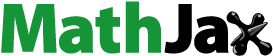
ABSTRACT
We investigated the contents and distributions of nutrients and heavy metals in sediment from the Yitong River. The main aims were to analyze heavy metals speciation and evaluate their bioavailability, in order to assess the risks they pose to both ecosystem and human health. The average total nitrogen (TN), total phosphorus (TP), organic matter (OM) and Cd, Cr, Cu, Ni, Pb and Zn contents were 1747.69, 895.58, 75103, 0.19, 75.64, 33.61, 47.17, 56.65 and 159.91 mg kg−1, respectively. Results from acid-extraction fraction analysis showed that the proportions of Cd and Zn ranged from 3.78% to 30.20% and 5.31% to 30.74%, respectively. Health risk assessment model showed the risk index values of children and adults were 0.412 and 0.059, respectively, indicating a minimal threat to human health. Multivariate statistical analyses revealed that TN and TP may have similar sources and could be attributed to combined sewer overflows, while Cr, Cu, Ni and Zn were primarily derived from industrial pollution sources. Toxicity bioassay tests demonstrated that the majority of sediment samples were classified as non-toxic. However, 25% of the samples showed slight toxicity towards Vibrio qinghaiensis Q67, while 7% of the samples exhibited slight toxicity towards Daphnia magna. Based on these findings, it is necessary to implement pollution control measures and conduct assessments of aquatic ecological risks in sediments from urban rivers. These results can be used to develop effective strategies for the management and governance of aquatic sediments.
1. Introduction
The rapid urban growth coupled with industrialization has led to a grave concern: urban river pollution, posing significant environmental problems and ecological risks [Citation1]. With anthropogenic activities, nitrogen and phosphorus nutrients, organic matter and heavy metals may be discharged into rivers and accumulate in sediments. Organic matter and nutrients can give rise to problems such as the development of black odour in river water and eutrophication, further exacerbating the degradation of the river aquatic environment. Heavy metal pollution has drawn significant attention as it poses risks to human health through biological enrichment and amplification in the food chain, influenced by both the total content and chemical form of the metals [Citation2–4]. In contrast to organics and nutrients, heavy metals are not easily removed through natural processes. The majority of heavy metals tend to adhere to suspended particles and accumulate in bottom sediments, leading to a potential threat of acute or chronic toxicity to benthic organisms and microorganisms [Citation5,Citation6].
The chemical fractions can be used to evaluate metal bioavailability and the environmental risk of heavy metals [Citation7]. The exchangeable metals are considered unstable and potentially bioavailable to aquatic organisms [Citation8]. The content of nutrients and the physicochemical properties of sediments can alter the ability of heavy metals to associate with weakly bound fractions in aquatic environments [Citation9,Citation10]. It is noteworthy that previous studies have mainly focused on the concentration and speciation of heavy metals in sediment [Citation1,Citation11,Citation12], while there has been limited research on directly analyzing the biological toxicity of sediments and their potential impact on human health for urban rivers. A previous study indicated that Daphnia magna can serve as a valuable monitoring tool for assessing the health of aquatic ecosystems [Citation13]. It is widely used in ecotoxicology studies to gain insights into the overall ecological condition of these ecosystems by measuring the survival, growth and reproduction of Daphnia magna [Citation14].
The Yitong River in China runs through the urban area of Changchun in Jilin province. This river has been highly correlated with the socioeconomic development of Changchun city [Citation15]. In the past, Changchun was a base for heavy industry, and large volumes of industrial and domestic sewage wastewater were discharged directly into the Yitong River. Consequently, many pollutants, including nutrients, organic matter (OM) and heavy metals, have accumulated in the river sediment. The Yitong River and its tributaries are malodorous black rivers and contain heavily polluted sediment [Citation16]. Between 2016 and 2021, comprehensive treatment of the Yitong River basin was carried out to eliminate black odorous water. Sewage interception, sediment treatment and ecological restoration have greatly improved the water quality of the Yitong River.
The main purposes of this study were to (1) identify the spatial distribution characteristics of nutrients and heavy metals in sediment from the Yitong River and its tributaries, (2) sediment biotoxicity with Vibrio qinghaiensis Q67 and D. magna and human health risk assessment. (3) To identify the potential sources of pollutants in the Yitong River using multivariate statistical methods. The results of sediment investigation and assessment are helpful to put forward strategies for urban river ecological management and restoration.
2. Materials and methods
2.1 Study area and sample collection
Yitong River originates in Dingdong Mountain in the southeast of Yitong County, Jilin province. The Yitong River is the main polluted river in Changchun, and it is also the most important water source for industrial water and irrigation water. The Xinlicheng Reservoir in the upper reaches of Yitong River is the drinking water source for Changchun. The main focus is on the section of the Yitong River that flows through the urban area of Changchun city, with a length of approximately 50 km. The upstream section of the Yitong River (YTU) is mainly a suburban area, the middle section of the catchment (YTC) is mainly residential land and roads and the downstream section (YTD) is mainly an industrial area and farmland. The Chuanhu River (CR), DongXinkai River (DXK) and Jing River (JR) are tributaries of the Yitong River. In the last century, over the past few decades, there has been a discharge of wastewater containing heavy metals into the Yitong River.
In May 2019, sediment cores were obtained from a depth of 0–10 cm using a grab bucket in 28 sampling points in the Yitong River and its tributaries (). The sediments were brought back to the laboratory in a car refrigerator. After drying, grinding and sieving (100 mesh) the sediment, it should be stored in a light-avoidant manner at 4°C
2.2 Sediment analyses
The concentration of TN and TP in sediment were determined based on standard methods [Citation17]. Organic matter in the sediment is determined by loss on ignition method [Citation18] after incineration at 550°C for 4 h. The European Community Bureau of Reference (BCR) was used to determine the chemical speciation of heavy metals, which were defined as exchangeable (F1), reducible (F2), oxidizable (F3) and residual (F4), as shown in Table S1 of support information (SI) [Citation8,Citation19]. The sediment samples were digested with HCl–HNO3–HF–HClO4 [Citation20]. The total amount of heavy metals and the content of the four extracted fractions in the sediment are determined by inductively coupled plasma mass spectrometry, which gave detection limits of 0.025–0.200 µg/L (Agilent 7500a, Agilent Technologies, U.S.A.). To ensure the quality of the analysis, we conducted quality assurance and control by analyzing three replicate samples, standard reference samples GBW07304 and GBW07436 (provided by the National Institute of Metrology in Beijing, China), as well as blank samples. The accuracy (recoveries of four fractions ranged from 78.32% to 114.68%) was suitable, and the relative standard deviations was of <5%.
2.3 Sediment quality assessment
The risk assessment code (RAC) is calculated by assessing the amount of metal in the acid-soluble phase relative to a total concentration [Citation21,Citation22].
The geo-accumulation index (Igeo) for each metal was calculated using the following equation [Citation23]:
where Cn is the concentration of the heavy metal, and Bn is the background value of the heavy metal (Cd, Cr, Cu, Ni, Pb and Zn were 0.099, 46.7, 17.1, 21.4, 28.8 and 80.4 mg kg−1, respectively). Each metal could be classified using the Igeo as shown in Table S2.
The enrichment factor (EF) is a normalization method widely used to separate natural metal variability in the sediment metal fraction from that associated with human activities [Citation8].
The potential ecological risk index (RI) was calculated using the following equation [Citation24]:
where Tri is the toxicity coefficient of the metals,the values of Cd, Cr, Cu, Ni, Pb and Zn were 30, 2, 5, 5, 5 and 1, respectively. Cin is the geological background values, and the Csi is the measured content of elements. The RI and Eir classifications are shown in Table S2 in SI.
The sum of the TU is used to evaluate the potential toxicity caused by metals, which is calculated using the following equation:
where is the total concentration of heavy metal i,
is the probable effect level of metal i [Citation25,Citation26].
The organic pollution index (OPI) is commonly used to evaluate the environmental status of sediments, and the organic nitrogen index (ONI) is a key indicator to measure whether surface sediments are polluted by nitrogen [Citation27]. The classifications for these indexes are shown in Table S2.
OPI = organic carbon (%) × organic nitrogen (%)
Organic carbon (%) = organic matter (%)/1.724
ONI (%) = total nitrogen (%) × 0.95.
2.4 Human health risk assessment
The Human Health Risk Assessment Model (HRAM) from the United States Environmental Protection Agency was used to assess the human health risk of metal in urban river sediment [Citation28]. Urban residents are exposed to heavy metals in sediments in three ways: ingestion (W1), inhalation (W2) and dermal intake (W3). The risk index (HI) was used to analyze the non-carcinogenic risk level of multiple substances, and the hazard quotient (HQ) was the ratio of HRAM (mg kg−1 d−1) and reference dose (RfD) (mg kg−1 d−1).
C is the content of metals in sediment, mg kg−1. The calculation parameter for HRAM and RfD is provided in Table S3 and Table S4 of SI, respectively [Citation28]. When , humans are exposed to the risk of non-carcinogenic effects.
2.5 Biotoxicity tests
We quantified the biotoxicity of Vibrio qinghaiensis Q67 based on the manufacturer’s guidelines (Binsong Guangzi, Hamamatsu, Japan). The porewater and Q67 solution were mixed 9:1, and the test samples were put into each well of the white 96-well plate (five replicates for samples). After cultivation for 15 min, the luminous value was measured with a microplate reader (Infinite M200, Tecan, U.S.A.). The luminous inhibition rate (LIR) was calculated with the formula:
where LB and Ln were luminous value of Vibrio qinghaiensis Q67 in blank group and experience group, respectively.
The acute biotoxicity for D. magna was as outlined in the experimental method of acute toxicity (GB/T 16,125-2012). For each sample, 10 offsprings were cultured with porewater with three replicates per sample. After 48-h cultivation, the survival number of D. magna was observed. Lethality of D. magna (Li) was calculated with the formula:
where NC and Ni were survival quantities of D. magna in control group and experience group, respectively.
2.5 Statistical analysis
The experimental data processing was conducted in ArcGIS 10.3. Principal component analysis was performed with IBM SPSS for Windows, version 18.0 (IBM Corporation, Armonk, NY, U.S.A.). Pearson correlation and two-way joining hierarchical cluster (heatmap) analysis were conducted with Origin 2021 software (OriginLab Inc., U.S.A.).
3 Results and discussion
3.1 Spatial distribution of heavy metals and nutrients in sediments
The TN, TP and OM concentrations in sediments from 28 sites in the Yitong River (). The highest TN concentration was approximately 5203.26 mg kg−1, and the average was 1747.69 ± 1282.44 mg kg−1. Total nitrogen and total phosphorus are mainly enriched in the middle section of the Yitong River, and the highest concentration occurred at site Z4. The TN concentrations in the river showed obvious regional differences with a much lower TN concentration in the YTU (911.19 mg kg−1) than in the YTC (2640.49 mg kg−1) or YTD (1973.86 mg kg −1). The TP concentration range was 103.64–2613.46 mg kg−1 (average: 655.58 ± 853.66 mg kg−1), and the highest concentration occurred at site Z5 in the YTC. Overall, the TN and TP concentrations in the surface sediments in two tributaries of the YTH (Jing River and Xinkai River) were relatively low, but there was an obvious area where TN and TP were enriched in the Chuanhu River. The spatial distribution of the OM was inconsistent with the TN and TP concentration distributions. The average content of OM was 7.51%, and the highest value occurred at site S2 in the YTU.
Figure 2. Heavy metals concentrations (mg kg−1), total nitrogen concentrations (TN, mg kg−1), total phosphorus concentrations (TP, mg kg−1) and organic matter contents (OM, %) in sediments from the Yitong River.

The spatial patterns of heavy metal concentrations in surface sediments collected from the Yitong River are illustrated in . The average concentrations of Cd, Cr, Cu, Ni, Pb and Zn were measured at 0.19, 75.64, 33.62, 41.03, 37.20 and 156.72 mg kg−1, respectively. The average concentrations of metals found in this study, as well as those observed in urban rivers in other regions, can be found in Table S5 provided in the Supplementary Information (SI). Notably, the concentrations of Cd, Cu and Zn were found to be 2.01, 2.23 and 2.54 times higher than their respective background values. The concentration of Zn in sediments ranged from 61.55 mg kg−1 at site S1 to 355.68 mg kg−1 at site C5, and content of Zn exceeded the background value, except S1. There are two main nutrient-rich areas in the main stream of the Yitong River. These areas are located at sites Z4 and C4 near the combined overflow pollution-discharge outlet in the built-up area of Changchun. The results indicate that large amounts of pollutants may enter the middle of the Yitong River from these discharge outlets, particularly during flooding [Citation29]. Changchun city drainage system is a combined system, where part of the rainwater could mix into the sewage pipe network, resulting in pipe network overload operation and sewage overflow into the river.
3.2 Heavy metal speciation in sediments
The studied heavy metal speciation was determined for 12 of the sample sites. The results indicated that the distribution of heavy metals in the sediments differed greatly (). Under certain conditions, the non-residual fractions of trace metals (i.e. the sum of F1, F2 and F3) can have harmful effects on benthic organisms [Citation26]. In this study, the order of the non-residual fractions of heavy metals was Zn > Pb > Cu > Cd > Cr > Ni. The acid-soluble fractions of Cd and Zn ranged from 3.78% to 30.20% and 5.31% to 30.74%, respectively. These results indicated that Cd and Zn had high potential bioaccessibility and migratory. The maximum proportion of F2 and F3 of Zn reached 46.99% and 25.33%, respectively. Results of the chemical fractionation analysis showed that a significant proportion of Cd (8.80–62.99%), Cr (13.80–67.93%), Cu (20.45−79.54%), Ni (14.88–43.84%), Pb (17.75–78.08%) and Zn (19.20–68.82%) were present in the bioavailable fractions of surface sediment samples collected from the Yitong River.
Figure 3. The chemical fractions of Cd, Cr, Cu, Ni, Pb and Zn in the sediment were divided into four categories: F1 (acid-soluble fraction), F2 (reducible fraction), F3 (oxidizable fraction) and F4 (residual fraction).

The strength of the bond formed between a metal and another compound determines its potential release capacity and bioavailability in aquatic environments [Citation26]. During sediment treatment (such as dredging), special attention should be paid to metals release because sediment disturbance may cause changes in redox potential and anoxic environment and change the form of metals from stable state to unstable state.
The previous study shows that when the EF values of metals exceed 1.5. the most metals were influence with anthropogenic sources [Citation8,Citation30]. The spatial distributions of the EFs calculated for each of the studied metals are displayed in Fig. S1. The average EF values showed that Zn had the highest contribution from anthropogenic sources, followed by Cu (2.42), Cd (2.15), Pb (1.74), Ni (1.66) and Cr (1.66). The maximum values of EF for Cu (9.63) and Zn (6.04) are found at site X1, which belongs to an overflow area of the drainage system in Changchun. There were some ship manufacturing factories that could discharge pollutants such as Cu and Zn into the river.
The use of metal chemical fractionation can help us better understand the sources and behavior of metals in aquatic environments and aid in the development of effective strategies for their management and control [Citation31]. The relationship between the EFs and chemical speciation is shown in . A noteworthy association was observed between the non-residual fraction and Zn’s enrichment factor (EF), with an R2 value of 0.74, indicating that anthropogenic sources such as industrial and urban activities were the primary contributors to Zn. The EF for Cu had a significant correlation with both the non-residual fraction and F4, with R2 values exceeding 0.7, revealing that Cu originated from both rock weathering and anthropogenic sources. Furthermore, the residual fractions showed significant correlations with the EFs for Cr (R2 = 0.547), Ni (R2 = 0.506) and Pb (R2 = 0.496). It is worth noting that the chemical fraction of heavy metals has an impact on their bioavailability. Thus, heavy metals with large non-residual state in the sediment may be more easily used by aquatic organisms, which would increase biotoxicity [Citation32].
3.3 Assessment of heavy metal risk and organic pollution in sediment
3.3.1 Organic pollution
The OPI results showed serious organic pollution of sediments in the YTD (0.86) and Chuanhu River (1.38). The OPI of the Jing River was grade II, which indicated mild contamination (). The YTC had an average ONI of 0.25% and maximum ONI of 0.49%, which indicated heavy contamination with organic nitrogen. In recent years, large volumes of domestic sewage in Changchun have entered the river either through runoff with rainwater or direct discharge. Because of the high nitrogen content in domestic sewage, the middle section of the catchment in the Yitong River is seriously polluted.
3.3.2 Ecological risk of heavy metals
The Index of Igeo is commonly utilized to evaluate heavy metal pollution. The results of this study indicated that the degree of heavy metal pollution was generally low (as shown in ). In most sites, the Igeo values for Cr were less than zero, suggesting that there was no contamination of sediment with Cr. For Cd, Cu and Ni, nearly all Igeo values were less than one, indicating that the river was unpolluted to moderately polluted by these metals. The maximum Igeo values for heavy metals were primarily observed in sediment collected from site C3. At this location, the Igeo values for Cr, Cu, Ni, Pb and Zn were 1.19, 1.84, 1.33, 1.51 and 1.12, respectively, implying that the sediment was moderately polluted by these metals. On average, the Igeo values followed the subsequent sequence: Zn (0.64) > Cu (0.36) > Cd (0.32) > Pb (0.03) > Ni (0.01) > Cr (−0.06).
Figure 6. Risk assessments for the metals. A rose chart for the geoaccumulation index, Igeo (a) and the risk assessment code, RAC (b). Igeo (c), RAC (b), TU (e) and Eri (f) for metals.

The RAC calculated using the BCR for F1 can be used to evaluate the metal mobility [Citation7,Citation33]. Cd (average: 16.12%, range: 3.78–30.20%) and Zn (average: 17.23%, range: 5.31–30.74%) presented high or moderate risks in most sediments (). As a result of their high availability and toxicity, both Cd and Zn have the potential to enter the food chain and pose significant threats to the ecosystem. The RACs of Ni and Pb ranged from 1% to 10% at more than 91.7% of the sites, indicating that they were of low risk and would preferentially remain bound to the solid phase of the sediment. From our results, Cd and Zn are considered high risk and should be controlled.
The TUs for individual metals were in the order of Ni (0.96) > Zn (0.57) > Cr (0.47) > Pb (0.33) > Cu (0.31) > Cd (0.05). The average contributions of the metals to the ∑TU were 35.7 ± 6.8% for Ni, 21.1 ± 5.2% for Zn, 17.5 ± 4.5% for Cr, 12.3 ± 4.3% for Pb, 11.6 ± 3.2% for Cu and 1.69 ± 2.1% for Cd. The findings indicated that the sediment toxicity was primarily attributed to Ni and Zn, with Cd having the lowest contribution among the heavy metals studied. Previous studies have indicated that heavy metals have remarkable toxicity when ∑TU is higher than four [Citation25,Citation34]. Site C3, which was located in an industrial zone in Changchun, showed higher ∑TU (5.70) than other sites. The ∑TU at the other sites were less than four, which meant they had low potential toxicity.
The values of Cd at sites D3 and J2 were 90.95 and 93.79, respectively, which indicated considerable potential ecological risk (Fig .6f). The remaining metals were found to have low risks with
values below 40. The maximum RI value was 139.29 at site C3, which exhibited low ecological risk from heavy metals. Based on the evaluations of
and RI, Cd was identified as a major contributor to the overall potential ecological risk index. This result could be explained by the high toxicity level and background value of Cd [Citation35]. Comparison of the Igeo, TU and
results revealed that Cd and Ni caused the most acute pollution in the sediment samples and posed a considerable risk ().
3.4 Human health risk assessment for sediment
The Yitong River is a typical urban river. Residents along the river are involved in water-related activities, swimming, fishing, walking, and so on, and may be directly or indirectly exposed to contaminated river sediments. Risk assessment is a crucial aspect to understand the impact of adverse human health information and key tools associated with exposure to environmental hazards. The results of the calculations (presented in ) demonstrated that heavy metals, including Cd, Cr, Cu, Ni, Pb and Zn, found in the sediments of the Yitong River and its tributaries did not pose a significant risk to the health of the local residents. The HI values for both children and adults were below 1, with values of 0.412 and 0.059, respectively. The individual HI results of residents along the river exhibited in the following order: Cr > Ni > Pb > Cu > Zn > Cd. The HI value for children was found to be 6.98 times higher than that of adults, indicating that children are more vulnerable to the effects of heavy metals in sediment than adults. The previous study indicated that the content of heavy metals in sediment of the urban section of Yitong River has an increasing trend with the increase in depth [Citation29]. When managing sediment, it is essential to pay close attention to heavy metals in order to prevent any adverse impact on human health caused by their release.
Table 1. The health risk for children and adults of heavy-metal-based hazard quotient (HQ) and the risk index (HI).
3.5 Biotoxicity assessment of the sediment
The inhibition rate of Vibrio qinghaiensis Q67 in each sample is shown in . Sediment from the mainstream of the Yitong River, particularly from the middle section, was more toxic than sediment from other rivers. The LIR in porewater from the different rivers decreased in the order YTC (20.91%) > YTD (18.89%) > XKR (14.19%) > JSR (12.95%) > CHR (11.69%) > YTU (6.04%). The LIR value was less than 20%, 20–50% and 50–100%, indicating the sample was non-toxic, slightly toxic and toxic, respectively [Citation36]. The acute toxicity of sediments in the Yitong River was generally non-toxic, and the proportion of LIR values below 20% was 75%. Vibrio qinghaiensis Q67 in porewater from site Z4 and Z5 had inhibition rate of 30.82% and 36.16%, respectively, indicating that the sediment from the middle section of the Yitong River is slightly toxic.
The lethality rates of D. magna were lower than Vibrio qinghaiensis Q67 for test sample of the Yitong River. Based on D. magna bioassay test (), the biotoxicity of the urban section of the Yitong River increased first and then decreased from the upstream to downstream, reaching the maximum at Z4 and Z5 (23%). The trend was consistent with the results of Vibrio qinghaiensis Q67. Toxicity classification of D. magna can refer to LIR, and the results showed about 7% of the samples were slightly toxic.
3.6 Multivariate statistical analyses
Principal component analysis (PCA) was utilized to identify potential sources of the heavy metals studied, with results presented in and Figure S2. The analysis revealed three principal components with eigenvalues >1, explaining approximately 69.87% of the total variance. PC1 contributed to 33.83% of the variance, while PC2 accounted for 23.05%. Cr, Cu, Ni and Pb were localized in the positive direction of PC1, while PC2 was only related to OM. The third principal component captures 12.99% of the variability, which is related to Zn. It suggested that Zn arises from different sources or that they have different migration processes in sediment.
Table 2. Factor loadings obtained from principal component (PC) analysis of the metal contents in sediments from the YTH.The factor loadings with an absolute value greater than 0.7 are highlighted in bold to signify a strong relationship between the variable and the factor.
displays the Pearson correlation coefficients between heavy metals, TN, TP, OM and the luminous inhibition rate and lethality of D. magna in the sediments. Significant positive correlations were observed between TP and TN (r = 0.73, p < 0.05), Cr and Pb (r = 0.51, p < 0.05), Ni and Pb (r = 0.49, p < 0.05) and Ni and Cu (r = 0.59, p < 0.05). Strong correlations suggest that these metals may originate from the same sources. Iron is found in a diverse array of river sediments and is considered representative of naturally occurring sources due to its limited susceptibility to human activities [Citation37]. Heavy metals showed no significant correlations with Fe concentrations and this indicates that the studied metals mainly arose from anthropogenic inputs. Sediment that accumulates heavy metals above the background value will affect aquatic and benthic organisms [Citation35,Citation38].
Figure 8. Correlation coefficients (a) and heatmap analysis (b) among heavy metals, TN, TP, OM, LIR and Li and in the river sediment (* indicates the correlation is significant at the 0.05 level [two-tailed]). Luminous inhibition rate (LIR), Lethality of D. magna (Li).
![Figure 8. Correlation coefficients (a) and heatmap analysis (b) among heavy metals, TN, TP, OM, LIR and Li and in the river sediment (* indicates the correlation is significant at the 0.05 level [two-tailed]). Luminous inhibition rate (LIR), Lethality of D. magna (Li).](/cms/asset/c3536b57-fa22-4377-8b7b-0f79a051c767/tcsb_a_2248384_f0008_oc.jpg)
The correlations were found between Zn and LIR (r = 0.50, p < 0.05) and Zn and Li (r = 0.56, p < 0.05), indicating biotoxicity in sediment may be affected by the concentration of Zn. Significant correlations for TN and TP were observed, but the correlations with heavy metals were small. As shown in , the results indicated that Fe formed a distinct group. The sediment from upstream section of the Yitong River included in the same group, and site Z4, Z5 and C5 also showed close proximities with each other. The Yitong River studied is in the old urban area, and the sewage pipe network is mainly combined. Site Z4, Z5 and C5 are located just downstream of the overflow outlet of the combined pipe network, resulting in a similar sediment pollution source. The river with higher biotoxicity of sediment is consistent with that in the overflow section. The combined sewer overflows were found to contain various substances, including nutrients, heavy metals and antibiotic resistance genes [Citation39,Citation40], which may induce higher biotoxicity in sediment of site C4 and C5 ().
The statistical analyses suggested that the sources of OM, nutrients (total nitrogen and total phosphorus) differed from the metals. With the rapid urbanization, combined sewer overflows are regarded as an important pollution source in the urban watersheds [Citation41]. Combined with the results of a pollution survey in Changchun, our results suggest that nitrogen and phosphorus pollution may come from domestic sewage in the rainy season. Confluence sewer sediment is formed in the non-rainy season and flushes out with rainfall [Citation42]. The primary sources of pollution in the combined sewer overflows were identified as organic matter, TN, TP and suspended solid discharge [Citation43,Citation44].
The primary source of Cr and Cu contamination was identified as industrial sewage, which accounted for 10% of the total concentration of both metals [Citation45,Citation46]. The polluted sites are located near two industrial zones (Laotiebei and Erdao) [Citation29]. From the end of the last century to the beginning of this century, metallurgy, papermaking, textile production, printing and dyeing were prevalent in the studied region, and the wastewater containing heavy metals was discharged into the river sediment.
4. Conclusions
In this study, we evaluated the pollution levels of heavy metals (Cd, Cr, Cu, Ni, Pb and Zn), organic matter and nutrients (TN and TP) in sediments from the Yitong River. The investigation revealed that copper, lead and zinc were predominantly present in non-residual fractions, suggesting their potential migration with changes in the anoxic environment at the water–sediment interface. Furthermore, the findings also indicated significant organic pollution in the sediments, particularly in the middle section of the Yitong River. The presence of nutrients in surface sediments from the Yitong River suggests possible contributions from combined sewer overflows, while heavy metals may originate from both natural and industrial sources.
The sediment analysis indicated slight acute biotoxicity in the middle section of the Yitong River, underscoring the importance of considering the potential negative impacts on aquatic organisms. Additionally, the results of the human health risk assessment indicated that heavy metals in the sediment posed little threat to the health of residents. However, it was observed that children may be more susceptible to the effects of heavy metals compared to adults, emphasizing the need for targeted monitoring and mitigation strategies.
To ensure ecological security and guide future river restoration projects on the Yitong River, attention should be given to the enrichment of nutrients and the speciation and mobility of heavy metals. These findings provide valuable insights for the development of effective management and remediation strategies to safeguard the ecosystem and protect human health.
Author contribution
Fujie Zhai and Xudong Deng: data collection and analysis, literature review, language services. Chao Zhang: manuscript writing, literature review, language services as well as research funding support. Baoqing Shan: Provide manuscript content and ideas.
Supplemental Material
Download MS Word (105.3 KB)Acknowledgments
We thank Gabrielle David, PhD, from Liwen Bianji (Edanz) (www.liwenbianji.cn/) for editing the English text of a draft of this manuscript.
Disclosure statement
No potential conflict of interest was reported by the author(s).
Data availability statement
The data used in this study are trustworthy and available upon reasonable request from the corresponding author
Supplementary material
Supplemental data for this article can be accessed online at https://doi.org/10.1080/26395940.2023.2248384
Additional information
Funding
References
- Zhang Y, Mao W, Li RH, et al. Distribution characteristics, risk assessment, and quantitative source apportionment of typical contaminants (HMs, N, P, and TOC) in river sediment under rapid urbanization: a study case of Shenzhen river, Pearl River Delta, China. Process SafEnviron Prot. 2022b;162:155–422. doi: 10.1016/j.psep.2022.03.032
- Fujita M, Ide Y, Sato D, et al. Heavy metal contamination of coastal lagoon sediments: Fongafale Islet, Funafuti Atoll, Tuvalu. Chemosphere. 2014;95:628–634. doi: 10.1016/j.chemosphere.2013.10.023
- Yang J, Chen L, Liu L-Z, et al. Comprehensive risk assessment of heavy metals in lake sediment from public parks in Shanghai. Ecotoxicol Environ Saf. 2014;102:129–135. doi: 10.1016/j.ecoenv.2014.01.010
- Zou Y, Wang X, Khan A, et al. Environmental remediation and application of nanoscale zero-valent iron and its composites for the removal of heavy metal ions: a review. Environ Sci Technol. 2016;50(14):7290–7304. doi: 10.1021/acs.est.6b01897
- Liu Y, Tang Y, Zhong G, et al. A comparison study on heavy metal/metalloid stabilization in Maozhou River sediment by five types of amendments. J Soils Sediments. 2019;19(12):3922–3933. doi: 10.1007/s11368-019-02310-w
- Wang F, Dong W, Zhao Z, et al. Heavy metal pollution in urban river sediment of different urban functional areas and its influence on microbial community structure. Sci Total Environ. 2021;778:146383. doi: 10.1016/j.scitotenv.2021.146383
- Liang G, Zhang B, Lin M, et al. Evaluation of heavy metal mobilization in creek sediment: influence of RAC values and ambient environmental factors. Sci Total Environ. 2017;607-608:1339–1347. doi: 10.1016/j.scitotenv.2017.06.238
- Gao XL, Chen CTA. Heavy metal pollution status in surface sediments of the coastal Bohai Bay. Water Res. 2012;46(6):1901–1911. doi: 10.1016/j.watres.2012.01.007
- Brady JP, Ayoko GA, Martens WN, et al. Temporal trends and bioavailability assessment of heavy metals in the sediments of Deception Bay. Queensland Australia Mar Pollut Bull. 2014;89(1–2):464–472. doi: 10.1016/j.marpolbul.2014.09.030
- Miranda LS, Ayoko GA, Egodawatta P, et al. Physico-chemical properties of sediments governing the bioavailability of heavy metals in urban waterways. Sci Total Environ. 2021;763:142984. doi: 10.1016/j.scitotenv.2020.142984
- Castro MF, Almeida CA, Bazan C, et al. Impact of anthropogenic activities on an urban river through a comprehensive analysis of water and sediments. Environ Sci Pollut Res. 2021;28(28):37754–37767. doi: 10.1007/s11356-021-13349-z
- Li XT, Wu PX, Delang CO, et al. Spatial-temporal variation, ecological risk, and source identification of nutrients and heavy metals in sediments in the peri-urban riverine system. Environ Sci Pollut Res. 2021;28(45):64739–64756. doi: 10.1007/s11356-021-15601-y
- Caixeta ES, Meza Bravo JV, Pereira BB. Ecotoxicological assessment of water and sediment river samples to evaluate the environmental risks of anthropogenic contamination. Chemosphere. 2022;306:135595. doi: 10.1016/j.chemosphere.2022.135595
- Zhang C, Shan B, Tang W, et al. Identifying sediment-associated toxicity in rivers affected by multiple pollutants from the contaminant bioavailability. Ecotoxicol Environ Saf. 2019;171:84–91. doi: 10.1016/j.ecoenv.2018.12.075
- Bian JM, Ma HY, Sun XQ, 2011. Analysis on water quality change and influence factors in the Yitong River basin, In: Sun D, Sung WP, editors. Applied Mechanics and Materials. Vols. 71–78, p. 2970–2973 https://doi.org/10.4028/www.scientific.net/AMM.71-78.2970
- Ji XY, Zhang WZ, Jiang MQ, et al. Black-odor water analysis and heavy metal distribution of Yitong River in Northeast China. Water Sci Technol. 2017;76(8):2051–2064. doi: 10.2166/wst.2017.372
- Zhang L, Shen T, Cheng Y, et al. Temporal and spatial variations in the bacterial community composition in lake bosten, a large, brackish lake in China. Sci Rep. 2020;10(1):304–304. doi: 10.1038/s41598-019-57238-5
- De Jonge M, Teuchies J, Meire P, et al. The impact of increased oxygen conditions on metal-contaminated sediments part I: effects on redox status, sediment geochemistry and metal bioavailability. Water Res. 2012;46(7):2205–2214. doi: 10.1016/j.watres.2012.01.052
- Arain MB, Kazi TG, Jamali MK, et al. Time saving modified BCR sequential extraction procedure for the fraction of Cd, Cr, Cu, Ni, Pb and Zn in sediment samples of polluted lake. J Hazard Mater. 2008;160(1):235–239. doi: 10.1016/j.jhazmat.2008.02.092
- Song Z, Shan B, Tang W. Evaluating the diffusive gradients in thin films technique for the prediction of metal bioaccumulation in plants grown in river sediments. J Hazard Mater. 2018b;344:360–368. doi: 10.1016/j.jhazmat.2017.10.049
- Pan Y, Wu Z, Zhou J, et al. Chemical characteristics and risk assessment of typical municipal solid waste incineration (MSWI) fly ash in China. J Hazard Mater. 2013;261:269–276. doi: 10.1016/j.jhazmat.2013.07.038
- Yu GB, Liu Y, Yu S, et al. Inconsistency and comprehensiveness of risk assessments for heavy metals in urban surface sediments. Chemosphere. 2011;85(6):1080–1087. doi: 10.1016/j.chemosphere.2011.07.039
- Song Z, Dong L, Shan B, et al. Assessment of potential bioavailability of heavy metals in the sediments of land-freshwater interfaces by diffusive gradients in thin films. Chemosphere. 2018a;191:218–225. doi: 10.1016/j.chemosphere.2017.10.048
- Maanan M, Saddik M, Maanan M, et al. Environmental and ecological risk assessment of heavy metals in sediments of nador lagoon, morocco. Ecological Indicators. 2015;48:616–626. doi: 10.1016/j.ecolind.2014.09.034
- Ji ZH, Zhang H, Zhang Y, et al. Distribution, ecological risk and source identification of heavy metals in sediments from the Baiyangdian lake. Chemosphere 237. Northern China, 2019.
- Wang M, Song G, Zhang C, et al. Chemical fractionation and risk assessment of surface sediments in luhun reservoir, Luoyang city, China. Environ Sci Pollut Res. 2020;27(28):35319–35329. doi: 10.1007/s11356-020-09512-7
- Zhang WQ, Jin X, Liu D, et al. Assessment of the sediment quality of freshwater ecosystems in eastern China based on spatial and temporal variation of nutrients. Environ Sci Pollut Res. 2017;24(23):19412–19421. doi: 10.1007/s11356-017-9532-1
- Li Z, Fu ZH, Wang SH, et al. Spatial distribution, ecological risk, and human health assessment of heavy metals in lake surface sections — a case study of Qinghai Lake, China. Environ Sci Pollut Res. 2023;30(2):5137–5149. doi: 10.1007/s11356-022-22293-5
- Jiang SX, Zhai FJ, Zhang C, et al. Speciation distribution and risk assessment of heavy metals in sediments from the Yitong River city area. Environ Sci. 2020;41:2653–2663.
- Feng H, Han XF, Zhang WG, et al. A preliminary study of heavy metal contamination in Yangtze River intertidal zone due to urbanization. Mar Pollut Bull. 2004;49(11–12):910–915. doi: 10.1016/j.marpolbul.2004.06.014
- Islam MS, Ahmed MK, Raknuzzaman M, et al. Heavy metal pollution in surface water and sediment: a preliminary assessment of an urban river in a developing country. Ecol Indic. 2015;48:282–291. doi: 10.1016/j.ecolind.2014.08.016
- Xia PH, Ma L, Sun RG, et al. Evaluation of potential ecological risk, possible sources and controlling factors of heavy metals in surface sediment of Caohai Wetland, China. Sci Total Environ. 2020;740:140231. doi: 10.1016/j.scitotenv.2020.140231
- Liu BX, Luo J, Jiang S, et al. Geochemical fractionation, bioavailability, and potential risk of heavy metals in sediments of the largest influent river into Chaohu lake, China. Environ Pollut [ Environmental pollution 290]. 2021;290:118018. doi: 10.1016/j.envpol.2021.118018
- Soliman NF, Younis AM, Elkady EM. An insight into fractionation, toxicity, mobility and source apportionment of metals in sediments from El Temsah lake, Suez Canal. Chemosphere. 2019;222:165–174. doi: 10.1016/j.chemosphere.2019.01.009
- Meng LZ, Zhao LF, Liu WT, et al. Risk assessment of bioavailable heavy metals in the water and sediments in the Yongding New River, North China. Environ Monit Assess. 2021;193(9). doi: 10.1007/s10661-021-09367-6
- Baran A, Tarnawski M, Koniarz T, et al. Content of nutrients, trace elements, and ecotoxicity of sediment cores from Rożnów reservoir (Southern Poland). Environ Geochem Health. 2019;41(6):2929–2948. doi: 10.1007/s10653-019-00363-x
- Sun X, Fan D, Liu M, et al. Source identification, geochemical normalization and influence factors of heavy metals in Yangtze River Estuary sediment. Environ Pollut. 2018;241:938–949. doi: 10.1016/j.envpol.2018.05.050
- Zhao S, Feng CH, Quan WM, et al. Role of living environments in the accumulation characteristics of heavy metals in fishes and crabs in the Yangtze River Estuary, China. Mar Pollut Bull. 2012;64(6):1163–1171. doi: 10.1016/j.marpolbul.2012.03.023
- Venditto T, Ponzelli M, Sarathy S, et al. A microsieve-based filtration process for combined sewer overflow treatment with nutrient control: modeling and experimental studies. Water Res 170. 2020;170:115328. doi: 10.1016/j.watres.2019.115328
- Zhang J, Yu D, Dian L, et al. Metagenomics insights into the profiles of antibiotic resistome in combined sewage overflows from reads to metagenome assembly genomes. J Hazard Mater. 2022a;429:128277. doi: 10.1016/j.jhazmat.2022.128277
- Mutzner L, Vermeirssen ELM, Mangold S, et al. Passive samplers to quantify micropollutants in sewer overflows: accumulation behaviour and field validation for short pollution events. Water Res. 2019;160:350–360. doi: 10.1016/j.watres.2019.04.012
- Yu D, Dian L, Hai Y, et al. Effect of rainfall characteristics on the sewer sediment, hydrograph, and pollutant discharge of combined sewer overflow. J Environ Manage. 2022;303:114268. doi: 10.1016/j.jenvman.2021.114268
- Ekhlas D, Kurisu F, Kasuga I, et al. Identification of new eligible indicator organisms for combined sewer overflow via 16S rRNA gene amplicon sequencing in Kanda River, Tokyo. J Environ Manage. 2021;284:284. doi: 10.1016/j.jenvman.2021.112059
- Schertzinger G, Itzel F, Kerstein J, et al. Accumulation pattern and possible adverse effects of organic pollutants in sediments downstream of combined sewer overflows. Sci Total Environ. 2019;675:295–304. doi: 10.1016/j.scitotenv.2019.04.094
- Iftikhar F, Liu SL, Sun YX, et al. Spatial distribution of trace elements associated with organic carbon along the Beiyun River basin, Beijing, China. Int J Of Sediment Res. 2022;37(3):335–345. doi: 10.1016/j.ijsrc.2021.10.005
- Sun YB, Zhou QX, Xie XK, et al. Spatial, sources and risk assessment of heavy metal contamination of urban soils in typical regions of Shenyang, China. J Hazard Mater. 2010;174(1–3):455–462. doi: 10.1016/j.jhazmat.2009.09.074