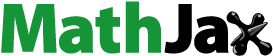
ABSTRACT
The concentration of heavy metals (HMs) in soil-plant systems was an important indicator of soil heavy metal pollution, and the migration and transportation abilities of HMs from soil to plants were of great significance for evaluating food safety and reducing health risks. This study was conducted to investigate the contamination and translocation of HMs in the soil-Paeonia lactiflora system in Bozhou, Anhui Province, China. The soil pollution was assessed by calculating the Geoaccumulation index (Igeo), Potential ecological risk index (RI), and Pollution load index (PLI). The transfer ability of HMs was evaluated by using the bioconcentration factor (BCF) and translocation factor (TF). The results showed that Fe, Mn , Cr , and As were the priority pollutants in soil. Paeonia lactiflora presented high potential to translocate Zn, Cu, and Fe from soil to roots, and had strong ability to translocate HMs except for Cd from roots to stems and leaves
1. Introduction
Paeonia lactiflora Pall. (Chinese herbaceous peony) is a famous perennial herb that is mainly cultivated in East Asia, such as China, Korea, and Japan [Citation1,Citation2]. Its root, also known as Paeoniae Radix Alba, is a traditional Chinese medicinal material, mainly has the effects of calming liver and relieving pain, nourishing blood and regulating menstrual cycle, collecting Yin and antiperspirant (Chinese Pharmacopoeia, 2020). In China, the efficacy and attending functions of Paeonia lactiflora were recorded in the famous book of ‘Shennong Materia Medica Classic’ (Eastern Han Dynasty, A.D. 25–220), indicating that Paeonia lactiflora has been used in medicine for more than 2,000 years [Citation2]. Paeonia lactiflora is suitable for mild temperature and full sunshine. It was historically produced in Bozhou (Anhui Province), Hangzhou (Zhejiang Province) and Zhongjiang (Sichuan Province) in China. Paeonia lactiflora cultivated in Bozhou (short for ‘Bo Baishao’) has been included in Chinese Pharmacopoeia (2020) as one of the four authentic medicinal materials in Anhui Province due to its large planting amount, better quality and better curative effect than other regions.
Heavy metals (HMs) are a class of elements with densities greater than 5 g/cm3 in the periodic table [Citation3,Citation4]. Their sources in soil include both natural sources caused by long-term geological process and parent material weathering and anthropogenic sources, such as fertilizer application, wastewater irrigation, traffic emissions, fossil fuel burning and other industrial, agricultural, and business activities [Citation4–6]. In recent years, with the continuous development of industrialization, agriculture and urbanization, heavy metals released by excessive human activities continue to accumulate in soil, resulting in serious soil heavy metal pollution, which has attracted worldwide attention [Citation6–8]. Heavy metals enriched in soil are characterized by non-biodegradability, enrichment, and toxicity, which in turn cause soil resource degradation and ecosystem deterioration [Citation6,Citation9]. Even more, the pollution of heavy metals on agricultural soil is of particular concern because agricultural soil is the main source of nutrients required for plant growth, and harmful substances such as heavy metals in soil can also be transferred to plants [Citation7,Citation10]. The accumulation of heavy metals in plants, especially edible plants (such as crops and medical plants) not only affects plant growth but also poses a great risk to human health through the food chain. According to the National Soil Pollution Survey Communique, jointly released by the Ministry of Environmental Protection and the Ministry of Land and Resources, 16.1% of China’s agricultural soil were contaminated to varying degrees, while inorganic pollution types, represented by heavy metals (Cu, Pb, Zn, Ni, As, Cd, Hg, and Cr) accounted for 82.8% of all sites exceeding the national standard level [Citation3,Citation6,Citation9,Citation11]. It is an effective method to explore the impact of soil heavy metals on plants by studying the distribution characteristics of heavy metals in the soil-plant system. At present, a large number of researches focus on the effects of heavy metals in soil on wheat [Citation9], rice [Citation12], corn [Citation13], vegetables [Citation14] and other plants [Citation11], while there are few studies on the soil-medicinal plant system. Therefore, in this study, the migration and transformation characteristics of heavy metals in a soil and medicinal plant system (Paeonia lactiflora Pall.) was investigated.
In this study, a total of 14 Paeonia lactiflora plants and corresponding rhizosphere soil samples were collected from Bozhou City, Anhui Province, China. The main objectives of this study were to investigate (1) the concentrations of Al, Fe, Mn, Cu, Pb, Zn, Cr, As and Cd in soil and different organs (root, stem, leaf) of Paeonia lactiflora; (2) the accumulation characteristics of these nine heavy metals in soil samples; (3) the transfer capacity of heavy metals among soil and different parts of Paeonia lactiflora; and (4) the correlation between heavy metals in soil and different parts of Paeonia lactiflora.
2. Materials and methods
2.1 Study area
Bozhou City is located in the northwest of Anhui Province, China, with a total area of 8,429 square kilometers (km2). This study observes the Qiaocheng District in Bozhou City as the main study area, which is about 50 kilometers wide from east to west, and about 70 kilometers long from north to south (115°32’24“E − 116°01’35”̋E, 33°42’48“N − 34°04’56“N, ). Qiaocheng District covers a total area of 2,226 km2, of which 1,320 km2 is cultivated land. All the Paeonia lactiflora plants and soil samples were collected from this area.
2.2 Sample collection and preparation
In total, 14 Paeonia lactiflora plants (Chinese herbaceous peony) were uprooted together with their stems and leaves from Qiaocheng District of Bozhou City, Anhui province, China, in May 2021 (). Then, after gently shaking to remove clumps of soil, the rhizosphere soil of each Paeonia lactiflora plant was collected with a brush. All the Paeonia lactiflora plants and soil samples were packed in sealed and labeled plastic bags and transported back to the laboratory for preparation.
In the laboratory, the Paeonia lactiflora plants were completely washed with tap water to remove adhering dust and soil, then washed several times with deionized water, and sequentially air-dried at room temperature. Then, the Paeonia lactiflora plants were separated into the roots (labeled as R1-R14), stems (labeled as S1-S14), and leaves (labeled as L1-L14) using a stainless steel knife. All the dried plant samples were ground into fine powder by an agate ball-grinder using 10-mm agate ball and then sieved through a 100-mesh sieve. Soil samples were also naturally air-dried and passed through a 2-mm nylon sieve to remove remnants of roots, twigs, stones, bricks, and other non-soil components. Then, the soil samples were ground into powder until all samples passed through the 100-mesh nylon sieve and labeled as T1-T14 for soil samples.
The powdered soil samples and roots, stems, and leaves of Paeonia lactiflora plants were all stored in sealed polyethylene containers, and kept in refrigerator at 4°C for preservation before further chemical assay.
2.3 Experimental determination
2.3.1 Soil physicochemical properties
The soil pH and organic matter (OM) of each soil sample were determined by standard methods. Soil pH was measured using the glass electrode method (NY/T 1377–2007) by soaking the pH probe in the soil suspension with a ratio of 1:2.5 of soil to ultrapure water (w/v). The content of the organic matter in each soil sample was measured using the potassium dichromate oxidation method (NY/T 1121.6–2006) through the oxidation of OM using K2Cr2O7 in an acid medium.
2.3.2 Total concentrations of heavy metals
An aliquot of 40.0 mg of each powdered soil sample and roots, stems, and leaves of Paeonia lactiflora plants was weighted into the clean and dry labeled Teflon bottles. Each part of Paeonia lactiflora was digested with 4 mL of concentrated nitric acid (HNO3), while an acid mixture of concentrated nitric acid (HNO3) and hydrofluoric acid (HF) with a ratio of 1:1 (3 mL: 3 mL) were added into soil samples. Thereafter, all the samples were digested using the oven digestion system with the temperature of 180°C for 12 h. For soil samples, after digestion for 12 h, the residual acids in the Teflon bottles were expelled in an acid capture instrument for approximately 30–45 min at 150°C. Then, an additional of 2 mL HNO3 and 1 mL of hydrogen peroxide (H2O2, 30%) were added and digested again in the oven at the temperature of 150°C for another 6 h. Finally, after cooling, all the digested soil samples, and digested root, stem and leaf samples of Paeonia lactiflora were filtered with 0.45 μm filter papers, and were diluted with distilled deionized water to 25 mL.
Total concentrations of HMs (Al, Fe, Mn, Cu, Pb, Zn, Cr, As, and Cd) in roots, stems, and leaves of Paeonia lactiflora, and that of Mn, Cu, Pb, Zn, Cr, As, and Cd in soil samples were determined by inductively coupled plasma-mass spectrometry (ICP-MS) (ICAPQ, Thermo, U.S.A). Due to the high content of Al and Fe in soils, the total concentrations of Al and Fe in soil samples were analyzed by inductively coupled plasma-optical emission spectrometry (ICP-OES) (ICAP 7200, Thermo, U.S.A) [Citation15,Citation16].
2.3.3 Extractable concentrations of heavy metals in soils
The total content of heavy metals in soils is a poor indicator of metal mobility and bioavailability to plants [Citation17,Citation18]. These properties of heavy metals in soils are based on their different chemical forms, which can be obtained through leaching of the soil using chemical extractants [Citation18,Citation19]. Diethylene triamine pentaacetic acid (DTPA), an efficient chelating agent with strong chelation, can quickly form water-soluble complexes with most of heavy metals. DTPA can release soluble, exchangeable, adsorptive, and organically bound metal fractions, and may also release some metals fixed by oxides [Citation20]. Thus, in the present study, to estimate the mobility and bioavailability of HMs in soils, the diethylene triamine pentaacetic acid (DTPA) method (NT/T 890–2004) was used to extract the available concentrations of Fe, Mn, Cu, Pb, and Zn in soil samples. An aliquot of 10.00 g of dried soil samples (<2 mm) were placed into 150 mL labeled centrifuge tubes, and 20 mL of DTPA extractant was added. Then, all the samples were shaken for 2 h at a frequency of 200 r/min. Thereafter, the samples were centrifuged for 10 min at 4500 r/min, and the supernate of each sample was filtered through 0.45 μm filter paper into 25 mL labelled volumetric flask for further determination. The DTPA-extractable concentrations of Fe, Mn, Cu, Pb, and Zn in the filtrate were detected by flame atomic absorption spectroscopy (FAAS) (AA−6880, Shimadzu, Japan).
During the experiment, before use, all the glassware and plastic containers were soaked in 10% HNO3 for at least 24 h, and then thoroughly washed with deionized water [Citation9]. In addition, the concentrated nitric acid, hydrofluoric acid, and hydrogen peroxide used in the digestion were all of guaranteed reagent grade.
2.4 Quality control
A quality control program was conducted which included procedural blanks, replicate samples, and standard reference material (GBW 10,027 (GSB−18) for plants, GBW07403 (GSS−3) for soils, and GBW 07460 (ASA−9) for DTPA method). The determined heavy metal concentrations showed good agreement with the standard reference material, and the recoveries varied from 73.29% to 101.0% for plant samples, 85.70% to 112.2% for total concentrations of HMs in soil samples, and 83.88% to 113.9% for available concentrations of HMs in soils. The relative standard deviations (RSD) of the randomly selected replicate samples ranged from 0.08% to 8.68%.
2.5 Assessment of potential heavy metal accumulation
In this study, to assess the heavy metal accumulation in soils, the geoaccumulation index (Igeo), potential ecological risk index (RI), and pollution load index (PLI) were used.
2.5.1 Geo-accumulation index
The geoaccumulation index (Igeo) was calculated to evaluate the heavy metal contamination in soils using the following equation [Citation21]:
Where Ci is the measured total concentration of a single heavy metal i in soil samples, andis the geochemical background values of the heavy metal i. In this study, the soil background values of heavy metals in (North) Anhui Province were used [Citation22,Citation23]. The constant 1.5 is a correction factor that accounts for possible natural variations due to the lithogenic effects [Citation24,Citation25].
The geoaccumulation index (Igeo) included the following seven categories: practically unpolluted (Igeo ≦ 0, Class 0), unpolluted to moderately polluted (0 <Igeo ≦1, Class 1), moderately polluted (1 <Igeo ≦2, Class 2), moderately to heavily polluted (2 <Igeo ≦3, Class 3), heavily polluted (3 <Igeo ≦4, Class 4), heavily to extremely polluted (4 <Igeo ≦5, Class 5), and extremely polluted (Igeo ≧5, Class 6) [Citation24,Citation26].
2.5.2 Potential ecological risk index
The potential ecological risk index (RI) is a widely used method to assess the risk of heavy metal elements in soils and first proposed by Hakanson [Citation27]. This index is calculated based on the following formula:
Whereis the contamination factor of an individual heavy metal i in soil samples,
describes the potential ecological risk coefficient of the heavy metal i, where r is the abbreviation of risk, and is not a variable;
is its ‘toxic-response’ factor, indicating the toxicity level of heavy metals to organisms. In this study, the
of Mn, Cu, Pb, Zn, Cr, As, and Cd is 1, 5, 5, 1, 2, 10, 30, respectively [Citation28]. In addition, RI represents the potential ecological hazard index of the total heavy metals in soil samples [Citation29]. The classifications of
and RI are presented as follows: low risk (
≦40; RI≦150), moderate risk (40<
≦80; 150≦300), considerable risk (80<
≦160; 3000), high risk (160<
≦320; RI > 600), extreme risk (
>320) [Citation30,Citation31].
2.5.3 Pollution load index
The pollution load index (PLI) is used to indicate the contamination level of the total heavy metals in soil samples and is defined as follows [Citation32,Citation33]:
Where n is the number of the analyzed HMs. The PLI divided the soil samples into four categories: no contamination (PLI ≦ 1), moderate contamination (1 <PLI ≦ 2), heavy contamination (2 < PLI ≦3), and extremely heavy contamination (PLI >3) [Citation24].
2.6 Transfer factor
In order to evaluate the potential capability of heavy metal transfer from soils to Paeonia lactiflora, the bioconcentration factor (BCF) and translocation factor (TF) were calculated using the following equations [Citation9,Citation11,Citation34,Citation35]:
Where BCFtotal represents the transfer capacity of a given heavy metal i from soil to root, calculated on the basis of the total (Ctotal(i)) concentrations of the heavy metal i in soil, and BCFavailable is the transfer factor of a given heavy metal i from soil to root, calculated on the basis of the available (Cavailable(i)) concentrations of the heavy metal i in soil. In addition, TFRS and TFSL express the transfer capacity of a given heavy metal i from root to stem, and from stem to leave, respectively. CRoot(i), CStem(i), and CLeaf(i) are the total concentrations of the heavy metal i in roots, stems, and leaves of Paeonia lactiflora, respectively.
2.7 Statistical analysis
In this study, correlation analysis was conducted to explore the relationship among soil pH, OM, and heavy metals in soils and each parts of Paeonia lactiflora. Data collection, statistics, and correlation analysis were conducted using Microsoft Office 2016 and the statistical software package SPSS 27 for Windows (IBM Corporation, U.S.A). In addition, the figures in this study were drawn using CorelDraw 2021 (Corel Corporation, Canada) and Origin 9.1 (Origin Lab, U.S.A), respectively.
3. Results and discussion
3.1 Soil physicochemical characteristics
Description of soil physicochemical parameters (pH and OM) of the 14 soil samples collected from Bozhou city are shown in . The soil pH values ranged from 8.06 to 8.32, with an average of 8.19, indicating slightly alkaline characteristics of the soils. The content of organic matter (OM) in the studied soil, varied from 10.50 to 29.87 g/kg, with an average of 17.00 g/kg.
Table 1. Statistical description of total and DTPA extracted heavy metal concentrations in soils and their background values (mg/kg).
3.2 Characteristics of heavy metals in soils
The total concentrations of Al, Fe, Mn, Cu, Pb, Zn, Cr, As, and Cd in these soil samples were quite different () and ranged from 34,434 to 67,264 mg/kg, 18495 to 41,200 mg/kg, 334.08 to 742.60 mg/kg, 12.28 to 30.74 mg/kg, 13.44 to 30.15 mg/kg, 36.24 to 70.25 mg/kg, 239.00 to 597.44 mg/kg, 7.40 to 15.51 mg/kg, 0.11 to o.20 mg/kg, respectively. The order on the basis of the average concentrations of HMs in soils was Al > Fe > Mn > Cr > Zn > Cu > Pb > As > Cd. This result is similar to that obtained in Hebei Province, China, which is close to Anhui Province in geological location [Citation36]. In comparison with their respective soil background values in Anhui Province, the HMs were enriched to different degrees. Among them, the average values of Fe, Mn, Cr, and As were, respectively, 1.05, 1.27, 5.80, and 1.18 times higher than their corresponding soil background values (), showing that Cr was the most enriched element.
Moreover, results of the geoaccumulation index (Igeo), potential ecological risk index (RI), and pollution load index (PLI) were shown in . Based on the average value of the geo-accumulation index (Igeo), Cr (Igeo = 1.87) was the only moderately polluted element in the soil, while the other heavy metals (Al, Fe, Mn,Cu, Pb, Zn, As, and Cd) were categorized in the non-polluted scope (Igeo <0). In general, the results of assessing the contamination level of the total heavy metals in soil samples through the pollution load index showed that the studied soils presented moderate contamination (1 <PLI ≦ 2), with an average PLI value of 1.20, of which Cr was the largest contributor. Nevertheless, in this study, HMs assessed by potential ecological risk index had no potential risk to the soil environment (≦40, and RI≦150). Due to the high enrichment of Cr in comparison with its soil background value, and its high Igeo value, Cr was considered as the priority pollutant of the soil-Paeonia lactiflora system in this study, and might be affected by human activities. Therefore, special attention should be paid to soil Cr to prevent further accumulation in the soil.
Table 2. Results of the geoaccumulation index (Igeo), potential ecological risk index ( and RI), and pollution load index (PLI).
3.3 Concentrations of DTPA extractable heavy metals
The bio-available concentrations of heavy metals extracted by DTPA in soils were also shown in . It is reported that the toxicity assessment of heavy metals based on their bio-available concentrations in the soil is better than the assessment of total metal concentrations [Citation19,Citation20]. DTPA extraction is a widely used and effective method to evaluate the heavy metal bioavailability in soils [Citation37]. In the present study, the content of Fe, Mn, Cu, Pb, and Zn extracted by DTPA were 43.97–64.18, 17.64–17.89, 1.44–3.09, 2.36–4.19, 1.15–2.19 mg/kg, respectively. On average, the proportion of metals extracted by DTPA was in a descending order as follows: Pb (13.78%) > Cu (8.57%) > Mn (3.04%) > Zn (2.84%) > Fe (0.15%), indicating the highest bioavailability of Pb in soil. The bio-available content of Cu, Pb, and Zn in this study was comparable with a previous study [Citation38], but far lower than that in soil irrigated with contaminated water [Citation5]. Due to the lack of the standard limits of bioavailable heavy metals in Chinese soil, the pollution degree based on DTPA extractable heavy metals was not evaluated [Citation5].
Besides, correlation analysis was conducted between the total and DTPA extractable concentrations of heavy metals to evaluate their relationship (). First, significant correlations were shown between each two of HMs (Al, Fe, Mn, Cu, Pb, Zn, Cd and As) in the soil, with the correlation coefficients ranging from 0.790 to 0.993 (P < 0.01). The cross-correlations indicated the same sources of these heavy metals in the soil. However, Cr in the soil showed a negative correlation with the other eight HMs. Second, the content of Cu extracted by DTPA displayed significant positive correlation with its total concentrations (r = 0.809, P < 0.01) in the soil, indicating that the mineralogical source and pedological processes of the available Cu was the same as their total content [Citation20]. However, with respect to Fe, Mn, Pb, and Zn, the relationship between their total and DTPA extractable content were poor.
Table 3. Correlation coefficient of total and DTPA extracted heavy metals in soils.
3.4 Heavy metals in different tissues of Paeonia lactiflora
The concentrations of HMs in different parts (roots, stems, and leaves) of Paeonia lactiflora were shown in . The average concentrations of HMs in stems and leaves adhered to the same order: Al > Fe > Zn > Mn > Cu > Cr > Pb > As > Cd, and the order of HMs in roots decreased in a similar pattern with that of HMs in soil: Al > Fe > Mn > Zn > Cr > Cu > Pb > As > Cd. By comparing the concentrations of HMs in different parts of Paeonia lactiflora, the average concentration of the nine heavy metals studied in leaves and stems were all higher than those in roots. Specifically, Al, Fe, Mn, Pb, Zn, Cr, and As were mainly concentrated in leaves, Cu and Cd were mainly accumulated in stems. Among the heavy metals studied, the Pb content in leaves and stems, respectively, was 17.49 and 9.25 times higher than that in roots, indicating a great transfer of Pb from root to stem and leaf. Furthermore, it is reported that air (atmospheric deposits) as well as soil is also an important source of heavy metals in plant leaves, which may lead to the higher content of heavy metals in leaves than other plant tissues [Citation39]. As known, Pb is a semi-volatile element, and Pb from coal combustion, automobile exhaust and industrial activities tends to be attached to atmospheric fine particles and move over a long distance away from the emission sources [Citation40]. It is reported that in the past few decades, with the rapid development of Bozhou’ economy, different types of industries have flourished in this city [Citation41]. The highest concentrations of Pb in leaves were probably resulted from the atmospheric deposition as well as from the soil. More importantly, heavy metals accumulated in plant leaves can re-enter the soil as a result of plant leaf litter, which may pose potential risks to the soil ecosystem, and therefore need to be strengthened monitoring [Citation5].
Table 4. Concentrations of heavy metals in root, stem, and leaf of Paeonia lactiflora, and their limits in CHMs (mg/kg).
In addition, as the edible and medicinal parts of Paeonia lactiflora, heavy metal concentrations of Cu, Pb, As, and Cd in the roots were compared with the standard limits formulated by the Chinese Pharmacopoeia Commission (2020), which were 20, 5, 2, and 1 mg/kg, respectively. The results in this study showed that there were no elements with concentrations higher than the respective standard limits.
3.5 Transfer capacity of heavy metals
The transfer of HMs from soil to plants is an important pathway of human exposure through food chain [Citation42]. Hence, the transfer capacity of HMs from the soils to different parts (root, stem, and leaf) of Paeonia lactiflora was evaluated using the bioconcentration factor (BCF) and translocation factor (TF), and the results were shown in . The values of BCF and TF varied significantly among different HMs. In general, the bioconcentration factors (BCFtotal) of HMs from soil to roots of Paeonia lactiflora were lower than 1 and descended in the following order: Zn > Cu > Cd > Mn > Cr > Pb > As > Fe > Al (). However, the bioconcentration factors (BCFavailable) from soil to roots of Paeonia lactiflora calculated based on the concentrations of HMs in the soil extracted by DTPA were 7.39, 2.30, 1.19, 0.34, and 0.03 for Zn, Cu, Fe, Mn, and Pb, respectively (). This result suggested that Paeonia lactiflora was a plant with high potential to absorb Zn, Cu and Fe from the soil [Citation11,Citation43]. Additionally, the average translocation factors between different parts of Paeonia lactiflora were higher than 1, except for Cd with the average TFSL value of 0.59 (). By analyzing the transfer factors (BCF and TF) of HMs from soil to root, root to stem and stem to leaf, the results showed that the transfer ability of most heavy metals was in the order of root to stem > stem to leaf > soil to root, indicating that the possibility of HMs transfer from soil to root was lower than the possibility of HMs transfer from root to stem and leaves. Our result was consistent with that in a soil-wheat system [Citation9]. This might be due to more obstacles preventing the transfer of heavy metals from soil to the root, and once the metals entered the root, they would be transferred to the aboveground parts of the plant [Citation42]. It is reported that soil properties, such as soil pH and organic matter, were important factors affecting HMs bioavailability and mobility [Citation42,Citation44]. The low BCF values of HMs in this study could be associated to the high soil pH (mean value of 8.19) in this study area.
Figure 2. Bioconcentration factor (BCF) and translocation factor (TF) of heavy metals (HMs) from soil to different parts of Paeonia lactiflora (a) BCF based on total concentrations of HMs from soil to root, (b) BCF based on concentrations of HMs extracted by DTPA from soil to root, (c) TF of HMs from root to stem, and from stem to leaf.

Among the heavy metals studied, the translocation factor of Pb from root to stem (TFRS = 13.19) was the highest. The transfer factor of Pb from stem to leaf (TFSL) and from soil to root (BCFavailable) was 2.47 and 0.03, respectively. As described in section 3.3, the Pb content in leaves and stems was 17.49 and 9.25 times higher than that in roots, respectively, which might be due to the higher transfer capacity of Pb from root to stem, and from stem to leaf. In addition, studies have shown that the translocation of heavy metals from soil to plants has a good correlation with their bio-available concentrations in soil [Citation19,Citation38]. Among the heavy metals of Cu, Pb, Zn, Fe, and Mn, the proportion (13.78%) of Pb extracted by DTPA was the highest. However, a low bioconcentration factor (BCFavailable) of Pb was found. The large amount of migration from root to stem could have resulted in the reduction of the Pb concentration in root, in turn leading to its low bioconcentration factor (BCFavailable) from soil to root.
In addition, according to the average values of BCFtotal and BCFavailable, Zn and Cu showed the highest transfer capacity from soil to roots of Paeonia lactiflora, but displayed lower transfer capacity from root to stem, and from stems to leaves in comparison with the other HMs. In contrast, based on BCFtotal, the proportion of Al and Fe transferred from soil to roots of Paeonia lactiflora was the least, while the transfer ability of Al and Fe was strong in the process of transferring from roots to stems and from stems to leaves. It has been reported that soil microorganisms, such as Bacillus subtilis and mycorrhizal fungi, can enhance metal solubility by increasing CO2 release and decomposition of organic matter, and play different roles in different metal removal [Citation45,Citation46]. Therefore, the soil microorganisms may have affected the transfer capacity of HMs in soil.
3.6 Correlation analysis
The potential relationship among soil pH, OM, and heavy metals in soil and different parts of Paeonia lactiflora was discussed based on the results of correlation analysis (). First of all, root Cd was significantly positively correlated with soil pH (P < 0.05), and root Cr showed significant positive correlation with organic matter (P < 0.05). However, correlations among soil pH, OM and the other heavy metals in the roots of Paeonia lactiflora were not significant, suggesting that in addition to soil pH and organic matter, other soil properties, such as cation exchange capacity, and soil microorganisms might also influence HM transfer [Citation10,Citation45,Citation46].
Table 5. Correlation coefficients of heavy metals between soil, root, stem and leaf of Paeonia lactiflora.
Second, Mn and Cd in roots of Paeonia lactiflora are significantly correlated with most metal elements in the soil. Specifically, Mn in roots is negatively correlated with Fe, Mn, Cu, Pb, Zn, and As in soil, and positively correlated with Cr in soil. Cd in roots is significantly positively correlated with Al, Fe, Mn, Cu, Pb, Zn, As and Cd in soil. In addition, Cu in roots has significant positive correlation with soil Al, Fe, and Cu. However, the other heavy metals (Al, Fe, Pb, Zn, Cr and As) showed no significant correlation between soil and roots of Paeonia lactiflora. Moreover, Cu and Zn extracted by DTPA were significantly positively correlated with As and Cd in the roots of Paeonia lactiflora, while negatively correlated with Mn in the root. Third, the correlation results of heavy metals in stems and roots showed that most metals in stems of Paeonia lactiflora were not highly correlated with root metals, but Al, Fe, Cu, Pb and Cd in stems were positively correlated with As and Cd in roots. Fourth, there is a significant positive correlation between Al, Fe in the stems of Paeonia lactiflora and Al, Fe, Pb, Cr, As, and Cd in the leaves.
4. Conclusion
The accumulation and translocation of heavy metals from the rhizosphere soil to Paeonia lactiflora grown in Bozhou, Anhui Province, China, was discussed in this study. Combined geoaccumulation index, potential ecological risk index, and pollution load index were used to evaluate the heavy metal contamination and their environmental risk in the soil. The results showed that the soil was moderately contaminated by the nine HMs (Al, Fe, Mn, Cu, Pb, Zn, Cr, As, and Cd), and Cr was the largest contributor.
Distribution of HMs in Paeonia lactiflora showed that the metals in leaves and stems were higher than those in roots. Specifically, Al, Fe, Mn, Pb, Zn, Cr, and As were mainly concentrated in leaves, Cu and Cd were accumulated in stems. The highest concentrations of Pb in leaves were probably resulted from the atmospheric deposition as well as from the soil. Zn and Cu showed the highest transfer capacity from soil to roots of Paeonia lactiflora, but the proportion of Al and Fe transferred from soil to roots of Paeonia lactiflora was the least, which might be due to the high content of Al and Fe in soil. Moreover, Mn, Cu and Cd in roots of Paeonia lactiflora were significantly correlated with soil Mn, Cu and Cd, respectively. However, Al, Fe, Pb, Zn, Cr and As showed no significant correlation between soil and roots.
Disclosure statement
No potential conflict of interest was reported by the author(s).
Data availability statement
The datasets analyzed during the current study are available from the corresponding author on reasonable request https://doi.org/0000-0002-8898-2779.
Additional information
Funding
References
- Bak S-M, Back S-M, Kim DY, et al. Genotoxicity assessment of root extracts of Paeonia lactiflora Pall. Mutat Res Genet Toxicol Environ Mutagen. 2023;886:503579. doi: 10.1016/j.mrgentox.2022.503579
- Jiang H, Li J, Wang L, et al. Total glucosides of paeony: a review of its phytochemistry, role in autoimmune diseases, and mechanisms of action. J Ethnopharmacol. 2020;258:112913. doi: 10.1016/j.jep.2020.112913
- Tholley MS, George LY, Wang G, et al. Risk assessment and source apportionment of heavy metalloids from typical farmlands provinces in China. Process SafEnviron Prot. 2023;171:109–303. doi: 10.1016/j.psep.2022.12.092
- Oves M, Khan MS, Zaidi A, et al. Soil contamination, nutritive value, and human health risk assessment of heavy metals: an overview. Vienna: Springer Vienna; 2012. doi: 10.1007/978-3-7091-0730-0_1.
- Yang L, Ren Q, Zheng K, et al. Migration of heavy metals in the soil-grape system and potential health risk assessment. Sci Total Environ. 2022;806:150646. doi: 10.1016/j.scitotenv.2021.150646
- Wu Y, Li X, Yu L, et al. Review of soil heavy metal pollution in China: spatial distribution, primary sources, and remediation alternatives. ResouConserv Recycl. 2022;181:106261. doi: 10.1016/j.resconrec.2022.106261
- Zhang G, Song K, Huang Q, et al. Heavy metal pollution and net greenhouse gas emissions in a rice-wheat rotation system as influenced by partial organic substitution. J Environ Manage. 2022;307:114599. doi: 10.1016/j.jenvman.2022.114599
- Yan X, An J, Yin Y, et al. Heavy metals uptake and translocation of typical wetland plants and their ecological effects on the coastal soil of a contaminated bay in Northeast China. Sci Total Environ. 2022;803:149871. doi: 10.1016/j.scitotenv.2021.149871
- Xu J, Li Y, Wang S, et al. Sources, transfers and the fate of heavy metals in soil-wheat systems: the case of lead (Pb)/zinc (Zn) smelting region. J Hazard Mater. 2023;441:129863. doi: 10.1016/j.jhazmat.2022.129863
- Chen R, Cai X, Ding G, et al. Ecological risk assessment of heavy metals in farmland soils in Beijing by three improved risk assessment methods. Environ Sci Pollut Res Int. 2021;28(41):57970–57982. doi: 10.1007/s11356-021-14695-8.
- Wang Z, Liu X, Qin H. Bioconcentration and translocation of heavy metals in the soil-plants system in Machangqing copper mine, Yunnan Province, China. J Geochem Explor. 2019;200:159–166. doi: 10.1016/j.gexplo.2019.02.005
- Li P, Hao H, Zhang Z, et al. A field study to estimate heavy metal concentrations in a soil-rice system: application of graph neural networks. Sci Total Environ. 2022;832:155099–. doi: 10.1016/j.scitotenv.2022.155099
- Fei Z, Wang S, Nan Z, et al. Accumulation, spatio-temporal distribution, and risk assessment of heavy metals in the soil-corn system around a polymetallic mining area from the Loess Plateau, northwest China. Geoderma. 2017;305:188–196. doi: 10.1016/j.geoderma.2017.06.008
- Qiutong X, Mingkui Z. Source identification and exchangeability of heavy metals accumulated in vegetable soils in the coastal plain of eastern Zhejiang province, China. Ecotoxicol Environ Saf. 2017;142:410–416. doi: 10.1016/j.ecoenv.2017.03.035
- Saputro SP, Godang S, Priadi B, et al. Geochemical study of Al–Fe–Ti enrichment in rock weathering: implications for the recognizing of igneous protolith and the enrichment of REE in soil profile. Appl Geochem. 2022;140:105259. doi: 10.1016/j.apgeochem.2022.105259
- Watanabe T, Ueda S, Nakao A, et al. Disentangling the pedogenic factors controlling active Al and Fe concentrations in soils of the Cameroon volcanic line. Geoderma. 2023;430:116289. doi: 10.1016/j.geoderma.2022.116289
- Qureshi AA, Kazi TG, Baig JA, et al. Exposure of heavy metals in coal gangue soil, in and outside the mining area using BCR conventional and vortex assisted and single step extraction methods. Impact on orchard grass. Chemosphere. 2020;255:126960. doi: 10.1016/j.chemosphere.2020.126960
- Nemati K, Abu Bakar NK, Abas MR, et al. Speciation of heavy metals by modified BCR sequential extraction procedure in different depths of sediments from Sungai Buloh, Selangor, Malaysia. J Hazard Mater. 2011;192:402–410. doi: 10.1016/j.jhazmat.2011.05.039
- Jamali MK, Kazi TG, Arain MB, et al. Heavy metal accumulation in different varieties of wheat (Triticum aestivum L.) grown in soil amended with domestic sewage sludge. J Hazard Mater. 2009;164(2–3):1386–1391. doi: 10.1016/j.jhazmat.2008.09.056.
- Dai J. Heavy metal accumulation by two earthworm species and its relationship to total and DTPA-extractable metals in soils. Soil Biol Biochem. 2004;36(1):91–98. doi: 10.1016/j.soilbio.2003.09.001.
- Muller G. Index of geoaccumulation in sediments of the Rhine River. GeoJournal. 1969;2:109–118.
- Li PF, Tao CJ. Heavy metal content characteristics and environmental quality assessment of agricultural land in the Yellow River flooded area of Anhui Province. J Geol. 2019;43(2): 301–306. in Chinese.
- Yang DL. Pollution characteristics and health risk assessment of heavy metals in topsoil of Hefei City proper [ M.S. Thesis]. Hefei: Anhui Medical University; 2021. doi: 10.26921/d.cnki.ganyu.2021.000673. [In Chinese]
- Aydi A, Ghannem S, Nasri A, et al. Evaluation of heavy metals contamination and pollution indices levels in surface sediments of the Bizerte coastal line, Tunisia. Mar Pollut Bull. 2022;184:114171. doi: 10.1016/j.marpolbul.2022.114171
- Liu Z, Du Q, Guan Q, et al. A Monte Carlo simulation-based health risk assessment of heavy metals in soils of an oasis agricultural region in northwest China. Sci Total Environ. 2023;857:159543. doi: 10.1016/j.scitotenv.2022.159543
- Magni LF, Castro LN, Rendina AE. Evaluation of heavy metal contamination levels in river sediments and their risk to human health in urban areas: a case study in the Matanza-Riachuelo Basin, Argentina. Environ Res. 2021;197:110979. doi: 10.1016/j.envres.2021.110979
- Lars H. An ecological risk index for aquatic pollution control.A sedimentological approach. Water Res. 1980;14(8):975–1001. doi: 10.1016/0043-1354(80)90143-8.
- Zhou L, Liu G, Shen M, et al. Potential ecological and health risks of heavy metals for indoor and corresponding outdoor dust in Hefei, Central China. Chemosphere. 2022;302:134864. doi: 10.1016/j.chemosphere.2022.134864
- Xiang Q, Yu H, Chu H, et al. The potential ecological risk assessment of soil heavy metals using self-organizing map. Sci Total Environ. 2022;843:156978. doi: 10.1016/j.scitotenv.2022.156978
- Hoshyari E, Hassanzadeh N, Keshavarzi B, et al. Spatial distribution, source apportionment, and ecological risk assessment of elements (PTEs, REEs, and ENs) in the surface soil of shiraz city (Iran) under different land-use types. Chemosphere. 2023;311:137045. doi: 10.1016/j.chemosphere.2022.137045
- Men C, Liu R, Xu L, et al. Source-specific ecological risk analysis and critical source identification of heavy metals in road dust in Beijing, China. J Hazard Mater. 2020;388:121763. doi: 10.1016/j.jhazmat.2019.121763
- Angulo E. The tomlinson pollution load index applied to heavy metal, ‘Mussel-Watch’ data: a useful index to assess coastal pollution. Sci Total Environ. 1996;187(1):19–56. doi: 10.1016/0048-9697(96)05128-5.
- Tomlinson DL, Wilson JG, Harris CR, et al. Problems in the assessment of heavy-metal levels in estuaries and the formation of a pollution index. Helgolnder Meeresuntersuchungen. 1980;33(1–4):566–575. doi: 10.1007/BF02414780.
- Waris M, Baig JA, Talpur FN, et al. Evaluation of selected halophytes for phytoextraction of Co, Cu, Zn and capability of desalination of saline soil. Int J Environ Sci Technol. 2021;19(4):2737–2746. doi: 10.1007/s13762-021-03269-3.
- Waris M, Baig JA, Talpur FN, et al. An environmental field assessment of soil quality and phytoremediation of toxic metals from saline soil by selected halophytes. J Environ Health Sci Eng. 2022;20(1):535–544. doi: 10.1007/s40201-022-00800-7.
- Chen X-X, Liu Y-M, Zhao Q-Y, et al. Health risk assessment associated with heavy metal accumulation in wheat after long-term phosphorus fertilizer application. Environ Pollut. 2020;262:114348. doi: 10.1016/j.envpol.2020.114348
- Xiao L, Li MH, Dai J, et al. Assessment of earthworm activity on Cu, Cd, Pb and Zn bioavailability in contaminated soils using biota to soil accumulation factor and DTPA extraction. Ecotoxicol Environ Saf. 2020;195:110513. doi: 10.1016/j.ecoenv.2020.110513
- Rezapour S, Atashpaz B, Moghaddam SS, et al. Heavy metal bioavailability and accumulation in winter wheat (Triticum aestivum L.) irrigated with treated wastewater in calcareous soils. Sci Total Environ. 2019;656:261–269. doi: 10.1016/j.scitotenv.2018.11.288
- Milicevic T, Urosevic MA, Relic D, et al. Bioavailability of potentially toxic elements in soil-grapevine (leaf, skin, pulp and seed) system and environmental and health risk assessment. Sci Total Environ. 2018;626:528–545. doi: 10.1016/j.scitotenv.2018.01.094
- Wang J, Liu G, Liu H, et al. Tracking historical mobility behavior and sources of lead in the 59-year sediment core from the Huaihe River using lead isotopic compositions. Chemosphere. 2017;184:584–593. doi: 10.1016/j.chemosphere.2017.06.022
- Sheng J. The research of the industrial economy development in Bozhou City [ M.S. Thesis]. Hefei: Hefei University of Technology; 2010. [In Chinese]
- Hu W, Huang B, Tian K, et al. Heavy metals in intensive greenhouse vegetable production systems along Yellow Sea of China: levels, transfer and health risk. Chemosphere. 2017;167:82–90. doi: 10.1016/j.chemosphere.2016.09.122
- Lorestani B, Cheraghi M, Yousefi N. Accumulation of Pb, Fe, Mn, Cu and Zn in plants and choice of hyperaccumulator plant in the industrial town of Vian, Iran. Arch Biol Sci (Beogr). 2011;63(3):739–745. doi: 10.2298/ABS1103739L.
- Xu L, Lu A, Wang J, et al. Accumulation status, sources and phytoavailability of metals in greenhouse vegetable production systems in Beijing, China. Ecotoxicol Environ Saf. 2015;122:214–220. doi: 10.1016/j.ecoenv.2015.07.025
- Al-Maliki S, Al-Shamary A. Enhanced phytoremediation of soil heavy metals by arbuscular mycorrhizal fungi, Bacillus subtilis and CO2 release in Typha domingensis rhizosphere. Arab J Geosci. 2022;15(22). doi: 10.1007/s12517-022-10968-9
- Al-Maliki S, Al-Shamary A. Vital evidence for arbuscular mycorrhizal fungi, bacteria and cattail plant to remove Pb-Cd heavy metals from contaminated soils. Acta Ecologica Sinica. 2022;42(4):392–397. doi: 10.1016/j.chnaes.2022.05.008.