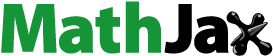
ABSTRACT
Effective passivation of heavy metals in sludge is the key to achieve its land use. Potassium feldspar was modified to obtain G×K900, with a lower crystallinity, a higher specific surface area and cationic exchange capacity. G×K900 was chosen to passivate heavy metals in the sludge, and the best passivation conditions were 30% addition and a 10-day passivation period. The passivated sludge extract had no adverse effects on ryegrass germination and promoted radicle growth. The pot experiment revealed that adding 10% passivated sludge encouraged ryegrass growth while having no appreciable impact on the contents of Cu, Cr, Zn and Pb in the roots and above-ground parts compared to control treatment. The transfer factors of the four metals were all less than 1. Passivated sludge was used as a growing medium for landscaping plants, which provided a theoretical foundation for sludge land use and a resource outlet for potassium feldspar minerals.
1. Introduction
The volume of municipal wastewater treatment has grown yearly, and sludge production has gradually increased, due to the rapid economic development and urbanization [Citation1,Citation2]. Up to 60% of the total operating costs of wastewater treatment plants are related to the management of sludge treatment [Citation3]. Sludge treatment and disposal are now environmental concerns on a global scale [Citation4,Citation5].
Sludge is generally treated and disposed of by simple and low-cost technologies like landfilling, incineration, anaerobic digestion, composting and using as construction materials [Citation3,Citation6,Citation7]. Sludge is rich in organic matter and plant-necessary nutrients like N and P. Its treatment and disposal must consider the recycling of these resource [Citation8], and land use can effectively solve this problem [Citation9]. Sludge land use can improve the organic matter content, water retention, and cation exchange capacity of soil, and increase plant growth [Citation10,Citation11], making it particularly suitable for remediation of contaminated soil [Citation12], remediation of abandoned mines [Citation13], and making organic-mineral fertilizers [Citation14]. Meanwhile land use of sludge can solve the disposal of the large amount of surplus sludge generated by wastewater treatment plants, and is considered an effective way to solve the current dilemma faced by sludge treatment and disposal [Citation15,Citation16]. However, the presence of heavy metals in sludge is a potential harm to the environment because they can continue to build up in the environment [Citation17,Citation18]. The heavy metals in sludge become the main factor limiting its land use.
Immobilization of heavy metals from sewage sludge by passivators can effectively reduce the bioaccessibility of heavy metals [Citation19]. Using clay minerals is one of the economical and cost-effective methods to stabilize heavy metals [Citation20,Citation21]. Clay minerals are environmentally friendly and biocompatible [Citation22] and could reduce the mobility and bioavailability of heavy metals through adsorption, complexation, precipitation, and ion exchange [Citation21,Citation23]. For instance, attapulgite addition could increased the conversion of heavy metals (Cr, Cu, Ni, and Zn) in sludge from acid-soluble, reducible, and oxidizable states to residual state and significantly reduced their ecotoxicity [Citation24]. The transfer of heavy metals from soil to spinach was decreased by the addition of seafoam, which converted a significant amount of exchangeable Cd and Pb to residual states [Citation25].
Natural clay minerals have a limited capacity for heavy metals passivation, but treatments like heat treatment, inorganic modification, and organic modification can significantly improve the capacity. The specific surface area and positive surface charge of montmorillonite were increased through organic modification by Yang et al., which enhanced its ability to passivate Cr6+ [Citation26]. According to Yu et al., the modified bentonite’s passivation effect on Cu, Zn, and Cd was primarily caused by cation exchange, Hg by physical adsorption, and Cr and As by adsorption and electrostatic adsorption, respectively [Citation27]. In order to expose more active centers in zeolites, Kosobucki et al. used ultrasonic treatment to increase the adsorption capacity of heavy metal ions by 3–7% [Citation28]. Therefore, clay minerals’ ability to passivate heavy metals could be effectively improved through effective modification treatment.
Potassium feldspar, widely distributed in the earth’s crust, is abundant in China, with a typical K2O content of 9–14% [Citation29]. It has a monoclinic skeletal structure and is made up of curved double chains of chemically stable silica-oxygen and alumina-oxygen tetrahedra [Citation30]. Due to the ultramicroporous channel effect, certain pore cations, including Na+, K+, and Ca2+, can engage in ionic exchange with heavy metal ions, like Cd2+ and Pb2+ [Citation31]. Potassium feldspar was modified by high-temperature cauterization [Citation32], hydrothermal reaction [Citation33], and microbial modification [Citation34] in order to increase the specific surface area, and release more active sites for passivation reactions with heavy metal ions. Meanwhile, potassium feldspar is abundant in minerals ions like K+, Na+, Ca2+, Mg2+, and Si4+ that can help soil form agglomerates and enhance its colloidal properties, water retention, and nutrient-saving capabilities [Citation35].
The passivation of heavy metals in sludge using modified potassium feldspar was discussed in this paper. The passivated sludge was then used in germination test and pot experiment, and the following research projects were conducted: (1) the modified potassium feldspar (GXK) was characterized to analyze the structural changes, (2) the passivation effect of GXK on heavy metals in sludge was investigated, (3) the effect of passivated sludge on ryegrass germination and growth was investigated, and (4) the heavy metal enrichment and transport during ryegrass growth was analyzed. This study could shift the sludge disposal route from ‘pollution control’ to ‘resource utilization’, and provide an outlet for resource utilization of the abundant clay minerals.
2. Materials and methods
2.1 Experimental materials
The soil, classified as ‘Luvisols’ according to the criteria of World Reference Base for soil resources (WRB), was taken from the mature soil at 0–20 cm from the ground on the campus of Qilu University of Technology in Jinan, air-dried to remove impurities, and bagged in polyethylene sealed bags. The sludge was dried sludge from Shandong Yucheng Fuhang Environmental Protection Co., Ltd., crushed and passed through a 20 mesh sieve for subsequent germination and pot experiments. The other part was passed through an 80 mesh sieve for determination of its physical and chemical properties. Both were bagged separately in polyethylene sealed bags.
The physicochemical properties of the sludge and soil were shown in . The contents of heavy metals in the sludge were lower than the limits for ‘Disposal of sludge from municipal wastewater treatment plant – Quality of sludge used in gardens or parks’ (GB/T23486–2009) ().
Table 1. Physicochemical properties of the sludge and soil.
Table 2. Limits of heavy metals in GB/T23486–2009 (mg/kg).
Both potassium feldspar and limestone were commercially available in 200 mesh, with the former coming from Yantai, Shandong Province, China, and the latter from Shijiazhuang, Hebei Province, China.
The ryegrass (Lolium multiflorum Lamk) was an annual variety.
The nitric acid used were of superior purity, and the rest of the reagents were of analytical purity.
2.2 Preparation of passivation agent
The passivation agent used in this paper was created by roasting potassium feldspar and limestone, according to our preliminary research, potassium feldspar, limestone and water were mixed in the ratio of 2:1:3 by mass, dried in an oven at 105°C for 8 h, grounded and sieved through 200 mesh, then placed in a box-type resistance furnace and calcined at 700, 800, and 900°C for 30 min to make the passivators (labelled as G×K700, G×K800, G×K900). Basing on our preliminary research, G×K900 showed the best adsorption performance of heavy metals, it was chosen as the passivator for heavy metals in the sewage sludge.
2.3 Passivation experiment
GXK with mass fractions of 0%, 5%, 10%, 15%, 20%, 25% and 30% was added into the sludge, noted as D0, D1, D2, D3, D4, D5 and D6, respectively. Deionized water was added to reach 50% (w/w) and mixed evenly. Deionized water was added periodically to keep the water content constant. At 5, 10, 15, and 20 days, various samples were taken, air-dried, and crushed. The pH, electrical conductivity (EC), total heavy metals contents (Cu, Cr, Zn, Pb) and their speciations were determined.
2.4 Seed germination assay
10 g of soil, 10 g of passivated sludge, and 10 g of sludge were each added to 3 conical flasks, respectively. Each flask also received 100 mL of deionized water, and the supernatant was shaken for 24 hours at room temperature. 5 mL of distilled water, soil extract, passivated sludge extract, and sludge extract were added to four petri dishes containing forty full-grained ryegrass seeds, designated as CK, T1, T2, and T3, respectively. Three replicates were set for each treatment.
The seeds were incubated at room temperature for 7 d, regularly supplemented with deionized water to the first weight, and the number of germination and root length were recorded. Relative seed germination (RSG), relative root elongation (RRG) and germination index (GI) of ryegrass were calculated according to equations (1), (2) and (3) [Citation36].
2.5 Ryegrass pot experiment
Three replicates were set up for each of the five treatments (), each with 1.5 kg of substrate. 0.4 g of ryegrass seeds were evenly distributed over the substrate’s surface, covered with 0.5 cm of soil, regularly hydrated, incubated at room temperature (20–30°C), and harvested after 30 days.
Table 3. Experimental design of ryegrass pot experiment.
10 to 15 ryegrass plants were randomly selected from each pot and their plant height, tiller number and chlorophyll content were recorded. For each treatment, the ryegrass was harvested at 1 mm from the ground, and the fresh weight of ryegrass, heavy metal content in the above-ground portion, roots, and soil matrix were measured.
Both the enrichment factor (BCF) and the transport factor (TF) were frequently used to assess the accessibility of heavy metals. Calculations were performed according to equations (4) and (5), respectively.
2.6 Analysis methods
The total content of heavy metals was determined by digested by an acid mixture (HNO3-HClO4-HF, 5:5:3, v/v) on a hot plate, filtered through a 0.45 μm filter membrane, and then the samples were analyzed using an OPTIMA 2000 inductively coupled plasma emission spectrometer [Citation37]. The determination of heavy metals in ryegrass was first carried out by dry ashing according to ‘Soil Agrochemical and Environmental Monitoring’ [Citation38] before determination.
A modified four-stage Community Bureau of Reference (BCR) sequential extraction method was used to analyze four speciations of heavy metals [Citation39], which classified heavy metals into four forms: exchangeable fraction (Ex), reducible fraction (Red), oxidizable fraction (Ox) and residual fraction (Res). Their mobility and bioavailability decreased in order, with Ex being prone to migration and considered the most bioavailable fraction, Red and Ox being potentially bioavailable, and Res being the non-plant-available fraction [Citation40]. The effect of passivation agents could be determined by analyzing the speciation changes during passivation treatments using BCR method. The stability coefficient was used to assess their stability and calculated using Equation (6). The larger the stability coefficient, the stronger the migration and bioavailability.
pH was detected by a pHS-3C type pH meter. The conductivity was detected by a LDO101 type conductivity meter. Organic matter content was determined by H2SO4-K2Cr2O7 wet oxidation method. CEC was determined by ammonium acetate method. Total nitrogen, total phosphorus and total potassium were analyzed by the method in ‘Soil Agrochemical Analysis and Environmental Monitoring’ [Citation38].
The plant height of ryegrass was measured with a measuring tape. The chlorophyll content of ryegrass was measured with a portable chlorophyll meter (TYS-B type).
All glassware was thoroughly cleaned with deionized water after being soaked in a 10% HNO3 solution for at least an entire night. The abbreviations and full names in the articles are listed in .
Table 4. Acronym list.
3. Results and discussion
3.1 Materials characterization
displayed the XRD images of original potassium feldspar and the modified potassium feldspar. The primary characteristic peaks were clearly visible in the original potassium feldspar, a natural mineral with a complex crystalline structure and more heterogeneous peaks. Its primary phases were SiO2, KAlSi3O8, and NaAlSi3O8. After modification, new phases CaMg(CO3)2 (dolomite) appeared. CaMg(CO3)2 was produced from Ca2+ and Mg2+ contained in potassium feldspar with CO32- in limestone during high temperature calcination. Its characteristic diffraction peaks (2θ = 30.9°, 41.1°) gradually weakened as the roasting temperature increased, probably due to the decomposition of CaMg(CO3)2 with increasing temperature [Citation41].
After the modification treatment, the intensity of KAlSi3O8‘s primary characteristic diffraction peak (2θ = 27.3°) decreased, indicating an increase in lattice defects. This suggested that potassium feldspar lost some of its crystalline integrity, and gradually transitions to an amorphous state [Citation42], which made it easier for K, Si, and Al to dissolve from potassium feldspar. Additionally, the characteristic diffraction peaks were slightly shifted to higher angles, probably due to the replacement of the K+ and Na+ in potassium feldspar by Ca2+ with smaller ionic radii during the modification process, resulting in changes in the lattice constants. G×K900 was chosen as the sludge passivator in the following experiments.
The infrared spectra were shown in . Since potassium feldspar had a silica-aluminate shelf structure, the absorption spectra at 775 cm−1 and 713 cm−1 were caused by the symmetric stretching vibration of Si-O-Si, while 1011 cm−1 corresponded to the anti-symmetric stretching vibration of Si-O-Si [Citation43,Citation44]. After the modification treatment, G×K900 at 775 cm−1, 713 cm−1, and 1011 cm−1 corresponding to higher transmittance suggested that the Si-O-Si chemical bond had been broken by the modification treatment. Additionally, the new absorption peak at 2874 cm−1 was a first order multiple of 1416 cm−1 of CaCO3, and the characteristic peak of the stretching vibration of the C=O bond was at 1795 cm−1. The asymmetric extension vibration of the C-O bond, which was the V3 distinctive absorption peak of calcite type CaCO3 crystals, exhibited an absorption peak at 1416 cm−1. After calcination at 900°C, some CaCO3 was still present in G×K900 as calcite crystals.
The specific surface area of G×K900 was 33.256 m2·g−1, which was 13.5 times higher than that of potassium feldspar. The increase in specific surface area implied that the thermal modification produced more adsorption active sites, which was beneficial for the adsorption of heavy metals.
displayed the SEM images of potassium feldspar and G×K900. Potassium feldspar was an irregular block structure with large particles, and no obvious pore structure was found on its surface. The surface of the modified GXK 900 became rougher and the pore structure increased, which increased its specific surface area, so that G×K900 could provide more adsorption sites and was more favorable to its adsorption of heavy metals.
The adsorption ability of the mineral passivator was closely correlated with its CEC. A higher CEC indicated a greater capacity for the mineral material to passivate heavy metals by the electrostatic adsorption. Additionally, the substances with high CEC was conducive to the formation of soil agglomerate structure. Better moisture-holding and buffering capabilities were also associated with higher CEC values [Citation45]. It was discovered that the CEC of G×K900 was 525.43 mmol·kg−1, which was 5.8 times greater than that of potassium feldspar. The use of G×K900 in the soil would facilitate the improvement of soil properties.
3.2 EC and pH of passivated sludge
As the EC of G×K900 (0.48 mS·cm−1) was smaller than that of unpassivated sludge (3.08 mS·cm−1), the EC of the passivated sludge gradually decreases with the increase of G×K900 addition. When the G×K900 addition was higher than 25%, the EC of the passivated sludge obtained by various passivation time treatments was less than 2 mS·cm−1 (), which satisfied the requirements for ‘Disposal of sludge from municipal wastewater treatment plant – Quality of sludge used in gardens or parks’ (GB/T23486–2009).
For each treatment, the pH changed between 0.1 and 0.2 unit during the 20-day passivation process. Since G×K900 was alkaline (pH = 10.74), the addition of G×K900 gradually raised the pH of the passivated sludge from (6.4–6.6) to (7.4–7.5). The pH of the passivated sludge was all between 6.5 and 7.5, which was the ideal range for agricultural soils and complied with GB/T23486–2009. The adsorption-desorption and precipitation-dissolution of heavy metals were strongly influenced by pH. pH increase in the immobilized sludge generated more negatively charged sorption sites on the organic matter surfaces, which consequently led to a decrease on metal mobility [Citation46]. This allowed D5 and D6 to achieve better heavy metal passivation.
3.3 Speciation changes of heavy metals in passivated sludge
The contents of Cu, Zn, Cr, and Pb in sludge were 108.06 mg·kg−1, 801.02 mg·kg−1, 728.32 mg·kg−1, and 72.34 mg·kg−1, respectively, as shown in . The contents of Cu, Zn and Pb met the requirements of GB/T23486–2009 for sludge used in landscaping (regardless of (acidic condition) pH < 6.5 and (alkaline condition) pH ≥ 6.5). The content of Cr only met the requirements in alkaline conditions (pH ≥ 6.5). The high content of Cr and Zn might be due to Zn, Cr and their compounds used in pigments, ceramics and agricultural fertilizers [Citation47]. Additionally, the high content of Zn was also likely ascribing to the widely used of galvanized pipes in wastewater pipelines in China [Citation48].
The speciation distribution of heavy metals affected their mobility, bioavailability, and ecotoxicity more than the total content because different speciations have different mobility and plant uptake [Citation49,Citation50]. displayed the outcomes of the BCR analysis. Pb was mainly in the residual fraction accounting for 87.23%. Samia et al. [Citation51] and Leng et al. [Citation52] had extensively confirmed the high proportion of residual Pb in the sewage sludge. Despite the high concentration of Cr in the sewage sludge, the oxidizable and residual fractions, which accounted for 69.4% and 20.3%, respectively, showed low environmental toxicity. This outcome was in accordance with prior research [Citation53]. Exchangeable Cu content in the sludge was only 2.65%, but the sum of its reducible and oxidizable fractions were 56.5%, suggesting the potential bioavailability of Cu. The percentage of exchangeable and reducible fractions Zn was about 60%, presenting the greatest bioavailability in all the four metals. Such a finding was clearly in conflict with the results of Samia et al., with only 29.9% of the exchangeable and reducible fractions [Citation51], which might be due to the different sources of the sludge.
The treatment with G×K900 passivation altered the speciations of heavy metals. According to , as G×K900 addition increased, the proportion of exchangeable and reducible Cu gradually decreased while gradually increasing the proportion of residual Cu. The proportion of residual and oxidizable Cu increased and the proportion of exchangeable and reducible Cu decreased as the passivation time was extended from 5 days to 10 days. However, the fraction of residual and oxidizable Cu did not significantly increase as the passivation time was further prolonged. The addition of 30% G×K900 and passivation treatment for 10d resulted in a minimum stability coefficient (1.03). The reduced exchangeable and reducible Cu was converted into more stable residual Cu.
The exchangeable and reducible Cr decreased, the oxidizable Cr changed barely at all, and the residual Cr increased with the increasing G×K900 addition and the lengthening of the passivation time. The stability coefficient dropped from 3.91 to a lower value (2.85–2.64) when the G×K900 addition amount was 30% and the passivation time exceeded 10 days ().
The exchangeable Zn gradually decreased and the residual Zn gradually increased with the increasing G×K900 addition. The exchangeable Zn decreased to the lowest level (12.7%) and the residual Zn reached its highest level (39.0%) when G×K900 was added at 30% and passivated for 10 days. The stability coefficient decreased from 2.94 to 1.56 (). If the passivation time continued to be extended, the exchangeable Zn increased and the residual Zn decreased.
For D1-D6 treatments with various passivation time, the four speciations fraction of Pb did not differ significantly. The residual Pb had the largest proportion (90.0–92.3%) ().
Considering the passivation effect of G×K900 on the four metals, 30% addition and passivation treatment for 10d could achieve the better passivation effect. The passivation effect did not significantly improve as the passivation time was further prolonged; instead, the stability coefficient of Zn and Cu rose. The passivated sludge used in the following germination test and pot experiment came from D6 by a 10-day passivation treatment.
The passivation effect of G×K900 on heavy metals could be analyzed from the following five aspects: First, G×K900 stabilized heavy metals by physical adsorption, chemisorption, or physical-chemical adsorption. Second, due to the mobility of cations in G×K900, heavy metals underwent ion-exchange reactions with cations of similar ionic radii (K+, Na+, Ca2+, etc.) in G×K900 [Citation54]. Third, the addition of G×K900 elevated the pH, which helped to generate hydroxide precipitation and also affected the complexation and ion exchange of metal cations in sludge [Citation55]. Fourth, some of the Si4+ and Al3+ in G×K900 were substituted for other cations (K+, Na+, Ca2+, etc.), increasing the net negative charge of sludge colloids and improving the ability to adsorb heavy metals; Fifth, G×K900 contained CaCO3, which was a crucial cement for microagglomerates, increasing sludge surface area and negative charge [Citation56].
3.4 Impact of sewage sludge on early stage ryegrass growth
The combination of biological testing and chemical speciation could more accurately determine the ecotoxicity of sludge, so as to determine whether it could be used for land use [Citation36,Citation57]. When seeds were exposed to a pollutant-stressed environment, seed germination and rooting, which were very active stages of physiological and biochemical changes in plant embryonic development, were inhibited. RSG and RRG were used to assess the influence of the planting medium on seed germination and root growth, respectively. The toxic level that inhibited seed germination was higher than that inhibited radicle elongation, thus RRG was a more sensitive indicator to toxicity than RSG [Citation36,Citation58]。
Ryegrass germination was totally inhibited by unstabilized sewage sludge extracts (RSG = 68.3%). Although the RSG was 100% in T2 treatment, the passivated sludge extract had a negative effect on seed germination (). In the initial 3 days, the germination rate of T2 treatment was lower than that of T1 treatment, which might be due to the fact that the EC (1.8 mS·cm−1) of the passivated sludge was still much higher than that of soil (0.13 mS·cm−1). High salinity could destroy the osmotic pressure balance in soil, which made it difficult for plants to absorb water. At the same time, it could interfere with the ion dynamic balance in plants and cause nutrient deficiency [Citation59]. Thus, high salinity would inhibit the seed germination or delay the initial germination time. However, the toxic effects of sludge could decline with time and seeds could adjust to the more adverse conditions. By the fourth day it was similar to that of T1 treatment, which was consistent with the results of Walter et al [Citation60].
It was worth noting that stabilized sludge had a stimulating effect on the radicle growth. The RRG for T2 treatment was higher than that for T1 treatment. The RRG for T3 treatment was only 45.2%, indicating that the unstabilized sludge seriously inhibited the radicle growth of ryegrass.
GI was used to assess the impact of sludge both on seed germination and radicle growth. According to previous literature, if GI ≤ 50%, the substance had a strong phytotoxic effect, in the range of 50–80%, showed a moderate or low phytotoxic effect, and in the range of GI ≥ 80%, no negative effect on the germination and plant growth was observed [Citation61]. The stabilized sewage sludge showed no phytotoxic properties for germination and radicle growth (GI = 98%), while the GI for the unstabilized sewage sludge decreased to 30.3%, suggesting that unpassivated sewage sludge had a strong phytotoxic effect.
Inhibition of radicle growth might be due to the presence of heavy metals, large amounts of NH4+ or salinity [Citation58,Citation62]. The inappropriate NPK ratio in sludge might also adversely affected the radicle growth and plant growth. In order to assess the impact of sludge on the plant growth, pot experiment were carried out.
3.5 Effect of sewage sludge on ryegrass growth
displayed the effects of different substrates on the ryegrass growth. The use of sewage sludge significantly influenced ryegrass growth. Sludge application to the soil would introduce salts, heavy metals, and other harmful substances that would inhibit plant growth, while also supplying plants with organic matter, NP, and other nutrients that would promote plant growth. In comparison to the CP treatment, the addition of 10% passivated sludge increased plant height, tiller number, fresh biomass, and chlorophyll content by 22.0%, 12.31%, 111.2%, and 17.7%, respectively. This was because that favor factor for plant growth had a greater impact than harmful substances. These indicators, however, showed a decreasing trend when 20% of passivated sludge was added, suggesting that the harmful substances exceeded ryegrass’s tolerance limit. Among them, high salinity affected the osmotic pressure of the soil solution, and heavy metals prevented mylase and protease from working and affected the production of the nutrients and energy needed for plant metabolism [Citation63]. Therefore, there was an optimum addition (10%) for the application of passivated sludge and exceeding it led to inhibition of plant growth, which was was consistent with the results of Nahar et al. [Citation64].
Figure 8. Effect of sludge addition on plant height (a), number of tillers (b), fresh weight (c), and chlorophyll content (d).

The application of unpassivated sludge had a more pronounced inhibitory effect on ryegrass. The tiller number increased slightly with the application of 10% unpassivated sludge, but the plant height, fresh weight and chlorophyll content were not as high as those of the control treatment, and the four indicators decreased further with increasing addition to 20%. In contrast, passivation treatment stabilized heavy metals and reduced EC, which could greatly reduce the inhibitory effect of harmful substances on plant growth.
The application of passivated sludge as a soil amendment has therefore become a crucial step in addressing the issues of sludge disposal and soil remediation, such as in abandoned mines, as the passivated sludge could provide organic matter, NP, and other trace elements for plant growth while improving soil properties [Citation13,Citation65]. However, sludge was thought to be a reservoir of antibiotic and metal resistance genes [Citation66,Citation67]. Its application in soil might encourage the spread of antibiotic, metal resistant strains, which became a limiting factor in restricting the land use of sludge. As a result, many scientists thought that additional research should be done on the long-term effects of repeated sludge addition on soil physicochemical properties as well as the possibility of resistance gene diffusion [Citation68,Citation69].
3.6 Enrichment and transport of heavy metals in ryegrass
Heavy metal accumulation and translocation in plants was an important environmental issue, especially in the process of sludge land use. The heavy metals contents in the plant biomass were presented in . The contents of the four metals in both roots and above-ground components in D10 and D20 treatments were lower than that in W10 and W20 treatments, respectively, indicating that the passivation treatment effectively reduced their mobility. When 10% passivated sludge was added, the content of the four metals in the roots and above-ground parts of ryegrass did not differ significantly from the CP treatment.
Figure 9. Heavy metals content ((above-ground part (a) and root (b)), BCF ((above-ground part (c) and root (d)) and TF (d) in ryegrass.

There were differences in the uptake properties for heavy metals in different parts of ryegrass. Plant roots had huge surface area, which could absorb, precipitate and enrich heavy metals in soil. In the five treatments, the contents in the roots, which directly contacted with the substrate, were all higher than those in the above-ground part. Thus, the BCFs of roots were greater than those of above-ground parts.
TF was used to evaluate the ability of plants to transported heavy metals from roots to above-ground parts such as leave, fruit and et al. The larger the TF values meaning the stronger transfaction ability for heavy metals. The TF values of the four metals were all less than 1, indicating that the transport capacity of ryegrass to them was low. Among them, Cu and Zn, as the elements required for plant growth, had higher TF than Pb and Cr.
4. Conclusions
Potassium feldspar was mixed with CaCO3 at a mass ratio of 2:1 and cauterized at 900°C for 30 min to make G×K900. The SEM and XRD analysis showed that the modified treatment broke the Si-O-Si chemical bond and the crystallinity of G×K900 decreased. Its specific surface area and CEC increased. G×K900 was used as a passivator for immobilizing the heavy metals in sludge. The best passivation effect was obtained by adding 30% G×K900 to the sludge and passivating it for 10 days, and the stability of Cu, Zn, Cr and Pb in the sludge was significantly increased.
The passivated sludge extract showed no negative effect on ryegrass germination and had a stimulating effect on the radicle growth, while the unpassivated sludge extract had a significant inhibitory effect on germination rate and radicle growth. The pot experiment showed that the addition of 10% passivated sludge to the soil could promote the growth of ryegrass, ryegrass plant height, tiller number, fresh biomass and chlorophyll content by 22.0%, 12.3%, 111.2% and 17.7%, respectively. The four metal contents of ryegrass roots and above-ground parts were not significantly different from the control treatment. The addition of 20% passivated sludge to the soil did not promote the growth of ryegrass as well as 10%, and the application of unpassivated sludge significantly inhibited ryegrass growth. The four heavy metals had a poor transport capacity in ryegrass. The study’s findings could serve as a theoretical foundation for sludge on land use and provide an outlet for the utilization of abundant potassium feldspar resources.
Land use of sludge may have impacts on soil microbes. Future work will focus on studying the risk of heavy metal leaching from passivated sludge after application to soil and the effects on soil microbial populations.
Data availability
The datasets supporting the relevant research findings in this article are available from the email of the corresponding author ([email protected]) through reasonable request.
Acknowledgments
This work was supported by the Natural Science Foundation of Shandong Province, China (ZR2020MB141) and the National-level and School-level Student Innovation and Entrepreneurship Training Program (202110431011).
Disclosure statement
The authors report there are no competing interests to declare.References
Additional information
Funding
- Raheem A, Sikarwar V, He J, et al. Opportunities and challenges in sustainable treatment and resource reuse of sewage sludge: a review. Chem Eng J. 2018;337:616–13.
- Guo Y, Guo Y, Gong H, et al. Variations of heavy metals, nutrients, POPs and particle size distribution during “sludge anaerobic digestion-solar drying-land utilization process” Case study in China. Sci Total Environ. 2021;801:149609.
- Chen H, Yan SH, Ye ZL, et al. Utilization of urban sewage sludge: Chinese perspectives. Environ Sci Pollut Res. 2012;19(5):1454–1463. DOI:10.1007/s11356-012-0760-0
- Leng LJ, Yuan XZ, Huang HJ, et al. The migration and transformation behavior of heavy metals during the liquefaction process of sewage sludge. Biores Technol. 2014;167:144–150.
- Iqba A, Zan F, Ahmar Siddiqui MA, et al. Integrated treatment of food waste with wastewater and sewage sludge Energy and carbon footprint analysis with economic implications. Sci Total Environ. 2022;825:154052.
- Chen R, Yuan S, Chen S, et al. Life-cycle assessment of two sewage sludge-to-energy systems based on different sewage sludge characteristics: energy balance and greenhouse gas-emission footprint analysis. J Environ Sci. 2022;111(1):380–391. DOI:10.1016/j.jes.2021.04.012
- Liew C, Yunus N, Chidi B, et al. A review on recent disposal of hazardous sewage sludge via anaerobic digestion and novel composting. J Hazard Mater. 2022;423(Pt A):126995. DOI:10.1016/j.jhazmat.2021.126995
- Csl A, Wk B, Jwl A, et al. Stabilization of heavy metals loaded sewage sludge: reviewing conventional to state-of-the-art thermal treatments in achieving energy sustainability. Chemosphere. 2021;277:130310.
- Wei LL, Zhu F, Li Q, et al. Development, current state and future trends of sludge management in China: based on exploratory data and CO2-equivaient emissions analysis. Environ Int. 2020;144:106093.
- Sharma B, Sarkar A, Singh P, et al. Agricultural utilization of biosolids: a review on potential effects on soil and plant grown. Waste Manage. 2017;64:117–132.
- Bai Y, Zang C, Gu M, et al. Sewage sludge as an initial fertility driver for rapid improvement of mudflat salt-soils. Sci Total Environ. 2017;578:47–55.
- Rajendran S, Priya TAK, Khoo KS, et al. A critical review on various remediation approaches for heavy metal contaminants removal from contaminated soils. Chemosphere. 2022;287:132369.
- Penido ES, Martins GC, Mendes TM, et al. Combining biochar and sewage sludge for immobilization of heavy metals in mining soils. Ecotoxicol Environ Saf. 2019;172:326–333.
- Kominko H, Gorazda K, Wzorek Z. Effect of sewage sludge based fertilizers on biomass growth and heavy metal accumulation in plants. J Environ Manage. 2022;305:114417.
- Alvarenga P, Mourinha C, Farto M, et al. Sewage sludge, compost and other representative organic wastes as agricultural soil amendments: benefits versus limiting factors. Waste Manag. 2015;40:44–52.
- Wahaab RA, Mahmoud M, Lier JV. Toward achieving sustainable management of municipal wastewater sludge in Egypt: the current status and future prospective. Renew Sust Energ Rev. 2020;127:127.
- Jaskulak M, Rostami S, Zorena K, et al. Transcriptome sequencing of Brassica napus highlights the complex issues with soil supplementation with sewage sludge. Chemosphere. 2022;298:134321.
- Hoang SA, Bolan N, Madhubashani A, et al. Treatment processes to eliminate potential environmental hazards and restore agronomic value of sewage sludge: a review. Environ Pollut. 2022;293:118564.
- Antonkiewicz J, Kowalewska A, Mikolajczak S, et al. Phytoextraction of heavy metals after application of bottom ash and municipal sewage sludge considering the risk of environmental pollution. J Environ Manage. 2022;306:114517.
- Chen G, Shah KJ, Shi L, et al. Red soil amelioration and heavy metal immobilization by a multi-element mineral amendment: performance and mechanisms. Environ Pollut. 2019;254(Pt A):112964. DOI:10.1016/j.envpol.2019.112964
- Otunola BO, Ololade OO. A review on the application of clay minerals as heavy metal adsorbents for remediation purposes. Environ Technol Innov. 2020;18:100692.
- Zarghami Z, Akbari A, Latifi M, et al. Design of a new integrated chitosan-PAMAM dendrimer biosorbent for heavy metals removing and study of its adsorption kinetics and thermodynamics. Biores Technol. 2016;205:230–238.
- Xu Y, Liang XF, Xu YM, et al. Remediation of heavy metal-polluted agricultural soils using clay minerals: a review. PEDOSPHERE. 2017;27(2):193–204. DOI:10.1016/S1002-0160(17)60310-2
- Dai L, Ren J, Ling T, et al. Chemical speciation and phytoavailability of Cr, Ni, Zn and Cu in loess amended with attapulgite-stabilized sewage sludge. Environ Pollut Bioavailabil. 2019;31(1):112–119. DOI:10.1080/26395940.2019.1588076
- Sun Y, Zhao D, Xu Y, et al. Effects of sepiolite on stabilization remediation of heavy metal-contaminated soil and its ecological evaluation. Front Environ Sci Engine. 2016;10(1):85–92. DOI:10.1007/s11783-014-0689-2
- Yang J, Yu K, Liu C. Chromium immobilization in soil using quaternary ammonium cations modified montmorillonite: characterization and mechanism. J Hazard Mater. 2016;321:73–80.
- Yu K, Xu J, Jiang XH, et al. Stabilization of heavy metals in soil using two organo-bentonites. Chemosphere. 2017;184:884–891.
- Kosobucki P, Kruk M, Buszewski B. Immobilization of selected heavy metals in sewage sludge by natural zeolites. Biores Technol. 2008;99(13):5972–5976.
- Salimkhani H, Joodi T, Bordbar-Khiabani A, et al. Surface and structure characteristics of commercial K-Feldspar powders: effects of temperature and leaching media. Chin J Chem Eng. 2020;28(1):307–317. DOI:10.1016/j.cjche.2018.11.016
- Zhang Y, Hu YH, Sun N, et al. Systematic review of feldspar beneficiation and its comprehensive application. Miner Eng. 2018;128:141–152.
- Lu HA. Mineralogical effects of ultra-microchannel structures in natural self-purification of inorganic minerals. Acta Petrologica Et Mineralogica. 2005;24(6):503–510.
- Torres-Luna JA, Carriazo JG. Porous aluminosilicic solids obtained by thermal-acid modification of a commercial kaolinite-type natural clay. Solid State Sci. 2019;88(2):29–35.
- Zheng T, Zhou X, Guo J, et al. Activated mineral adsorbent for the efficient removal of Pb(II) and Cd(II) from aqueous solution: adsorption performance and mechanism studies. Water Sci Technol. 2020;82(9):1896–1911. DOI:10.2166/wst.2020.453
- Han M, Zhu YX, Chen WG, et al. Advances on potassium-solubilizing bacteria and their microscopic potassium solubilizing mechanisms. Acta Pedologica Sinica. 2022;59(2):334–348.
- Ribeiro PG, Aragão OODS, Martins C, et al. Hydrothermally-altered feldspar reduces metal toxicity and promotes plant growth in highly metal-contaminated soils. Chemosphere. 2022;286:131768.
- Fuentes A, Lloréns M, Sáez J, et al. Phytotoxicity and heavy metals speciation of stabilised sewage sludges. J Hazard Mater. 2004;108(3):161–169. DOI:10.1016/j.jhazmat.2004.02.014
- Liu TT, Liu ZG, Zheng QF, et al. Effect of hydrothermal carbonization on migration and environmental risk of heavy metals in sewage sludge during pyrolysis. Biores Technol. 2017;247:282–290.
- Yang JH, Wang CL, Dai H. Soil agrochemical analysis and environmental monitoring[M]. Beijing: China Land Press; 2008.
- Rauret G, López-Sánchez JF, Sahuquillo A, et al. Improvement of the BCR three step sequential extraction procedure prior to the certification of new sediment and soil reference materials. J Environ Monitor. 1999;1(1):57–61. DOI:10.1039/a807854h
- Wang S, Wu W, Liu F, et al. Accumulation of heavy metals in soil-crop systems: a review for wheat and corn. Environ Sci Pollut Res. 2017;24(18):15209–15225. DOI:10.1007/s11356-017-8909-5
- Li Q, Z JF, Xu B, et al. Consolidation mechanism of gold concentrates containing sulfur and carbon during oxygen-enriched air roasting. Int J Miner Metall Mater. 2017;24(4):36–42. DOI:10.1007/s12613-017-1418-1
- Zhang Y, Asselin E, Li Z. Laboratory and pilot scale studies of potassium extraction from K-feldspar decomposition with CaCl2 and CaCO3. J Chem Eng Jpn. 2016;49(2):111–119.
- Lusvardi G, Malavasi G, Menabue L, et al. A computational tool for the prediction of crystalline phases obtained from controlled crystallization of glasses. J Phys Chem B. 2005;109(46):21586–21592. DOI:10.1021/jp0546857
- Pisciella P, Pelino M. Thermal expansion investigation of iron rich glass-ceramic. J Eur Ceram Soc. 2008;28(16):3021–3026.
- Szatanik-Kloc A, Szerement J, Adamczuk A, et al. Effect of low zeolite doses on plants and soil physicochemical properties. Materials. 2021;14(10):2617. DOI:10.3390/ma14102617
- Cambier P, Michaud A, Paradelo R, et al. Trace metal availability in soil horizons amended with various urban waste composts during 17 years – monitoring and modelling. Sci Total Environ. 2019;651(Pt 2):2961–2974. DOI:10.1016/j.scitotenv.2018.10.013
- Man XB, Jiang HL, Zhang X. Spatial distribution and source analysis of heavy metals in the marine sediments of Hong Kong. Environ Monitor Assess Int J. 2015;187(8):504.
- Jin JW, Wang MY, Cao YC, et al. Cumulative effects of bamboo sawdust addition on pyrolysis of sewage sludge: biochar properties and environmental risk from metals. Biores Technol. 2017;228:218–226.
- Hahn J, Mann B, B U, et al. Horizon-specific effects of heavy metal mobility on nitrogen binding forms in forest soils near a historic smelter (Germany). Geoderma. 2019;355(355):113895. DOI:10.1016/j.geoderma.2019.113895
- Li Q, Wang Y, Li Y, et al. Speciation of heavy metals in soils and their immobilization at micro-scale interfaces among diverse soil components. Sci Total Environ. 2022;825:153862.
- Khadhar S, Sdiri A, Chekirben A, et al. Integration of sequential extraction, chemical analysis and statistical tools for the availability risk assessment of heavy metals in sludge amended soils. Environ Pollut. 2020;263:114543.
- Leng LJ, Leng SQ, Chen J, et al. The migration and transformation behavior of heavy metals during co-liquefaction of municipal sewage sludge and lignocellulosic biomass. Biores Technol. 2018;259:156–163.
- Zhao J, Qiu C, Fan X, et al. Chemical speciation and risk assessment of heavy metals in biochars derived from sewage sludge and anaerobically digested sludge. Water Sci Technol. 2021;84(5):1079–1089. DOI:10.2166/wst.2021.305
- Jia HZ, Nulaji G, Gao HW, et al. Formation and stabilization of environmentally persistent free radicals induced by the interaction of anthracene with Fe(III)-modified clays. Environ Sci Technol. 2016;50(12):6310–6319. DOI:10.1021/acs.est.6b00527
- Lee SH, Lee JS, Jeong Choi Y, et al. In situ stabilization of cadmium-, lead-, and zinc-contaminated soil using various amendments. Chemosphere. 2009;77(8):1069–1075. DOI:10.1016/j.chemosphere.2009.08.056
- Kužel S, Kolář L, Gergel J, et al. Influence of the degree of Soil organic matter lability on the calcium carbonate equilibrium of soil water. Soil Water Res. 2010;5(2):58–68. DOI:10.17221/18/2009-SWR
- Roig N, Sierra J, Nadal M, et al. Relationship between pollutant content and ecotoxicity of sewage sludges from Spanish wastewater treatment plants. Sci Total Environ. 2012;425:99–109.
- Luo Y, Liang J, Zeng GM, et al. Seed germination test for toxicity evaluation of compost: its roles, problems and prospects. Waste Manage. 2017;71(109):109–114. DOI:10.1016/j.wasman.2017.09.023
- Xiao Y, Hu Q, Li H, et al. Effect of 2,4,6-trimercaptotriazine, trisodium salt, nonahydrate on heavy metals bioavailability in soils and accumulation in tobacco. Plant Soil Environ. 2018;65(1):9–14. DOI:10.17221/512/2018-PSE
- Walter I, Martinez F, Cala V. Heavy metal speciation and phytotoxic effects of three representative sewage sludges for agricultural uses. Environ Pollut. 2006;139(3):507–514.
- Kebrom TH, Woldesenbet S, Bayabil HK, et al. Evaluation of phytotoxicity of three organic amendments to collard greens using the seed germination bioassay. Environ Sci Pollut Res. 2019;26(6):5454–5462. DOI:10.1007/s11356-018-3928-4
- Miaomiao H, Wenhong L, Xinqiang L, et al. Effect of composting process on phytotoxicity and speciation of copper, zinc and lead in sewage sludge and swine manure. Waste Manage. 2009;29(2):590–597. DOI:10.1016/j.wasman.2008.07.005
- Zhang WJ, Li Y, Chen ZL, et al. Effects of heavy metals stress on seed germination and seedling physiological characteristics of Festuca arundinacea L and Lolium perenne L. J Anhui Agric Sci. 2017;45(06):1–4.
- Nahar N, Hossen MS. Influence of sewage sludge application on soil properties, carrot growth and heavy metal uptake. Commun Soil Sci Plant Anal. 2021;52(1):1–10.
- Chen J, Aihemaiti A, Xia Y, et al. The effect of soil amendment derived from P-enhanced sludge pyrochar on ryegrass growth and soil microbial diversity. Sci Total Environ. 2022;813:152526.
- Östman M, Fick J, Tysklind M. Detailed mass flows and removal efficiencies for biocides and antibiotics in Swedish sewage treatment plants. Sci Total Environ. 2018;640–641:327–336.
- Zhang Y, Gu AZ, Cen TY, et al. Sub-inhibitory concentrations of heavy metals facilitate the horizontal transfer of plasmid-mediated antibiotic resistance genes in water environment. Environ Pollut. 2018;237:74–82.
- Urra J, Alkorta I, Mijangos I, et al. Application of sewage sludge to agricultural soil increases the abundance of antibiotic resistance genes without altering the composition of prokaryotic communities. Sci Total Environ. 2019;647:1410–1420.
- Li ZH, Yuan L, Shao W, et al. Evaluating the interaction of soil microorganisms and gut of soil fauna on the fate and spread of antibiotic resistance genes in digested sludge-amended soil ecosystem. J Hazard Mater. 2021;420:126672.