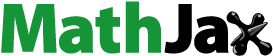
ABSTRACT
In this study, activated residual Dodonaea Viscosa (ARDV) was synthesized by the simple chemical activation process as a cost-effective and sustainable adsorbent for the efficient elimination of methyl green molecule (MG) from water. The prepared ARDV was characterized by TGA, SEM, EDX, FTIR, and BET surface area analysis. The adsorption properties and mechanisms toward MG were examined using the batch method. The optimal conditions for adsorption of Pb(II) were pH= 6.6, [ARDV] =0.1 g, time=180 min, and temperature= 65°C. The adsorption isotherms and kinetics study was conducted and the resulting data fitted well with Langmuir and PSO model. The maximum sorption capacity of 99 mg/g was obtained from Langmuir isotherm for MG at 60 °C. According to thermodynamic parameters, the adsorption of MG on ARDV was endothermic and spontaneous. Hence, it could be concluded that ARDV is an inexpensive and effective bio-adsorbent for the removal of MG from polluted water.
1. Introduction
Dye wastewater is generated by different industries such as textile, plastics, printing, electroplating, paper dye synthesis, leather and cosmetics [Citation1]. ~10% to 20% of these dyes are existing in the effluent of these industries [Citation2]. Disposal of these dyes into the environment without any treatment is hazardous to the ecosystem and health due to some of the dyes being toxic, carcinogenic and degradation resistant [Citation3–7]. Besides, the presence of a very low concentration of methyl green, in water, is undesirable. Methyl green (MG) [C27H35BrClN3ZnCl2] is a cationic dye that is used in medicine and biology such as staining DNA and cell nuclei, as well as used as a photochromophore to sensitize gelatinous films [Citation8,Citation9]. Owing to the negative effects of organic dyes on the ecosystem and human health, it is vital to eliminate these organic dyes before releasing them into the water environment.
Various dyes removal methods, such as photodecolorization [Citation10], membrane [Citation11], photocatalytic degradation [Citation12], UV photolysis [Citation13] and adsorption [Citation14], were developed to limit their effect on the environment. Among them, adsorption is believed to be the most promising method owing to its effectiveness, low cost, easy operation, and efficient technique for the removal of nonbiodegradable pollutants [Citation15,Citation16]. Many adsorbents have been studied for the elimination of methyl green from the water systems, namely, activated carbon [Citation17], reduced graphene oxide [Citation18], MCM-41 [Citation17] and graphene sheets [Citation19]. However, most of them have drawbacks such as high cost and low adsorption efficiency. The efficiency of the adsorption process mainly depends on the abundance and cost in nature, easy regenerability and stability [Citation20].
Recently, biosorbents made from plants are getting much more interest as effective adsorbents for the elimination of molecule dyes from water systems owing to their advantages of low cost, availability, eco-friendliness and accessibility [Citation21]. Therefore, many researchers have examined the elimination of pollutants from aqueous solutions by bio adsorbent materials like, parviflora plant leaves [Citation22], Platanus orientalis leaves [Citation23], algal biomass [Citation24], pine leaves [Citation25], phoenix tree leaf powder [Citation26], Solanum incanum [Citation22], activated Aerva javanica and dead leaves of Ficus racemosa [Citation27].
Dodonaea viscosa is used traditionally as folk medicine in many countries to treat many diseases like rheumatism, malaria, cold, fever, indigestion, headaches, toothaches, diarrhea, constipation and dysmenorrhea [Citation28]. This is maybe a plant container much important like tannins, flavonoids, alkaloids, saponins, triterpenoids, steroids, volatile oil and phytosterols [Citation29]. Therefore, Dodonaea viscosa has numerous highly reactive chemical functions which use them as adsorption sites for adsorption pollutants.
Usually, after extraction of medicine materials from raw plants, the residues materials are thrown out. To the best of our knowledge, this is the first time to use residual waste of a medicinal plant after their extraction and use it for dye adsorption after activation by hydrogen peroxide and sodium hydroxide. The extraction of the active medicine materials had been used as therapeutic compounds for anticancer and antimicrobial activities [Citation30]. Therefore, this work has thus focused on the development a new biosorbent from residual waste of a medicinal plant (Dodonaea viscosa). The residual waste of a medicinal plant was activated by H2O2 and NaOH reagents to enhance the adsorption properties of RDV toward MG adsorption from aqueous solutions. The activated residual Dodonaea viscosa (ARDV) was characterized by SEM, EDX, BET, TGA and FTIR. Besides, the influence of adsorbent dose, solution pH, temperature, contact time and initial MG concentration on the methyl green adsorption using activated residual Dodonaea viscosa (ARDV) was achieved. The thermodynamics, kinetics and isotherm adsorption were investigated to establish the mechanism and nature of the adsorption process of MG on synthesized bio adsorbent (ARDV).
2. Experiments
2.1. Chemicals
Hydrogen peroxide (H2O2, 30%), methyl green (MG) and ethanol (C2H5OH, 99.8%) were gotten from Sigma Aldrich. Nitric acid (HNO3, 68.0–70.0%), hydrochloric acid (HCl, 36%) and dodium hydroxide (NaOH, ≥ 97%) were procured from BDH, England.
2.2. Characterization techniques
The surface morphology and elemental analysis of the DV, RDV, ARDV and MG/ARDV powders were evaluated by a scanning electron microscope (SEM) and energy-dispersive X-ray (JEOL 200KV, Tokyo, Japan), respectively. Fourier transform infra-red spectra of the Dodonaea viscosa (DV), Residual Dodonaea viscosa (RDV), Activated Residual Dodonaea viscosa (ARDV) and MG-loaded ARDV adsorbents were recorded by Nicolet iS50, Thermo Scientific (Madison, WI, USA). Surface area, pore volumes and pore radius of DV, RDV and ARDV were determined using BET method and BJH (Barrett-Joyner-Halenda) method. The thermal stability of DV and ARDV adsorbents were carried out by thermogravimetric analyzer (Mettler Toledo GA/SDTA851) in nitrogen atmosphere under the heating rate of 10 ͦC min−1 varying from room temperature to 800 ͦC.
2.3. Chemical treatment of Dodonaea viscosa
Dodonaea viscosa (DV) was collected from Albaha, Saudi Arabia. The Dodonaea viscosa was washed several times with distilled water and then dried and ground into powder using an electrical grinder. Stock samples were prepared by extracting 80 g of Dodonaea viscosa powder using hexane (nonpolar solvent) and ethanol (polar solvent) at room temperature. All DV extracts were prepared in the Soxhlet apparatus and refluxing for one day according to the boiling point of each solvent. After extraction, the solvent was evaporated using a rotary evaporator [Citation30]. The extracted fraction in each solvent was calculated using the following Equationequation 1(1)
(1) :
The use of ethanol in the extraction from Dodonaea viscosa ensured a better extraction yield around 25.45%. The residual of the Dodonaea viscosa (RDV) (not extracted) portion underwent activation treatment and was used for the adsorption experiments. To improve the adsorption properties of RDV powder and eliminate the impurities from its surface, the dried RDV was treated with H2O2 and 0.1 M NaOH [Citation31]. Briefly, 20 g of RDV powder was immersed in 150 mL of H2O2 under magnetic stirring for 24 h, then, the RDV powder was washed several times with deionized water. Following that, 20 g of activated residual Dodonaea viscosa (ARDV) powder was immersed in 150 mL of 0.1 M NaOH under stirring for 24 hrs. Finally, the ARDV was washed several times with deionized water and then dried in an oven at 60°C for 24 hrs. ().
2.4. Adsorption studies
A standard curve of absorbance-methyl green concentration was plotted based on the measured absorbance of MG at five concentration levels (2–10 mg/L) by UV/Visible spectrometer at λmax 632 nm. This standard curve was plotted to obtain the concentration of MG at any time throughout the period, and the MG dye exhibited satisfactory linearity with correlation coefficients (R2 = 0.999). The adsorption process was carried out using batch method. The influence of various parameters on adsorption of MG dye by using ARDV adsorbents, namely, contact time (5–240 min), pH (3.4–11), adsorbent mass (0.01–0.2 g), initial MG concentration (45–200 mg/L and temperature (25–65°C), was investigated. All adsorption experiments were carried out three times to ensure accuracy and repeatability, and to minimize errors. The general procedure was as follows: 0.1 g of ARDV adsorbent was added to 100 mL of MG dye solutions of 15 to 200 mg/L concentration. Then the contents were adjusted using 0.1 M HCl or NaOH to the wanted pH. After that the contents were shaken at 100 rpm using a mechanical shaker to 180 min. Then the solution was filtered and the residual MG concentration was measured. The adsorbent capacity (qe mg/g) and removal efficiency (R%) were calculated according to EquationEq. 2(2)
(2) and Equation3
(3)
(3) , respectively.
where Co (mg/L) and Ce (mg/L) refer to the initial concentrations of MG and the equilibrium concentrations of MG, respectively; V(L) is the volume of the MG solution, and m (g) is the mass of ARDV.
For the influence of the adsorbent dose: different ARDV adsorbent quantities (0.01–0.2 g) were added into a series of flasks containing 100 mL of MG dye solution of 15 mg/L concentration at pH = 6.6, separately. The contents of the conical flasks were shaken at 100 rpm under 25 ͦC for 1440 min. After the adsorption process, the residual concentration of the MG dye ions was determined. For the influence of contact time, the adsorption experiments were performed by placing 0.1 g of ARDV adsorbent in a series of conical flasks containing 100 mL (15 mg/L) MG solution at pH 6.6. The time gradient was set to 5, 10, 15, 30, 45, 60, 120, 180 and 240 min, then the samples were taken at time intervals for the analysis of residual MG concentration. The influence of initial MG concentration and temperature on the MG adsorption was carried out by placing 0.1 g of ARDV in a series of conical flasks containing 100 mL of MG dye at definite concentrations (45, 60, 75, 100, 150, 200 mg/L) at pH 6.6. The contents of the conical flasks were shaken at 100 rpm at 25 ͦC for 180 min. After the adsorption process, the residual MG concentration of the MG dye ions was determined. These steps were repeated at 45 ͦC and 65 ͦC, and residual MG concentration was determined.
3. Results and discussion
shows the FTIR of RDV, ARDV and MG-saturated ARDV. For RDV adsorbent, the band at 3302 cm−1 represents the – OH group in tannins, flavonoids, alkaloids, saponins, triterpenoids, steroids, volatile oil, and phytosterols, indicating the existence of -OH and -COOH groups over ARDV surface. This band slightly augmented and shifted to 3330 cm−1 after being activated via H2O2 and NaOH reagents. The bands at 1926 and 2852 cm−1 were assigned to the asymmetric and symmetric stretching vibration of–C-H in methylene. The weak and strong characteristic absorption bands at 1726 cm−1 represent the C = O group [Citation32]. Additionally, the characteristic band at 1618–1516 cm−1 was attributed to aromatic C = C bond stretching which is due to the phenolic compounds [Citation33]. The band of C = C was reduced in intensity and shifted to 1612 cm−1 after being activated with chemical reagents. The absorption bands at 1442, (1367–1311), and 1238 cm−1 were assigned to asymmetric stretch vibrations of COO− [Citation34], the bending vibration of – CH3 and – CH2, and the stretch vibrations of C–OH [Citation33]. The strong absorption band at 1154 cm−1 and a weak band at 1024 cm−1 were corresponding to C-O-C groups in tannins, saponins and flavonoids [Citation35]. The bands around 800–500 cm−1 assigned to CH = CH2 stretching vibrations in aromatic structures [Citation36]. After MG adsorption on ARDV adsorbent, it was observed that some bands were reduced or increased in their intensities and shifted. In detail, the strong adsorption band at 1600 cm−1 was decreased owing to π–π interactions between the MG molecules with – C = C–in ARDV. The band of–OH group with intermolecular hydrogen bond was shifted from 3330 to 3335 cm−1. Besides, the band of carboxylic acid had a slight increase in intensity due to the electrostatic attraction between a cationic MG molecule and the negative charge of COO− onto the ARDV surface. The intensity band of C-O-C was significantly increased in intensity after MG adsorption onto ARDV adsorbent. These bands provide enough evidence to support the MG adsorption onto the ARDV surface by π-π interactions, hydrogen bonding and electrostatic interaction.
represents the thermal gravimetric analysis of RDV and ARDV adsorbents. It was observed that the total weight loss for RDV and ARDV adsorbents were 80% and 85%, respectively, in the range of 30–800°C, indicating that the RDV was activated using the chemical reagent sodium hydroxide and hydrogen peroxide. For more details, a two-stage thermal decomposition can be observed for both RDV and ARDV adsorbents. In the first stage, a 9% weight loss was observed for both RDV and ARDV adsorbents in the range of 30–230°C and 30–285°C, respectively, due to the moisture evaporation and solvent. In the second stage, a weight loss of 61% in the range of 230 to 475°C and 285 to 400°C was observed in both RDV and ARDV adsorbents, which might be due to the decomposition of organic compounds like flavonoids, alkaloids, saponins, triterpenoids, steroids, volatile oil and phytosterols. These organic materials underwent continuous degradation until 800°C with weight loss of 10% and 15% for RDV and ARDV adsorbents, respectively. However, the rate of loss of volatiles for ARDV adsorbent was much higher as compared to the DV. The total weight losses of DV and were 80% and 85%, respectively. Thus, the oxygen-content functional in the ARDV adsorbent was about 5% and that is due to the activation of DV adsorbent with sodium hydroxide and hydrogen peroxide.
depicts the morphological characteristics of RDV, ARDV and MG-saturated ARDV using SEM technology. It was observed that the RDV surface exhibits a rough surface with pores (). While the surface of RDV becomes highly porosity due to the activation of RDV with H2O2 and NaOH reagents (). After MG adsorption over ARDV, the surface looks smooth and dark which can be taken as a sign of adsorption of MG dye in the pores of ARDV ().
The elemental analysis of the RDV, ARDV and MG saturated ARDV were checked by EDX as shown in . In the case of RDV, two peaks with content (75.79%) and (24.21%) for carbon and oxygen were observed (). After activation of RDV with hydrogen peroxide, the oxygen content was increased to 27%, while the carbon content was reduced to 72.16% ().
The BET specific surface area, pore volume and average pore radius were observed to be (0.425 m2/g, 0.021 cc/g and 3.10 nm), (5.167 m2/g, 0.018 cc/g and 2.148 nm), (12.789 m2/g, 0.031 cc/g and 1.996 nm) for DV, RDV and ARDV respectively. The surface area of the ARDV adsorbent is 96.7% greater than DV, and 59.6% higher than RDV, indicating the ARDV adsorbent has a greater adsorption capacity toward MG dye due to the adsorption capacity increased with the surface area.
3.1. Adsorption studies
3.1.1. Adsorbent selectivity
The prepared adsorbents namely, Dodonaea viscosa (DV), activated Dodonaea viscosa (ADV) residual Dodonaea viscosa (RDV) and activated residual Dodonaea viscosa (ARDV) powders, were tested for adsorption of MG dye. Tests were performed at Co = 15 mg/L; adsorbent; 0.1 g; pH = neutral; T = 25°C; agitated speed = 100 rpm; contact time = 1440 min) as indicated in . The maximum elimination efficiency of MG on the DV, ADV, RDV and ARDV powders were 88.57%, 95.55%, 91.74% and 96.35%, respectively. Therefore, the elimination of MG by ARDV is considerably favored owing to its good performance. This increase in the elimination efficiency of MG dye onto ARDV adsorbent is owing to the usage of NaOH and H2O2 as activation agents [Citation37].
Figure 4. (a) Adsorbent selectivity toward MG [Co = 15 mg/L; adsorbent mass; 0.1 g; pH = neutral; T = 25°C; agitated speed = 100 rpm; contact time = 1440 min), (b) effect of pH on adsorption of MG by ARDV [Co = 15 mg/L; adsorbent mass = 0.1 g; T = 25°C, agitated speed = 100 rpm; contact time = 1440 min], (c) Zeta potential at different pH, (c) effect of adsorbent dose [at Co = 15 mg/L; adsorbent; pH = 6.6; T = 25°C; agitated speed = 100 rpm; contact time = 180 min)], (e) contact time [adsorbent mass = 0.1 g; pH = 6.6; T = 25°C, agitated speed = 100 rpm)], and (f) initial MG concentration on adsorption of MG by ARDV adsorbent [adsorbent mass = 0.1 g; agitated speed = 100 rpm; contact time = 180 min; pH = 6.6].
![Figure 4. (a) Adsorbent selectivity toward MG [Co = 15 mg/L; adsorbent mass; 0.1 g; pH = neutral; T = 25°C; agitated speed = 100 rpm; contact time = 1440 min), (b) effect of pH on adsorption of MG by ARDV [Co = 15 mg/L; adsorbent mass = 0.1 g; T = 25°C, agitated speed = 100 rpm; contact time = 1440 min], (c) Zeta potential at different pH, (c) effect of adsorbent dose [at Co = 15 mg/L; adsorbent; pH = 6.6; T = 25°C; agitated speed = 100 rpm; contact time = 180 min)], (e) contact time [adsorbent mass = 0.1 g; pH = 6.6; T = 25°C, agitated speed = 100 rpm)], and (f) initial MG concentration on adsorption of MG by ARDV adsorbent [adsorbent mass = 0.1 g; agitated speed = 100 rpm; contact time = 180 min; pH = 6.6].](/cms/asset/b79e3dfe-6d2f-4693-8212-113589cd2479/tcsb_a_2168761_f0004_oc.jpg)
3.1.2. Effect of pH
The influence of the solution pH on the adsorption process of MG using ARDV powder was studied in the range of 3.11 to 11 as indicated in . Tests were performed at Co = 15 mg/L; adsorbent mass = 0.1 g; T = 25°C, agitated speed = 100 rpm; contact time = 1440 min. The results showed that the efficiency of MG removal increased from 44.16% to 95.71% with the increase in pH from 3.4 to 6.6 then, the removal efficiency decreased to 91.78% with increased pH from 6.6 to 8.2, owing to precipitation of the methyl green. Besides, when the pH is greater than 8.2, the efficiency of MG removal was augmented to 97.73, due to the cationic MG molecule being converted into the colorless carbinol base (CB) [Citation38]. Therefore, the pH of the solution had a great influence on the ARDV surface and MG dye which can be confirmed by correlating the zeta potential with the pH (). The pHpzc of ARDV was found to be 3.9; thus at a pH< pHpzc (3.9), the ARDV surface has a positive surface charge. Consequently, the electrostatic repulsion increase between a cationic MG molecule and the positive charge of the surface ARDV, resulting in a reduction in the removal efficiency of MG dye and the adsorption capacity of ARDV. With the rise in pH value from 3.4 to 6.6, the adsorbent surface of ARDV became negatively charged owing to the deprotonation of the ARDV surface. Consequently, the electrostatic attraction increases between a cationic MG molecule and the negative charge of the surface ARDV, increasing the removal efficiency of MG dye. Thus, the maximum removal efficiency of MG dye and adsorption capacity of ARDV toward MG dye was 95.71% and 19.14 mg/g, respectively, at pH = 6.6. Similar results have been reported by Alalwan et al. (2021), who investigated MG removal by eggshell waste [Citation39]. In addition, the obtained outcomes were consistent with those articles by researchers who achieved the MG adsorption using various adsorbents [Citation40,Citation41].
3.1.3. Effect of dose
The effect of the adsorbent amount, ranging from 0.01 to 0.2 g, on the MG adsorption onto ARDV adsorbent was studied as shown in . Tests were performed at Co = 15 mg/L; adsorbent; pH = 6.6; T = 25°C; agitated speed = 100 rpm; contact time = 180 min). It is clear that the elimination efficiency of MG dye improved from 79.52% to 95.55% as the amount of the ARDV in the solution increased from 0.01 to 0.1 g and reached a maximum value of 95.6% at 0.1 g. After 0.1 g, the elimination efficiency remains constant. This increase in removal efficiency is basically owing to the existence of more adsorption sites on the ARDV surface with increasing adsorbent dose. On the other hand, the adsorption capacity of ARDV toward MG dye was reduced from 119.29 to 95.55 mg/g as the adsorbent amount increased to 0.1 g. This is owing to the aggregation of adsorbent at a high adsorbent mass which results in a reduction in the total surface area of the ARDV adsorbent. Thus, 0.1 g was selected as the operating adsorbent mass for subsequent studies.
3.1.4. Effect of contact time
The impact of equilibrium time on the efficiency of MG removal by ARDV was analyzed in the time range of 5–240 min at different initial MG concentrations as indicated in . Tests were performed at adsorbent mass = 0.1 g; pH = 6.6; T = 25°C, agitated speed = 100 rpm). The results exhibited that the efficiency of MG removal of MG from its aqueous solution was increased from (56.82% to 93.01%) and (62.06% to 91.74%) with the increased contact time from 5 to 180 min at 15 and 30 mg/L, respectively. The adsorption of MG onto ARDV was rapid at first contact time owing to the availability of vacant sites on the ARDV surfaces. ~62.06% of MG dye was adsorbed in the first 5 min at 30 mg/L. In addition, the results exhibited that the quantity of MG removal rises fast with the initial MG concentration increases may be owing to the increase in the driving force of MG dye and availability of maximum active sites. Thus, the equilibrium time was set at 180 min for the subsequent experiments. Similar findings were reported by Satlaoui et al. [Citation41], who achieved the MG adsorption using activated bentonite from aqueous solutions.
3.1.5. Effect of initial concentration and temperatures
The impact of MG concentrations on the adsorption process was studied over a range of initial MG concentrations from 45 to 200 mg/L at various temperatures (25,45, 65°C) as shown in . Tests were performed at adsorbent mass = 0.1 g; agitated speed = 100 rpm; contact time = 180 min. The results exhibited that the amount of MG adsorption increases from 41.45 to 81.70 mg/g while increasing the concentration of MG from 45 to 200 mg/L at 25°C. This may be due to increasing the driving force that overcomes the mass transfer resistance of MG molecules from the solution to the ARDV surface [Citation42]. The influence of temperature on the MG adsorption on ARDV was also achieved at various concentrations and temperatures. also shows that the amount of MG adsorption increases from 79.21 to 82.71 mg/g at 100 mg/L and from 81.70 to 92.54 mg/g, at 200 mg/L, while increasing the temperature from 25 to 65°C, respectively, indicated that the MG adsorption is endothermic. This is because, with an increase in temperature, the rate of diffusion of the MG ions to the external boundary layer and pores of ARDV was increased [Citation43]. Similar findings were reported by Satlaoui et al. [Citation44], who achieved the MG adsorption using raw and purified sejnane clay type at various temperatures.
3.2. Adsorption modeling
3.2.1. Adsorption isotherm
To determine the maximum adsorption amount of ARDV toward MG dye, three nonlinear isotherm models, namely, Freundlich EquationEq. 4(4)
(4) [Citation45], Langmuir EquationEq. 5
(5)
(5) [Citation46], and Dubinin- R (EquationEq. 6
(6)
(6) , Equation7
(7)
(7) , and Equation8
(8)
(8) ) [Citation47], were used. Tests were performed at various MG concentrations (45–200 mg/L) and different temperatures (25, 45, 65°C); adsorbent mass = 0.1 g; agitated speed = 100 rpm; contact time = 180 min.
where qm (mg/g) is the maximum adsorption capacity of the ARDV; Ce (mg/L) is the content of MG at equilibrium; KL (L/mg) refers to the heat of adsorption (Langmuir constant). KF (mg/g) and n refers to the Freundlich isotherm constant (bonding energy) and the adsorption intensity, respectively. KD-R (mol2/kJ−2) is the D-R constant, ε refers to the Polanyi potential; and E represents the mean free energy (kJ mol−1). The results of the isotherm models employed are listed in . exhibits the model graphs describing the isotherm data for the MG adsorption onto ARDV.
Figure 5. Nonlinear isotherms at different temperature, 25 ͦC (a), 45 ͦC (b), 65 ͦC (c) [conditions: [MG]: 45 − 200 mg/L, adsorbent dose: 0.1 g, time: 180 min, pH:6.6 and agitation: 100 rpm], and (b) Nonlinear kinetic models at 15 mg/L (d) and 30 mg/L for adsorption of MG using ARDV adsorbent (e) [conditions: [MG]: 15 and 30 mg/L, pH:6.6, adsorbent dose: 0.1 g, time: 180 min, T: 25°C and agitation: 100 rpm).
![Figure 5. Nonlinear isotherms at different temperature, 25 ͦC (a), 45 ͦC (b), 65 ͦC (c) [conditions: [MG]: 45 − 200 mg/L, adsorbent dose: 0.1 g, time: 180 min, pH:6.6 and agitation: 100 rpm], and (b) Nonlinear kinetic models at 15 mg/L (d) and 30 mg/L for adsorption of MG using ARDV adsorbent (e) [conditions: [MG]: 15 and 30 mg/L, pH:6.6, adsorbent dose: 0.1 g, time: 180 min, T: 25°C and agitation: 100 rpm).](/cms/asset/a79cc1a5-a318-4980-bd28-c5330697ecfe/tcsb_a_2168761_f0005_oc.jpg)
Table 1. Isotherm data for MG adsorption by ARDV adsorbent [conditions: [MG]: 45 − 200 mg/L, adsorbent dose: 0.1 g, time: 180 min, pH:6.6 and agitation: 100 rpm].
Based on the high value of R2, the order of isotherm models are as follows: Langmuir (R2 = 0.99257)> Dubinin-R (R2 = 0.96457)> Freundlich (R2 = 0.93463) models at 65 ͦC, indicating that the Langmuir model exhibited the best fit to the adsorption process which suggests a monolayer adsorption of MG occurred onto the ARDV surface. The maximum monolayer coverage was 99.03 mg/g at 65°C. Similar trends have been reported by Al-Tameemi et al. (2022) and by Naushad et al. (2019) for the removal of textile dye onto Saccharomyces cerevisiae (Baker’s yeast) [Citation48] and para-aminobenzoic acid functionalized activated carbon, respectively [Citation49]. shows the results of monolayer maximum adsorption of MG dye on various adsorbents, such as Saccharomyces cerevisiae (20.04 mg/g) [Citation48], almond shell (1.1 mg/g) [Citation50], mobil composition of matter No. 41 (18.45 mg/g) [Citation9], bamboo(20.41 mg/g) [Citation51], activated carbon (67.93 mg/g) [Citation52], activated carbon (71.43 mg/g) [Citation53], mesoporous SBA-S4 (39.4 mg/g) [Citation54]. It was observed that the ARDV is a good adsorbent to eliminate MG dye compared with the other adsorbents, which was because of the high oxygen-containing functionalities on the ARDV surface. According to Dubinin-R model, the mean free energy of adsorption was in the range of 0.228–0.339 kJ mol−1 below 8 kJ mol−1 (), indicating that the adsorption of MG by ARDV occurred by physical adsorption.
Table 2. Kinetic data for MG adsorption by ARDV adsorbent [conditions: [MG]: 15 and 30 mg/L, pH:6.6, adsorbent dose: 0.1 g, time: 180 min, T: 25°C and agitation: 100 rpm).
Table 3. Comparison of ARDV with other reported adsorbents.
3.2.2. Kinetics adsorption
To predict the mechanism of the adsorption of MG dye onto ARDV, nonlinear kinetics models namely, pseudo-first-order EquationEq. 9(9)
(9) [Citation55] and pseudo-second-order EquationEq. 10
(10)
(10) [Citation56], were applied. Tests were performed at Co = 15 and 30 mg/L; adsorbent mass = 0.1 g; contact time = 180 min; agitated speed = 100 rpm; T = 25°C.
where qe and qt (mg/g) represent the adsorption capacity of ARDV toward MG at equilibrium and time t (min), respectively. k1 and k2 represent the rate constants of the PFO and PSO, respectively. exhibits the model graphs describing the kinetic data for the MG adsorption onto ARDV and shows the kinetic data of the adsorption process. The results revealed that the best kinetic plot using the proposed equation was of the PSO kinetic model due to the PSO having a good regression coefficient (R2 > 0.99) compared with PFO (R2 > 0.92), respectively. Besides, the adsorbed quantity calculated (qe,cal = 14.11 mg/g) at 15 mg/L and (qe,cal = 27.11 mg/g) at 30 mg/l by the PSO model is closer to the experimental value (qe,exp = 14.31 mg/g) at 15 mg/L and (qe,exp = 27.69 mg/g) at 30 mg/L, indicating the MG dye adsorption might be chemisorption. The existence of oxygen-containing functionalities on the ARDV surface would accelerate the kinetic process of MG molecule adsorption, resulting in a stronger adsorption ability for the MG dye. Similar findings were reported in the literature for elimination of MG by Saccharomyces cerevisiae [Citation48], and activated carbon [Citation52].
3.2.3. Adsorption thermodynamics
The degree of the spontaneity of the adsorption process could be determined by Gibbs free energy (ΔG°) (EquationEq. 11)(11)
(11) and the entropy change (∆S°) and enthalpy change (∆H°) can be calculated by using the van’t Hoff equation EquationEq. 12
(12)
(12) [Citation57]. Tests were performed at different MG concentrations (45–200 mg/L) and temperatures (25, 45, 65°C); adsorbent mass = 0.1 g; contact time = 180 min; agitated speed = 100 rpm.
where Kc refers to the thermodynamic equilibrium constant [Citation58]. The values of (ΔH°) and (ΔS°) are acquired by the plot of lnKc versus 1 /T (). shows the thermodynamic parameters for the adsorption process.
Table 4. Thermodynamic parameters for MG adsorption by ARDV adsorbent [conditions: [MG]: 45 − 200 mg/L, adsorbent dose: 0.1 g, time: 180 min, pH:6.6 and agitation: 100 rpm].
The negative values of ΔG° indicate the feasibility of adsorption process and its spontaneous nature. These values reduced with rise in temperatures, indicating favorable adsorption at a higher temperature. The positive values of ΔH° indicate that the adsorption process was endothermic, which was supported by the improvement of the removal efficiency of MG with an rise in temperature. The positive values of ΔS° indicate the good affinity of ARDV adsorbent toward MG and the increasing randomness during the adsorption process. Besides, the ΔH° values were in the range between 13.43 and 33.81 kJ mol−1 which indicated that the adsorption process is in the physical adsorption range due to the ΔH° <40 kJ mol−1.
3.3. Adsorption mechanism
To understand the mechanism of the adsorption process, it is necessary to know the structure of the adsorbent and adsorbate. MG molecule dye is a basic triphenylmethane-type dicationic dye with amine groups in its structure. On the other hand, the ARDV adsorbent is a plant material consisting of tannins, flavonoids, alkaloids, saponins, triterpenoids, steroids, volatile oil and phytosterols. These important materials have the majority of functional groups, such as COOH, -OH, C = O groups and highly aromatic rings. Based on the structure of the MG dye, ARDV and experimental results of kinetic, isotherm, thermodynamic, FTIR and EDX analysis, we can determine the adsorption mechanism of MG onto ARDV adsorbent. Based on FTIR results, the strong adsorption band of at – C = C–group was decreased in intensity and shifted from 1681 to 1600 cm−1 owing to π–π interactions between MG molecules with – C = C–onto the surface of ARDV. While the band of – OH groups was increased in intensity and a slight shift from 3330 to 3335 owing to hydrogen bond formation between the – N(CH3)2 group of MG molecules and the – OH group in the RDV surface. The band of C = O stretch vibration of carboxylic acid was a slight increase in intensity due to electrostatic attraction between a cationic +N(CH3)3+ group of MG molecules with the negative charge of COOH group onto the ARDV surface. According to the results of thermodynamics, the adsorption mechanism is a physical process due to a low ΔH° values. Therefore, these results provide enough evidence to support the MG adsorption onto the ARDV surface by different mechanisms such as hydrogen bonding, electrostatic interaction and π-π interactions ().
4. Conclusion
In conclusion, activated residual Dodonaea Viscosa (ARDV) is a promising adsorption material for methyl green molecule (MG) removal from aqueous solutions. The batch method with the influence of parameters such as pH, temperature, contact time, adsorbent dose and initial MG concentration on the adsorption of MG dye were optimized. The results exhibited that the high adsorption of MG dye was found at pH = 6.6, [ARDV] = 0.1 g, time = 180 min and temperature = 65°C. Adsorption equilibrium was satisfactorily represented by the PSO and the Langmuir models, with adsorption capacity of 99 mg/g. The interaction between ARDV and MG dye occurred through physical adsorption through π–π interactions, hydrogen bonding and electrostatic attractions. The thermodynamic data confirms that the MG adsorption was endothermic and spontaneous, and their values support the physical adsorption mechanism.
Acknowledgments
We gratefully acknowledge the financial support by Albaha University (Project No. 1441/3) and are grateful to the Scientific Research Deanship.
Disclosure statement
No potential conflict of interest was reported by the author(s).
Correction Statement
This article has been corrected with minor changes. These changes do not impact the academic content of the article.
References
- Alshahrani AA, Alorabi AQ, Hassan MS, et al. Chitosan-functionalized hydroxyapatite-cerium oxide heterostructure: an efficient adsorbent for dyes removal and antimicrobial agent. Nanomaterials. 2022;12(15):2713.
- Rummi D. Saini textile organic dyes : polluting effects and elimination methods from textile waste water. Int J Chem Eng Res@. 2017;9:121–13.
- Saleh TA, Gupta VK. Column with CNT/magnesium oxide composite for lead(II) removal from water. Environ Sci Pollut Res. 2012;19(4):1224–1228.
- Alorabi AQ, Hassan MS, Alam MM, et al. Natural clay as a low-cost adsorbent for crystal violet dye removal and antimicrobial activity. Nanomaterials. 2021;12(1):11.
- Saleh TA. Nanomaterials: classification, properties, and environmental toxicities. Environ Technol Innovation. 2020;20:101067.
- Alorabi AQ. Effective removal of malachite green from aqueous solutions using magnetic nanocomposite: synthesis, characterization, and equilibrium study. Adsorpt Sci Technol. 2021;2021:2359110.
- Aldawsari AM, Alsohaimi IH, Al-Kahtani AA, et al. Adsorptive performance of aminoterephthalic acid modified oxidized activated carbon for malachite green dye: mechanism, kinetic and thermodynamic studies. Sep Sci Technol. 2021;56(5):835–846.
- Muhd Julkapli N, Bagheri S, Bee Abd Hamid S. Recent advances in heterogeneous photocatalytic decolorization of synthetic dyes. Sci World J. 2014;2014:692307.
- Alardhi SM, Alrubaye JM, Albayati TM. Adsorption of methyl green dye onto MCM-41: equilibrium, kinetics and thermodynamic studies. Desalin Water Treat. 2020;179:323–331.
- Nezamzadeh-Ejhieh A, Shahriari E. Heterogeneous photodecolorization of methyl green catalyzed by Fe (II)-o-phenanthroline/zeolite Y nanocluster. Int J Photoenergy. 2011.
- Mohsen Alardhi S, Alrubaye JM, Albayati TM. Removal of methyl green dye from simulated waste water using hollow fiber ultrafiltration membrane. IOP Conf Ser Mater Sci Eng. 2020;928(5):052020.
- Tatarchuk T, Danyliuk N, Shyichuk A, et al. Photocatalytic degradation of dyes using rutile TiO2 synthesized by reverse micelle and low temperature methods: real-time monitoring of the degradation kinetics. J Mol Liq. 2021;342:117407.
- Bousnoubra I, Djebbar K, Abdessemed A, et al. Decolorization of methyl green and bromocresol purple in mono and binary systems by photochemical processes: direct UV photolysis, Acetone/UV and H2O2/UV. A comparative study. Desalin Water Treat. 2016;57:27710–27725.
- Melhi S, Algamdi M, Alqadami AA, et al. Fabrication of magnetically recyclable nanocomposite as an effective adsorbent for the removal of malachite green from water. Chem Eng Res Des. 2022;177:843–854.
- AL-Shehri HS, Alanazi HS, Shaykhayn AM, et al. Adsorption of methylene blue by biosorption on alkali-treated solanum incanum: isotherms, equilibrium and mechanism. Sustainability. 2022;14(5):2644.
- Alhumaimess MS, Alsohaimi IH, Alqadami AA, et al. Synthesis of phosphorylated raw sawdust for the removal of toxic metal ions from aqueous medium: adsorption mechanism for clean approach. J Sol Gel Sci Techn. 2019;89(2):602–615.
- Dai M. The effect of zeta potential of activated carbon on the adsorption of dyes from aqueous solution: i. The adsorption of cationic dyes: methyl green and methyl violet. J Colloid Interface Sci. 1994;164(1):223–228.
- Sharma P, Saikia BK, Das MR. Removal of methyl green dye molecule from aqueous system using reduced graphene oxide as an efficient adsorbent: kinetics, isotherm and thermodynamic parameters. Colloids Surf A Physicochem Eng Asp. 2014;457:125–133.
- Farghali AA, Bahgat M, El Rouby WMA, et al. Preparation, decoration and characterization of graphene sheets for methyl green adsorption. J Alloys Compd. 2013;555:193–200.
- Saleh TA. Protocols for synthesis of nanomaterials, polymers, and green materials as adsorbents for water treatment technologies. Environ Technol Innovation. 2021;24:101821.
- Lin C-H, Gung C-H, Wu J-Y, et al. Cationic dye adsorption using porous composite membrane prepared from plastic and plant wastes. J Taiwan Inst Chem Eng. 2015;51:119–126.
- Alkadir OKA, Taifi A, Aljeboree AM, et al. Environmental removal of some dyes from aqueous solution by using highly adsorbent surface derived from malva parviflora plant leaves: multifactors studies. IOP Conf Ser Earth Environ Sci. 2022;1029:12022.
- Peydayesh M, Rahbar-Kelishami A. Adsorption of methylene blue onto Platanus orientalis leaf powder: kinetic, equilibrium and thermodynamic studies. J Ind Eng Chem. 2015;21:1014–1019.
- Shaikh WA, Chakraborty S, Islam RU, et al. Fabrication of biochar-based hybrid Ag nanocomposite from algal biomass waste for toxic dye-laden wastewater treatment. Chemosphere. 2022;289:133243.
- Yagub MT, Sen TK, Ang HM. Equilibrium, kinetics, and thermodynamics of methylene blue adsorption by pine tree leaves. Water, Air, & Soil Pollution. 2012;223(8):5267–5282.
- Han R, Wang Y, Zhao X, et al. Adsorption of methylene blue by Phoenix tree leaf powder in a fixed-bed column: experiments and prediction of breakthrough curves. Desalination. 2009;245(1–3):284–297.
- Jain SN, Gogate PR. NaOH-treated dead leaves of ficus racemosa as an efficient biosorbent for Acid Blue 25 removal. Int J Environ Sci Technol. 2017;14(3):531–542.
- Marvilliers A, Illien B, Gros E, et al. Modified clerodanes from the essential oil of dodonea viscosa leaves. Molecules. 2020;25(850):850.
- Lawal D, Yunusa IA. Dodonea viscosa linn: its medicinal, pharmacological and phytochemical properties. Int j innov appl res studies@. 2013;2:477–483.
- Alghamdi MD, Nazreen S, Ali NM, et al. ZnO nanocomposites of juniperus procera and dodonaea viscosa extracts as antiproliferative and antimicrobial agents. Nanomaterials. 2022;12(4):664.
- Ndazi BS, Karlsson S, Tesha JV, et al. Chemical and physical modifications of rice husks for use as composite panels. Compos Part A Appl Sci Manuf. 2007;38(3):925–935.
- Sharma S, Sharma G, Kumar A, et al. Adsorption of cationic dyes onto carrageenan and itaconic acid-based superabsorbent hydrogel: synthesis, characterization and isotherm analysis. J Hazard Mater. 2022;421:126729.
- Han X, Wang W, Ma X. Adsorption characteristics of methylene blue onto low cost biomass material lotus leaf. Chem Eng J. 2011;171(1):1–8.
- Li H-Z, Zhang Y-N, Guo J-Z, et al. Preparation of hydrochar with high adsorption performance for methylene blue by co-hydrothermal carbonization of polyvinyl chloride and bamboo. Bioresour Technol. 2021;337:125442.
- Anand SP, Doss A, Nandagopalan V. Qualitative and quantitative analysis of phytochemicals in aerva javanica (Burm. F) shult. Acta Biomedica Scientia. 2014;1:93–97.
- Bedin KC, Martins AC, Cazetta AL, et al. KOH-activated carbon prepared from sucrose spherical carbon: adsorption equilibrium, kinetic and thermodynamic studies for methylene blue removal. Chem Eng J. 2016;286:476–484.
- Guo Y, Yang S, Yu K, et al. The preparation and mechanism studies of rice husk based porous carbon. Mater Chem Phys. 2002;74(3):320–323.
- Vargas-Rodríguez YM, Obaya A, García-Petronilo JE, et al. Adsorption studies of aqueous solutions of methyl green for halloysite nanotubes: kinetics, isotherms, and thermodynamic parameters. Am J Nanomater. 2021;9(1):1–11.
- Alalwan HA, Mohammed MM, Sultan AJ, et al. Adsorption of methyl green stain from aqueous solutions using non-conventional adsorbent media: isothermal kinetic and thermodynamic studies. Bioresour Technol Rep. 2021;14:100680.
- Ben Mosbah M, Alsukaibi AK, Mechi L, et al. Ecological synthesis of CuO nanoparticles using punica granatum L. Peel extract for the retention of methyl green. Water. 2022;14(9):1509.
- Maghni A, Ghelamallah M, Benghalem A. Sorptive removal of methyl green from aqueous solutions using activated bentonite. Acta Phys Polonica A. 2017;132(3):448–450.
- Eltaweil AS, Abd El-Monaem EM, El-Subruiti GM, et al. Fabrication of UiO-66/MIL-101(Fe) binary MOF/carboxylated-GO composite for adsorptive removal of methylene blue dye from aqueous solutions. RSC Adv. 2020;10(32):19008–19019.
- Arellano-Cárdenas S, López-Cortez S, Cornejo-Mazón M, et al. Study of malachite green adsorption by organically modified clay using a batch method. Appl Surf Sci. 2013;280:74–78.
- Satlaoui Y, Trifi M, Fkih Romdhane D, et al. Mechanisms, and performance of methyl green from aqueous solution using raw and purified sejnane clay type. J Chem. 2019;2019:4121864.
- Freundlich H. Über die Adsorption in Lösungen . Z Phys Chem. 1907;57U(1):385.
- Wallis A, Dollard MF. Local and global factors in work stress - The Australian dairy farming examplar. Scand J Work Environ Health Suppl. 2008;(6):66–74.
- Dubinin MM. The equation of the characteristic curve of activated charcoal. Proceedings of the academy of sciences. Phys Chem Section. 1947;55:331.
- Al-Tameemi MSM, Mohammed M, Al-Sadoon AAT. Study the ability of Saccharomyces cerevisiae to remove methyl green dye from water as a pollutant agent. J Chem Health Risks. 2022;12:93–104.
- Naushad M, Alqadami AA, Al-Kahtani AA, et al. Adsorption of textile dye using para-aminobenzoic acid modified activated carbon: kinetic and equilibrium studies. J Mol Liq. 2019;296:112075.
- Tanaydin MK, Goksu A. Optimization of the adsorption of methyl green dye on almond shells using central composite design. Desalin Water Treat. 2021;227:425–439.
- Atshan AA. Adsorption of methyl green dye onto bamboo in batch and continuous system. Iraqi j chem pet eng. 2014;15:65–72.
- Rida K, Chaibeddra K, Cheraitia K. Adsorption of cationic dye methyl green from aqueous solution onto activated carbon prepared from brachychitonpopulneus fruit shell. Ind J Chem Technol. 2020;27:51–59.
- Belgacem A, Ould Brahim I, Belmedani M, et al. Removal of methyl green dye from aqueous solutions using activated carbon derived from cryogenic crushed waste tires. Iran J Chem Chem Eng (IJCCE). 2022;41:207–219.
- Arjomandi-Behzad L, Rofouei MK, Badiei A, et al. Simultaneous removal of crystal violet and methyl green in water samples by functionalised SBA-15. Int J Environ Anal Chem. 2020;1–17. 10.1080/03067319.2020.1804895
- Lagergren S. About the theory of so-called adsorption of soluble substances. Handlingar. 1898;24:1–39.
- Wingenfelder U, Hansen C, Furrer G, et al. Removal of heavy metals from mine waters by natural zeolites. Environ Sci Technol. 2005;39(12):4606–4613.
- Alghamdi AA, Al-Odayni A-B, Saeed WS, et al. Adsorption of azo dye methyl orange from aqueous solutions using alkali-activated polypyrrole-based graphene oxide. Molecules. 2019;24(20):3685.
- Lee LY, Gan S, Yin Tan MS, et al. Effective removal of acid blue 113 dye using overripe cucumis sativus peel as an eco-friendly biosorbent from agricultural residue. J Clean Prod. 2016;113:194–203.