ABSTRACT
Di(2-ethylhexyl) phthalate (DEHP) is an extensively used plasticizer and being non-covalently bound to plastics it leaches out into the environment. This addresses a grave concern on its potential human exposure and subsequent deleterious effects. The ameliorative potential of Spirulina (Arthrospira platensis) against DEHP-induced toxicity was assessed in rats. Group I was control. Group II and III, were exposed to a low dose of DEHP (125 mg/kg), and Group IV and V were exposed to a high dose of DEHP (250 mg/kg). Group III and V were treated with spirulina orally (1000 mg/Kg) for 3 weeks. Exposure to DEHP elicited marked oxidative stress and associated DNA damage in a dose-dependent manner. The hepatic expression of p53 was also significantly suppressed. Liver and kidney function tests showed marked alterations. Treatment with spirulina efficaciously reversed the adverse effects. Thus, these findings offer a nutritional intervention of spirulina to combat the environmental exposure to plasticizers.
GRAPHICAL ABSTRACT
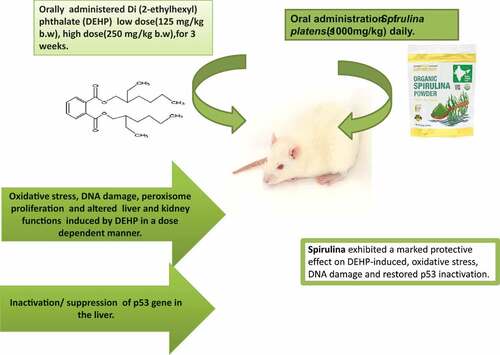
1. Introduction
Environmental exposure to several chemicals can plausibly interfere with the genetic and epigenetic processes which can affect the normal growth and development of an organism [Citation1]. Among these exposures, endocrine-disrupting chemicals (EDCs) are of significant public concern due to their ubiquitous presence, extensive use and continual human exposure [Citation2]. Most commonly reported EDCs are bisphenol A and phthalates. BPA and phthalates are commonly used plasticizers in the plastic industry which has been thriving even more successfully during the ongoing pandemic. The UNEP Report UN Environment Annual Report [Citation3], shows that the annual global production of plastic has been more than 400 million tons. However, post COVID-19 the plastic production has escalated due to the upsurge in the demand for protective equipment (PPEs) and single use face masks and plastic products [Citation4]. Subsequently, the global plasticizer market is steered by an increase in the demand and production of flexible PVC (poly vinyl chloride) due to the parallel growth in the packaging/plastic industry. The plasticizer di(2-ethylhexyl) phthalate (DEHP) offers wide application in the manufacture of polyvinyl chloride-containing products (PVC)such as medical and consumer goods. Human exposure is inevitable due to its ubiquitous environmental presence which addresses grave health concerns. Food packaging, medical devices and toys are considered as the primary source of exposure to DEHP in our daily lives [Citation5]. Since DEHP is non-covalently bound to plastics, it can easily leach out into the environment at high temperatures or on contact with hydrophobic materials. Its presence in air is easily detected as it does not evaporate easily. Furthermore, DEHP binds to dust particles in air, to soil, and dissolves gradually in groundwater [Citation6]. Thus, DEHP is a pervasive contaminant in the human body, wildlife, and the environment (CDC, 2018). Its major monoester metabolite, mono-2-ethylhexyl phthalate (MEHP), has been reported to be more toxic than the parent compound [Citation2]. DEHP has been reported to be carcinogenic and causes reproductive, developmental, neuro and immune toxicity in addition to endocrine disruption in rodents [2]. Among DEHP-induced toxicities [20–22], hepatic carcinogenicity was the most significant issue reported in the past three decades. In addition, DEHP is a well-known peroxisome proliferator (PP) and is also nephro-hepatotoxic. It primarily acts via generation of reactive oxygen species that results in oxidative stress and DNA damage. Furthermore, p53, a key target of the JNK/p38MAPK signaling pathway and has been reported to be instrumental in DEHP-induced hepatotoxicity [Citation7,Citation8]. Apoptosis and senescence in the liver and reproductive tract of rodents due to phthalate exposure through the induction of p53 gene, a tumor suppressor gene has been reported [Citation9]. The enhancement of ROS during oxidative stress leads to lipid peroxidation (LPO) of polyunsaturated fatty acids in cell membranes. This further causes an increase in the MDA levels and modulation of activities of antioxidant enzymes such as SOD and catalase [Citation8]. It has been reported since ancient times that in all cultures, plants with therapeutic benefits play a vital role in disease prevention. Spirulina, a bluegreen algae has three species, Spirulina platensis, Spirulina maxima and Spirulina fusiformis [Citation10]. Arthrospira platensis commonly known as spirulina is a fresh water species (Oscillatoriaceae) occurs in India, China and the U.S.A. Spirulina is a nutrient dense filamentous cyanobacterium exhibiting potent antioxidant, immunomodulatory, and anti-inflammatory properties. In the current times, it is a widely used nutraceutical and food supplement [Citation11,Citation12]. The chemical constituents of Arthrospira platensis are protein (55%–70%), carbohydrates (15%–20%), lipids (approximately 7%), fiber, ash, and water, including various minerals, vitamins, γ-linolenic acid, chlorophyll, carotenoids, and phycocyanin [Citation11]. The key constituent in the alga attributed to its antioxidative activity is the phycocyanin. Spirulina platensis is thus well recognized for its antioxidant and anti-cancerous properties as well as its ability of carcinogen-induced DNA repair [Citation13]. Keeping this premise, the present study was designed to investigate the protective effect of Arthrospira platensis on DEHP (a phthalate)-induced toxicity in adult female Wistar rats.
2. Materials and methods
2.1. Chemicals and kits
Analytical grade Di (2-ethylhexyl) phthalate (DEHP) was procured from Lobachemie, India. Organic spirulina (Arthrospira platensis) powder was purchased from iHerb (California, CA). Commercial ELISA kits for Catalase (CAT) activity and Malondialdehye (MDA) were purchased from Abcam (Cambridge, UK). Commercial ELISA kits Serum 8-hydroxydeoxyguanosine (8-OHdG), acyl‐CoA oxidase (AOX) and p53 protein were purchased from MyBioSource (U.S.A) (). For cDNA synthesis, the PureLink RNA mini kit Quick Reference was used (Qiagen, Limburg, Netherlands).
2.2. Spirulina
Organic spirulina powder is manufactured by California Gold Nutrition ® (Perris, CA, U.S.A) which contains the blue-green algae, Arthrospira platensis which is commonly known as spirulina (biomass of cyanobacteria). It is a USDA approved nutraceutical. The nutritional facts include; total fat 0%, cholesterol 0%, sodium 20 mg 1%, total carbohydrate <1%, protein 2 g and iron 1.3 mg 8%. Spirulina Powder (15 g) was dissolved in 100 ml of distilled water [Citation12] to be administered to the rats orally.
2.2.1. Phytochemical screening
Phytochemical screening based on total phenolic content and total flavonoids was performed for the spirulina powder using colorimetric assay. The total phenol content was quantitated using the Folin Ciocalteu reagent with gallic acid as the standard [Citation14], while total flavonoids were quantitated [Citation15] and expressed as catechin in milligrams equivalents per gram of the sample (mg/g).
2.2.2. Determination of in vitro antioxidant activity
The antioxidative activity of the spirulina (Arthrospira platensis) was measured by three complementary tests.
2.2.2.1. 2,2′-azino-bis(3-ethylbenzothiazoline-6-sulfonic acid) (ABTS•+) radical scavenging activity
ABTS radical-scavenging activity was evaluated in accordance with the protocol given by Re et al. with some modifications [Citation16]. Trolox was used as a standard for the assay and the optical density (OD) was recorded at 734 nm. The results were expressed in terms of g trolox equivalent per g of dry weight (g eq. trolox/g).
2.2.2.2. α, α-diphenyl-β-picrylhydrazyl (DPPH) radical scavenging activity
This assay was performed in accordance with the standard protocol by [Citation17]. The OD of the solutions was measured at 515 nm. The DPPH radical scavenging activity was illustrated as percentage inhibition and computed as follows:
DPPH scavenging activity(%)=(A0-A1)/A0×100, where A0 is the OD of the control and A1 is the OD of the sample.
2.2.2.3. Ferric reducing antioxidant power (FRAP) assay
A FRAP assay with certain modifications as previously reported by Benzie and Strain [Citation18] was performed keeping Trolox as a standard for the assay and the absorbance of the samples was measured at 593 nm. The FRAP results were expressed in terms of g Trolox equivalent per g of dry weight (g eq.Trolox/g).
2.3. Experimental design
Adult female Wistar rats (Total = 60), weighing 150 ± 10 g, were procured from the Animal House Facility at King Saud University, Riyadh. The study conformed to the standards within the national and institutional guidelines for the protection of animal welfare. The rats were acclimated to the laboratory conditions at 22 ± 2°C in cages and maintained under a 12 h light/dark cycle. The rats were fed commercial diet and given tap water ad libitum. After the period of acclimation (7 days), rats were randomly allocated into six groups comprising 10 rats each. The rats were administered two oral doses of (DEHP), a low and a high dose in corn oil followed by the treatment with spirulina (Arthrospira platensis) supplement as an oral dose for a period of 21 days. The doses of DEHP and spirulina were co-administered.
Group I - control was administered distilled water.
Group II- received a low oral dose of DEHP (125 mg/kg b.w) [Citation8].
Group III- received a high oral dose of DEHP (250 mg/kg b.w) [Citation8].
Group IV- received the low oral dose of DEHP (125 mg/kg b.w) and was treated with an oral dose of spirulina (1000 mg/kg) [Citation12].
Group V- received the low oral dose of DEHP (250 mg/kg b.w) and was treated with an oral dose of spirulina (1000 mg/kg) [Citation12].
Group VI- received only an oral dose of spirulina (1000 mg/kg) [Citation12].
After an exposure period of 3 weeks, whole blood samples were collected from all rats. Serum was prepared to assess the liver and kidney functions and other biomarkers of oxidative stress. In addition, tissue samples of liver and kidneys were excised for bioassays. All tissue and serum samples were stored at −80°C till further analysis
2.4. Estimation of biomarkers of oxidative stress
Biomarkers of oxidative damage, serum levels of 8-hydroxydeoxyguanosine (8-OHdG), hepatic malondialdehyde (MDA) levels, catalase (CAT) activity and Acyl‐CoA Oxidase (ACOX1) activity in the liver were assessed using commercial ELISA kits in accordance to the manufacturer’s protocol.
2.5. Gene expression
The assay was performed according to the manufacturer’s instructions.
For cDNA synthesis, the PureLink RNA mini kit Quick Reference was used (Qiagen, Limburg, Netherlands).
After genomic DNA elimination, the RNA sample was used for reverse transcription using a master mix prepared from Solis Green qPCR Mix, Primer forward and Revers, DNA template and H2O PCR grade. An aliquot of each tube was added to a real-time polymerase chain reaction (PCR) mix.
Real-time PCR:
A two-step process was employed for cytokine mRNA quantification (SYBR Green PCR Master Mix kit, Qiagen). Following the synthesis reaction, 2× QuantiTect SYBR Green PCR Master Mix was thawed. cDNA for liver from the experimental groups with specific forward and reverse primers for β-Actin (housekeeping gene) were used. The procedure was repeated using specific forward and reverse primers for the target gene p53 (). The amplifications were done in duplicates with a real-time PCR instrument (7500, Applied Biosystems, Grand Island, U.S.A) with an initial activation at 95°C, denaturation at 95°C, annealing at 62°C, and extension at 72°C. The ΔCT value was calculated by the subtraction of the β-actin CT from each p53 CT. The ΔΔCT value was calculated by subtraction of the control ΔCT from each p53 ΔCT. The expression relative to the control was calculated using the equation 2−ΔΔCT.
Table 1. Phytochemical profile and the antioxidant activity of Spirulina (Arthrospira platensis) powder.
2.6. Liver and kidney function tests
Serum levels of aspartate aminotransferase (AST) and alanine aminotransferase (ALT) were measured using a Beckman Coulter AU680 (Switzerland). Levels of urea and serum creatinine were also measured using a Beckman Coulter AU680 (Switzerland). The analysis was done in accordance with the manufacturer’s protocol.
2.7. Statistical analysis
All presented data is expressed as mean ± standard error (SE). One way analysis of variance (ANOVA) was performed followed by Tukey’s test to analyze group differences. Numerical values were correlated with SPSS 22.0 statistical software Chicago, IL, U.S.A. The significance level was set to p ≤ 0.05.
3. Results
3.1. Phytochemical profile and antioxidant activity
The phytochemical content; total phenols (72.177 ± 0.719 mg gallic acid/g sample), total flavonoids (1.204 ± 0.024 mg catachin/g sample) of the spirulina (Arthrospira platensis) powder used is illustrated in . In addition, the free radical scavenging property of the spirulina powder was assessed by three complementary in vitro antioxidant assays. In the DPPH assay, the radical scavenging activity with a percent inhibition of 34.342 ± 0.331 was observed. The antioxidant activity observed in the ABTS assay was 1.196 ± 0.005 g equiv. Trolox/g sample while the FRAP assay showed a value of 1.474 ± 0.025 equiv.Trolox/g sample)().
Table 2. Primers for target and housekeeping gene.
3.2. Biomarkers of oxidative stress
Exposure to high dose (HD) of DEHP for 21 days significantly (p ≥ 0.05) enhanced the CAT activity in the liver (0.3567 ± 0.0063 nmol/min/ml) as compared to the control (0.2534 ± 0.01424 nmol/min/ml). Exposure to the low dose (LD) also showed a significant (p ≥ 0.05) increase in the CAT activity (0.3742 ± 0.0028 nmol/min/ml) compared to the control. Treatment with Spirulina significantly (p ≥ 0.05) decreased the CAT activity in both treated groups, HD+S (0.2253 ± 0.0015 nmol/min/ml) and LD+S (0.2555 ± 0.0038 nmol/min/ml). The group treated with only Spirulina (S) showed no significant difference from the control ().
Figure 1. (A)mean (±SE) levels of CAT (nmol/min/ml) in the liver of rats exposed to high dose (HD) and low dose (LD) of DEHP and treated with spirulina (HD+S) and (LD+S).

Exposure to the high dose (HD) of DEHP for 21 days showed a significant (p ≥ 0.05) increase in the MDA levels (0.0517 ± 0.0016 nmol/mg) as compared to the control (0.0196 ± 0.0012 nmol/mg). Exposure to the low dose (LD) also showed a significant (p ≥ 0.05) increase in the MDA levels (0.0437 ± 0.00046 nmol/mg) compared to the control. Treatment with Spirulina significantly (p ≥ 0.05) reduced the MDA levels in both treated groups, HD+S (0.0301 ± 0.0024 nmol/mg) and LD+S (0.0342 ± 0.0022 nmol/mg). The group treated with only Spirulina (S) showed no significant difference from the control (). The serum the 8-OHdG levels were significantly (p ≥ 0.05) enhanced upon exposure to high dose (HD) of DEHP for 21 days (174.9210 ± 0.3780 pg/ml) as compared to the control (52.790 ± 0.3079 pg/ml). Exposure to the low dose (LD) also showed a significant (p ≥ 0.05) increase in the 8-OHdG levels (100.9365 ± 1.7210 pg/ml) compared to the control. Treatment with Spirulina significantly (p ≥ 0.05) decreased the 8-OHdG levels in both treated groups, HD+S (154.4670 ± 6.6479 pg/ml) and LD+S (82.71 ± 1.1133 pg/ml). The group treated with only Spirulina (S) was not significantly different from the control (). Exposure to high dose (HD) of DEHP elicited a significant (p ≥ 0.05) increase in the AOX levels (7.2746 ± 0.0539 ng/ml) as compared to the control (6.4338 ± 0.0153 ng/ml). Exposure to the low dose (LD) also showed a significant (p ≥ 0.05) increase in the ACOX1 levels (7.6086 ± 0.0520 ng/ml) compared to the control. Treatment with Spirulina significantly (p ≥ 0.05) reduced the ACOX1 levels in both the groups, HD+S (6.8480 ± 0.0832 ng/ml) and LD+S (6.7130 ± 0.0585 ng/ml). The group treated with only Spirulina (S) showed no significant difference from the control ().
Figure 2. Mean (±SE) levels of MDA (nmol/mg) in the liver of rats exposed to high dose (HD) and low dose (LD) of DEHP and treated with spirulina (HD+S) and (LD+S).

3.3. Expression of hepatic p53 gene
The expression of β-actin was not affected by experimental exposure to DEHP (both doses; low and high) and treatment with spirulina, therefore the use of β-actin as a housekeeping gene was justified. The expression of the selected target DNA tumor suppressor gene, p53 analyzed in the liver was significantly (p ≤ 0.05) downregulated on exposure to both the low and high doses of DEHP in comparison to the negative control. Supplementation with Spirulina enhanced the expression of the gene. However, the effect observed was statistically significant (p ≤ 0.05) in the group exposed to a high dose of DEHP expression and treated with Spirulina (HD+S). The group treated only with spirulina was statistically non-significant with the negative control ().
3.4. Liver and kidney function tests
A 21 day of exposure to high dose (HD) of DEHP showed a significant (p ≥ 0.05) increase in the serum ALT levels (78.600 ± 0.2309 U/L) as compared to the control (62.500 ± 0.2309 U/L). Exposure to the low dose (LD) also showed a significant (p ≥ 0.05) increase in the ALT levels (85.450 ± 0.0288 U/L) compared to the control. Treatment with Spirulina significantly (p ≥ 0.05) reversed the effect in both treated groups, HD+S (62.600 ± 0.2309 U/L) and LD+S (55.00 ± 2.829 U/L). The group treated with only Spirulina (S) showed no significant difference from the control (). Exposure to high dose (HD) of DEHP showed a significant (p ≥ 0.05) increase in the serum ALT levels (201.86 ± 0.2107 U/L) as compared to the control (130.650 ± 9.4974 U/L). Similarly, exposure to the low dose (LD) also significantly (p ≥ 0.05) enhanced the ALT levels (255.90 ± 0.1154 U/L) compared to the control. Treatment with Spirulina showed a significant (p ≥ 0.05) reduction in the ALT levels in both treated groups, HD+S (156.25 ± 18.792 U/L) and LD+S (125.650 ± 7.7653 U/L) ().
Figure 6. Mean (±SE) levels of ALT and AST (U/L) in the liver of rats exposed to high dose (HD) and low dose (LD) of DEHP and treated with spirulina (HD+S) and (LD+S).

Exposure to high dose (HD) of DEHP as well as the low dose (LD) for 21 days did not show any significant change in the serum creatinine levels in all experimental groups (). The serum urea levels were significantly (p ≥ 0.05) enhanced on exposure to high dose (HD) of DEHP (44.975 ± 1.0017 mg/dl) as compared to the control (33.00 ± 0.2886 mg/dl). Exposure to the low dose (LD) also showed (p ≥ 0.05) enhanced urea levels (43.230 ± 0.4272 mg/dl) compared to the control. Treatment with Spirulina significantly (p ≥ 0.05) reduced the urea levels in the group exposed to the high dose, HD+S (42.41 ± 0.2944 mg/dl). However, treatment with Spirulina in the low dose group showed no significant effect. The group treated with only Spirulina (S) was comparable to the control ().
4. Discussion
The key findings of the present study showed that exposure to DEHP in rats for a period of 3 weeks induced oxidative stress and associated DNA damage, which was evident by marked alterations in the biomarkers assessed. Generation of free radicals and subsequent oxidative stress have been incriminated in the phthalate-induced toxicity [Citation7]. Exposure to DEHP-induced lipid peroxidation, which was reflected by enhanced MDA levels in the liver. It has been recognized that an increase of ROS can lead to the oxidation of polyunsaturated fatty acids in cell membranes that results in the formation of MDA, a well-established biomarker of oxidative stress [Citation8]. Further, exposure to DEHP in rats also induced the CAT activity in the liver since it acts as a frontline defense against the free radicals that induce oxidative stress. This is in line with a study that reported a significant increase in hepatic CAT activity on DEHP exposure for 5 weeks [Citation19]. In addition, there was a parallel oxidative DNA damage observed.8-OHdG, the biomarker of DNA damage, was significantly elevated in a dose-dependent manner. This was in consensus with the findings of a study by Ha et al. [Citation7] who reported a DNA damage on exposure to DEHP in rat liver and hepatocytes. Studies have also reported that the DEHP and its major metabolite MEHP caused DNA damage in human cell lines (in vitro) and leukocytes and showed an enhanced tail moment in Comet assay [Citation20,Citation2]
The tumor suppressor protein, p53 is a redox sensitive protein known to be vital in controlling the integrity and correctness of all processes in each individual cell. Activation of p53 gene by ROS and exogenous DNA damages can lead to growth arrest of the cell, DNA repair induction or apoptosis [Citation2122Citation22. Several environmental chemicals, including phthalates, have been shown to induce apoptosis in the reproductive tract of rodents through p53 induction [Citation21]. The expression of p53 is dependent on its negative regulator Mdm2 and oxidative stress causes a degradation in the Mdm2 which contributes to the accumulation and activation of p53 [Citation7]. On the other hand, the inactivation of the p53 tumor suppressor gene is a frequent event in tumorigenesis, and impairment of the p53 pathway is a critical event in cancer [Citation23]. This explains the results of the current study, which showed a significant suppression of hepatic p53 gene on exposure to both doses of DEHP. Phthalates such as DEHP have been recognized as possible human carcinogens based on previous literature on their carcinogenicity that has been reported in experimental animal studies, as well as genotoxicity assays and cellular studies [Citation24] Treatment with spirulina restored the expression of p53 gene. Reactivation of p53 has been reported as a therapeutic strategy. Several genetic studies have shown that the loss of p53 induces tumor formation in mice, whereas its restoration leads to a rapid regression of in situ tumors, further showing the cancer-therapeutic potential of p53 restoration. Over the last two decades, a number of distinct therapeutic strategies have been pursued to restore p53 function for cancer treatment [Citation23]. Phthalates have been known as hepatocarcinogenic peroxisome proliferators in rodents [Citation24] and the activation of peroxisome proliferator-activated receptor α (PPARα) has been postulated as a plausible mechanism of hepatocarcinogenesis. Further, the findings of the present study also showed that exposure to DEHP in rats enhanced the ACOX1 levels in the liver. In line with these results, Uren-Webster et al. [Citation25], studied the transcription profiles for three peroxisome proliferator-activated receptor (PPARα) regulated genes (acox1, ehhahh, lpl) in zebrafish exposed to DEHP. Acox1 has been the most common PPARα target gene used as an indicator of exposure to peroxisome proliferators, including phthalates, in mammalian studies. In the current study, statistically significant elevated values of serum AST and ALT were observed in both the groups exposed to DEHP. Similar pattern in the liver function tests were also observed in a previous study by Baralić et al. [Citation26], and Huang et al. [Citation8]. This, along with our findings, suggests that DEHP possibly induced hepatocellular injury and intrahepatic biliary obstruction, which can be associated with PPAR agonists including DEHP and DBP [Citation27]. Previously it has been reported that low doses of PPAR agonists suppress the hepatic apoptosis, while the high doses increased the frequency of apoptotic hepatocytes [Citation26]. The findings of the present study noted elevated urea levels in the serum on DEHP exposure. However, DEHP exposure had no significant effect on the levels of serum creatinine. In congruence with these findings, a recent study by Baralić et al. [Citation26], reported increased urea levels in the rats but showed no changes in the levels of the serum creatinine in rats exposed to a mixture of DEHP and BPA for 28 days. DEHP is reported to be nephrotoxic in mice and may exacerbate this pathological alteration towards chronic progressive nephropathy. Previously, it has been reported that chronic progressive nephropathy was observed in male mice treated with 1500 ppm DEHP. After 104 weeks of DEHP exposure, kidney weights were significantly lower than those of the controls [Citation9].
Spirulina has garnered scientific attention over the past few decades for being nutrient dense with several health benefits [Citation28]. There have been controlled studies on its extracts that have been shown to prevent cancer, and this anti-apoptotic activity has been ascribed to its antioxidant potential, which has been widely reported [Citation28,Citation29]. The antioxidant status and free radical scavenging properties of Arthrospira platensis is associated to its constituent natural pigments, such as chlorophyll, beta-carotene, phycoerythrin, and phycocyanin, phycobilins [Citation30]. Furthermore, apart from phycocyanin, spirulina contains carotenoids and xanthophylls, which have the ability to modulate inflammatory responses [Citation28,Citation29]. Quantitative phytochemical analysis of Arthrospira platensis powder in the present study showed considerable content of total phenols and total flavonoids. Additionally, the results of the in vitro antioxidant assays revealed that the spirulina(Arthrospira platensis)powder also had considerable free radical scavenging properties owing to its antioxidant status. These findings were in line with several other previous studies that have reported the phytochemical profile and antioxidant activity of Arthrospira platensis as a natural extract of alga or in the form of commercial formulations [Citation28,Citation31,Citation32]. This explains the ameliorative effect of Arthrospira platensis against the DEHP induced oxidative stress in the present study, which is illustrated by the modulatory effects on the endpoints assessed including serum levels of 8-OHdG, hepatic MDA levels, CAT and ACOX1 activity. This possibly is attributed to the constituent antioxidant compounds in spirulina that also inhibit the activities of catalytic enzymes, such as lipoxygenase and cyclooxygenase and/or enhance the activity of antioxidant enzymes, such as glutathione peroxidase, catalase, and superoxide dismutase [Citation28,Citation30].
In line with the current findings, a study by [Citation33] also reported that treatment with spirulina for 30 and 60 days at (500 × 2) mg dose had a marked protective effect that was evident from a significant reduction in serum MDA, lipid hydroperoxide, and cholesterol levels with enhanced GSH, Vit C level, SOD and GST activities. In consensus with the results of the present study, a previous study on humans from China showed that the use of dissolved polysaccharide extracted from Arthrospira platensis in water significantly enhanced the repair activity of radiation damaged DNA [Citation34].
In line with the results of the present study, Ibrahem and Ibrahim [Citation35], also observed a decreased expression of the p53 in the liver on treatment with Arthrospira platensis in Nile tilapia. Thus, spirulina has the protective ability against carcinogenesis owing to its potent anti-oxidant status [Citation35].
4.1. Conclusions
Taken together, Arthrospira platensis intervention brought about a profound ameliorative effect against oxidative stress, DNA damage, hepatic and renal toxicity induced by DEHP exposure in rats. In addition, a modulatory effect was also observed on the hepatic expression of p53, most frequently mutated genes in human cancers. This plausibly is attributed to the constituent phyto compounds that subsequently contribute to their antioxidant potential. The appreciable antioxidant and phytonutrient contents in Arthrospira platensis can facilitate its wider commercial exploitation in human nutrition and therapeutics.
Acknowledgments
The authors extend their appreciation to the Deputyship for Research & Innovation, Ministry of Education in Saudi Arabia for funding this research work through the project number IFKSURG-434.
Disclosure statement
No potential conflict of interest was reported by the authors.
References
- Giuliani A, Zuccarini M, Cichelli A, et al. Critical review on the presence of phthalates in food and evidence of their biological impact. Int J Environ Res Public Health. 2020;17(16):5655.
- Erkekoglu P, Kocer-Gumusel B. Environmental effects of endocrine-disrupting chemicals: a special focus on phthalates and bisphenol a. In: Marcelo L. Environmental health risk - hazardous factors to living species. Larramendy and Sonia Soloneski, IntechOpen; 2016. Retrieved from DOI:10.5772/62455
- UNEP Report UN Environment Annual Report, 2017. 2017. http://unenvironment.org/annualreport
- Mallick SK, Pramanik M, Maity B, et al. Plastic waste footprint in the context of COVID-19: reduction challenges and policy recommendations towards sustainable development goals. Sci Total Environ. 2021;796:148951.
- Ye H, Ha M, Yang M, et al. Di2-ethylhexyl phthalate disrupts thyroid hormone homeostasis through activating the Ras/Akt/TRHr pathway and inducing hepatic enzymes. Sci Rep. 2017;7:40153.
- Ito Y, Kamijima M, Nakajima T. Di(2-ethylhexyl) phthalate-induced toxicity and peroxisome proliferator-activated receptor alpha: a review. Environ Health Prev Med. 2019;24(47). DOI:10.1186/s12199-019-0802-z
- Ha M, Wei L, Guan X, et al. P53-dependent apoptosis contributes to di-(2-ethylhexyl) phthalate-induced hepatotoxicity. Environ Pollut. 2016;208:416–425.
- Huang Y, Wu C, Ye Y, et al. The increase of ROS caused by the interference of DEHP with JNK/p38/p53 pathway as the reason for hepatotoxicity. Int J Environ Res Public Health. 2019;16(3):356.
- Rowdhwal SSS, Chen J. Toxic effects of di 2-ethylhexyl phthalate: an overview. Bio Med Res Int. 2018;22:1750368. 2018 PMID: 29682520; PMCID: PMC5842715. DOI:10.1155/2018/1750368.
- Abu Zaid AA, Hammad DM, Sharaf EM. Antioxidant and anticancer activity of spirulina platensis water extracts. Int J Pharm. 2015;11:846–152.
- Brito ADF, Silva AS, de Oliveira CVC, et al. Spirulina platensis prevents oxidative stress and inflammation promoted by strength training in rats: dose-response relation study. Sci Rep. 2020;10(1):6382. DOI:10.1038/s41598-020-63272-5
- Sixabela PSS, Chivandi E, Badenhorst M, et al. The effects of dietary supplementation with Spirulina platensis in growing rats. Asian J Animal Vet Adv. 2011;6(6):609–617.
- Romay C, Gonzalez R, Ledon N, et al. C-Phycocyanin: a biliprotein with antioxidant, anti-inflammatory and neuroprotective effects. Current Protein Pept Sci. 2003;4(3):207–216.
- Siddiqui N, Rauf A, Latif A et al. Spectrophotometric determination of the total phenolic content, spectral and fluorescence study of the herbal Unani drug Gul-e-Zoofa (Nepeta bracteata Benth). J. Taibah. Univ. Med. 2017;12(4):360–363. DOI:10.1016/j.jtumed.2016.11.006
- Fattahi S, Zabihi E, Abedian Z et al. Total Phenolic and Flavonoid Contents of Aqueous Extract of Stinging Nettle and in vitro Antiproliferative Effect on Hela and BT-474 Cell Lines. Int J Mol Cell Med. 2014;3(2):102–107.
- Re R, Pellegrini N, Proteggente A et al. Antioxidant activity applying an improved ABTS radical cation decolorization assay. Free Radical Bio. Med.26. 1999. DOI:10.1016/S0891-5849(98)00315-3
- Adebiyi OE, Olayemi FO, Ning-Hua T et al. In vitro antioxidant activity, total phenolic and flavonoid contents of ethanol extract of stem and leaf of Grewia carpinifolia, Beni-Suef Univ. J. Basic Appl Sci. 2017;6 (1):10–14. Doi:10.1016/j.bjbas.2016.12.003
- Benzie IFF, Strain JJ. The Ferric Reducing Ability of Plasma (FRAP) as a Measure of “Antioxidant Power”: The FRAP Assay. Anal Biochem. 1996;239:70–76. DOI:10.1006/abio.1996.0292
- Erkekoglu P, Arnaud J, Rachidi W, et al. The effects of di (2-ethylhexyl) phthalate and/or selenium on trace element levels in different organs of rats. J Trace Elem Med Biol. 2015;29:296–302.
- Asghari A, Fazilati M, Latifi AM et al. A review on antioxidant properties of Spirulina. Appl. Biotechnol. Rep. 2016;3(1):345–351.
- Erkekoglu P, Rachidi W, Yuzugullu OG, et al. Induction of ROS, p53, p21 in DEHP- and MEHP-exposed LNCaP cells-protection by selenium compounds. Food Chem Toxicol. 2011;49:1565–1571.
- Pflaum J, Schlosser S, Müller M. Family and Cellular Stress Responses in Cancer.Frontiers in Oncology.4; 2014. p. 15. DOI:10.3389/fonc.2014.00285
- Ramos H, Soares MIL, Silva J, et al. A selective p53 activator and anticancer agent to improve colorectal cancer therapy. Cell Rep. 2021;35(2):108982.
- Wang Y, Chen HS, Long CY, et al. Possible mechanism of phthalates-induced tumorigenesis.The. Kaohsiung J Med Sci. 2012;28(7):S22–27.
- Uren-Webster TM, Lewis C, Filby AL, et al. Mechanisms of toxicity of di (2-ethylhexyl) phthalate on the reproductive health of male zebrafish. Aquatic Toxicol. 2010;99(3):360–369.
- Baralić K, Buha Djordjevic A, Živančević K, et al. Toxic effects of the mixture of phthalates and bisphenol a—subacute oral toxicity study in Wistar rats. Int J Environ Res Public Health. 2020;17:746.
- Docea AO, Goumenou M, Calina D, et al. Adverse and hormetic effects in rats exposed for 12 months to low dose mixture of 13 chemicals: rLRS part III. Toxicol Lett. 2019;310:70–91.
- Kumar A, Ramamoorthy D, Verma DK, et al. Antioxidant and phytonutrient activities of Spirulina platensis. Energy Nexus. 2022;6:100070.
- Miranda MS, Cintra RG, Barros SBM, et al. Antioxidant activity of the Microalga Spirulina maxima. Braz J Med Biol Res. 1998;31(8):1075–1079. 1998. DOI:10.1590/S0100-879X1998000800007
- Gad AS, Khadrawy YA, El-Nekeety AA, et al. Antioxidant activity and hepatoprotective effects of whey protein and Spirulina in rats. Nutrition. 2011;27(5):582–589.
- Al-Dhabi NA, Arasu MV. Quantification of phytochemicals from commercial spirulina products and their antioxidant activities. Evid Based Complement Alternat Med. 2016:13 2016 Article ID 7631864. DOI:10.1155/2016/7631864
- Ngu EL, Chen-Lin K, Tan CY, et al. Phytochemical profiling and in vitro screening for neuritogenic and antioxidant activities of Spirulina platensis. Indian J Pharm Educ Res. 2021;55(3). DOI:10.5530/ijper.55.3.154
- Ismail M, Hossain MF, Tanu AR, et al. Effect of spirulina intervention on oxidative stress, antioxidant status, and lipid profile in chronic obstructive pulmonary disease patients. Bio Med Res Int. 2015:486120. 2015. DOI:10.1155/2015/486120
- Asghari AM, Fazilati M, Latifi AM, et al. A review on antioxidant properties of Spirulina. J Appl Biotechnol Rep. 2016;3(1):345–351.
- Ibrahem MD, Ibrahim MA. The potential effects of Spirulina platensis (Arthrospira platensis) on tissue protection of Nile tilapia (Oreochromis niloticus) through estimation of P53 level. J Adv Res. 2014;5(1):133–136.