg kg−1 and 1.663 mg kg−1, respectively, when the dosage of KBC reached 8%.
Figure 6. Effect of combined chemical reduction and stabilization remediation on the effective speciation Cr(VI) content in soil for different types of extraction methods with EDTA (a) and DTPA (b).

The correlations between the effective speciation Cr(VI) content and Cr(VI) leaching concentration in soil for different types of extraction methods are shown in and . Significantly positive correlation (p < 0.01) was found between the effective speciation Cr(VI) content and Cr(VI) leaching concentration in soil. The correlation coefficients (R) of EDTA extraction method were 0.967 for CMP, 0.877 for CaO, and 0.798 for KBC, respectively; those of DTPA extraction method were 0.914 for CMP, 0.955 for CaO, and 0.811 for KBC, respectively. The fitted equation changes mainly conformed to the linear equation. This indicated that when the biologically effective speciation Cr(VI) content in soil decreases, the Cr(VI) concentration in the soil leachate was also affected and tends to decrease.
Figure 7. Stepwise regression analysis between Cr(VI) leaching concentration and EDTA -Cr(VI) content in soil with different remediation agency of CMP (a), CaO (b) and KBC (c) and DTPA-Cr(VI) content with CMP (d), CaO (e) and KBC (f).

Table 5. Correlation coefficients (R) between Cr(VI) leaching concentration and effective speciation Cr(VI) content in soil for different types of extraction methods with EDTA and DTPA (n = 9).
4. Conclusions
In this study, the idea of combined treatment of chemical reduction and stabilization was adopted, and the optimal experiment conditions of the combined remediation method were determined as follows: FeSO4 was used as the reducing agent, the dosage was 2.5 times theoretical reaction dosage, water (mL)-soil (g) ratio was 50%, and remediation time was 7 days. KBC was used as stabilizer, and its dosage was 10%. The bioavailability coefficient of Cr(VI) reduced from 0.5763 to 0.0029 under the above conditions to remediate the chromium-contaminated soil. The total Cr and Cr(VI) leaching concentrations and Cr(VI) content of the remediated soil were 0.114 mg L−1, 0.125 mg L−1, and 18.909 mg kg−1, respectively. They were according with comprehensive wastewater discharge standards and construction land soil contamination risk control standards. The effective speciation Cr(VI) contents extracted by DTPA and EDTA were 0.098 mg kg−1 and 1.663 mg kg−1, respectively, which were significantly and positively correlated with the Cr(VI) leaching concentration. The results of chemical speciation analysis showed that the highly reactive EX and CB fractions decreased significantly when FeSO4 treatment alone. After the reduction and stabilization of FeSO4 combined with stabilizer, the OX and OM fractions gradually decreased with the increase of the dosage, while the RS fraction showed an increasing trend. These results indicated that chemical reduction and stabilization could effectively improve the stability of Cr(VI) in soil and reduce the toxicity of Cr(VI). These results were highly favorable and provide useful insights and directions for future efforts in chemical reduction and stabilization of chromium-contaminated soil.
Disclosure statement
No potential conflict of interest was reported by the author(s).
Additional information
Funding
References
- Graham AM, Wadhawan AR, Bouwer EJ. Chromium occurrence and speciation in Baltimore Harbor sediments and porewater. Environmental Toxicology and Chemistry. 2009;28(3):471–12. Environmental Toxicology & Chemistry Baltimore Maryland.
- Lindsay DR, Farley KJ, Carbonaro RF. Oxidation of Cr(III) to Cr(VI) during chlorination of drinking water. J Environ Monit. 2012;14(7):1789–1797.
- Apte AD, Tare V, Bose P. Extent of oxidation of Cr(III) to Cr(VI) under various conditions pertaining to natural environment. J Hazard Mater. 2006;128(2/3):164–174.
- Wang Y, Fang Z, Liang B, et al. Remediation of hexavalent chromium contaminated soil by stabilized nanoscale zero-valent iron prepared from steel pickling waste liquor. Chem Eng J. 2014;247(6):283–290.
- Gong YL, Song AK, Gao XM, et al. Stabilization effect of composite medicament on soil contaminated with Cr. Chemical reagents. 2020;42(8): 982–986
- Zhang XY, Zhong TY, Liu L, et al. Chromium occurrences in arable soil and its influence on food production in China. Environ Earth Sci. 2016;75(3):257.
- Xia SP, Song ZL, Jeyakumar P, et al. Characteristics and applications of biochar for remediating Cr(VI)-contaminated soils and wastewater. Environ Geochem Health. 2019;42(6):1543–1567.
- Yang Z, Zhang X, Jiang Z, et al. Reductive materials for remediation of hexavalent chromium contaminated soil-A review. SciTotal Environ. 2021;773:1–13.
- Fu LJ, Feng AX, Xiao JJ, et al. Remediation of soil contaminated with high levels of hexavalent chromium by combined chemical-microbial reduction and stabilization. J Hazard Mater. 2021 Feb.5 Pt.2;403:123847.1–123847.12.
- Su H, Fang Z, Tsang PE, et al. Remediation of hexavalent chromium contaminated soil by biochar-supported zero-valent iron nanoparticles. J Hazard Mater. 2016;318:533–540.
- Beretta G, Mastorgio A, Pedrali L, et al. Support tool for identifying in situ remediation technology for sites contaminated by hexavalent chromium. Water. 2018;10(10):1344.
- Palma LD, Gueye MT, Petrucci E. Hexavalent chromium reduction in contaminated soil: a comparison between ferrous sulphate and nanoscale zero-valent iron. J Hazard Mater. 2015 Jan 8;281:70–76.
- Zhao SH, Chen ZL, Zhang TP, et al. Advances in solidification/stabilization technology treatment of heavy metals in contaminated soils. Chinese Journal of Soil Science. 2013;44(6): 1531–1536
- Wang D, Li G, Qin S, et al. Remediation of Cr(VI)-contaminated soil using combined chemical leaching and reduction techniques based on hexavalent chromium speciation. Ecotoxicol Environ Saf. 2021;208:111734.
- Li M, Sun ZM, Ma C, et al. Electrochemical combined remediation of chromium contaminated soil based on strengthening by anode consumption. Environ Eng. 2020;38(9):224–230.
- He ZG, Hu YT, Yin Z, et al. Microbial diversity of chromium-contaminated soils and characterization of six chromium-removing bacteria. Environ Manage. 2016;57(6):1319–1328.
- Xu R, Wang WH, Sun YJ, et al. Remediation of Cr(VI) from contaminated soil with the combination of biogas residue and ferrous sulfate. Acta Scientiae Circumstantiae. 2021;41(10):4161–4169.
- Yang XW, Zhang L, Bi X, et al. Research advancement of stabilization materials for hexavalent chromium (VI) contaminated site soils. Environ Eng. 2020;38(6):16–23.
- Lu QP. Study of stabilization treatment technology on chromium residue from a factory in Chongqing. Chongqing: Master Dissertation of Southwest University; 2012.
- Li QW, Zhang LW, Ji WW, et al. Feasibility of using ammonium iron (II) sulphate to passivate hexavalent chromium in polluted soil. Environ Technol. 2020;43(10):1522–1531.
- Zhang H, Fu RB, Guo XP, et al. Experimental research on stabilization remediation of chromium contaminated soil. Chin J Environ Eng. 2017;11(11):6163–6168.
- Zheng JC, Zhang JR, Liu XW, et al. Bioremediation of chromium (Ⅵ) contaminated site by reduction and microbial stabilization of chromium. Environ Sci. 2014;35(10):3882–3887.
- Warren GP, Alloway BJ. Reduction of arsenic uptake by lettuce with ferrous sulfate applied to contaminated soil. J Environ Qual. 2003;32(3):767–772.
- An MG, Zhao QL, Tan XF, et al. Research on the effect of chemical reduction-stabilization combined remediation of Cr-contaminated soil. Rock and Mineral Analysis. 2019;38(2): 204–211
- Wang W, Ma XL, Sun J, et al. Adsorption of enrofloxacin on acid/alkali-modified corn stalk biochar. Spectrosc Lett. 2019;52(7):367–375.
- He LZ, Fan SL, Muller K, et al. Comparative analysis biochar and compost-induced degradation of di-(2-ethylhexyl) phthalate in soils. SciTotal Environ. 2018;625:987–993.
- Huang P, Ge CJ, Feng D, et al. Effects of metal ions and pH on ofloxacin sorption to cassava residue-derived biochar. SciTotal Environ. 2018;616:1384–1391.
- Luo JW, Ge CJ, Muller K, et al. Sorption of norfloxacin, sulfamerazine and oxytetracycline by KOH-modified biochar under single and ternary systems. Bioresour Technol. 2018;263:385–392.
- Shaheen SM, Niazi NK, Hassan NE, et al. Wood-based biochar for the removal of potentially toxic elements in water and wastewater: a critical review. Int Mater Rev. 2019;64(4):216–247.
- Sun YQ, Iris KM, Tsang DC, et al. Multifunctional iron-biochar composites for the removal of potentially toxic elements, inherent cations, and hetero-chloride from hydraulic fracturing wastewater. Environ Int. 2019;124:521–532.
- Fan ZX, Zhang Q, Gao B, et al. Removal of hexavalent chromium by biochar supported nZVI composite: batch and fixed-bed column evaluations, mechanisms, and secondary contamination prevention. Chemosphere. 2019;217:85–94.
- Zhou JM, Chen HL, Thring RW, et al. Chemical pretreatment of rice straw biochar: effect on biochar properties and hexavalent chromium adsorption. Int J Environ Agric Res. 2019;13(1):91–105.
- Zhang S, Lyu HH, Tang JC, et al. A novel biochar supported CMC stabilized nano zero-valent iron composite for hexavalent chromium removal from water. Chemosphere. 2019;217:686–694.
- Yang LX. Study on the effect of bio-carbon on chromium in soil. Shenyang Agricultural University. 2017
- Xu YG, Bai TX, Yan YB, et al. Enhanced removal of hexavalent chromium by different acid-modified biochar derived from corn straw: behavior and mechanism. Water Sci Technol. 2020;81(9/10):2270–2280.
- Mahdi Z, Hanandeh AE, Yu QM. Preparation, characterization and application of surface modified biochar from date seed for improved lead, copper, and nickel removal from aqueous solutions. J Environ Chem Eng. 2019;7(5):103379.
- Shi YY, Shan R, Yuan HR. Effects and mechanisms of methyl Orange removal from aqueous solutions by modified rice shell biochar. Environ Sci. 2019;40(6):2783–2792.
- Hao ZK. Study on the effectiveness of modified wheat straw biochar to remediate chromium pollution. Beijing: China University of Geosciences; 2021.
- MEPPRC (Ministry of Environmental Protection of the People’s Republic of China). The technical specification for soil environmental monitoring. 1st ed. Beijing: China Standard Press; 2004. p. 1–44.
- Tessier A, Campbell PG, Bisson M. Sequential extraction procedure for the speciation of particulate trace metals. Anal Chem. 1979;51(7):844–851.
- Lu RK. Soil Agrochemical Analysis Method. Beijing: China Agricultural Science and Technology Press; 2000. p. 146–213.
- Mou HY, Liu WC, Zhao LL, et al. Stabilization of hexavalent chromium with pretreatment and high temperature sintering in highly contaminated soil. Front Environ Sci Eng. 2021;15(4):165–177.
- Chen QY, Ke YJ, Zhang LN, et al. Application of accelerated carbonation with a combination of Na2CO3 and CO2 in cement-based solidification/stabilization of toxic metal-bearing sediment. J Hazard Mater. 2009;166(1):421–427.
- Yuan WY, Xu WT, Zhang ZW, et al. Rapid Cr(VI) reduction and immobilization in contaminated soil by mechanochemical treatment with calcium polysulfide. Chemosphere. 2019;227:657–661.
- Yin Z, Liao SL, Ma Q, et al. stabilization of chromium in the contaminated soil by several different chemicals. Environ Eng. 2016;34(5):166–169.
- Ashraf A, Bibi I, Niazi NK, et al. Chromium(VI) sorption efficiency of acid-activated banana peel over organo-montmorillonite in aqueous solutions. Int J Phytoremediation. 2017;19(7):605–613.
- Li YY, Cundy AB, Feng JX, et al. Remediation of hexavalent chromium contamination in chromite ore processing residue by sodium dithionite and sodium phosphate addition and its mechanism. J Environ Manage. 2017;192:100–106.
- Chen Z, Liang Y, Zhao B, et al. Adsorption characteristics and mechanism of modified biochar to Cr(VI). Journal of Fudan University (Natural Science). 2021;60(6): 779–788
- Wang X, Zhang J, Wang L, et al. Long-term stability of FeSO4 and H2SO4 treated chromite ore processing residue (COPR): importance of H+ and SO42−. J Hazard Mater. 2017 jan 5;321:720–727.
- Zhang MK, Liu ZY, Wang HW. Use of single extraction methods to predict bioavailability of heavy metals in polluted soils to rice. Commun Soil Sci Plant Anal. 2010;41(5/8):820–831.