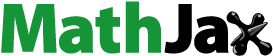
ABSTRACT
The present study analyzed soil samples from flood-prone Unions in two Coastal Upazilas of Bangladesh using Proton Induced X-Ray Emission (PIXE) techniques with Van de Graaff Accelerator for detecting heavy trace elements and Gamma spectrometry techniques. The findings indicate that while Potassium (averaging 19,62 μg/g for Sutarkhali; 21364.67 μg/g for Amtoli) and Calcium (averaging 36,923.92 μg/g for Sutarkhali; 30404.33 μg/g for Amtoli) levels were high naturally, the levels of Lead (averaging 71.8 μg/g for Sutarkhali; 171.44 μg/g for Amtoli), and Chromium (averaging 6.87 μg/g for Sutarkhali; 340.22 μg/g for Amtoli) were posing a serious risk to the inhabitants. The evaluation contamination factor (CF), pollution load index (PLI), potential ecological risk index (ERI) and health risk assessment indicate severe heavy metal pollution in both regions, with young children being particularly vulnerable to lead poisoning. Nonetheless, the radiation levels were below the safe limit set by the International Atomic Energy Agency (IAEA).
1. Introduction
Recent increasing rates of industrialization and urbanization are considered as the one of the main causes for the increase of environmental toxins observed in the globe’s environment at the present time [Citation1]. Heavy metals (HMs) are regarded as the most dangerous of these pollutants due to the fact that they cannot be degraded by biological processes and have been found to have detrimental physiological effects on living organisms even when present in minute levels [Citation2]. Due to excessive use of fertilizers and chemicals in agriculture, industrialization at the upstream waste materials are often drained into river water [Citation3]. After entering a river system, HMs have the potential to be disseminated between the aqueous system and bed sediments by leaching, absorption and infiltration, where they then aggregate in sediment and biota. Industrial waste from various sources also mixes with water, and both cases lead to HMs contamination in soil during flooding caused by sea or river water. In recent time, multiple studies have demonstrated the significance of coastal contamination with HMs all over the world [Citation3–5]. Elevated concentrations of HMs in sediment and water, can be transferred into vegetation, animals, and even humans. For this phenomenon, many scientists are interested in assessing the dispersion of HMs in various environmental components [Citation4,Citation6,Citation7]. Additionally, research has revealed flooding as a key source of HMs pollution in surrounding catchment areas [Citation8,Citation9]. Erosion, accumulation, and the reworking of sediment all play a role in the storage of sediment-associated HMs in a channel. Researchers have also found that, despite the reduction of flood conditions, HMs concentration can remain elevated [Citation10,Citation11]. This condition poses a significant risk to many coastal nations, especially Bangladesh, where flooding is a frequent and unavoidable occurrence [Citation12,Citation13].
Natural gamma radiation from terrestrial or environmental sources is also a significant concern for environmental safety as well as human health [Citation14]. Significant amounts of natural radiation are absorbed by soil and sediment, and this radiation can be transferred up the food chain, posing substantial health risks [Citation15]. However, guidelines have been published by the International Atomic Energy Agency and the Environmental Protection Agency of the United States to monitor natural radiation exposure (IAEA Safety Standards Series No. GSR Part 3 2014). Estimating the radiation-dose distribution is crucial for assessing the health risks to the population, and it can serve as a reference for measuring changes in environmental radioactivity due to anthropogenic activities [Citation16].
With a population density of 1,278 people per square kilometer, Bangladesh is highly populated [Citation17]. In recent years, there has been a rise in the number of reported cases of poisoning in Bangladesh caused by exposure to heavy metals and metalloids [Citation18]. The majority of Bangladesh’s ecosystems, including agricultural land, foods, suburban soil, and rivers, all contain high levels of harmful metals and metalloids (HMs) (M. K [Citation19–22]. There have been reports of high levels of HMs being found in a variety of agricultural goods, including rice, vegetables, fruits, and seafood [Citation23]. According to a study that was published by [Citation24], the sediments that are carried by the rivers of Bangladesh are considerably polluted with HMs. Even groundwater in the nation’s coastal region is contaminated by HMs [Citation25]. The coastline of the Bay of Bengal is subject to tidal fluctuations in water levels that can be felt up to 150 km away from the coast. The major rivers and smaller streams account for the majority of the pollution that is found in virtually all of the countries which are located around the Bay of Bengal’s coastline. In addition, sewage has a substantial impact on the coastal waterways of the Bay of Bangel, an effect that is exacerbated by the lack of economic activity in coastal states. Because of this, the rivers that are found along the shore, particularly Matamuhuri, Bakkahali, Karnophuli, Sangu, Pasur, and Rupsha, are regarded to be among the most polluted rivers in the country [Citation26–30]. Due to cheap and facile settlements, coastal businesses appear to discharge waste directly into rivers, estuaries and the sea with negligible or no treatment at all. This problem becomes severe during flood events when the soil is flooded by these river water that contain and deposit HMs in the sediment which have the capacity to transfer them to humans [Citation31].
This study therefore aims to investigate the health risks associated with terrestrial gamma radiation and HMs pollution in two particularly exposed unions along the coast of Bangladesh.
2. Description of study area
The investigation was carried out in two coastal districts of Bangladesh, Khulna and Barguna, specifically in the Sutarkhali Union at Dacope Upazila, Khulna, and the Amtoli Union at Amtoli Upazila, Barguna. These areas have been frequently flooded by sea water due to natural disasters like Sidor, Aila, and Amphan. The map of these two Unions, respectively, is shown in .
The coastline of Khulna is notoriously vulnerable to natural disasters such as cyclones and tidal surges originating in the Bay of Bengal. Due to its geographical location, Dacope Upazila is one of the locations along the coast of Khulna that is most vulnerable to floods [Citation12]. During a cyclone, the wind speed along the shore can reach 240 kmh−1, accompanied by heavy rainfall and tidal surges of up to 6–7 m [Citation32]. Since 1970, 36 cyclones have struck the country, causing over 450,000 deaths and a massive economic loss [Citation33]. The Dacope Upazila encompasses a total area of 991.56 square kilometers, of which 210.87 square kilometers are designated as river area [Citation34]. The river beds and waterways of the Upazila are progressively silting up and losing its capacity for navigational, drainage, and water retention in wet places as a result of huge sediment loads that are carried downstream from upstream by rivers. As a consequence of this, flooding takes place in the downstream shallow lowland of the study region in the Khulna District during the monsoon season when the water level of the Ganges reaches the danger level as a result of excessive rainfall upstream. It causes loss of life, livelihoods, and misery; furthermore, it damages crops, homes, and infrastructure [Citation32]. Sutarkhali Union is located at the base of Dacope Upazila, near to the Sundarbans. It is one of the most susceptible unions to the detrimental impacts of climate change [Citation35]. Sutarkhali union covers 47.55 square kilometers and has a total population of 30,430, of which 15,663 are male and 14,740 are female [Citation36].
According to the BBS report, Amtoli Upazila has a total area of 720.75 square kilometers, of which 129.81 square kilometers are riverine land. This upazila has a population of 2,70802 individuals. This area is situated in a portion of Bangladesh that is particularly prone to natural disasters due to its location on the country’s southern coast. This upazila is being situated between the coordinates of 21°51′ N and 22°18′ N, and 90°00′ E and 90°23′ E. Galachipa and Kalapara upazilas to the east; Patuakhali sadar to the north; the Bay of Bengal to the south. The Amtoli union is regarded as the commercial district of this upazila. Previous research indicated that Amtoli Upazila in the Barguna district is frequently afflicted by natural calamities including cyclones, storm surges, tidal flooding, and salt water intrusion, among others [Citation37–39]. The research area is situated in a portion of Bangladesh that is particularly prone to natural disasters due to its location on the country’s southern coast. Study conducted by [Citation31,Citation40] reveals that this area is highly contaminated by HMs and even children in this region is exposed to this HMs contamination.
3. Methods and materials
3.1. Sample collection and preparation
Twenty-one soil samples were obtained from various locations of Amtoli Union, Amtoli, Barguna and Sutarkhali Union, Dacope, Khulna, which were inundated by flooding due to the tide of different natural disasters. Twelve samples were collected from Sutarkhali Union, Dacope Upazila, Khulna, and nine samples were collected from Amtoli Union, Amtoli Upazila, Barguna. Soil samples were collected from depths ranging from 5 to 20 cm to prevent fresh soil surface and dust accumulation and guarantee the soil was appropriate for agricultural use. Considerable amounts of soil were collected from each location to ensure soil homogeneity. represents the soil sampling location for each union. All laboratory work was conducted at Atomic Energy Center Dhaka’s Experimental Facilities (AECD).
3.1.1. Preparation of samples for PIXE analysis
To find level of HMs in soil, samples of powdered soil were compressed at a force of 250 kg/cm2 using a compression machine to produce pellets (0.2 g) measuring 7 mm in diameter and 1 mm in thickness. For heavy metal detection through Van de Graaff accelerator, the PIXE facilities were upgraded by IAEA TC Project at AECD. A 170 µm of Mylar absorber has been used to save the detector from high level of X-rays. A spectroscopy amplifier and an MCA were used in data acquisition setup. Charges were collected by Faraday Cup, set behind the sample wheeler and connected through a copper spring. To establish accuracy, the measured values were compared against thick soil standards known as Soil-7 and SRM 2586, provided by NIST to make pellets of similar dimension for onward irradiation by 3 MV Van de Graaff accelerator. The density of heavy metal was determined by analyzing X-ray spectra obtained. The PIXE experiments employ a Si (Li) detector in conjunction with other circuitries utilizes MAESTRO-32 version 6.05 and GUPIX/DAN-32 software. The data acquisition process carried out using both the MAESTRO-32 PIXE program and the Guelph GUPIX PIXE program, with the interface of DAN32 software. 3.1.2 Preparation of Samples for γ-Ray Spectrometry Analysis:
The soil samples were placed in cylindrical plastic containers with a geometry of 346 cm3 each (7 cm in diameter and 9 cm in height). Shaking the samples of soil in each container by hand allowed the powders to settle uniformly. Using a digital electronic balance, the mass of the blank plastic container was determined, and then the sample was added to the container and the entire weight was determined. The sample’s net weight was calculated by deducting the mass of the vacant containers from the weight of the container containing the sample. In order to avoid the samples from coming into contact with air, the specimen containers were then carefully sealed and secured with vinyl tapes that were 1-in. thick all the way around the screw necks. Gamma measurements were conducted using an HPGe detector with an active volume of 132 cc, which was supplied by Oxford Instruments Inc. Nuclear Measurement Group (Model No. CPVDS 30–30185 and Serial No. 2604). Gamma-ray spectra were acquired, displayed, and analyzed using the commercial program EMCAPLUS version 1.012 (Supplied by Silena, Germany). To establish a reliable efficiency vs. energy curve for the HPGe gamma-ray detector, measurements were taken using known activity levels of uranium standard reference materials (RGU-1), thorium standard reference materials (RGTh-1), and potassium standard reference materials (RGK-1) provided by the IAEA, Vienna [Citation41].
Pollution assessment
In this study, contamination factors, pollution load indexes and ecological risk index were utilized to assess the extent of which HMs in the soil contribute to environmental pollution and to quantify the overall level of pollution on a specific union [Citation42,Citation43]
3.1.3. Contamination Factor (CF)
The contamination factor, often known as CF, can be calculated using the following formula. This represents the amount of metals introduced to the soil as a result of human activity [Citation44]. The CF assesses the quality of soil and identifies any harmful chemicals present. It takes into account both the soil surface metal content and the preindustrial reference level or background environment values. The CF was estimated using the following formula:
where Cmetal is the concentration of the HMs at the studied area and Cbackground indicates the background concentration for the analyzed metal. As the background value for the metal, the mean geochemical baseline values for metals with in earth’s crust was utilized [Citation43,Citation45]. The CF was divided into four categories: (i) if CF value is less than 1 represents little contamination, (ii) if CF value is between 1 and 3 suggests moderate contamination, (iii) if CF value is between the range of 3 and 6 denotes substantial contamination, and (iv) if CF has higher value than 6 denotes significant degree of contamination [Citation46]
3.1.4. Pollution load index (PLI)
The pollution load index (PLI), which was developed by Tomlinson [Citation47], was applied to each site in order to estimate the amount of the metal pollution level that was already present. PLI assists to the evaluation of the environmental quality of any particular region [Citation48,Citation49]. This was done with the help of the following equation:
Where CF obtained with the instructions given in the previous equation and n represents the total number of metals examined. The PLI gives a proper set of metrics for assessing the quality of a site [Citation50]. In general, (i) a PLI less than one is regarded as ideal, (ii) a PLI equal to one is believed to show that just the reference line amounts of contaminants are present, and (iii) a PLI more than one is believed to suggest that the site’s quality is degrading.
3.1.5. Potential ecological risk index (ERI)
The potential ecological risk index (ERI) was used to assess the comprehensive potential ecological risk of heavy metals in sediment and was initially introduced by [Citation42]. The potential ecological risk factor of a given metal is defined as
Here, the potential ecological risk of HMs is denoted by, ., the accumulating coefficient of HMs is denoted by,
, and the toxic response factor of HMs is denoted by,
. Zn, Cr, Pb, Cu, and Ni have. values of 1, 2, 5, 5, and 30, respectively, in this research (M. S [Citation18,Citation51].
3.2. Health risk assessment
Human health risk assessment is a method for evaluating the possible adverse health effects of exposure to carcinogenic and noncancerous chemicals [Citation52]. The risk evaluation method includes four stages: hazard adjustment, exposure evaluation, toxic effects (dose-response) evaluation, and risk identification using USEPA model [Citation53]. For the study of the exposures of adults and children to hazardous soil elements, the US Environmental Protection Agency’s [Citation54] and the Dutch National Institute of Public Health and the Environment’s [Citation55] health risk assessment models were used to explain recommendations or testing levels of toxins in soil samples in exposure scenarios. In this investigation, the elements Ni, Cr, Cu, Mn, Zn, Hg, and Pb were identified as potential community hazards.
3.2.1. Exposure assessment
An exposure assessment’s purpose is to provide an evaluation or estimate of the magnitude, frequency, and length of human exposed to environmental toxins. In this study, the normal daily dosage (D) of HMs received by adults and children by ingestion (EquationEquation (3)(3)
(3) ), inhalation (EquationEquation (3)
(3)
(3) ), and cutaneous absorption (EquationEquation (5)
(5)
(5) ) was determined. Due to their social and physiological differences, adults and children are separated from each other [Citation43,Citation56,Citation57].
Here, C represents the amounts of HMs in the soil samples (exposure-point concentration), including the exposure parameters used to determine the ‘sensible most severe exposure’ [Citation55], and InhR is the inhalation rate [Citation55], IngR is the ingestion rate [Citation52], ED is the exposure duration in years [Citation52], EF is the exposure frequency in days/year [Citation52], BW is the individual’s body weight in kilograms [Citation52,Citation54], CR is the contact or adsorption rate, and PEF is the particle discharge factor in m3/kg [Citation52], SA and SL are skin area exposed and skin adherence factor, respectively, [Citation52], and ABS is the dermal absorption factor [Citation58].
3.2.2. Hazard Index (HI)
The term ‘no-carcinogenic risk’ refers to the HI. It is a measure that incorporates the overall contribution that is provided by doses received via skin contact, inhalation, and ingestion consumption. The following equations (6) and (7) define a Hazard Index (HI), which is the total of hazard quotients (HQs) for all paths and similar hazardous effects. The HI can be determined by the following equation [Citation43,Citation53]:
Here, RfD is the relevant reference dosage of the analyzed elements and Di is the element’s dose. Afterwards, the ratios between the dosage of I element and the corresponding reference dose (Di/RfD) is known as the hazard quotient (HQ) or non-cancer risk. The HI is utilized to measure the risk associated with ingestion, inhalation, and dermal exposure to an element. According to the USEPA, HI ≤ 1 implies that there are no adverse health impacts, however HI > 1 suggests that harmful health effects are probable [Citation53]. Using the reference dosage values for ingestion, inhalation, and skin contact, the values of HI were calculated [Citation43].
4. Result and discussion
4.1. HMs concentration
This study’s primary purpose was to discover the levels of HMs, including Cu, Ni, Zn, Pb and Hg, and estimate their concentrations in places flooded by natural disasters such as Sidor, Aila, and Amphan. Some produced pellets of the samples analyzed and Standard Reference Materials, such as SRM 2586 and Soil-7, were irradiated with a 2.2–2.5 MeV proton beam at the 3 MV Van de Graaff Accelerator to determine the elemental content. Each sample was individually irradiated, and the x-rays emitted during the irradiation procedure were counted using a high-resolution Si (Li) detector equipped with the required electronics. The resulting x-ray spectra were analyzed using GUPIXWIN for materials irradiated by a tandem accelerator and Maestro-32 with GUPIX/DAN 32 for Van-Graaff Accelerator samples. For the accelerator, quality control (QC) checks on the measurements were also performed.
There were 12 soil samples collected from the Sutarkhali Union, whereas 9 soil samples were gathered from the Amtoli Union. It has been discovered that potassium (K), calcium (Ca), and iron (Fe) are the most prevalent components in both unions (shown in ). These phenomena can be explained as these metals are prevalent in nature, also other studies carried out in the surrounding areas showed identical findings [Citation59–61].
On the other hand, Hg and Pb are displayed at lowest concentration compared with other metals. The Ni concentration at the Sutarkhali union ranges between 213 and 611 µg/g, whereas at Amtoli union it has a relatively low concentration ranging between 163 and 312 µg/g. In contrast, the range of copper concentration at Amtoli union (ranges between 157 and 1264 µg/g) is significantly greater than that of Sutarkhali union (381 to 247 µg/g). However, the Zn concentration ranges between 158 and 461 µg/g (Sutarkhali Union) and 8 and 179 µg/g (Amtoli Union). As these elements are the result of anthropogenic activity, relative low concentration is expected (M. S [Citation59; Citation61]).
4.2. Spatial distribution
The spatial distribution of metal concentrations reveals that the largest concentrations are often found in locations adjacent to rivers (). indicates that the Sutarkhali union includes rivers that flow both east and west. In the majority of the cases, the lowest concentration is observed in the union’s central or top region. Regardless, the distribution of Ca reveals an uncommon instance in which the upper portion of the union has the largest concentration. The content and distribution of all the other metals indicate that the river adjacent soil is highly polluted.
Figure 4. Spatial variation of HMs concentrations in Sutarkhali Union, Dacope Upazila, Khulna soil samples.

As depicted in , in the case of the Amtoli Union, the river flows through a location that is nearly in the center of this union. In contrast to the Sutarkhali union, the Amtoli union has an embankment that effectively restricts the amount of water that infiltrates the union. Despite this, the riverbank has the highest concentration of the elements Fe, Cr, Ca, Mn, and K. As illustrated in , the distribution and concentration of other metals, including Pb, Zn, Ti, and Hg, indicate that human activity, such as agricultural pesticide application and transportation, is the predominant source of this contamination. Human activities such as metallurgical extraction (As, Ni, Zn, Cd, Cu, Pb), smelters (As, Cd, Pb), metallurgy, the electronic industry (which uses HMs in batteries, semiconductors, and circuitry), rolling (Ni, Cd, Pb, Hg, Se), the dyes and paint industry (Pb, Cr, As, Se, Mo, Cd, Co, Ba, Zn), and the plastics industry (As, Cr and Cu). These components were frequently detected as soil and water contaminants in the area of furniture companies and wood-processing [Citation62–65].
4.3. Pollution assessment
represents a quantitative description of CF for analyzed HMs. According to the findings of previous studies, larger values of CF indicated that human induced sources play a more significant role in the formation of pollutants, whereas lower values reflect the natural origin of components found in the soil [Citation66,Citation67]. Hence, the relatively low average CF values for Ca and Fe can be linked to their geological origin. In contrast, the relatively high average concentrations of Pb, Zn, and Cr in the studied area can be attributed to anthropogenic or other type of activities regulating their distribution. Pollutants such as Pb, Cr, and Zn are found to be extensively contaminated in both studied areas, as shown in . For Ni, however, an exception can be noted. It indicates highly contamination for only the Sutarkhali union, which is consistent with Islam et al. [Citation59], findings. Since this region is so near to the Mangrove Forest.
Table 1. Potential ecological risk index recommended limit in soil [Citation42].
Table 2. Statistical description of CF and PLI for studied HMs.
This study also found that for both of the study locations, the PLI value is greater than 1, indicating that the site quality is deteriorating and that immediate intervention is necessary to improve the situation. The high CF of metals (Cr, Ni, and Pb), as shown by the study, are the direct consequence of human activity in these coastal regions [Citation67].
The findings of ERI in the soil samples for only five different metals (Zn, Cr, Pb, Cu, and Ni) are depicted in . The ERI could not be computed because the biological toxicity factors (.) for the remaining HMs were not available. From the ERI calculation, it is possible to obtain it the HMs pollution caused by a single element, and the degree of pollution caused by each of the five HMs declined in the following order: Pb > Ni > Cr > Cu > Zn (Sutarkhali Union); Pb > Cu > Cr > Ni > Zn (Amtoli Union). According to the ERI value (), the studied regions are subject to a significant amount of lead contamination. Despite the fact that when compared to the Ni, Cu, and Cr values of other parts of Bangladesh, commonalities can be observed with these values (M. S [Citation18; Citation51]). According to the findings of this investigation, Zn has the lowest ERI value. Similar findings have been found in other research as well (M. S [Citation18; Citation51; Citation68]). The fact that both of these regions are in a very high ecological risk zone, as shown by the RI score (427.03 for Sutarkhali Union; 352.35 for Amtoli Union), is a very concerning circumstance for the people who live in these locations.
4.4. Health risk assessment
HMs can be ingested, breathed in, or come into touch with the skin, and all three of these modes of HMs exposure have been identified as having distinct doses associated with them (Safiur [Citation61]). On the other hand, the evaluation of the health risks posed by HMs in soil takes into account children and adults in a manner that is distinct, and the results are provided in .
Table 3. Values of reference dose for ingestion, inhalation and dermal contact, and the calculated values of hazard index (HI) for non-carcinogenic elements in soil sample at Sutarkhali Union.
Table 4. Values of reference dose for ingestion, inhalation and dermal contact, and the calculated values of hazard index (HI) for non-carcinogenic elements in soil sample at Amtoli Union.
Following the determination of doses for each particular metal and route of exposure, those doses were then divided by the appropriate reference dose in order to arrive at a hazard quotient (HQ) number. presents the findings that were obtained from the health risk assessment that was conducted for this study. According to the findings of this research, the hazard quotients (HQs) of most soil particles for ingestion are significantly higher than those for inhalation and dermal adsorption, with the exception of Zn at Amtoli union, where dermal absorption is higher compared to ingestion for children. In the case of children, all of the values of HQ and HI were, in general, smaller than 1, with the exception of Pb in both areas. It is also possible to deduce from the risk assessment that, in most instances, children are more susceptible to the harmful effects of pollutants in comparison to adults. The results of this most recent investigation are consistent with the findings of earlier research that has been described in the relevant body of scholarly work [Citation69,Citation70]. Due to the fact that children spend more time playing outside, they are at a greater risk of ingesting HMs than adults because the dirt in which they play may contain metals [Citation71]. As mentioned, the HI value of the majority of contaminants is less than 1, lead is an exception. This also indicates that lead concentrations have the potential to cause adverse effects in children. According to research, high levels of pb in the soil can generate significant levels of pb contamination in children’s blood due to their hand-to-mouth activity [Citation72–74]. Lead (Pb) poisoning is the cause of more than one million deaths and 24.4 million disability-adjusted life years each year around the globe [Citation75]. A community cohort study that was conducted by [Citation76] found that lead is responsible for roughly 412,000 deaths that occur annually in the United States. Lead contamination has a deleterious effect on every system in the body, but it is especially linked to dysfunctions in the organs that control the cardiovascular, renal, and neurological systems [Citation77]. On the other hand, this exposure is frequently modulated by social factors like income [Citation78]. It has been known for a very long time [Citation75,Citation79,Citation80] that populations with low incomes are subjected to an unfairly high level of disproportionate environmental lead exposure.
According to the findings of this investigation, the areas that were investigated contain a significant level of contamination, with potential health risk specially for children. Additional research is necessary in order to correctly identify the source of the pollution and the level of contamination. In many parts of Bangladesh, farming is the primary means of subsistence for the local population. Through the process of biotransfer, agricultural activities conducted on these soils have the potential to cause severe harm to human health [Citation81–83].
4.5. Terrestrial radioactivity measurements and their effects
The radionuclides 226Ra was identified by the decay products through the γ-ray spectrum having peaks of energies 351.92 keV, 295.21 keV and 241.98 keV from the decay of 214Pb and 609.31 keV, 1120.3 keV, 1238.1 keV and 1764.5 keV peaks formed in the decay of 2l4Bi. The 232Th radioactivity was determined by γ-ray counts of products formed via the decay of the 212Pb (238.63 keV), 228AC (911.07 keV and 969.11 keV) and 208Tl (583.14 keV and 2614.7 keV) radionuclides. The radionuclide 40K was identified by its single γ -line at an energy of 1460.8 keV.
Within the scope of this study, artificial fallout was also analyzed and considered. However, there was no peak for 137Cs at the energy of 661.66 keV in the spectrum. This is likely due to the fact that the radioactivity of this radionuclide in the samples that were analyzed was lower than the detection threshold. Similar investigation carried out in the surrounding area came to the same conclusions [Citation84]. It may be mentioned that the background counts were subtracted from the total counts obtained during measurements with the samples. Gamma-ray spectra were acquired, displayed, and analyzed using the commercial program EMCAPLUS version 1.012 (Supplied by Silena, Germany).
4.5.1. Sutarkhali union
The measured specific radioactivity values (in Bq kg-1) in the soil samples collected from different parts of Sutarkhali Union, Dacope, Khulna are given in . It is found that the specific radioactivity values of 238 U, 232Th and 40K in the soil samples varied within the range: 16.50 ± 1.22–60.40 ± 7.21 (mean: 33.88 ± 4.37), 23.19 ± 2.43–55.55 ± 4.12 (mean: 42.08 ± 3.97) and 167.45 ± 23.42–592.50 ± 27.31 (433.67 ± 25.52) Bq.kg-1, respectively.
Table 5. Specific radioactivity measured in the soil samples Sutarkhali Union, Dacope, Khulna.
4.5.2. Amtoli union
shows the measured radioactivity values (Bq kg-1) and associated uncertainties (± lσ) for nine samples taken from different regions of Amtoli Union, Amtoli, Borguna. The specific radioactivity values of 238 U, 232Th, and 40K in soil samples from Amtoli varied between 18.46 ± 3.43 and 33.76 ± 1.85 (mean: 31.15 ± 3.69), 26.57 ± 3.09 and 51.94 ± 3.87 (mean: 42.49 ± 3.48), and 135.72 ± 21.69 and 369.46 and 33.42 (mean: 225.74 ± 27.96) Bq kg-1, respectively.
Table 6. Specific radioactivity measured in soil samples of Amtoli Union, Amtoli, Barguna.
The Raeq, external γ-absorbed dose (D) and annual effective dose (AED) in Sutarkhali Union, Dacope Upazila () the corresponding values are found to vary within 87.34 ± 8.40–181.31 ± 15.01 Bq/kg (mean: 117.54 ± 11.83 Bq/kg), 42.59 ± 3.97–86.34 ± 6.97 nGy/h (mean: 59.28 ± 5.49 nGy/h) and 86.98 ± 8.10–176.31 ± 14.22 µSv/yr (mean: 121.05 ± 11.21 µSv/yr), respectively.
Table 7. Radium equivalent activity, external effective dose and annual effective dose at Dacope Upazila, Khulna.
For Amtoli soils, the corresponding values () were found to vary within 77.75 ± 9.96–132.65 ± 12.45 Bq/kg (mean: 107.71 ± 10.63 Bq/kg), 37.35 ± 4.72–61.90 ± 5.86 nGy/h (mean: 49.53 ± 4.98 nGy/h) and 76.28 ± 9.64–126.41 ± 11.96 µSv/yr (mean: 101.14 ± 10.17 µSv/yr). The external gamma absorbed dose (D) and annual effective dose (AED) also correspond these Unions regarding the comparisons of the values.
Table 8. Radium equivalent activity, external effective dose and annual effective dose at Amtoli Union, Amtoli, Barguna.
The mean values of Raeq, D and AED are, respectively, 112.62 Bq/kg, 54.4 nGy/yr and 111.1 µSv/yr. All these values are within world average and they do not exceed the dose recommended value by UNSCEAR-2000.
4.5.3. External hazard index, internal hazard index, and excess lifetime cancer risk
External hazard index (Hex), internal hazard index (Hin) and excess lifetime cancer risk (ELCR) in Sutarkhali Union, Dacope, the corresponding values were 0.24 ± 0.02–0.50 ± 0.04 (mean: 0.343 ± 0.033), 0.30 ± 0.04–0.64 ± 0.06 (mean: 0.416 ± 0.05) and 304.4 10−6 − 617.1
10−6 (mean: 423.7
10−6). For Amtoli Upazila, the corresponding values are 0.22 ± 0.03–0.37 ± 0.03 (mean: 0.29 ± 0.03), 0.25 ± 0.04–0.43 ± 0.04 (mean: 0.36 ± 0.04) and 267.0
10−6 − 442.4
10−6 (mean: 354.0
10−6). The minimum mean of Hex is found at Amtoli Union, Amtoli, whereas the value is slightly high at Sutarkhali Union at Dacope, Khulna. However, the Hex and Hin values for the measured areas do not exceed the limit (<1) recommended by UNSCEAR-2000.
The estimated values for the Hex, Hin, and the surplus lifetime cancer risk fall below the safe thresholds. The findings indicate that the Hex and Hin values for the soil samples are less than one, indicating that the radiation dose is below the IAEA-recommended limit of 1 mSvy-1. Compared to global averages and suggested values, the radiation hazard parameter values from this study are not excessively high and are therefore unlikely to provide additional radiological health concerns to the residents of the studied localities.
This study analyzed the increased cancer risks throughout a lifetime. Due to a lack of trustworthy, standardized mortality and morbidity data for the population in the investigated area, the health risk posed by the presence of HMs and radioactivity cannot be assessed in this study. Notably, the scope of this investigation was limited to the measurement of background radiation levels.
5. Conclusion
This study investigated the degree of soil contamination with HMs including lead, mercury, manganese, nickel, cadmium, zinc, and copper due to a lack of information concerning the baseline level of soil pollution in the area under study. The soil samples of Sutarkhali Union, Dacope and Amtoli Union, Amtoli showed some different values. The samples from Sutarkhali, Dacope, Khulna were collected from the land which were inundate for longer period of time than the samples from Amtoli Union, Amtoli, Barguna. Results also show that the concentration of HMs and radioactivity concentration is higher at Sutarkhali than Amtoli. It indicates that all the affected areas were not contaminated similarly by the natural disaster may be the cause of inundate period. Due to the higher occurrence of Hg and Pd in samples from long-term inundated areas, prompt research is required to determine the soil’s status following any cyclone-caused destruction in the area.
The concentration of radioactivity not exceeded the limit of radioactivity suggested by IAEA. But it may find that the activity is more where the HMs concentration is also more. This study found a correlation between the HMs presence in the soil samples and terrestrial radioactivity of those samples. So, further study is required to investigate the correlation between the HMs and radioactivity of the non-biotic samples [Citation85–88].
Author conurbations
Conceptualization, SAKA, and MuMM; methodology, SAKA, HGA, MHRS MoMM and MuMM; writing – original draft preparation, SAKA, HGA, MHRS, MuMM, JF, AFH, MJA., H.A., AAA. and JAA.; writing – review and editing, SAKA, HGA, MHRS MoMM, JF, AFH, MJA., H.A., AAA. and JAA. All authors have read and agreed to the published version of the manuscript.
Ethical approval
This article does not include any studies with human participants or animals performed by any of the authors.
Acknowledgments
The authors express gratitude to AECD (Atomic Energy Center, Dhaka; Bangladesh) Experimental Facilities for laboratory support and to the editors and potential reviewers.
Disclosure statement
No potential conflict of interest was reported by the author(s).
Data availability statement
The data that support the findings of this study are available on request from the corresponding author.
References
- Jafari A, Ghaderpoori M, Kamarehi B, et al. Soil pollution evaluation and health risk assessment of heavy metals around Douroud cement factory, Iran. Environ Earth Sci. 2019;78(8):1–436. doi: 10.1007/S12665-019-8220-5
- Kabata-Pendias A. Trace elements in soils and Plants. 4th. 2010. p. pp. 1–520. doi: 10.1201/B10158.
- Nour HE, Alshehri F, Sahour H, et al. Evaluation of sediment and water quality of Ismailia Canal for heavy metal contamination, Eastern Nile Delta, Egypt. Reg Stud Mar Sci. 2022;56:102714. doi: 10.1016/J.RSMA.2022.102714
- Alharbi T, Al-Kahtany K, Nour HE, et al. Contamination and health risk assessment of arsenic and chromium in coastal sediments of Al-Khobar area, Arabian Gulf, Saudi Arabia. Mar Pollut Bull. 2022;185:114255. doi: 10.1016/J.MARPOLBUL.2022.114255
- Al-Kahtany K, Nour HE, Giacobbe S, et al. Heavy metal pollution in surface sediments and human health assessment in southern Al-Khobar coast, Saudi Arabia. Mar Pollut Bull. 2023;187:114508. doi: 10.1016/J.MARPOLBUL.2022.114508
- Alkan N, Alkan A, Akbaş U, et al. Metal pollution assessment in sediments of the Southeastern black sea coast of Turkey. Soil And Sediment Contamination: An Int J. 2014;24(3):290–305. doi: 10.1080/15320383.2015.950723
- Gu C, Liu Y, Liu D, et al. Distribution and ecological assessment of heavy metals in irrigation channel sediments in a typical rural area of south China. Ecol Eng. 2016;90:466–472. doi: 10.1016/J.ECOLENG.2016.01.054
- Ciszewski D, Grygar TM. A review of flood-related storage and remobilization of heavy metal pollutants in river systems. Water Air Soil Pollut. 2016;227(7):1–19. doi: 10.1007/S11270-016-2934-8
- Matys Grygar T, Elznicová J, Bábek O, et al. Obtaining isochrones from pollution signals in a fluvial sediment record: a case study in a uranium-polluted floodplain of the Ploučnice river, Czech Republic. Appl Geochem. 2014;48:1–15. doi: 10.1016/J.APGEOCHEM.2014.06.021
- Coynel A, Schäfer J, Blanc G, et al. Scenario of particulate trace metal and metalloid transport during a major flood event inferred from transient geochemical signals. Appl Geochem. 2007;22(4):821–836. doi: 10.1016/J.APGEOCHEM.2006.10.004
- Resongles E, Casiot C, Freydier R, et al. Variation of dissolved and particulate metal(loid) (as, Cd, Pb, Sb, Tl, Zn) concentrations under varying discharge during a Mediterranean flood in a former mining watershed, the Gardon river (France). J Geochem Explor. 2015;158:132–142. doi: 10.1016/J.GEXPLO.2015.07.010
- Musfike Meraz M, Riad Hossain M, Sultana R, et al. Flood prediction and vulnerability assessment at the south-western region of Bangladesh. Environ Monit Assess. 2023;195(6):1–20. doi: 10.1007/S10661-023-11418-Z
- Rahman R, Salehin M. Flood risks and reduction approaches in Bangladesh. 2013;pp. 65–90. doi: 10.1007/978-4-431-54252-0_4
- Audu KE, Adeniji SE, Obidah JS. Bioremediation of toxic metals in mining site of Zamfara metropolis using resident bacteria (Pantoea agglomerans): A optimization approach. Heliyon. 2020;6(8):e04704. doi: 10.1016/J.HELIYON.2020.E04704
- Munir MT, Federighi M. Control of foodborne biological hazards by ionizing radiations. Foods. 2020;9(7):878. doi: 10.3390/FOODS9070878
- Gaur A, Adholeya A. Prospects of arbuscular mycorrhizal fungi in phytoremediation of heavy metal contaminated soils. Curr Sci. 2004;86(4): 528–534. Available from: http://www.jstor.org/stable/24107905
- Rakib MRJ, Rahman MA, Onyena AP, et al. A comprehensive review of heavy metal pollution in the coastal areas of Bangladesh: abundance, bioaccumulation, health implications, and challenges. Environ Sci Pollut Res. 2022;29(45):67532–67558. doi: 10.1007/S11356-022-22122-9
- Islam MM, Karim MR, Zheng X, et al. Heavy metal and metalloid pollution of soil, water and foods in bangladesh: a critical review. Int J Environ Res Public Health. 2018;15(12):2825. doi: 10.3390/IJERPH15122825
- Ahmed MK, Baki MA, Islam MS, et al. Human health risk assessment of heavy metals in tropical fish and shellfish collected from the river Buriganga, Bangladesh. Environ Sci Pollut Res. 2015;22(20):15880–15890. doi: 10.1007/S11356-015-4813-Z
- Hasan MF, Nur-E-Alam M, Salam MA, et al. Health risk and water quality assessment of surface water in an Urban river of Bangladesh. Sustainability. 2021;13(12):6832. doi: 10.3390/SU13126832
- Kibria G, Hossain MM, Mallick D, et al. Monitoring of metal pollution in waterways across Bangladesh and ecological and public health implications of pollution. Chemosphere. 2016;165:1–9. doi: 10.1016/J.CHEMOSPHERE.2016.08.121
- Proshad R, Kormoker T, Islam MS, et al. Potential health risk of heavy metals via consumption of rice and vegetables grown in the industrial areas of Bangladesh. Hum Ecol Risk Assess: Int J. 2019;26(4):921–943. doi: 10.1080/10807039.2018.1546114
- Shafiuddin Ahmed AS, Sultana S, Habib A, et al. Bioaccumulation of heavy metals in some commercially important fishes from a tropical river estuary suggests higher potential health risk in children than adults. PLoS One. 2019;14(10):e0219336. doi: 10.1371/JOURNAL.PONE.0219336
- Jolly YN, Rakib MRJ, Kumar R, et al. Deciphering the source of heavy metals in industrially affected river sediment of Shitalakshya river, Bangladesh, and potential ecological and health implications. J Hazard Mater Adv. 2023;10:100268. doi: 10.1016/J.HAZADV.2023.100268
- Khan MS, Paul SK. Groundwater quality assessment and health issues in coastal zone of Bangladesh. J Hazard Mater Adv. 2023a;10:100278. doi: 10.1016/J.HAZADV.2023.100278
- Proshad R, Kormoker T, Islam S. Distribution, source identification, ecological and health risks of heavy metals in surface sediments of the Rupsa river, Bangladesh. Toxin Rev. 2019;40(1):77–101. doi: 10.1080/15569543.2018.1564143
- Sabbir W, Rahman MZ, Hasan MM, et al. Assessment of heavy metals in river water, sediment and fish mussel in rupsha river under Khulna district, Bangladesh. Int J Exp Agric. 2018;8:1–5. doi: 10.9734/ajfar/2020/v6i230091
- Shil SC, Irin A, Tusher TR, et al. Heavy metal contamination in water and sediments of Passur river near the Sundarbans mangrove of Bangladesh. J Environ Sci Nat Res. 2017;10(1):15–19. doi: 10.3329/jesnr.v10i1.34688
- Siddique MAM, Aktar M. Heavy metals in salt marsh sediments of Porteresia bed along the Karnafully river coast, Chittagong. Soil Water Res. 2012;7(3):117–123. doi: 10.17221/7/2012-SWR
- Uddin MR, Hossain MM, Akter S, et al. Assessment of some physicochemical parameters and determining the corrosive characteristics of the Karnaphuli estuarine water, Chittagong, Bangladesh. Water Sci. 2020;34(1):164–180. doi: 10.1080/11104929.2020.1803662
- Khaliq MA, Javed MT, Hussain S, et al. Assessment of heavy metal accumulation and health risks in okra (Abelmoschus Esculentus L.) and spinach (Spinacia Oleracea L.) fertigated with wastwater. Int J Food Contam. 2022;9(1):1–11. doi: 10.1186/S40550-022-00097-2
- MoL. (2011). Land Zoning report of Dacope Upazila of Khulna district, study of detailed coastal land Zoning with two pilot districts of plain land project.
- United Nations (UN). Cyclone Aila: joint UN multi-sector assessment and response framework. New York, NY, USA; 2010.
- BBS (Bangladesh Bureau of Statistics). District Statistics 2011 Khulna. Agargoan, Dhaka, Bangladesh: Ministry of Planning; 2013.
- Roy T, Matiul Islam M, Sarwar Jahan M, et al. Adaptive strategies of coastal people in response to climate change: experiences from two villages of Dacope Upazila in Bangladesh. Environ Ecosyst Sci. 2022;6(1):17–28. doi: 10.26480/ees.01.2022.17.28
- BBS (Bangladesh Bureau of Statistics). District Statistics 2011 Barguna. Agargoan, Dhaka, Bangladesh: Ministry of Planning. 2013.
- Ali A. Climate change impacts and adaptation assessment in Bangladesh. Clim Res. 1999;12(2–3):109–116. doi: 10.3354/CR012109
- Singh OP, Ali Khan TM, Murty TS, et al. Sea level changes along Bangladesh coast in relation to the southern oscillation phenomenon. Mar Geod. 2010;24(1):65–72. doi: 10.1080/01490410120192
- World Bank. Rural Development Unit, South Asia Region. Bangladesh: Climate change and sustainable development; 2000. ( Report No. 21104-BD) .
- Khan MS, Paul SK. Groundwater quality assessment and health issues in coastal zone of Bangladesh. J Hazard Mater Adv. 2023b;10:100278. doi: 10.1016/J.HAZADV.2023.100278
- International Atomic Energy Agency (IAEA) (1987). Radiometric reference materials; RGU-1, RGTh-1 and RGK-1. IAEA Report No. RL/148, Vienna, Austria.
- Hakanson L. An ecological risk index for aquatic pollution control.A sedimentological approach. Water Res. 1980;14(8):975–1001. doi: 10.1016/0043-1354(80)90143-8
- Rahman MS, Kumar P, Ullah M, et al. Elemental analysis in surface soil and dust of roadside academic institutions in Dhaka city, Bangladesh and their impact on human health. Environ Chemi Ecotoxicol. 2021;3:197–208. doi: 10.1016/J.ENCECO.2021.06.001
- Ahmed F, Fakhruddin ANM, Imam MDT, et al. Spatial distribution and source identification of heavy metal pollution in roadside surface soil: a study of Dhaka Aricha highway, Bangladesh. Ecological Processes. 2016;5(1):1–16. doi: 10.1186/S13717-016-0045-5
- Taylor S.R., and McLennan S.M. The continental crust: its composition and evolution: An examination of the geochemical record preserved in sedimentary rocks. United States: Blackwell, Oxford; 1985. p.1–312.
- Mmolawa KB, Likuku AS, Gaboutloeloe GK. Assessment of heavy metal pollution in soils along major roadside areas in Botswana. Afr J Environ Sci Tech. 2011;5(3):186–196.
- Tomlinson DL, Wilson JG, Harris CR, et al. Problems in the assessment of heavy-metal levels in estuaries and the formation of a pollution index. Helgoländer Meeresuntersuchungen. 1980;33(1–4):566–575. doi: 10.1007/BF02414780
- Aradpour S, Noori R, Vesali Naseh MR, et al. Alarming carcinogenic and non-carcinogenic risk of heavy metals in Sabalan dam reservoir, Northwest of Iran. Environ Pollut Bioavailabil. 2021;33(1):278–291. doi: 10.1080/26395940.2021.1978868
- Wang J, Hu Y. Translocation and accumulation of heavy metals from the rhizoshphere soil to the medicinal plant (Paeonia Lactiflora Pall.) grown in Bozhou, Anhui Province, China. Environ Pollut Bioavailabil. 2023;35(1): doi: 10.1080/26395940.2023.2223768
- Addo MA, Darko EO, Gordon C, et al. Heavy metal concentrations in road deposited dust at Ketu-south district, Ghana. Int J Sci Technol. 2012;2(1):28–39.
- Kabir MH, Islam MS, Hoq ME, et al. Appraisal of heavy metal contamination in sediments of the Shitalakhya river in Bangladesh using pollution indices, geo-spatial, and multivariate statistical analysis. Arabian J Geosci. 2020;13(21):1–13. doi: 10.1007/S12517-020-06072-5
- USEPA. Guidance on cumulative risk assessment of pesticide chemicals that have a common mechanism of toxicity. Washington, DC: United States Environmental Protection Agency; 2002.
- USEPA. Risk assessment guidance for superfund, In: human health evaluation manual (Part a), interim Final. Washington, DC: United States Environmental Protection Agency;1989. (no. EPA/540/1-89/002).
- USEPA. Soil screening Guidance: technical background document. Washington, DC: United States Environmental Protection Agency; 1996. (no. EPA/540/R95/128).
- Van den Berg M, Sinnige TL, Tysklind M, et al. Individual PCBs as predictors for concentrations of non and mono-ortho PCBs in human milk. Environ Sci Pollut Res. 1995;2(2):73–82. doi: 10.1007/BF02986720
- Zheng N, Liu J, Wang Q, et al. Health risk assessment of heavy metal exposure to street dust in the zinc smelting district, Northeast of China. Sci Total Environ. 2010;408(4):726–733. doi: 10.1016/J.SCITOTENV.2009.10.075
- Zhou L, Liu G, Shen M, et al. Characteristics and health risk assessment of heavy metals in indoor dust from different functional areas in Hefei, China. Environ Pollut. 2019;251:839–849. doi: 10.1016/J.ENVPOL.2019.05.058
- Ferreira-Baptista L, De Miguel E. Geochemistry and risk assessment of street dust in Luanda, Angola: A tropical urban environment. Atmos Environ. 2005;39(25):4501–4512. doi: 10.1016/J.ATMOSENV.2005.03.026
- Islam MM, Akther SM, Hossain MF, et al. Spatial distribution and ecological risk assessment of potentially toxic metals in the Sundarbans mangrove soils of Bangladesh. Sci Rep. 2022;12(1):1–14. doi: 10.1038/s41598-022-13609-z
- Kumar A, Ramanathan AL. Speciation of selected trace metals (Fe, mn, Cu and Zn) with depth in the sediments of Sundarban mangroves: India and Bangladesh. J Soils Sediments. 2015;15(12):2476–2486. doi: 10.1007/S11368-015-1257-5
- Rahman MS, Hossain MB, Babu SMOF, et al. Source of metal contamination in sediment, their ecological risk, and phytoremediation ability of the studied mangrove plants in ship breaking area, Bangladesh. Mar Pollut Bull. 2019;141:137–146. doi: 10.1016/J.MARPOLBUL.2019.02.032
- European Commission. (2013). In-Depth Report, Soil Contamination: Impacts on Human Health.
- Liang J, Chen C, Song X, et al. Assessment of heavy metal pollution in soil and plants from dunhua sewage irrigation area. Int J Electrochem Sci. 2011;6(11):5314–5324. doi: 10.1016/S1452-3981(23)18409-4
- Soil Screening Guidance: User’s Guide. US EPA. 2 ed. (Washington, DC: United States Environmental Protection Agency; 1996. p. 39.
- Wuana RA, Okieimen FE, Montuelle B, et al. Heavy metals in contaminated soils: a review of sources, chemistry, risks and best available strategies for remediation. Int Scholarly Res Netw ISRN Ecol. 2011;2011:20. doi: 10.5402/2011/402647
- Kabata-Pendias A. Trace elements in soils and plants. Trace Elements In Soils And Plants. 2000. doi: 10.1201/9781420039900
- Rahmanian M, Safari Y. Contamination factor and pollution load index to estimate source apportionment of selected heavy metals in soils around a cement factory, SW Iran. Arc Agron Soil Sci. 2020;68(7):903–913. doi: 10.1080/03650340.2020.1861252
- Mohammad Ali M, Lokman Ali M, Saiful Islam M, et al. Assessment of toxic metals in water and sediment of Pasur river in Bangladesh. 2018. 10.2166/wst.2018.016.
- Hossain MS, Ahmed MK, Liyana E, et al. A case study on metal contamination in water and sediment near a coal thermal power plant on the eastern coast of Bangladesh. Environments. 2021;8(10):108. doi: https://doi.org/10.3390/ENVIRONMENTS8100108
- Rinklebe J, Antoniadis V, Shaheen SM, et al. Health risk assessment of potentially toxic elements in soils along the Central Elbe river, Germany. Environ Int. 2019;126:76–88. doi: 10.1016/J.ENVINT.2019.02.011
- Wuana RA, Okieimen FE. Heavy metals in contaminated soils: a review of sources, chemistry, risks and best available strategies for remediation. ISRN Ecology. 2011;2011:1–20. doi: 10.5402/2011/402647
- Carrizales L, Razo I, Tellez-Herna´ndez J, et al. Exposure to arsenic and lead of children living near a copper-smelter in San Luis Potosi, Mexico: Importance of soil contamination for exposure of children. Environ Res. 2006;101(1):1–10. doi: 10.1016/j.envres.2005.07.010
- Lake LM, Basta NT, Barker DJ. Modifying effect of soil properties on bio-accessibility of as and Pb from human ingestion of contaminated soil. Geosciences. 2021;11(3):126. doi: 10.3390/GEOSCIENCES11030126
- Mielke HW, Gonzales CR, Powell ET, et al. The concurrent decline of soil lead and children’s blood lead in New Orleans. Proc Natl Acad Sci USA. 2019;116(44):22058–22064. doi: 10.1073/pnas.1906092116
- Egendorf SP, Mielke HW, Castorena-Gonzalez JA, et al. Soil lead (Pb) in New Orleans: a spatiotemporal and racial analysis. Int J Environ Res Public Health. 2021;18(3):1314. doi: 10.3390/IJERPH18031314
- Lanphear BP, Rauch S, Auinger P, et al. Low-level lead exposure and mortality in US adults: a population-based cohort study. Lancet Public Health. 2018;3(4):e177–e184. doi: 10.1016/S2468-2667(18)30025-2
- Abadin H, Ashizawa A, Llados F, et al. Toxicological profile for lead. Atlanta, Georgia: U.S. Department of Health and Human Services; 2007.
- Sampson RJ, Winter AS. The racial ecology of lead poisoning: toxic inequality in chicago neighborhoods, 1995-2013. Du Bois Rev: Soc Sci Res On Race. 2016;13(2):261–283. doi: 10.1017/S1742058X16000151
- Leech TGJ, Adams EA, Weathers TD, et al. Inequitable chronic lead exposure: a dual legacy of social and environmental injustice. Family And Community Health. 2016;39(3):315–336. doi: 10.1097/FCH.0000000000000106
- Whitehead LS, Buchanan SD. Childhood lead poisoning: a perpetual environmental justice issue? J Public Health Manag Pract. 2019;25(1):S115–S120. doi: 10.1097/PHH.0000000000000891
- Alengebawy A, Abdelkhalek ST, Qureshi SR, et al. Heavy metals and pesticides toxicity in agricultural soil and plants: ecological risks and human health implications. Toxics. 2021;9(3):42. doi: 10.3390/TOXICS9030042
- Arao T, Ishikawa S, Murakami M, et al. Heavy metal contamination of agricultural soil and countermeasures in Japan. Paddy Water Environ. 2010;8(3):247–257. doi: 10.1007/S10333-010-0205-7
- Mao C, Song Y, Chen L, et al. Human health risks of heavy metals in paddy rice based on transfer characteristics of heavy metals from soil to rice. Catena. 2019;175:339–348. doi: 10.1016/J.CATENA.2018.12.029
- Biswas KP, Hossain S, Deb N, et al. Assessment of the levels of pollution and of their risks by radioactivity and Trace metals on Marine Edible fish and Crustaceans at the Bay of Bengal (Chattogram, Bangladesh). Environments. 2021;8(2):13. doi: 10.3390/ENVIRONMENTS8020013
- Islam MS, Hossain MB, Matin A, et al. Assessment of heavy metal pollution, distribution and source apportionment in the sediment from Feni river estuary, Bangladesh. Chemosphere. 2018;202:25–32. doi: 10.1016/J.CHEMOSPHERE.2018.03.077
- Islam MS, Ismail Z, Jamal MH, et al. Heavy metals from different land use soil in the capital of ancient Pundranagar, Bangladesh: a preliminary study for ecological risk assessment. Chem Ecol. 2022;18(8):720–743. doi: 10.1080/02757540.2022.2100360
- Nour HE, Alshehri F, Sahour H, et al. Assessment of heavy metal contamination and health risk in the coastal sediments of Suez Bay, Gulf of Suez, Egypt. J Afr Earth Sci. 2022;195:104663. doi: 10.1016/J.JAFREARSCI.2022.104663
- Safiur Rahman M, Khan MDH, Jolly YN, et al. Assessing risk to human health for heavy metal contamination through street dust in the Southeast Asian megacity: Dhaka, Bangladesh. Sci Total Environ. 2019;660:1610–1622. doi: 10.1016/J.SCITOTENV.2018.12.425