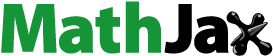
ABSTRACT
Due to rapid industrialization and urbanization, large quantities of wastes are released into the rivers of Kombolcha city. The vegetables grown on the polluted sites can take up potentially toxic trace metals at concentrations, which are toxic to human health. The current study determined the amount of potentially toxic trace metals in soil and vegetable samples grown around polluted river and assessed the potential health risks to consumers. Samples were collected in June 2020, digested using the wet digestion method and analyzed quantitatively using inductively coupled plasma optical emission spectrometer (ICP-OES). The concentrations of selected trace metals in soil samples were much lower than the recommended limit; however, the levels of nickel, lead and cadmium in vegetable samples were above the international guideline values. The result also indicated that the average target hazard quotient of lead, nickel and cadmium in most studied areas were higher than the limit. The target hazard quotients of these potentially toxic trace metals were higher in lettuce vegetables especially in children consumer. Thus, frequent consumption of lettuce may cause a health risk in children.
1. Introduction
Environmental contamination by the trace metals and related food protection are the main worldwide concern at the present time. Trace metals can cause a serious health inference to all existing things in common and humans in exacting stipulation raised in concentration on top of body necessities (Altarawneh, Citation2021; Gupta & Gupta, Citation1998). There exists enough literature indicating that the demand and use of vegetables are considerably raising everywhere around the world as it comprises significant part of the human diet and nourishment (Fonge et al., Citation2021). Kachenko and Singh (Citation2019) have explained that profitable and housing vegetable cultivating are frequently situated in town areas and are subjected to anthropogenic pollution from different sources, mainly from contaminated irrigation water, use of fertilizers and pesticides and industrial activities (Hamid et al., Citation2020). Pollution of irrigation soil by trace metals is recognizable and that can be the main source of trace metals transfer to vegetables and lastly might be the major route of human exposure (Ahmad & Goni, Citation2010). The concentrations of trace metals changes in different vegetables, which may be recognized to a gap absorption ability of vegetables for different trace metals (Singh et al., Citation2010).
In developing countries like Ethiopia, trace metal contamination of the environment is a worldwide phenomenon that has attracted a great deal of attention. Because urban rivers are receiving contaminants from municipal wastes, industrial effluents, different engine fuels, garages disposals, constructions and car washes. Especially, vegetables are able to accumulate trace metals in their edible and non-edible parts. Food safety issues and potential health risks make this as one of the most serious environmental concerns (Odoh & Kolawole, Citation2011).
Trace metals could easily be absorbed and accumulated in the eatable portions of vegetables at elevated amounts and sufficient to cause trace metal toxicity problems to living things. Excess amount of trace metals further than maximum permissible level (MPL) direct to a number of diseases, such as cardiovascular disease, kidney disease, nervous disease, bone disease, lung disease and other numerous healthiness disarrays (Temesgen & Seyoum, Citation2017). The former study revealed that the vegetables grown on the Borkena River banks north east of Ethiopia had higher concentration of trace metals (Awol & Adugna, Citation2021; Yihenew & Suwanarit, Citation2007). Therefore, there is a pressing need to monitor the levels of trace metals in Borkrna river catchment so as to protect the community from using unsafe vegetables (Damtew et al., Citation2019). The vegetables grown on the banks of Borkena river (Borkena upper, Leyole, Worka & Borkena lower) include Swiss chard (Beta vulgaris L. var. cicla), lettuce (Lactuca sativa L.), and others. Thus, the goal of this study was to determine the concentrations of the selected trace metals like Zn, Cd, Cr, Pb, Cu, Fe, Ni and Mn in soil and vegetable (Lettuce & Red beet) samples taken from Borkena river farmlands, which are found in Kombolcha city in South Wollo zone of Amhara region, North-eastern Ethiopia. Furthermore, it was intended to describe the level of pollution and the daily ingestion of toxic trace metals by investigating the transfer factor (TF) and hazard quotient (HQ).
2. Materials and methods
2.1. Description of the study area
Kombolcha city is found in Amhara regional state in the South Wollo zone and located about 380 km from north east of Addis Ababa and 20 km from Dessie town. The study was conducted in Borkena river, which drains Kombolcha city. It is geographically located in the North East of Amhara region Ethiopia between 11°4ʹ59.74”N &11°4ʹ44.14”N latitude and 39°43ʹ57.48”E and 39°39ʹ31.26”E Longitude. Based on the 2020 Kombolcha city meteorological branch station report, the mean annual rainfall of the city is 1,030 mm, and the average annual, monthly temperature ranges from 24°C in January to 28°C in August. The climate is described by two different seasonal weather conditions.
The major rainy season is summer, which extends from June to September and there is also some humidity or wet to the area in the winter season from February to April. The rest five months are dry season (Demlie & Wohnlich, Citation2006). The landform of the study area includes rising and falling mounts, with relatively high plateaus and plain with an elevation range of 1842 meter and 1915 meter above sea level. Based on the central statistical agency (CSA) of Ethiopia report in 2007, Kombolcha has an estimated total population of 85,367 of whom 41,968 are men and 43,399 are women; 58,667 are urban inhabitants living in city of Kombolcha, the rest of the population is living around Kombolcha city (CSA, Citation2007). The Borkena river basin begins from the highlands of South Wollo Zone of the Amhara region and enlarges about 300 km to the lowland of the Afar region. The Borkena river basin includes three hydrological sub-basins: upper (Dessie town), middle (Kombolcha city) and lower (Cheffa village) sub-basins.
The Borkena river is the tributary of the Awash river. The river receives industrial effluents, municipal discharges, etc., from the surrounding catchment area (Zinabu, Citation2011). The city is gifted with suitable situations for developing urban agriculture through the application of irrigation using the accessible water sources. To this end, several inhabitants in the city are growing vegetables such as tomato, cabbage, onion, lettuce, Swiss chard, potato, pepper, etc., and perennial crops including coffee and Chat (Catha edulis). Industrial activities are especially one of the major economic sources in the city, and farming is the main living of the city and bucolic areas. Afforestation and grassland are common in the highlands of the catchments. Infertile land is, however apparent in these highlands of catchments mainly due to overgrazing and deforestation on the side of the hill lands (Zinabu, Citation2011).
To study the concentration of trace metals on the Borkena river, Leyole river, and Worka river, soil and vegetable samples were collected in season and cross-sectional study design was used. Initially, sampling site survey was made to select a suitable sampling area based on the irrigation site, waste disposal points, the source of pollutants, and the types of vegetables growing and identification of the irrigated farmlands. The sampling points of the study area or site were selected according to the nearby pollution sources, population, density, factories, farming activities, and other potential sources of contaminants. Representative soil and vegetable samples were collected from four different sampling sites ().
Figure 1. Geographical map of the study area (Borkena upper, Leyole, Worka and Borkena lower irrigated farm land).

Site1: Borkena Upper river receives (effluent-free water of Borkena river)
Site 2: Loyele river receives sewages from the following four industries; leather industry,
steel processing industry, meat processing industry, and textile industry
Site 3: Worka river receives sewages and effluents from a brewery industry
Site 4: Borkena Lower river receives sewages from all industries and other pollution sources of the city.
2.2. Materials and reagents
The chemicals and reagents were used concentrated nitric acid (HNO3, 69%), concentrated hydrochloric acid (HCl, 37%), hydrogen peroxide (H2O2), tap water & deionized water. An inductively coupled plasma optical emission spectrometer, ICP-OES, (Arcos FHS2, Germany) was used for the determination of trace metals in soil and vegetable samples.
2.3. Collection of soil and vegetable samples
2.3.1. Soil samples
The soil samples were collected from the study site in June 2020 (). About 150 g of soil samples were randomly collected in each of the four sites at a depth of 0–20 cm where Lettuce & Swiss chard vegetables were grown (Poggio et al., Citation2008). Then after, the samples were placed in a sterilized/washed polyethylene bag and transported to Debre Berhan University chemistry department laboratory for the pretreatment. The soil samples were air dried at room temperature for two weeks. After that the soil samples were oven dried at 60°C for 24 h before being ground into a fine powder using mortar and pestle. The samples were passed through 75 μm sieve mesh. Then the sieved powder soil samples were stored in polyethylene bags for further analyses.
2.3.2. Vegetable samples
The vegetable samples were collected from the aforementioned sites in June 2020, where the soil samples were collected. The fresh vegetable samples of lettuce and Swiss chard (100 g) were collected separately with a clean polyethylene bags from the agricultural farm land. The vegetable samples were safely packed and transported to the department of chemistry laboratory of Debre Berhan University for processing and analysis. Initially the vegetable samples were washed with tap water, and then washed with de-ionized water to remove unwanted dust particles. Subsequently, the vegetable samples were cut and chopped into small pieces by using plastic knife in order to facilitate drying. Then, the samples were heated in an oven at 80°C to constant weights. Finally, the dried samples were ground into powder by using mortar and pestle and stored in desiccators until digestion.
2.4. Digestion and instrumental analyses
2.4.1. Digestion of soil samples
2.5 g soil samples were transferred into a volumetric flask before being mixed with 10 mL of aquaregia, which consisted of HCl: HNO3 (3:1). The mixture was then digested on a hot plate at 95°C for 1 h and was allowed to cool to room temperature. The sample was then diluted to 50 ml using de ionized water and was left to settle overnight (Mackay et al., Citation2013). The supernatant was filtered through Whatman No. 42 filter paper and (<0.45 µm) Millipore filter paper, (Merck Millipore, graphite furnace atomic Darmstadt, Germany) prior to analysis by ICP-OES (Inductive Coupled Plasma Optical Emission Spectroscopy).
2.4.2. Digestion of vegetable sample
A 0.5 g of homogenized and powdered vegetable samples were placed in a borosilicate digestion flask to which 10 mL of acid mixture containing HNO3—HCl-H2O2 (8:1:1, v/v/v) ratio were added. The mixture was heated at 120°C over 3 h on block digester. After digestion was completed, the clear and colorless solution was filtered out into 100 mL volumetric flask. Each digestion tube was rinsed with distilled water to collect any possible residue, and added to the volumetric flask and finally made up to volume with de-ionized water. All the dilute samples were stored in 100 mL plastic bottles (high-density polyethylene) until analysis. Each vegetable sample was digested and analyzed in triplicate to confirm precision of the results. The blank solution was prepared by taking a mixture of 8 mL HNO3, 1 mL HCl and 1 mL H2O2 and treating similarly as that of the sample (Street, Citation2008). The trace metal concentrations were analyzed by Inductive Coupled Plasma Optical Emission Spectroscopy in Debrezeyit Horticoop Agricultural Research Center Chemistry Laboratory, Debre Zeit, Ethiopia.
2.5. Transfer factor and health risk assessment
The transfer factor expresses the bioavailability of a metal at a particular position on a species of plant (Khan et al., Citation2009). It is calculated as the ratio of trace metal concentration in the edible part of vegetables to the metal concentration in soil.
Where; TF stands for transfer factor or daily intake of metal, [metal]vegetable is the total metal concentration in vegetable; [metal]soil is the concentration of metal in soil where the vegetable was grown.
Daily intake of metal (DIM) is the exposure to the population defined as the mass of a substance per unit body weight per unit time average over a long period of time. Daily intake through vegetable consumption was calculated using the formula:
where, Conc. of metal is the concentration of a trace metal in the vegetable (Milligrams per kilogram); Daily food intake is the daily intake of vegetables for children and adults and average body weight in kg. The mean daily intake of vegetable for children and adults were found to be 0.232 kg/day and 0.345 kg/day, respectively (Wang et al., Citation2005) whereas the mean children and adults body weights were believed to be 32.7 kg and 55.9 kg, respectively (Khan et al., Citation2009).
2.5.1. Hazard quotient (HQ)
Hazard quotient has been computed as the ratio of the average daily dose of a chemical to the dose level below which there will not be any significant risk.
Where; DIM is the daily intake of the metal; RfDo is the oral reference dose (milligrams per kilogram per day) is an estimation of the daily exposure to which human population is likely to be exposed without any significant harmful effects during a lifetime. Hazard index (HI) approach is used to assess the overall potential health effects posed by more than one trace metal based on the EPA’s Guidelines for Health Risk Assessment of Chemical Mixtures. The oral reference dose (RfD) is an estimate of a daily oral exposure of the human population, which does not cause deleterious effects during a lifetime, generally used in USEPA’s non-cancer health assessments (USEPA, Citation2008). The oral reference dose (RfD) values for Fe, Mn, Zn, Cu, Cr, Ni, Pb, and Cd are 0.02, 0.7, 0.14, 0.3, 0.04, 1.5, 0.0035 and 0.001 mg/kg-day, respectively (USEPA (U.S. Environmental Protection Agency), Citation2002, USEPA (Citation2004, Citation2005, USEPA, Citation2008).
2.5.2. Hazard index
Hazard index is computed as the sum of the Hazard quotients due to individual trace metal, as illustrated in the following equation (USEPA, Citation1986).
Where; HQi is the target hazard quotient of an individual metal
2.6. Quality control systems
All the glassware used were carefully washed with detergent, soaked in 10% HNO3 for one day and clean with de-ionized water. All the reagents used were analytical grade. All measurements were performed using inductively coupled plasma optical emission spectrometer (ICP-OES) optimized with small volume and 32 linear CCD detectors the wavelength range between 130 and 770 nm. Simultaneously, the analyses nebulizer was set into the argon gas field plasma and the energy excitation proceeds in the single spectra and the energy is expressed in intensity that is directly proportion to concentration. The concentration is calculated on the linear graph of the standard concentration and the corresponding intensities. All the measuring conditions, like plasma power, gas flows, torch positions and measuring time are configured according to the standard calibration and standardization of the spectra, but standardization is daily and it is a quick procedure for correcting measuring intensities so that the correct concentration are obtained using the original calibration curve. So as to verify and appraise the accuracy of the method used, certified reference material was employed. Blank analyses were carried out to check interference from the laboratory. To certify the analytical method, detection limit, instrumental detection limit, limit of quantification analyses were achieved.
2.6.1. Method detection limit
Method detection limit is described as the lowest concentration of analyte that can be calculated. Method detection limit (MDL) was then calculated according to the equation indicated below:
Where, YB represents blank mean and SD standard deviation of the bank.
2.6.2. Method validation
In order to make certain that the accuracy of the results obtained with the method, the spike recoveries from the eight trace metals were accomplished on the samples. The test recoveries for the soil and vegetable samples were accomplished in triplicates.
2.6.3. Recovery of metals
To calculate the percent recovery of the spiked sample the following equation was used.
The analytical method for the samples of vegetable was certified by using spiking experiments, in which known amounts of mixed standard solutions having 10 mg/kg were added into 0.2 g fractions of the vegetable samples. As well as the concentration of soil sample certified for the trace elements Fe, Mn, Zn, Cu, Cr, Ni, Pb and Cd was studied as the method illustrated for soil analysis. The spiking experiment performing the addition of known volumes of standard solutions containing the trace metals into the soil sample and use similar methods. Consequently, the spiked and the percentage recoveries trace metals were determined ().
Table 1. Unspiked sample, spiked sample results (mg/kg) and percentage recoveries of trace metals (Fe, Mn, Zn, Cu, Cr, Ni, Pb and Cd)
2.7. Statistical analysis
All the investigations of the samples were performed in triplicates as well as the mean, standard deviation and range values for each trace metals were calculated in Microsoft Excel and presented in tables. The concentrations of trace metals in the analyzed soil and vegetable samples were compared with the suggested standard of WHO and FAO.
3. Result and discussion
3.1. Concentration of trace metals in soil samples
The concentration of trace metals in soil samples of Borkena river is summarized in . The data indicated from the table that the concentration of iron (Fe) was 618.87 mg/kg, 0.14 mg/kg, 0.24 mg/kg and 0.18 mg/kg in the study area of Borkena lower, Leyole, Worka and Borkena upper river soils, respectively. The maximum amount of iron might be resulting from its abundance in nature as well as effluents from the industries in the city. The concentration of manganese (Mn) was in the range of 0.44 mg/kg—48 mg/kg. The concentration of Mn obtained in soil samples of the present study were found much lower than (2000 mg/kg) previous reported by Mahmood and Malik (Citation2014). The relative high manganese merits the high accessibility of Mn for the plant absorbed in the sampling of vegetable farmland. The amount of zinc (Zn) concentration in study site was in the range of 0.45 mg/kg—3.18 mg/kg. But the zinc concentration in this study is significantly lower to the standard guideline (Ewers, Citation1991). The relatively elevated concentration of Zn in the study area might come from the use of different agricultural activities, such as pesticides, herbicides and fertilizers (Luo et al., Citation2007). The concentration of copper (Cu) ranged between 0.06 and 1.03 mg/kg. Generally, the amount of Cu in soil reported in this study was lower than the tolerable levels (Mekonnen et al., Citation2014).The level of nickel in the Borkena lower site is 1.83 mg/kg which is higher than Leyole, Worka and Borkena upper site, but lower than the permissible set by Europeans (50 mg/kg) and Chinese (40 mg/kg) standard. The concentration of nickel that is shown in this study was lower than the previously reported by (Miller & Miller, Citation2010).
Table 2. The mean concentration of trace metals (mg/kg dry weight) in Soil samples
The content of chromium (Cr) in the study area was 1.42, 0.42, 0.34 and 0.38 mg/kg in Borkena lower site, Leyole site, Worka site, and the Borkena upper site, respectively. The level of chromium in this study was found to be below the safe limits set by various organizations 100 mg/kg (Ewers (Citation1991) and 150 mg/kg (European Union (EU), Citation2002). The result of lead (Pb) in the study site ranged between 0.98 mg/kg and 4.68 mg/kg. Consequently, the amount of lead in the present study indicated that the concentrations of trace metals in the soil samples were lower than the result obtained in Tigray, Northern Ethiopia (5 mg/kg; Gebrekidan et al., Citation2013). The result of cadmium in the present study was higher than the result obtained in Akaki, Addis Ababa, Ethiopia (1.6 mg/kg; Weldegebriel et al. (Citation2012); but it was lower than the permitted level (Ewers, Citation1991).
3.2. Concentration of trace metals in vegetables samples
Toxic trace metals entered into the environment and lead to a bioaccumulation, especially by eating fruits and vegetables (Weldegebriel et al., Citation2012).This may result excess accumulation of trace metals in the human organs. The average trace metal content from vegetables from Borkena river catchment is presented in . The concentration of Pb in both lettuce and Swiss chard samples in all the study areas were higher than the maximum limit (FAO/WHO (Citation2001), 0.3 mg/kg). The average concentration of lead (Pb) in this study ranged between 0.59—0.73 mg/kg, which is largely lower than those previously reported in Tigray, Ethiopia (1.55–5.85 mg/kg; Abraha et al., Citation2013) and Addis Ababa, Ethiopia (0.25–1.71 mg/kg; Rahlenbeck et al., Citation1999). The sources of Pb in the study area might be due to the discharge of effluents from the different industries, vehicles as well as the continuous use of fertilizers, pesticides and herbicides to the farmland of vegetables (Gezahegn et al., Citation2017). The content of iron in lettuce and Swiss chard vegetables was higher from all sampling sites because of its natural abundance; but more iron concentration was recorded in Swiss chard than lettuce vegetable. The concentration of manganese in Swiss chard (1.03 mg/kg) was greater than lettuce (0.4 mg/kg). The current result of manganese (Mn) was lower than those previously reported by Gebrekidan et al. (Citation2013).
Table 3. Average trace metals contents (mg/kg) in vegetables from Borkena River catchment
The present study revealed that the concentration of Zn in lettuce ranged from 0.25 to 0.37 mg/kg and in Swiss chard from 0.10 to 0.30 mg/kg, which were lower than the vegetables reported in Bahir Dar, Ethiopia (2.2–4.2 mg/kg; Gebregziabher & Tesfaye, Citation2014). The concentration of nickel (Ni) in this study was less than the result obtained in North Tigray, Ethiopia (0.63 mg/kg in lettuce, 0.68 mg/kg in Swiss chard; Gebrekidan et al., Citation2013). The level of Cu in all the tested vegetables was significantly lower than those (15.26 mg/kg—32.11 mg/kg) in India and (1.5–5 mg/kg) in Bangladesh previously reported by (Gupta et al., Citation2012), and (Islam et al., Citation2015), respectively. The result of Cd (0.11–0.16 mg/kg) content in the studied vegetables was higher than the mean (0.01–0.15 mg/kg) previously reported by Radwan and Salama (Citation2006) in vegetables from Alexandria city, Egypt, and is relatively lower than the previously reported in Dhaka, Bangladesh (2.05–2.91 mg/kg; Ahmad & Goni, Citation2010) and Gejius, China (0.12–0.92 mg/kg; Li et al., Citation2014). As indicated in the present study, the level of chromium within the tested vegetables were much lower than the concentration of chromium (0.8 mg/kg–4.21 mg/kg) those previously reported in Western Cape Province, South Africa (Malan et al., Citation2015).
3.3. Transfer factor of trace metals from soil to vegetables
The amount of trace metals taken up by plants was calculated to determine the transfer factor (TF) of trace metals from soil to edible vegetable. Transfer factor is a significant measurement for the pollution assessment of soils with the highest level of trace metals. The ratio >1 means higher accumulation of metals in plant parts than soil (Barman et al., Citation2000). The result of trace metal transfer factor depends on various factors, such as the nature of metals, characteristics of the soil, type of vegetable, etc. (Fonge et al., Citation2021). The transfer factor of different trace metals from soil to vegetable is one of the main criteria of human revelation to metals using the food chain.
In all the studied lettuce and Swiss chard vegetables from the four sites, iron (Fe) had the highest transfer factor (17.94) at Borkena upper river. This shows that the trace metal might originate from natural sources and available for uptake and has a low retention rate of the metal in soil (European Union (EU), Citation2002). Manganese (Mn) had the lowest transfer factor (0.006) in the tested samples. It might be bonded to the more soil and turn into the soil composition. This signifies that the maximum concentration of the trace metal from the soil was absorbed by the plant tissue. The overall transfer pattern for trace metals in the tested vegetables was iron > copper > chromium> lead > nickel > cadmium > zinc > manganese ().
Table 4. The transfer factors of trace metals in Lettuce and Swiss chard vegetables grown by Borkena River and its tributaries farm land
Generally, in the tested vegetables the transfer factor value of toxic trace metals was lower than the other (essential) metals in all the sampling area except Borkena lower site. But the transfer factor values of lead (0.63) and cadmium (0.53) were above the maximum permissible limit by (FAO/WHO, Citation2001). Therefore, in order to reduce the risk of human health people should avoid eating the vegetables in lead or cadmium contaminated areas. In the present study, the transfer factor of Cd (0.06–0.52) was higher than those (0.021–0.083) in Haryana, India previously reported by Garg et al. (Citation2014) and lower than (0.4–0.73) in Tigray North Ethiopia stated by (Abraha et al., Citation2013). While the transfer factor of Pb (0.15–0.63) which was greater than (0.001–0.27) in Romania by Roba et al. (Citation2015) and slightly lower than those (0.17–0.78) in Addis Ababa, Ethiopia reported by Yirgalem et al. (Citation2012).
3.5. Assessment of health risk
As the result indicated that lead (Pb) in lettuce and Swiss chard vegetables from all sampling area in both children and adults was higher than the oral reference doses (RfD) recommended by the USEPA (U.S. Environmental Protection Agency; Citation2002), (USEPA (U.S. Environmental Protection Agency), Citation2005), (USEPA, Citation2008); whereas nickel (Ni) and cadmium (Cd) in both lettuce and Swiss chard vegetables from the same site in children and adults were closest to the oral reference doses (RfD) recommended by FAO/WHO (Citation2001).The maximum ingestions of trace metals (potential toxic elements) were from the use of both lettuce and Swiss chard for only children. As a result the consumption of lettuce and Swiss chard in all the sampling site can causes a serious health risks. The average nutritional intake of iron (Fe), manganese (Mn), zinc (Zn), and copper (Cu) in all the studied area were under the suggested oral reference doses (RfD) for both children and adults via the consumption of vegetable in present study.
3.6. Hazard quotient (HQ)
The HQ value of lead, nickel and cadmium were normally more than one and signifying that the local society consuming these vegetables could experience health risk. Studies indicated that nickel, lead and cadmium pollution in vegetables would pose a health risk to consumers (Jolly et al., Citation2013a). The present study showed that the average daily intake values of these trace metals (Pb, Ni and Cd) were greater than the RfD and thus, the HQ exceeded the safe value of one. The HQ values of iron, manganese, zinc, copper and chromium were less than one in both lettuce and Swiss chard vegetables for children and adult (). Therefore, it may cause minimum health effect. The descending order of the mean HQ values for children and adults were lead > nickel > cadmium >copper >manganese > iron > zinc > chromium.
Table 5. The mean hazard quotient values of trace metals by eating of lettuce and Swiss chard vegetables in the study area
4.6. Hazard index
The hazard index values in both lettuce and Swiss chard vegetables were an increasing rate with HI (3.657) and (3.739) because of the use of lettuce and Swiss chard vegetables, respectively (). In the same way, the maximum transfer of HI values was due to the consumption of lettuce vegetables that accounted by Pb (40%) subsequently nickel and cadmium that accounts below 35% in both children and adults. Overall, about 55.3% (in children) and 45% (in adult) contribution to the health index (HI) were reported by Swiss chard intake, while in lettuce consumption accounted 53.4% (in children) and 46.6% (in adults). Trace metals (nickel, lead and cadmium) were the major contributors to the cumulative health index (HI).These trace metals were transferred in the range of 23% to 59.7% in each sampling site from both vegetables. The ingestion of these trace metals contaminated lettuce and Swiss chard vegetables by the local population, which is greater than the tolerable limit and may pose a health risk.
Table 6. The higher, the lower and the percentage hazard index within the tested vegetables in each sampling site
4. Conclusion
The result of this study indicated that the amounts of nickel, cadmium and lead were found to be an increasing rate in each sampling site as compared to the standard recommended values for irrigation. However, except lead the data of soil and vegetable investigation showed that, trace metals such as iron, manganese, zinc, copper, chromium, nickel, and cadmium were not severe problems on the studied site. Except lead in soil and lead and cadmium in vegetables, all the concentration of trace metals were below the acceptable limit of FAO/WHO and USEPA guideline values. Furthermore, the transfers of trace metals from soil to lettuce vegetable were (nickel > chromium > lead), (iron > copper > manganese), (iron > copper > chromium) and (iron> copper > lead) in Borkena lower, Leyole, Worka and Borkena upper site, respectively, whereas from the soil to Swiss chard vegetable were (nickel > chromium > lead), (iron > chromium > copper), and (iron > copper> chromium). From tested vegetables, the daily intake of trace metals due to the consumption of lettuce and Swiss chard vegetables were found under the highest acceptable daily intake suggested for each metal. Similarly, the target hazard quotients of trace metals indicated that the values were higher than the standard for nickel, lead, and cadmium due to the consumption of Swiss chard in each sampling area. Only on Borkena lower site, about 65% of the hazard index was accounted due to the consumption of lettuce vegetable. While 51.3% and 52% of the hazard index were recorded as a result of the consumption of lettuce and Swiss chard vegetables in worka and Borkena upper sites, respectively. Thus, periodic monitoring of trace metals in vegetables and other environmental media is very important to identity the pollution sources and eliminates or reduce exposure of plants (and humans) to high levels of these contaminants.
Authors’ contributions
All authors contributed at different stages of this study. YAW designed the study, collected and analyzed samples and interpreted the data. He also wrote the draft manuscript. ABK involved in the design of the study, supervised the progress, provide comments on the manuscript, and prepared answers to reviewers’ comments.
Availability of data and materials
The data set and materials used in this manuscript are available and can be shared whenever necessary. The data was generated by the author from the field sample collection, processing and laboratory analysis.
Consent for publication
Not applicable.
Ethics approval and consent to participate
Not applicable.
Acknowledgements
The first author would like to thank Debre Berhan University for financial support.
Disclosure statement
No potential conflict of interest was reported by the author(s).
Additional information
Funding
Notes on contributors
Alemnew Berhanu Kassegne
Alemnew Berhanu Kassegne is an environmental analytical chemist and holds a Bachelor's degree in chemistry, Bahir Dar University, Ethiopia, and Master of Science in inorganic chemistry and Doctor of Philosophy in environmental science, both from Addis Ababa University, Ethiopia. Now, he is an assistant professor of environmental science at Debre Berhan University, Ethiopia. From 2005 to 2009 he served as pre-college mentor, at Bole preparatory School and since September 2009 he moved to Debre Berhan University. Over the years he has taught a number of undergraduate and post-graduate environmental chemistry courses. So far he advised more than 10 MSc students and more than 20 BSc students. His research areas include water quality assessment, persistent organic pollutants (OCPs, PCBs and PBDEs), potentially toxic trace metals, solid and liquid waste management. In these areas, he has done extensive research works and published 8 peer-reviewed papers in different international reputable journals. The current study assessed the level of potentially toxic elements in irrigation water, soil and vegetable samples grown around polluted river and potential health risks to consumers at Kombolcha town, Ethiopia. It helps to understand the current state of pollution in the study area and provides precautionary measures taken by the respective stakeholders.
References
- Abraha, G., Yirgaalem, W., Amanual, H., & Bruggen, B. V. D. (2013). Toxicological assessment of trace metals accumulated in vegetables and fruits grown in GinfelRiver near Sheba Tannery. Ecotoxicology and environmental safety, 95, 171-178.
- Ahmad, J. U., & Goni, M. A. (2010). Trace metal contamination in water, soil, and vegetables of the industrial areas in Dhaka. Environmental monitoring and assessment, 166(1), 347–357.
- Altarawneh, R. M. (2021). Levels of selected heavy metals (Pb, Ni, Cd, and Cr) in various widely consumed fruits and vegetables in Jordan. International Journal of Environmental Analytical Chemistry, 101(7), 1026–12. https://doi.org/10.1080/03067319.2019.1675653
- Awol, M. J., & Adugna, A. T. (2021). Assessing the industrial effluent effect on irrigation water quality and farm soil near Kombolcha Town, Ethiopia. Journal of Chemistry, 2021, 1–9. https://doi.org/10.1155/2021/5586213
- Barman, S. C., Sahu, R. K., Bhargava, S. K., & Chatterjee, C. (2000). Distribution of trace metals in wheat, mustard and weed grains irrigated with industrial effluents. Bulletin of Environmental Contamination and Toxicology, 64(4), 489–496. https://doi.org/10.1007/s001280000030.
- Central statistical agency (CSA). 2007. Population and housing census of Ethiopia: Administrative report. Springer, Cham.
- Damtew, A. A., Ketema, A. A., & Behailu, B. M. 2019. Assessment of industrial effluent pollution on borkena river, kombolcha, Ethiopia. International conference on advances of science and technology, Springer, 325–333.
- Demlie, M., & Wohnlich, S. (2006). Soil and groundwater pollution of an urban catchment by trace metals: Case study of the addis ababa region. Environmental geology, 51(3), 421–431.
- European Union (EU). 2002. Trace metals in wastes, European commission on environment (http://ec.europa.eu/environment/waste/studies/pdf trace metal report.
- Ewers, U. (1991). Standards, guidelines and legislative regulations concerning metals and their compounds. In E. Merian (Ed.), Metals and their compounds in the environment: Occurrence, analysis and biological relevance. Metals and their compounds in the environment, 760–710.
- FAO/WHO. (2001). Food additives and contaminants, joint codex alimentarius commission, FAO/WHO food standards programme.
- Fonge, B. A., Larissa, M. T., Egbe, A. M., Afanga, Y. A., Fru, N. G., & Ngole-Jeme, V. M. (2021). An assessment of heavy metal exposure risk associated with consumption of cabbage and carrot grown in a tropical Savannah region. Sustainable Environment, 7(1), 1909860. https://doi.org/10.1080/27658511.2021.1909860
- Garg, V. K., Yadav, P., Mor, S., Singh, B., & Pulhani, V. (2014). Trace metals bio-concentration from soil to vegetables and assessment of health risk caused by their ingestion. Biological trace element research, 157(3), 256–265. https://doi.org/10.1007/s12011-014-9892-z
- Gebregziabher, B., & Tesfaye, S. (2014). Assessment of levels of lead, cadmium, copper and zinc contamination in selected edible vegetables. International Journal of Innovation and Applied Studies, 7(1), 78. http://www.ijias.issr-journals.org/
- Gebrekidan, A., Weldegebriel, Y., Hadera, A., & Bruggen, B. V. D. (2013). Toxicological assessment of trace metals accumulated in vegetables and fruits grown in Ginfelriver near Sheba Tannery, Tigray, and Northern Ethiopia. Ecotoxicol Environ Safe.
- Gezahegn, W. W., Srinivasulu, A., Aruna, B., Banerjee, S., Sudarshan, M., Narayana, P. L., & Rao, A. (2017). Study of heavy metals accumulation in leafy vegetables of Ethiopia. IOSR J. Environ Sci Toxicol Food Technol, 11(5), 57–68. https://doi.org/10.9790/2402-1105015768
- Gupta, U. C., & Gupta, S. C. (1998). Trace element toxicity relationship to crop production and livestock and human health: Implications for management commu. Soil Sci. Plant Anal, 29(11–14), 1491–1522. https://doi.org/10.1080/00103629809370045
- Gupta, S., Satpati, S., Nayek, S., & Garai, D. (2012). Effect of wastewater Irrigation on vegetables in relation to bioaccumulation of trace metals and biochemical changes. Environ. Monit, 165(1), 169–177.https://doi.org/10.1007/s10661-009-0936-3
- Hamid, A., Wasim, A., Azfar, A., Amjad, R., & Nazir, R. (2020). Monitoring and health risk assessment of selected trace metals in wheat rice and soil samples. Food Science and Technology, 40(4), 917–923. https://doi.org/10.1590/fst.23319
- Islam, S., Ahmed, K., & Al-Mamun, H. (2015). Metal speciation in soil and health risk due to vegetables consumption in Bangladesh. Environmental monitoring and assessment, 187(5), 1–15. https://doi.org/10.1007/s10661-015-4533-3
- Jolly, Y., Akter, S., Kabir, J., & Islam, A. (2013a). Health risk assessment of trace metals via dietary intake of vegetables collected from an area selected for introducing a nuclear power plant. Res J Phys Appl Sci, 2(4), 43–51.
- Kachenko, A. G., & Singh, B. (2019). Trace metals contamination of home grown vegetables near metal smelter in NSW supper soil. In 3rd Australian New Zealand Soils Conference (pp. 5–9).
- Khan, S., Farooq, R., Shahbaz, S., Khan, M. A., & Sadique, M. (2009). Health risk assessment of trace metals for population via consumption of vegetables. World Appl Sci J, 6(12), 1602–1606.
- Li, Y., Wang, H., Wang, H., Yin, F., Yang, X., & Hu, Y. (2014). Trace metal pollution in vegetables grown in the vicinity of a multi metal mining area in Gejiu, China: Total concentrations, speciation analysis, and health risk. Environmental Science and Pollution Research, 21(21), 12569–12582, https://doi.org/10.1007/s11356-014-3188-x
- Luo, W., Lu, Y., Giesy, J. P., Wang, T., Shi, Y., Wang, G., & Xing, Y. (2007). Effects of land use on concentrations of metals in surface soils and ecological risk around guanting reservoir, China. Environmental Geochemistry and Health, 29(6), 459–471. https://doi.org/10.1007/s10653-007-9115-z
- Mackay, A. K., Taylor, M. P., Munksgaard, N. C., Hudson-Edwards, K. A., & Burn-Nunes, L. (2013). Identification of environmental lead sources and pathways in a mining and smelting town: Mount Isa, Australia. Environmental Pollution, 180, 304–311.
- Mahmood, & Malik. (2014). human health risk assessment of trace metals via consumption of contaminated vegetables collected from different irrigation sources in Lahore, Pakistan. Arabian Journal of Chemistry (2014), 7(1), 91–99. https://doi.org/10.1016/j.arabjc.2013.07.002
- Malan, M., Müller, F., Cyster, L., Raitt, L., & Aalbers, J. (2015). Trace metals in the irrigation water, soils and vegetables in the Philippi horticultural area in the western cape province of South Africa. https://doi.org/10.1007/s10661-014-4085-y
- Mekonnen, K. N., Ambushe, A. A., Chandravanshi, B. S., & Redi-Abshiro, M. (2014). Assessment of potentially toxic elements in Swiss chard and sediments of Akaki River, Ethiopia. Toxicological and Environmental Chemistry, 96(10), 1501–1515. https://doi.org/10.1080/02772248.2015.1025788
- Miller, J. N., & Miller, J. C. (2010). Statistics and chemo metrics for analytical chemistry (6th ed.). Pearson.
- Odoh, R., & Kolawole, S. A. (2011). Assessment of trace trace metal contaminations of some selected vegetables irrigated with water from river Benue within Makurdi metropolis, Benue State NigeriaAdvances in applied science research. Advances in applied science research, 2(5), 590–615.
- Poggio, M., Hepperle, E., & Marsan, F. A. (2008). Metals pollutions and human bioaccessibility of topsoils in Grugliasco, Italy, environmental pollution, Environmental Pollution, 157(2), 680–689. https://doi.org/10.1016/j.envpol.2008.08.009
- Radwan, M. A., & Salama, A. K. (2006). Market basket survey for some trace metals in Egyptian fruits and vegetables. Food ChemToxicol.
- Rahlenbeck, S. I., Berberg, A., & Zimermmann, R. D. (1999). Lead and cadmium in Ethiopian vegetables. Bulletin of Environmental Contamination and Toxicology, 62(1), 30–33. https://doi.org/10.1007/s001289900837
- Roba, C., Rosu, C., Pistea, I., Baciu, C., Costin, D., & Ozunu, A. (2015). Transfer of trace metals from soil to vegetables in a mining/smelting influenced area (Baia Mare – Ferneziu. Journal of environmental protection and ecology, 16, 891–898.
- Singh, A., Sharma, R. K., Agrawal, M., & Marshall, F. M. (2010). Risk assessment of trace metal toxicity through contaminated vegetables from waste water irrigated area of Varanasi. Tropical ecology, 51(2), 375–387.
- Street, R. A. 2008. Trace metals in South African medicinal plants research center for plant growth and development, PhD Dissertation, University of KwaZulu-Natal.
- Temesgen, E., & Seyoum, L. (2017). Trace metals bio concentration from soil to vegetables and appraisal of health risk in Koka and Wonji farms. Environmental Science and Pollution Research, 24(12), 11807–11815.
- USEPA. 1986. United States environmental protection agency: Guide- lines for the health risk assessment of chemical mixtures.
- USEPA. (2008). Handbook for developing watershed plans to restore and protect our waters. U.S. Environmental Protection Agency.
- USEPA (U.S. Environmental Protection Agency (2004). Integrated risk information system database (IRIS), http://www.epa.gov/iris/subst/0410.htm
- USEPA (U.S. Environmental Protection Agency) (2002).Region 9, preliminary remediation goals. http://www.epa.Gov/region09/waste/sfund/prg
- USEPA (U.S. Environmental Protection Agency) (2005).Integrated risk information system database (IRIS).BariumandCompounds(CASR).http://www.epa.gov/iris/subst/0010.htm
- Wang, X., Sato, T., & Xing, T. (2005). Health risks of trace metals to the general public in Tianjjin, Chainavia consumption of vegetables and fish. Science of the total environment, 350(1-3), 28–37. https://doi.org/10.1016/j.scitotenv.2004.09.044
- Weldegebriel, Y., Chandravanshi, B. S., & Wondimu, T. (2012). Concentration levels of metals in vegetables grown in soils irrigated with river water in Addis Ababa. Ecotoxicology and Environmental Safety, 77, 57–63. https://doi.org/10.1016/j.ecoenv.2011.10.011
- Yihenew, G. S., & Suwanarit, A. (2007). Evaluation of soil-test methods for indexing availability of phosphorus for maize (zea mays l.) grown on alfisols of northwestern Ethiopia. Ethiopian Journal of Natural Resources, 9(1), 1–18.
- Yirgalem, W., Bhagwan, S. C., & Taddese, W. (2012). Concentration levels of metals in vegetables grown in soils irrigated with river water in Addis Ababa.
- Zinabu, E. (2011). Assessment of the impact of industrial effluents on the quality of irrigation water and changes in soil characteristics: The case of Kombolcha city. Irrigation and Drainage, 60(5), 644–653. https://doi.org/10.1002/ird.609