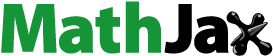
ABSTRACT
The activities of illegal mining have caused devastating effect to the environment, particularly, water and soil. There is therefore the need to ascertain the extent of pollution these activities have caused both soil and water bodies. To this end, this study seeks to identify the prevalence of heavy metal contamination in soil and surface water, determine the correlation between the dominant heavy metals and to ascertain the ecological risk of pollutants in both the waterbody and the soil. The levels of nine predominant heavy metals within Prestea-Huni Valley District of artisanal mining sites were determined using Atomic Absorption Spectrometer (AAS). The extent of pollution within the soil and surface water was determined using Enrichment Factor (EF), Geoaccumulation Index (Igeo), Pollution Index (PI) and Contamination Factor (CF). The concentration of metals in water were below WHO permissible limits, except Ni and Co. The mean concentration of Hg and Cd in the soils of the study area were 2.02 mg/kg and 13.2 mg/kg respectively, which exceeded the WHO permissible limits. The study revealed that the soil and water samples were polluted with heavy metals, particularly Hg and Cd. It is recommended that the polluted sites should be remediated using eco-friendly approach. In addition, Artisanal Mining activities need to be properly regulated to protect both water and soil from further pollution.
Introduction
Surface mining is the dominant method of mining practiced by Artisanal Small Scale Gold Miners (ASGM) due to its cost effectiveness, low capital intensity and minimal technical skill requirement (Boakye, Citation2020). In most parts of the world, ASGM is as important as Large-Scale Mining (LSM), particularly due to the number of people employed. ASGM plays a crucial role in poverty alleviation and rural development of most poor people living in rural mining communities. The Global Report (2002) on Artisanal & Small-Scale Mining noted that about 13 million people across the world are directly engaged in small-scale mining (Hentschel et al., Citation2002). Flavia (2009) stated that small-scale mining is a major source of income for the people living in rural communities in 23 Sub-Saharan African countries. The Ghana Statistical Service (2015) reported that about 300,000 people in Ghana are engaged in small-scale mining. Over the years, small-scale mining has played a very significant role in the socio-economic development of Ghana. It generates both direct and indirect employment, contributing about 40% of the country’s gross foreign exchange earnings (an equivalent of about 5.7% of GDP; Aryee, Citation2001). However, it is marked with huge negative environmental impact (Darko & Ansa-Asare, Citation2014; Duncan et al., Citation2018; Sun et al., Citation2019), both on land and in water bodies. Their operations have caused significant damages to the environment, mainly manifested in land degradation and water contaminations (Hilson, Citation2002). The relative absence of legislation and government controls in Africa makes the environmental impacts of ASGM possibly larger than large scale mining.
Despite the many economic benefits, Ghana is recognized as one of the countries plagued with rising environmental health risk, resulting from illegal mining activities (Darko & Ansa-Asare, Citation2014). ASGM like many extractive activities has bequeathed the environment with enormous quantities of chemical waste having detrimental impact on humans and other living organisms (Ali et al., Citation2016; Duncan et al., Citation2018). ASGM activities have been found to cause severe short and long-term environmental effects including land degradation, air pollution, human health diseases, heavy metal contamination of soil and water sources (Akabzaa & Darimani, Citation2001). Release of heavy metals like Mercury (Hg) into the environment resulting from artisanal mining is a major environmental concern (Rajaee et al., Citation2015). A recent survey by (UNEP, Citation2013) suggested that “ASGM sector is the largest source of Hg into the environment, accounting for about 37% (727 tones) of all global emissions. Studies have shown that chemicals may accumulate in fruits, leaves of vegetables and arable cash crops grown around mine sites (Bartrem et al., Citation2014; Lu et al., 2009). Besides, contamination of nearby farmlands can cause serious heavy metal pollution of water resources, consequently poisoning of humans and animals if ingested (Bartrem et al., Citation2014; Lo et al., Citation2012; Plumlee et al., Citation2013). In addition, heavy metal contamination has been noted as the cause of kidney and respiratory diseases, miscarriages in pregnant women, and death in many mining communities (Twerefou et al., Citation2015).
Several research has been conducted on the extent of heavy metal pollution at different locations using varied geostatistical signatures. Rajkumar et al. (Citation2018) conducted an appraisal on the soil regime pollution by heavy metals in the hilly tracts of Nalagarh valley, Himachal Pradesh, India, using geochemical indexing approaches and chemometric techniques. They conducted their studies using nine heavy metals (As, Cd, Cr, Cu, Fe, Mn, Ni, Pb and Zn). Their results on geochemical indices (Pollution Load Index, Enrichment Factor and ecological risk showed that Cd, Pb and Cu were gradually polluting the valley soil. Hefni et al. (2016) also carried out pollution risk assessment of the surface sediments of Mahakam Delta, East of Kalimantan on four heavy metals (Zn, Cr, Cd and Ni). Their results showed that all the heavy metals were in elevated level (i.e. above WHO permissible threshold). They also concluded that the ecological potential risk of the heavy metals was in the order of Cd>Ni>Zn>Cr. Hefni et al. (2016) only used the Ecological risk potential index as prescribed by Hankinson method (1980). They did not really delve into other criteria for calculating pollution risk assessment such as enrichment factor, pollution load etc. Ecological risk assessment of heavy metals in the soil at a former painting and varnish industry facility at ex-Yugoslavia was also conducted by Radomirović et al. (2020). The heavy metals analysed were Pb, Cd, Zn, Cr, Ni, Cu, Fe and Hg. Their study adopted only the geoaccumulation index to assess the ecological risk of their studied metals, and it was found out that only Pb and Zn exhibited moderate level of pollution in the study sites. Darko et al. (2021) conducted ecological risk of seven heavy metals on surface soils within auto mechanic enclave in Sunyani, Ghana (As, Cr, Cu, Mn, Pb, Ti and Zn). Their analysis was based on Pollution Load Index and Contamination Factor of the heavy metals in the study area. The results showed moderate Contamination factor of Pb with the rest of the metals exhibiting lower contamination factors.
The above literature indicates various research works carried out at different locations prone to heavy metal contamination. Not much research on the comprehensive ecological risk assessment has been carried out in Ghana, particularly at the Artisanal Small Scale Gold Miners (ASGM) zones. It is necessary to establish local geochemical baseline concentrations (GBCs) due to lack or inapplicability of background values in the study area. The establishment of BNCs of heavy metals in both the water and soil would assist in making accurate assessment of heavy metal pollution, and then provide the basis for appropriate pollution control and remediation. This research seeks to undertake ecological risk assessment of heavy metals at the enclave of ASGM in Ghana. Prestea-Huni Valley District (PHD) is a mining community in the Western Region of Ghana. The district is endowed with rich mineral resources and has therefore attracted major mining companies such as Abosso Goldfields, Golden Star Resources, Bogoso/Prestea Ltd, Tarkwa Goldfields and AngloGold Ashanti Ltd. However, due to its richness in mineral resources, the community has been clouded with small-scale mining with many of them been illegal (referred to as “galamsey”; Osei-Bagyina,). As a result, agricultural lands have been converted to mining leading to the shortage of food and cash crop production, reduction in fuel wood production with it associated environmental degradation (Owusu-Koranteng, 2008). Water resources in the community have been woefully polluted.
This study aims at assessing the extent of heavy metal pollution in soil and water bodies in the Prestea Huni-Valley (mining catchment). To achieve this aim, the study seeks to identify and “characterize the prevalence of heavy metal contamination in surface water bodies and soil within the study area; analyze the correlation between the dominant heavy metals in surface water and soil, and assess the toxicity of the pollutants to ascertain the ecological risk of the heavy metals to the environment. The heavy metals selected for analysis were Cadmium(Cd), Chromium (Cr), Copper (Cu), Cobalt (Co), Nickel (Ni), Lead (Pb), Zinc (Zn), Arsenic (As) and Mercury (Hg). These heavy metals were selected based on their toxicity and prevalence in the study area. The study reveals the extent of heavy metals contamination in the available water resources and the soil, as well as the risk of consuming food products and water emanating from the community. The overarching purpose of this research is to serves as the baseline to initiate reclamation and the type of remediation process for the purposes of creating healthy environment for sustainable development.
Materials and methods
Study area
The “study was conducted in the Prestea-Huni Valley District (PHD) of the Western Region, Ghana (). The district is located at 5.472°N, 2.0665° W, and has a land area of about 1,809 km2, accounting for nearly 7% of the region’s total land area. It is bordered on the north by Wassa Amenfi East and Wassa Amenfi Central Districts, on the west by Wassa Amenfi West District, on the south by Tarkwa Nsuaem Municipality, and on the east by Mpohor and Wassa East Districts (). According to the 2010 Population and Housing Census, the district had a population of 159,304 representing 6.7% of the region’s overall population (SSD,2010). PHD has male population of 50.5 %, and female population of 49.5%, and a rural population” of 60%. PHD lies in the “rain forest zone of Ghana, with a humid tropical environment. There are two rainy seasons; March to July (the main season) and September to November (minor season). It has an average yearly rainfall of 187.83 mm and a high year-round temperature ranging from 26 to 30 °C. The humidity of the wet season ranges from 75 to 80%, while the dry season ranges from 70 to 80% (GMA, 2010). The soil is deep, open and acidic in many places due to significant leaching of base from the top soil induced by high rainfall, humidity, and temperatures.
Figure 1. Prestea Huni-Valley District map with the study community marked with a green pentagon symbol.

The vegetation in the area is tropical rainforest, with trees ranging in height from 15 to 40 m. It has many forest reserves, notably among them are the Bonsa Reserve at Abosso (160.58 km2), Ben West at Huni-Valley (26 km2) and Nkontoben also at Huni-Valley with 49.98 km2(SSD,2010). The forest is densely populated, with trees such as mahogany, wawa, odum and sapele, and has abundant growth of food and cash crops such as cocoa, cassava, maize, oil palm, plantain, coffee, rubber and coconut. Over seven out of ten people (73.2%) are economically active, while 26.8% are economically inactive (SSD,2010). Agricultural, forestry, and fisheries (44%) are the most common occupations, followed by plant and machine operators (21%), sales and services (15.2%), craftsmen and associated trade (9.8%), and managers, professionals and technicians (4.4%). Abosso Goldfields, Golden Star Resources, Bogoso/Prestea Ltd, Tarkwa Goldfields and AngloGold Ashanti Ltd are all located in the district (www.statsghana.gov.gh).
Material sampling
Water sampling
The water samples were taken from twenty (20) sampling points spread over four different locations in Huni Valley. Prestia Huni-Valley was selected mainly because, it is noted for unrestricted and indiscriminate indulgence of illegal mining activities. There are also reported cases of surge in Kidney disease in the district resulting from the incessant use of Hg in the activities of illegal mining (Kusi-Ampofo, 2018). Nine heavy metals (Cd, Cr, Cu, Co, Ni, Pb, Zn, As and Hg) were selected for the study. These metals were selected based on their prevalence and toxicity. At each sampling site, five (5) water samples were taken and placed in 500 ml bottles as described by Chapman (Citation1996). The bottles were acid cleaned for 24 hours in 10% nitric acid, rinsed with distilled water, and then oven dried (APHA, 2005). The water samples were then acidified with 0.5% nitric acid for heavy metal determination. The water samples were labeled, placed in an ice chest at 4°C as previously described by Duncan (2018), transferred to the lab within 24 hours, and then placed in a refrigerator before analyzed (APHA, 2005). At each of the sampling location, triplicate sampling was undertaken.
Soil sampling
Soil samples were taken at a depth of 0–15 cm using a soil auger at four distinct sampling sites as described by Bhuyan and Baker (2017). In all, twenty (20) samples were collected. The soil samples were placed in a clean and clearly labeled zip lock polyethylene bags and transported to the Ecological Laboratory, University of Ghana for pre-treatment and analysis. The coordinates of the various sampling places (5° 27.59°, 1° 54.59°W) were captured using the GARMIN Global Positioning System (GPS). Triplicate soil sample from each location were collected. The soil samples were immediately sent to the laboratory for air drying at room temperature, grinding, and sieving for the various analytical tests (Chrastny et al., 2012). The goal of this action was to remove coarse debris and rubbles, as well as to remove any potential interference. The samples were air dried for 5 days, pounded using a porcelain pestle and mortar, sieved with a 2 mm mesh, and packed in well-labeled zip lock bag for various analytical tests.
Digestion of samples
The wet digestion method was used to determine Cd, Cr, Cu, Co, Ni, Pb, Zn, As and Hg in soil samples. 0.5 g of soil samples were weighed into a digestion tube, and digested with 10 mL of Ternary mixture (20 mL of HClO4; 500 mL of HNO3; 50 mL of H2SO4). The digested samples were placed on a digestive furnace and heated at 180°C for 3 hours. The digested samples were filtered using Whatman No. 42 filter paper into a 100 mL volumetric flask and filled to the mark with distilled water after cooling.
Determination of heavy metals
The heavy metals (Cd, Hg Cr, Cu, Co, Ni, Pb and Zn) were determined using Flame Atomic Absorption Spectrophotometer (FAAS) with air-acetylene as the source of fuel to analyze the element (Abbasi et al., 2013). However, the flow injection analysis system of atomic absorption spectrophotometer (FIAS-ASS) involving hydride generation technique and cold vapour technique was used to determine As and Hg. The Atomic Absorption Spectrophotometer (Perkin Elmer PINAAcle 900 t) was calibrated using established standard concentrations of Cu, Zn, As, Cr, Hg, Cd, Pb, Ni, and Co which were then injected into the various systems of the AAS for heavy metal determination (Amoako Ofori, 2015). Measurements were taken three times and the concentrations calculated using the formula below:
Determination of soil contamination
Several methods have been used to calculate the degree of metal enrichment or pollution in soils, sediments and dusts (Schiff K.C. et al., 2006). The Enrichment Factor (EF), Geoaccumulation Index (Igeo), Contamination Factor (CF) and Pollution Load Index (PLI) were used to determine the level of heavy metal contamination” in the soil.
1. Enrichment Factor (EF)
The presence and severity of anthropogenic pollutant deposition on soil surfaces is determined using the Enrichment Factor. It is calculated by standardizing the concentration of one metal in the top soil to the concentration of a reference element (Barbieri M, 2016). The reference element must be stable in the soil, with no vertical mobility or degradation. Al, Fe, Mn and Rb are among the most commonly employed elements in numerous investigations. In this study, “Al was used as the reference material to standardize the heavy” concentration. EF is calculated using the formula as described by (Abrahim and Parker, Citation2008):
Where Ca is the measured “concentration of the element and Cb is the background concentration of the measured element. Caref is the measured concentration of the reference element, whereas Cbref is the background concentration of the reference element” (Appiah-Adjei et al., 2019). According to Yongming et al. (Citation2006), the quality of a soil can be measured by its EF classification. The classification ranges from: deficiency to minimum enrichment (EF<2), moderate enrichment (2< EF<5), significant enrichment (5< EF<20), very high enrichment (20< EF<40), to extremely high enrichment (EF>40).
2. Geoaccumulation Index (Igeo)
The Index of Geoaccumulation (Igeo) was originally proposed by Muller K. (1979), and it’s used to characterize heavy metal contamination in sediments by comparing the current concentration to previous times when there were no or little industrial activities. It is calculated as described by (Vaezi et al., 2015):
Where Cn is the measured concentration (mg/kg) of the metal under investigation and Bn is the geochemical background value of the metal recorded in mg/kg. For modest anthropogenic impact on a specific metal in the environment, the factor 1.5 was employed due to possible changes in background values (Appiah-Adjei et al., 2019). The pollution of the soil can be determined from the Igeo values using Muller classification: “Igeo≤0 (uncontaminated), 0< Igeo<1 (uncontaminated to moderately contaminated), 1< Igeo<2 (moderately contaminated), 2< Igeo<3 (moderately to heavily contaminated), 3< Igeo<4 (heavily contaminated), 4< Igeo<5 (heavily contaminated to extremely contaminated) and Igeo≥5 (extremely contaminated)”.
3. Contamination factor and Pollution Index (PI)
The contamination factor is the ratio of an element’s measured concentration to its background concentration, and it represents each element’s distinct impact on the sediments (Olatunji et al., 2009). It is represented by the equation:
Where Cs is the measured concentration of the element and Cb is the background concentration of the same element. The degree of heavy metal contamination in soils can be expressed using (Martin & Meybeck, 1979) classification tool. They classified the degree of contamination “into four (4) groups; Cf<1(low contamination), 1≤ Cf<3(moderate contamination), 3< Cf<6 (considerate contamination) and Cf>6 (very high contamination”).
The PLI is a summative indication of metal toxicity in a specific sample, measuring the number of times the metal content in the soil exceeds the normal natural background concentration (Confidence, 2017). The PLI of the study area was determined by taking the n-root of the n-CFs obtained for all metals as follows:
Where n = the number of metals analyzed in a study.
Thomilson et al. (1980), classified the PLI degree of contamination of an area in this order: PLI < (the study area is free from contamination), PLI = 1 (base line level of contamination), PLI>1 (deterioration of the area quality).
Soil and water resources within the catchment were the sample materials used for the various geospatial analysis.
Quality assurance control
To assure the reliability of the results, quality assurance and control methods were followed. The samples were handled with care to avoid any external interference and to ensure the integrity of the final result. Blanks and replicate samples were analyzed and the results compared to the test results. For heavy metal determination, the Atomic Absorption Spectrophotometer (Perkin Elmer PINAAcle 900 t) was calibrated using known standard concentrations of Cd, Cr, Cu, Co, Ni, Pb, Zn, As and Hg. Measurements were repeated three times. The plastic bottles and glassware used throughout the study were cleansed with acid.
Statistical analysis of heavy metals
Statistical analysis of the raw data was performed using one-way analysis of variance to examine substantial variations in the mean concentrations of the heavy metals. Pearson’s correlation coefficient was used to establish the association between heavy metals, and the Tukey Test was used to compare mean values. All statistical analysis was performed using the Statistical Package for Social Sciences (SPSS version 24), with a p < 0.05 probability level considered statistically significant. The calculations of the background concentration for the geostatistical signatures were referenced based on water and sediments of water bodies within the catchment of the study area.
Results
Analysis of “heavy metals in water samples”
The mean Zn concentration in surface water samples examined “ranged from 0.002 ± 0.001 mg/L at Site B to 0.016 ± 0.03 mg/L at Site” D. The mean Zn content, on the other hand, did not differ significantly (p > 0.05) among the sites studied. All of the samples tested were below the 3 mg/L acceptable limit (WHO, 2011). Zinc is insoluble and therefore cannot easily be dissolved in water unless oxidized with heated oxygen. And since the density of Zn is higher than water, there is the possibility of it settling at the bottom of the water body. This may account for the limited quantity of Zn in the water. Ultimately, the geological formation of the study area may not have deposits of Zn in the underlying rocks and soil materials. Also, various anthropogenic activities in the study might not have Zn as a by-product to be leached into the water bodies.
At Site C and Site A, the mean Ni concentration ranged from 0.004 ± 0.002 mg/L to 0.21 ± 0.182 mg/L, respectively. According to the analysis of variance, there were no significant differences (p > 0.05) between the sites studied. The results also revealed that Site A and Site B, with 0.21 ± 0.182 and 0.20 ± 0.014 mg/L, respectively, exceeded the allowed limits of 0.07 mg/L. (WHO, 2011). Nickel is abundant natural element which are mostly soluble in water. They might have entered the surface water through leaching from farmlands since most fertilizers are made with Ni, and also waste from industrial areas. The results of Co () revealed that all the readings were above the 0.08 mg/L acceptable limit. The mean Co concentrations ranged from 1.30 ± 0.31 mg/L at Site B to 1.52 ± 0.01 mg/L at Site D. Statistically, there was no statistically significant difference (p > 0.05) in the mean values of Co between the four sites tested. The levels of Co in water is a result of the underlying rock and soil formation having Co as a constituent. They might have leached into the water through surface runoffs as well as through rocks and soils containing Co. Though pure Co is insoluble in water, the compounds above 0.1 g/L are soluble in water. This may account for the elevated levels of Co in water. On average, Pb concentrations ranged from 0.002 mg/L to 0.003 mg/L. The concentrations at all the study sites were almost the same. The mean Pb levels across the four sites were less than the WHO’s tolerable limit of 0.01 mg/L. Furthermore, analysis of variance indicated no statistically significant differences (p > 0.05) across the various sites examined. Cu concentrations varied from 0.03 mg/L and 0.050 mg/L at Sites A and D, respectively, to 0.050 mg/L at Site C. The acceptable level of 2 mg/L was not exceeded in any of the surface water samples evaluated at the four sampling sites. There was no significant variation in Cu concentrations across the four sampling sites (p > 0.05). The results for Cd showed that the mean concentration of Cd was between 0.05 and 0.06 mg/L, respectively. It further revealed that none of the Cd levels measured at the various sampling sites exceeded the allowed limit of 0.03 mg/L. Furthermore, an analysis of variance revealed that there were no significant differences amongst the sites investigated. Cadmium poisoning has been linked to serious renal damage and hypertension (Krishna & Govil, Citation2007). The Cd concentrations measured in this study were lower than the values of 0.02–0.05 mg/L recorded by Orosun et al. (Citation2016).
Analysis of heavy metals in soil samples
The Zn concentrations at the four sampling sites ranged from 14.92 ± 5.43 mg/kg at Site A to 156.72 ± 8.95 mg/kg at Site B, with a mean of 81.37 ± 54.34 mg/kg. Zn levels at all four sites were below the WHO permitted limit of 300 mg/kg. The concentration of Zn among the sites differed significantly (p < 0.05) according to the analysis of variance. Except for Site C and Site D, all of the locations showed substantial variations. Ni levels varied from 4.78 ± 1.63 mg/kg at Site D to 8.10 ± 0.09 mg/kg at Site A, with a mean value of 6.47 ± 1.91“mg/kg. The mean values of Ni at the sites were less than the acceptable limit of 50 mg/kg. The concentration of Ni differed significantly (p < 0.05) among the sites studied, according to analysis of variance. Furthermore, with the exception of Site A and Site C, and Site B and Site C, D, substantial variations were identified at all of the sites.
The concentration of Co ranged from 3.42 ± 1.95 mg/kg at Site A to 8.12 ± 3.23 mg/kg at Site C, with a mean value of 5.96 ± 2.57 mg/kg (). The results were all below the acceptable limit of 100 mg/kg at each location. The concentrations of Co were found to vary significantly amongst the sites. The Turkey test of mean comparison, on the other hand, revealed only a significant difference between Site A and Site C. Lead concentrations in the “soil samples ranged from 0.91 ± 0.27 mg/kg at Site D to 2.48 ± 0.64 mg/kg at Site B, with a mean of 1.67 ± 0.75 mg/kg. The Pb levels found were modest, well below the acceptable limit of 50 mg/kg. The concentrations of Pb among the sites were also shown to differ significantly (p < 0.05) using analysis of variance. However, the Turkey test of mean comparison revealed that significant differences were only seen at Sites B and D.
Cu levels were between 2.40 ± 0.61 mg/kg at Site A to 16.12 ± 7.96 mg/kg at Site C, with an average of 10.76 ± 6.94 mg/kg. The quantities measured were below the WHO acceptable limit (50 mg/kg). The concentration of Cu at the various sites differed significantly, according to the analysis of variance. The mean comparison analysis revealed significant differences at Sites A, B and C, respectively. Chromium levels varied from below the detection limit (BDL) at Site D to Site B (2.32 ± 3.54 mg/kg), with a mean of 0.93 and 1.90 mg/kg. The Cr mean values in this study were also below the 100 mg/kg allowable limit. There was no statistically significant difference (p > 0.05) between the Cr concentrations at the different sites. Site D recorded the lowest value of Cd (8.88 ± 1.94 mg/kg), while Site A recorded the highest value of 16.42 ± 1.62 mg/kg, and a mean value of 13.20 ± 5.25 mg/kg. The mean Cd levels recorded at the study sites were much greater than the 3 mg/kg permissible limit. The concentration of Cd among the various sites did not differ significantly (p > 0.05) according to the analysis of variance. The concentration of As in the soil samples ranged from 2.00 ± 0.90 mg/kg at Site A to 5.08 ± 2.61 mg/kg at Site D, with a mean value of 3.13 ± 1.88 mg/kg. As levels in all the study “sites” were below the permissible limit of 20 mg/kg. The concentration of As among the sites differed significantly, according to the analysis of variance. The observed differences, however, were limited to Sites A and D. The mean Hg concentration was 2.02 ± 0.94 mg/kg, ranging from 1.22 ± 0.44 mg/kg at Site A to 3.08 ± 0.94 mg/kg at Site D. The concentrations of Hg in all the study areas were above the acceptable limit of 2.0 mg/kg, and differed significantly, according to the analysis of variance. However, there was a considerable difference between Site A and D, as well as Site B and D.
Contamination assessment
Enrichment Factor (EF)
The enrichment factors of the samples examined were 0.14, 0.009, 0.08, 0.07, 0.029, 2714.8, 7.94 and 2597.6 for Zn, Ni, Co, Pb, Cu, Cd, As and Hg, respectively (). As a result, the predicted mean values of enrichment factors increased in the order of Ni<Cu< Co<Pb<Zn<As<Cd<Hg. The EF gives an indication of the presence and the intensity of anthropogenic contaminants deposition of soils. As indicated in the results, the enrichment of Hg in study site is more pronounced than the rest of the contaminants. This is followed by Cd and As. The EF of Hg is high because it is mostly in the amalgamation of the gold ore by the small-scale mining operators. Arsenic and Cd are prevalent in these areas because of the geological formations and the anthropogenic activities within the small-scale mining sites.
Geoaccumulation Index (Igeo)
The geoaccumulation index of the materials examined were −0.82, −4.20, 7.67, −3.64, −3.31,
-8.70, 5.37, 0.001, 3.93 for Zn, Ni, Co, Pb, Cu, Cr, Cd, As and Hg, respectively (). The geoaccumulation is an indicator of the magnitude of contamination at the study site. The geoaccumulation index of the contaminants are in the order of Cr<Ni<Pb<Cu<Zn<As<Hg<Cd<Co. Thus Co is assuming alarming proportions in terms of contamination in the study area, which is followed by Cd, Hg and As. These pollutants are dominant in the soil samples than water because the first point of leaching of heavy metals are through the soils. Most of these contaminants are dominant in the study sites because of the geological formations and anthropogenic activities. The high levels of Co in the soil can be attributed to mafic and ultramafic parent rocks in the study area (Albanese et al., Citation2015).
Contamination factor and pollution index
The mean contamination factors of the soil samples obtained were 1.16, 0.86, 0.24, 0.13, 0.20, 0.009, 65.9, 1.74 and 25.3 for Zn, Ni, Co, Pb, Cu, Cr, Cd, As and Hg respectively. The overall degree of contamination of the heavy metals examined rose in the order of Cr< Pb<Cu<Co<Ni<Zn<As<Hg<Cd as shown in the . The ratio of the heavy metal loadings in the soil sample collected are higher for Cd followed by Hg and then As, with the least been Cr. Thus, predominantly, there are elevated levels of Cd, Hg and As in the soil samples, making the study sites to be highly polluted with these contaminants. With these background, an effective remediation measures could be employed to clean the environment.
Relationships between the heavy metals
The association between heavy metals found in water and soil samples was determined using Pearson’s product of correlation coefficient. The threshold “value of probabilities (p < 0.05 and p < 0.01)” were utilized to determine correlation significance.
Pearson’s correlation coefficient of heavy metals in water samples
The metals Co/Zn (r = 0.773) and Cu/Pb (r = 0.870) showed a strong positive association. Cu/Zn (r = −0.851), Co/Ni (r = −0.773), Pb/Zn (r = −0.511), Cd/Zn (r = −0.566), Pb/Ni (r = −0.600), Cd/Co (r = −0.692) all had high negative correlations ().
Pearson’s correlation coefficient of heavy metals in soil samples
Pb/Zn (r = 0.808), Cr/Zn (r = 0.991**), Cd/Ni (r = 0.908), Cu/Co (r = 0.974*), Cr/Pb (r = 0.879), Hg/As (r = 0.879); all have strong positive correlations (). The positive correlation between Pb and Zn indicates the linear relationship between the two metals. The rise in levels of Pb increases the tendency of Zn to increase. This is collaborated by work carried out by Golshan et al. (2013). They investigated the relation between some selected heavy metals (Cd, CU Pb and Zn) and found a strong correlation between Pb and Zn. Though there is a correlation between Pb and Zn in this study, the correlation between Pb and Zn is not significant. So the increase in levels of Pb may not necessarily lead to the increase in the levels of Zn and vice versa. The positive correlation between Cr and Zn is significant. There is therefore high probability to have high levels of Zn where Cr is in elevated levels and vice versa. Also, the correlation between Cu and Co is statistically significant. Cobalt is a metal that is mainly obtained as a by-product of Cu mining activities. This could account for the significant correlation between Cu and Co. Though there is a positive correlation between Cd/Ni, Cr/Pb and Hg/As, but the extent of correlation between them is not statistically significant. Furthermore, there was a high negative association between As/Ni (r = −0.817), As/Pb (r = −0.701), Hg/Pb (−0.791), As/Cd (r = −0.980**), and Hg/Cd (r = −0.887), but the extent of correlation between them are not statistically significant. Though an increase in the level of one of the metals may lead in the decrease of the other metal, there exist less than 5% chance of occurring. The correlation between As and Cd is Significant. Both As and Cd are mostly found in the environment from natural occurrence and due to anthropogenic activities (EFSA, 2009).
Discussion
Heavy metals in water samples
shows the results of Zn, Ni, Co, Pb, Cu, Cr, Cd, As and Hg concentrations in water from the study area. The results revealed extremely low amounts of (Cr, As, and Hg) in all four sites. This could be due to the binding properties of the heavy metals, and the fact that they were sequestered at the bottom of the water. Sediments near the bottom of the water column, according to Forstner et al. (1983), have a substantial influence in heavy metal poisoning of river systems. They represent the current state of the water system and can be used to detect the presence of non-soluble contaminants after they have been discharged into water. Zn has been identified as an essential element for “immune system development, neurobehavioral development, reproductive health, sensory function, and plant and animal growth” (Krishna & Govil, Citation2007). The concentrations of Zn (“0.2 to 0.7 mg/L) with a mean of (0.32 ± 0.17) found in this work were lower than those found by Egbueri et al. (2020) in their pollution and ecological risk assessment of potentially harmful elements in natural waterways. Low natural Zn deposits in sediment of water, or less industrial operations such as zinc mine, effluent discharge, and the use of sewage sludge as fertilizer, could be the cause of the low Zn levels detected in the study area. As a result, Zn contamination in surface water does not constitute a quality issue for surface water supply and development in the study area. Nickel pollution in the soil is mostly caused by “anthropogenic activities such as smelting, metal mining, automobile emissions, fossil fuel burning, domestic, municipal, and industrial waste disposal, fertilizer and organic” manure application, and electroplating (Khodadoust et al., Citation2004; Salt, Citation2000). Akan et al. (2012) found mean Ni concentrations of (0.024–0.043 mg/L), much higher than those found in this study. The presence of Ni in the water could have resulted from biological cycles and the solubilization of its compounds in soil or sediments from Ni in the atmosphere (Adelekan & Abegunde, 2011).
Cobalt is an essential element, and can be introduced into the environment through human activities like runoff from industrial and agricultural operations. Inhalation of Co can cause bronchial asthma, interstitial lung disease, lung cancer, pneumonia, heart difficulties and thyroid harm in humans (www.lenntech.com). The Co concentration in this study (2.0 mg/L) is above WHO allowable limit and similar to obtained by Akan et al. (2012). As a result, the water from these places is contaminated with cobalt (Co), which could be due to automotive exhaust emissions, industrial activity related to metal mining and processing, and other factors (Faroon et al., 2004). Cobalt concentrations over a certain level become hazardous to aquatic creatures and plants, and water quality suffers as a result (Bhagure & Mirgane, 2010). Lead has been discovered to be an extremely dangerous trace element that builds up in living things (Akhileshi et al., 2009). Ingestion of Pb is known to induce a variety of negative health effects, including “mental retardation, impaired hearing, reproduction problems, anemia, and cancer” (Egbueri et al., 2020). Pb concentrations ranged from 3.1 to 76.9 mg/kg, and are comparable to those found by Obiri (2007). Copper is introduced into water systems through mineral dissolution, home and industrial effluents, and pesticide application. It is a necessary element for food metabolism and production of haemoglobin, myoglobin, cytochrome and numerous enzymes (Maughan, Citation1999). However, ingestion of high levels of copper can cause neurological complications, as well as liver and kidney diseases (Krishna & Govil, Citation2007). Generally, almost all the elevated heavy metals were recorded due to the geological formations of the area, within their rock and soil constituents.
Heavy metals in soil samples
The mean concentrations of Zn, Ni, Co, Pb and Cu in this study were greater than the 0.55 mg/kg for Zn, 0.32 mg/kg for Ni, 0.62 mg/kg for Co, 0.16 mg/kg for Pb and 0.34 mg/kg for Cu found by Lukuku et al. (2013). The concentrations of Zn, Ni, Co, Pb and Cu were significantly greater than their background concentrations, indicating that they emanated from natural sources. Yongming et al. (Citation2006), recorded 167.3 mg/kg for Cr and 9.78 mg/kg for As which were higher than those recorded in this study. The ores of Zn are formed by natural geological processes and are naturally present in the earth crust as part of rocks or Zn-rich ores. They are present in almost all soil. Their levels are enriched in soil through the human activities fertilizer application as well as atmospheric depositions (Mertens et al., 2013). The levels of Ni in the soil may be a result of weathering activities of geological rock formations of the area, as well as forest fires in the study area as ascribed by Genchi et al. (Citation2020). The levels of Co, Pb and Cu are naturally found in soils due to the geological settings/formations of the area. The elevated levels of these contaminants may be due to anthropogenic activities such as mining, leaching of waste products from industries etc.
Contamination Assessment
Enrichment factor
The enrichment factors of Zn, Ni, Co, Pb and Co were less than 2, indicating that the heavy metals in the soil have deficient to minimum enrichment (EF<2). The mean enrichment factor value of As estimated, on the other hand, indicates moderate soil enrichment with As (EF = 2–5), whereas Cd and Hg indicated exceptionally high soil enrichment in the soils examined (EF > 40). Comparing the EF values of the different sites shows that Site C is the most contaminated, followed by Site D, with Site A and B having approximately identical EF’s. The research conducted by Radomirovic et al. (2020) on the ecological risk assessment of heavy metals (Pb, Cd, Zn, Cr, Ni, Cu, Fe and Hg) in soil of former paint industry in Yugoslavia revealed that that there was only moderate soil enrichment for Pb and Zn among six other heavy metals studied. They ascribed EF value of heavy metal less than one as originating from the earth crust or from natural weathering process. Furthermore, Zepeng Jiao et al. (Citation2018) conducted a study of ecological risk assessment of heavy metals (Cr, Cd, Cu, Zn, Co, Ni and Ti) on the sediments of Pearl River estuary in China. Their studies concluded that the sediments of the river were highly enriched with all the heavy metals studied. They ascribed the high enrichment of the heavy metals to a mixture of anthropogenic activities, industrial effluents etc.
Geoaccumulation Index (Igeo)
The geoaccumulation indexes for Zn, Ni, Pb, Cu, Cr and As indicated that the soils in the study area are virtually free from these heavy metals, but were heavily polluted with Hg and extremely polluted with Cd and Co. Hence, the mean geoaccumulation index values is in the order of Cr< Ni<Pb<Cu<Zn<As<Hg <Cd<Co. According to Basta et al. (2005), this could be due to the continuous application of fertilizer and biosolids. Similar work carried out by Radomirovic et al. (2020) concluded that the soil studied were moderately polluted with Cr, Cd, Ni and Hg, Cu whilst As in the soil was revealed to be moderately to strongly polluted. Again, they identified that the soil was strongly polluted with Pb and Zn. The study conducted by Zepeng Jiao et al. (Citation2018) on the Pearl River also concluded that the Igeo of the heavy metals in the sediments of the River were moderately polluted with Cr, Co, Ni, Pb and Cu and strongly polluted by Zn and Cd.
Contamination factor and pollution index
The contamination factor for Ni, Co, Pb, Cu and Cr were less indicating low heavy metal pollution in the soils. Moderate As and Zn contamination, on the other hand was recorded whilst Cd and Hg indicated extremely high levels of contamination. However, the pollution load index of the soil (all four sites) indicated little contamination, since the all the results fell within PLI<1 group (). This indicates that the presence of these heavy metals was limited (Pradhan & Kumar, Citation2014). Rahmania (2020) carried out a research on contamination factor and pollution load index of selected heavy metals (Pb, Cd, Ni and Mn) in soils around a cement factory in South-West Iran. The contamination factor analysis revealed extreme pollution of Pb and Mn in almost all the samples collected. The study further revealed that the Pollution Load Index was between 1 and 2.3, which suggests that industrial activities and bedrock weather contributed to the high CF and PLI of the study area.
Correlation analysis
There is a strong positive correlation between Pb and Zn, Cr and Zn, Cd and Ni, Cu and Co, Cr and Pb as well as Hg and As. This correlation analysis is an indication of the fact that, the increase or decrease in the levels of Pb may lead to a corresponding increase or decrease in the level of Zn. Where there is either an elevated or lower levels of Cr, there is the tendency to have a corresponding higher or lower level of Zn. The positive correlation between Cd and Ni shows that the levels of Cd have a direct influence on the levels of Ni within the affected catchment. The increase or decrease in the levels of Cu may lead to a corresponding increase or decrease in the level of Co. Where there is either an elevated or lower levels of Cr, there is the tendency to have a corresponding higher or lower level of Pb. The increase or decrease in the levels of Hg may lead to a corresponding increase or decrease in the level of As.
There is a strong negative correlation between As and Ni, As and Pb, Hg and Pb, As and Cd, as well as Hg and Cd. The negative correlation between the paired heavy metals shows that there is no direct or corresponding increase or decrease in the levels of the heavy metals with change in level of the one paired heavy metal.
Conclusion
The study assessed the extent of heavy metal pollution in soil and water bodies of illegal mining catchment within Prestea Huni-Valley. To achieve this, the study sought to characterize and “identify the prevalence of heavy metal contamination in surface water bodies and soil within the study” area; analyze the correlation between the dominant heavy metals in surface water and soil, and assessed the ecological risk of the heavy metals to the environment. The levels of Zn, Ni, Co, Pb, Cu, Cr, Cd, As and Hg concentrations observed in the water bodies were within the acceptable standards. However, the levels of Ni and Co in water were in elevated levels above the WHO Standard. In the soil strata, the levels of Cd and Hg where also in elevated levels. With time, there is the tendency for the Ni and Co levels to be high within the soil. It is expected that there will be the likelihood of the Cd and Hg being leached into the water bodies as a result of erosion and other anthropogenic activities. There are both strong positive and negative correlations between some of the heavy metals.
Pollution levels in the soil samples were minimal, according to the pollution index of the metals examined. However, Cd, As and Hg recorded moderate to high pollution. This might be due to the increasing anthropogenic activities such as mining, agriculture, coal combustion, landfilling etc. The enrichment factor analysis revealed that Cd and Hg had exceptionally high enrichment in the soil, while As had a moderate enrichment. The geoaccumulation index “indicated that the soils were heavily contaminated with Cd and Hg”. Finally, because bioaccumulation of heavy metals in the environment causes severe health risk, routine monitoring of these metals is necessary to protect human health and the ecosystem. Subsequently, further studies would adopt Artificial Intelligence method to establish the pollution and contamination levels of the heavy metals in the study area in comparison with the results of the geoaccumulation indices. This would enable us ascertain the most effective approach in determining baseline indicators of the occurrence of heavy metal pollution and contamination in the study area.
Author Contributions
Samuel Wiafe: Conceptualized the research topic, formulated the objectives and methodology of the research, and participated the data collection and analysis.
Yeboah Eric Awuah: He carried out both the laboratory and Field Investigation as well as the Data Collection of the research work.
Boakye Ebenezer: He carried out laboratory work and assisted in the writing of the manuscript.
Ofosu Samuel: He participated in the writing of the manuscript (Original Draft Preparation) as well as reviewed and edited the manuscript.
Ethical conduct
This manuscript has never been published in any journal or sent to any journal for consideration and publication. Strict scientific ethical standards were adhered to.
Consent for Publication
The author fully bore the responsibility of this manuscript and have therefore given their respective consent to the publication of this manuscript.
Availability of data and Materials
All data used in this manuscript are available at the discretion of the authors.
Disclosure statement
No potential conflict of interest was reported by the author(s).
Additional information
Funding
References
- Abrahim, G. M. S., & Parker, R. J. (2008). Assessment of heavy metal enrichment factors and the degree of contamination in marine sediments from Tamaki Estuary, Auckland. New Zealand.Environ Monit Assess, 136(1–3), 227–15. https://doi.org/10.1007/s10661-007-9678-2
- Akabzaa, T., & Darimani, A. (2001). Impact of mining sector investment in Ghana: A study of the Tarkwa mining region. Third World Network, 11(2), 47–61. https://doi.org/10.12691/aees-3-3-3
- Albanese, S., Segedhi, M., & Lima, A. (2015). GEMAS: Cobalt, Cr, Ni Cu Distribution in Agricultural and Grazing Land Soil of Europe. J. Geochem. Explor, 154, 81–93. https://doi.org/10.1016/j.gexplo.2015.01.004
- Ali, M. M., Ali, M. L., Islam, M. D. S., & Rahman, M. D. Z. (2016). Preliminary assessment of heavy metals in water and sediment of Karnaphuli River, Bangladesh. Environmental Nanotechnology, Monitoring & Management, 5, 27–35. https://doi.org/10.1016/j.enmm.2016.01.002
- Aryee, B. N. (2001). Ghana’s mining sector: Its contribution to the national economy. Resources Policy, 27(2), 61–75. https://doi.org/10.1016/S0301-4207(00)00042-8
- Bartrem, C., Tirima, S., von Lindern, I., von Braun, M., Worrell, M. C., Mohammad Anka, S., Abdullahi, A., & Moller, G. (2014). Unknown risk: Co-exposure to lead and other heavy metals among children living in small-scale mining communities in Zamfara State, Nigeria. International Journal of Environmental Health Research, 24(4), 304–319. https://doi.org/10.1080/09603123.2013.835028
- Boakye, E., Anyemadu, F.O.K., Quaye-Ballard, J.A., and Donkor, E.A.(2020). Spatio-temporal analysis of Land use/cover changes in the Pra River Basin, Ghana. Applied Geomatics, 12(1), 83–93.
- Chapman, D. (1996). Water quality assessments - A guide to use of Biota, sediments and water in environmental monitoring. In Printed in Great Britain at the (Second ed., pp. 1–609). University Press, UNESCO/WHO/UNEP.
- Darko, H. F., & Ansa-Asare, O. D. (2014). Water quality assessment of the Southwestern and Coastal River Systems of Ghana. Journal of Environment and Earth Science, 4(14), 118–128.
- Duncan, A. E., de Vries, N., & Nyarko, K. B. (2018). Assessment of heavy metal pollution in the sediments of the River Pra and Its Tributaries. Water, Air, & Soil Pollution, 229(8), 272. https://doi.org/10.1007/s11270-018-3899-6
- Genchi, G., Carocci, A., Lauria, G., Sinicropi, M. S., & Catalano, A. (2020). Nickel: Human health and environmental toxicology. International Journal of Environmental Research and Public Health, 17(3), 679. https://doi.org/10.3390/ijerph17030679
- Hentschel, T., Hruschka, F., & Priester, M. (2002). Global report on artisanal and small-scale mining. Report commissioned by the Mining, Minerals and Sustainable Development of International Institute of Environment and Development. http:www.iied.org/mmsd/mmsd_pdfs/asm_global_report_draft_Jan02. pd
- Hilson, G. (2002). Small-scale mining in Africa: Tackling pressing environmental problems with improved strategy. The Journal of Environment & Development, 11(2), 149–174. https://doi.org/10.1177/10796502011002003
- Jiao, Z., Huanyong, L., Song, M., & Wang, L. (2018). Ecological risk assessment of heavy metals in water and sediment of the Pearl River Estuary, China. Conf. Series: Materials Science and Engineering, 394(2018), 052055. https://doi.org/10.1088/1757-899X/394/5/052055
- Khodadoust, A. P., Reddy, K. R., & Maturi, K. (2004). Removal of nickel and phenanthrene from kaolin soil using different extractants. Environmental Engineering Science, 21(6), 691–704. https://doi.org/10.1089/ees.2004.21.691
- Koellner, T., De Baan, L., Brandao, M. (2013). UNEP-SETAC guideline on global land use impact assessment on biodiversity and ecosystem services in LCA. The International Journal of Life Cycle Assessment, 18(6), 1188–1202.
- Krishna, A. K., & Govil, P. K. (2007). Soil contamination due to heavy metals from an industrial area of Surat, Gujarat, Western India. Environmental Monitoring and Assessment, 124(1), 263–275. https://doi.org/10.1007/s10661-006-9224-7
- Lo, Y. C., Dooyema, C. A., Neri, A., Durant, J., Jefferies, T., Medina-Marino, A., & Brown, M. J. (2012). Childhood lead poisoning associated with gold ore processing: A village-level investigation—Zamfara State, Nigeria, October–November 2010. Environmental Health Perspectives, 120(10), 1450–1455. https://doi.org/10.1289/ehp.1104793
- Maughan, R. J. (1999). Role of micronutrients in sport and physical activity. British Medical Bulletin, 55(3), 683–690. https://doi.org/10.1258/0007142991902556
- Orosun, M. M., Tchokossa, P., Nwankwo, L. I., Lawal, T. O., Bello, S. A., & Ige, S. O. (2016). Assessment of heavy metal pollution in drinking water due to mining and smelting activities in Ajaokuta, Nigeria. Nigerian Journal of Technological Development, 13(1), 31–39. https://doi.org/10.4314/njtd.v13i1.6
- Plumlee, G. S., Durant, J. T., Morman, S. A., Neri, A., Wolf, R. E., Dooyema, C. A., & Brown, M. J. (2013). Linking geological and health sciences to assess childhood lead poisoning from artisanal gold mining in Nigeria. Environmental Health Perspectives, 121(6), 744–750. https://doi.org/10.1289/ehp.1206051
- Pradhan, J. K., & Kumar, S. (2014). Informal e-waste recycling: Environmental risk assessment of heavy metal contamination in Mandoli industrial area, Delhi, India. Environmental Science and Pollution Research, 21(13), 7913–7928. https://doi.org/10.1007/s11356-014-2713-2
- Rajaee, M., Obiri, S., Green, A., Long, R., Cobbina, S. J., Nartey, V., Buck, D., Antwi, E., & Basu, N. (2015). Integrated assessment of artisanal and small-scale gold mining in Ghana—Part 2: Natural sciences review. International Journal of Environmental Research and Public Health, 12(8), 8971–9011. https://doi.org/10.3390/ijerph120808971
- Rajkumar, H., Naik, P.K., & Rishi, M.S. (2018). Evaluation of heavy metal contamination in soil using geochemical indexing approaches and Chemometric techniques. International Journal of Environmental Science and Technology, 16(11), 7467–7486.
- Salt, D. E. (2000). Phytoremediation of Contaminated Soil and Water (pp. 189–200). Lewis Publishers.
- Sun, D. M., Ardestani, V., & Frouz, M. M. J. (2019). Nitrogen fertilization and its legacy have inconsistent and often negative effect on plant growth in undeveloped post mining soils. Soil Tillage Res, 195, 104380. https://doi.org/10.1016/j.still.2019.104380
- Twerefou, D. K., Tutu, K., Owusu-Afriyie, J., & Adjei-Mantey, K. (2015). Attitudes of local people to mining policies and interventions. International Growth Centre, Ref: E-33107-GHA-1. https://www.theigc.Org/wp-content/uploads/2015/08/Twerefou-et-al-2015-Working-paper-1.pdf.
- Yongming, H., Peixuan, D., Junji, C., & Posmentier, E. S. (2006). Multivariate analysis of heavy metal contamination in urban dusts of Xi’an, Central China. Science of the Total Environment, 355(1–3), 176–186. https://doi.org/10.1016/j.scitotenv.2005.02.026