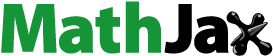
ABSTRACT
Understanding soil types in a specific region is crucial for developing effective approaches to control irrigation water usage and promote sustainable agriculture. This research aimed to characterize and classify the soils of Ajacho Lintala irrigation scheme located in Soro woreda of Southern Ethiopia. Through the analysis of 25 auger observations, 4 pedons, a comprehensive reconnaissance survey, and laboratory analysis, this study examined the morphological, and physicochemical characteristics of the soils in the irrigation. The study identified 16 soil samples from four pedons, consisting of both undisturbed and disturbed soil profiles, which were collected for laboratory analysis. The soils in the research irrigation scheme were classified using the FAO/WRB 2015 classification system. The findings revealed that the texture classes of the study irrigation scheme’s soils varied from loam to clay loam for Nitisols, silt loam to clay loam for Luvisols, and clay for Vertisols. Moreover, the available phosphorus, total nitrogen, and organic carbon in the soils were categorized as very low to low range, while cation exchange capacity and basic cations (Ca2+, Mg2+, K+, and Na+) were in the medium to high range. Based on morphological and physicochemical investigations, the soils were classified as Humic Nitisols, Rhodic Luvisols, Haplic Luvisols, and Pellic Vertisols. These classifications align with the equivalent USDA classification of Ultisols (Typic Haplustults), Alfisols (Typic Haplustalfs), and Vertisols (Typic Haplusterts), respectively. To maintain and enhance soil fertility a sustainable soil management system is recommended, which includes practices such as continuous crop cover, mulching, and organic manuring with suitable inorganic fertilizers. Additionally, implementing physical land management measures to control soil erosion in slope areas is crucial.
1. Introduction
Life on earth is supported by soil, a finite natural resource that is a three-phase (solid, liquid, and gaseous), dynamic resource (Yacob & Nigussie, Citation2022). It provides essential resources for human and animal life, such as food, fodder, and fuel (Schoonover & Crim, Citation2015). The goal of soil characterization is to categorize and identify chemical and physical characteristics which may indicate the soil’s functionality but are not readily apparent during field research (Sanchez et al., Citation2003).
The classification of soils facilitates knowledge transmission and idea exchange among academics, decision-makers, developers, and agronomic extension experts (Assen & Yilma, Citation2010). It also helps determine the utmost suitable use of land, estimates crop productivity, and facilitates these interactions among these groups (Dinssa & Elias, Citation2021).
Evidence on soil classification and distribution is critical for proper planning and implementation of sound management practices that allow for the restoration of degraded lands and fertility status (Dessalegn et al., Citation2014). Soil information can be obtained through systematic field observation, evaluation, and identification, and it provides information about the possibilities and limitations of the land (Lufega & Msanya, Citation2017). Because soils have extensive use of morphological, physical, chemical, and biological characteristics, their categorization and classification are critical for obtaining soil information (Yacob & Nigussie, Citation2022).
Soil classification is a scientific method of gathering soil data that allows for the identification of chemical, physical, and mineralogical parameters of soils (Sharu et al., Citation2013). It is a perilous constituent in understanding the soil situation (Onyekanne et al., Citation2012). Furthermore, soil classification records enable the ideal classification of the soil to be used as the foundation for a more detailed assessment of the soil (Sharu et al., Citation2013). Soil characteristics are important to make decisions with regard to soil management practices for sustainable agricultural production, rehabilitation of degraded ecosystems, and sound research on soil fertility (Ayalew et al., Citation2015; Dessalegn et al., Citation2014). Pedological characterization and classification of soils of a given area is crucial for the determination of their potential and constraints for enhanced and sustained agricultural production (Alemu & Buraka, Citation2018). Soil characterization is intended to classify soil and determine chemical and physical attributes that can reflect the capacity of soil to function when not visible in field examination (Sanchez et al., Citation2003). Soil classification is helpful to identify the most appropriate use of the land, estimate the production, and facilitate technology transfer and information exchange between soil scientists, policymakers, planners, researchers, and agricultural extension consultants (Assen & Yilma, Citation2010; Dinssa & Elias, Citation2021).
Ethiopian soils have been classified into 19 types of reference soil groups, according to the recent FAO/WRB’s (Citation2015) classification. Nitisols, Vertisols, and Luvisols are among the utmost widespread soil kinds elucidate for 13.5, 10.5, and 5.8% of the agrarian farmlands of the country's uplands, respectively (Abebe, Citation1988; Elias, Citation2017; Mengistu, Citation2003), since they have moderate to significant possibility of rain-fed agriculture if managed properly.
Nonetheless, in the research scheme, these various types of soil were not fully identified, and their characteristics for irrigation purposes were not well established. Specific areas of Ethiopia have recommended different farming techniques and inputs based on the variations in soil types and their intrinsic physicochemical and morphological characteristics (Getahun, Citation2015). Soils differ in their parameters, and major soil types and rainfall regimes influence crop productivity. In Ethiopia, the consideration of soil types has influenced agricultural production and methods for ecologically sound land management (Assen & Yilma, Citation2010).
Furthermore, soil characterization and classification in Ethiopia have been studied at a regional and small scale, which is insufficient to provide basic soil information that can benefit soil management based on local variability (Hailu et al., Citation2015). Providing a basis for blanket recommendation of various packages of practices including fertilizers and other inputs disregarding the mosaic soil nature of Ethiopia (Deressa et al., Citation2013). Due to the inherent diversity in soils and the adopted practices with intensive application, the soils are evidently expressing numerous complex problems, which are identified at different stages (Hailu et al., Citation2015). However, the soil information currently available is very limited and derived from small-scale studies. As a consequence, it is not possible to give site-specific appropriate recommendations for agricultural problems based on spatial variability of soil properties (Buraka & Lelago, Citation2016).
Evidence on agricultural soil types, morphological, physical, and chemical properties in the southern highlands is essential for proper judgments and maintaining land productivity. Previous soil resource studies, on the other hand, are notable for their limited scope and a high degree of broadening. All irrigation schemes, particularly those in the Soro district, must conduct extensive research to tackle this problem to specify the specific research objectives or goals. Nonetheless, the soils of this small-scale irrigation scheme have not been thoroughly researched. As a result, the purpose of this research was to categorize and characterize the soils of the Ajacho Lintala small-scale irrigation scheme in the Soro district of Southern Ethiopia.
2. Materials and methods
2.1 The description of the irrigation scheme
Ajacho Lintala small-scale irrigation project is located in Harchuu-Yaya village, Soro District, Hadiya Zone administration of Southern Nation and Nationalities People Region (SNNPR) State. It is located around 255 km west of Hawassa City, 32 km all weathered road west of Hosanna town, and 7 km East of Gimbicho Town (Figure ). Geographically, it is between 37° 39’E–37° 40’E longitude and 7° 26’N–7° 27’N latitude, with an elevation range from 2119 to 2030 m above sea level. The research scheme shields a total command area of 113.7 ha.
The study area is categorized into a moist mid-highlands climatic zone with the average annual maximum and minimum temperatures of 24.2°C and 8.9°C respectively. The mean monthly rainfall varied from 17 to 193 mm with the highest in August and July months, while the mean yearly rainfall is 1194 mm (Erango et al., Citation2017).
The prevailing land use of the study irrigation scheme is intensive cultivation, a rainfed arable cropping system. The main crops are maize (Zea mays), sorghum (Sorghum bicolor), barley (Hordeum vulgare), teff (Eragrostis tef), potato (Solanum tuberosum), and haricot bean (Phaseolus vulgaris). Livestock grazing occurs along the Lintala River’s banks on deteriorated exposed parts with patches of grassland. A small portion of the irrigable area is used for perennial crops such as false banana, but the majority of the land is intensively farmed for cereal crops. Eucalyptus trees are found in patches around homes and along parcel boundaries, and the farm yards were mostly surrounded by indigenous trees.
Around 80% of the command area varies with slope class rated as gentle slope suitable for irrigation, according to FAO (Citation2006), which categorizes, describes, and presents the slope class of the research area in Figure .
2.2 Soil profile opening and sampling
Soil profile pits were opened in representative locations and used to characterize the soil (Table ). The locations were chosen to represent three soil types (Nitisols, Vertisols, and Luvisols), and four pits were opened with the dimensions of 2 m in depth, 1 m in width, and 2 m in length. The soil profiles’ morphological characteristics were characterized in their original state using the FAO Guidelines for soil description (FAO, Citation2006). Soil Munsell charts were used to describe the color of the soil.
Table 1. Selected environmental information of representative profiles at Soro district
To better understand the variations in the land surface of the irrigation location, a reconnaissance field visit was made. After a preliminary assessment of the irrigation scheme and boundary demarcation, auger observations were undertaken to look into the features of the farming. Fifty-three augured samples were collected from various portions of the irrigation site to characterize the disparity in the soil in this research location. The augured observation locations were collected using a Global Positioning System (GPS) and geo-referenced using ArcGIS.
The soil field descriptions were completed following the FAO procedures for the description of soil horizons and site characterizations (FAO, Citation2006). Data related to physicochemical and morphological characteristics were noted on a typical depiction sheet while in the scheme, along with other pertinent site data. Soil samples were taken from each layer of soil profiles, starting from the deep layer and working up to the surface soils, to prevent contamination for laboratory examination.
Following the guidelines provided by FAO (Citation2006), the pedons were split based on the presence of soil-forming processes that contributed to horizon development, described, and the hue of each horizon was inferred using the updated soil color diagram (Munsell, Citation2000). For laboratory examination, a total of 16 destructed and un-destructed soil samples were taken from each visible diagnostic layer. Using a core sampler, un-destructed soil samples were obtained to calculate the bulk density (BD), field capacity (FC), and permanent wilting points (PWP).
2.3 Soil sample preparation
Standard laboratory practices were used to gather soil samples from every layer in the soil profiles, packed, categorized, and sent to the Ethiopian construction and supervision works for physicochemical investigation. The soil samples were air-dried and passed through a 2-mm sieve in preparation for laboratory analysis. To avoid coarser material, soil samples for organic carbon (OC) and total nitrogen (TN) were passed through a 0.5 mm mesh.
2.4 Laboratory analysis
2.4.1 Physical properties
Following a suggestion from Bouyoucos (Citation1962), the soil’s particle size distribution was examined. The bulk density (BD) of the soil was ascertained using the core sampler technique as designated in Blake and Hartge (Citation1986). The total porosity (TP) was evaluated by taking the mean value of soil particle density (PD) of 2.65 g/cm3 using equation 1:
Where, TP = total porosity (%); PD = particle density, and BD = bulk density.
Field capacity (FC) and permanent wilting point (PWP) water retention contents were measured using the pressure plate apparatus technique at water potentials of −1/3 bar and −15 bar, respectively (Richards, Citation1965). The available water content (AWC) of the soils (mm/m) was measured using equation 2:
2.4.2 Chemical properties
In suspensions of 1:2.5 between the soil and the water, the pH of the soil was measured electrochemically employing a pH meter (Jackson, Citation1958). To measure the pH of fresh soil, duplicate subsamples (15 g fresh weight) from a sieved soil sample are suspended in solution by adding deionized water in a soil:water ratio of 1:2.5. The amount of organic carbon (OC) in the soil was measured with the aid of a rapid titration approach (Walkley & Black, Citation1934). Oxidisable matter in the soil is oxidised by 1 N K2Cr2O7 solution. The reaction is assisted by the heat generated when two volumes of H2SO4 are mixed with one volume of the dichromate, whereas, in a soil water extract, the electrical conductivity (EC) was estimated with a conductivity meter (Okalebo et al., Citation2002). A special apparatus, namely, conductivity meter and cell, and shaking bottles were used. Using reagents such as distilled water and potassium chloride, the procedure is to dissolve 0.746 g KCl AR (previously dried at 105°C for 2 hours) and make volume to 1 L with CO2 free de-ionised water. This solution has an electrical conductivity of 1.413 dS/m at 25°C.
Kjeldahl methods were employed to estimate the amount of total nitrogen (TN) in the soil described by Jackson (Citation1958), this method of determination involves three successive phases which are, digestion of the organic material to convert nitrogen into HNO3. Distillation of the released ammonia into an absorbing surface or medium, and volumetric analysis of the ammonia formed during the digestion process. The excess of standard HCl is titrated against standard NaOH using Methyl Red as an indicator. The decrease in the multiple equivalence of acid, as determined by acid-base titration, gives a measure of the N content of the sample. The end point is determined by a change of colour from pink to yellow (Jackson, Citation1958). The available phosphorus was determined by the Olsen extraction method as designated by Bray and Kurtz (Citation1945). The Olsen method uses NaHCO3 extractant that can remove Ca-phosphates and phosphates adsorbed on surfaces of calcium and magnesium carbonates along with Al-phosphates. Phosphorus is extracted from the soil with 0.5 M NaHCO3 at a nearly constant pH of 8.5. The OH− and CO32- in the NaHCO3 solution controls the activity of Ca2+, Al3+, and Fe by precipitation of calcium as carbonate, and aluminum and iron as hydroxides. Soil extraction was carried out for 30 min at a soil/solution ratio of 1:20. P extraction is affected by several analytical factors: soil/solution ratio, shaking time, shaker type, shaking bottle position, capacity and type of shaking bottles, contact time of the soil in the extractant and temperature (Bray & Kurtz, Citation1945).
The basic cations and CEC were obtained by exhausting the ammonium acetate system (Van Reeuwijk, Citation2002). Ca2+ and Mg2+ were obtained by atomic absorption spectrophotometer (AAS); the flame photometer method was used for the determination of Na+ and K+. Then, base saturation (BS) was determined from the summation of basic cations as a percentage of the summation of CEC as described in equation 3:
2.5 Methods of data analysis
The data were analyzed by simple measures of dispersion. The laboratory figures were also evaluated using critical levels defined by various authors. FAO/WRB (Citation2015) described the methods for identifying soil types. To determine the strength and way of correlations between soil physicochemical parameters, a straightforward linear correlation investigation was performed with the assistance of the analysis of statistics (SAS, Citation2003).
3. Results and discussions
3.1 Morphological characteristics of the studied soil
The surface layer of Nitisols soil color is red to reddish-brown and dark reddish-brown to dark brown color (2.5 YR 2.5/3 to 2.5 YR 2.5/4), whereas the Luvisols are dark brown (7.5 YR 3/4) to brown (7.5 YR 4/4), and Vertisols are 10 YR 3/1 to 10 YR 5/2. Regarding the color of the underlying strata of soil, Luvisols and Vertisols ranged from extremely dark brown to dark brown (Table ), while Nitisols ranged from dark reddish-brown to dusky red (Table ). These variations in iron oxide forms, parent material types, organic matter concentration, and drainage conditions are most likely accountable for the color variations across the sampled four pedons and within a soil profile (Abate et al., Citation2014; Buol et al., Citation2011).
Table 2. Morphological properties of selected pedons in the Soro district
The soil arrangement of Nitisols in the surface soils ranged from weak to medium grade, fine to medium size, and angular blocky to subangular blocky type. The Luvisols are characterized by weak to medium grade, fine to medium size, and sub-angular blocky to granular soil structure type (Table ). The vertisols of the surface soil are characterized by weak to moderate grade, fine to coarse size, and angular blocky structure type (Table ). Granular soil structure is exhibited in surface soils, whereas in the underlying horizons, angular to sub-angular structures are formed (Yitbarek et al., Citation2016).
The consistency of the Nitisols varies from slightly hard to hard when dry, friable to very friable, and slightly firm when wet, sticky, and plastic when moist. The consistency of the Luvisols varies from friable to very friable when wet, and they become sticky and plastic when moist (Table ). The Vertisols are slightly hard to hard when dry, friable when moist, slightly sticky and slightly sticky when wet; slightly moist in the surface layer, very sticky and plastic when wet; slightly moist; compacted and non-cemented; with few fine roots in the underlying horizons (Table ).
The variations in soil consistencies of Vertisols, particularly in the sticky, extremely sticky and plastic, and very plastic subsurface layer, were attributed to an increase in clay particles, a decrease in organic matter content, and the presence of difficult-to-work-with soils (Ayalew et al., Citation2015; Dinssa & Elias, Citation2021). The difference in color among the pedons and within a pedon is most probably due to variation in the forms of iron oxide, the types of parent material, OM content, and drainage conditions (Abate et al., Citation2014; Alemu & Buraka, Citation2018; Ali et al., Citation2010; Dinssa & Elias, Citation2021). Moreover, the variations in color observed could probably be attributed to differences in depth and topographic position in clay content in the study area. Dengiz et al. (Citation2012) also indicated that soil color could be related to organic matter, waterlogging, carbonate accumulation, and redoximorphic features.
The soil depths of Nitisols, Luvisols, and Vertisols were deep, that is greater than 150 cm (FAO, Citation2006). According to Moshago et al. (Citation2022), the depth of soil influences both the storage of water and plant nutrients in addition to its effect on root development. Therefore, soils in study area were deep which had capacity to hold nutrients and moisture for long period and were favorable for root development, and this facilitated the irrigation practices with any difficulties. Soil depth can influence water availability when irrigation the major crops in the study area. For any given soil, the greater the rooting depth, the larger will be the quantity of soil water available to the crop. This is particularly important for annual crops as they have less time to develop deep and extensive rooting systems than perennial crops (Fekadu et al., Citation2018). The rooting depth of the soil determines how much water and nutrients are available to plant roots. The majority of research irrigation scheme soils are deep so that the accessibility of those crop nutrients and water is not limited.
In Nitisols, the lower limits of the surface horizon were clear and smooth, and the subsurface horizons were diffused and smooth (Table ); clear and smooth designated the lower border surface horizon of pedon 2 before becoming gradual and smooth at sub-surface horizons. The bottom border of pedon 4’s surface horizon was clear and smooth up to a depth of 30 cm, and below that depth, the subsurface limits were gradual and irregular (Table ).
The Vertisols of the command area are extremely hard when dry and very sticky and very plastic when wet. These soil cracks are widely observed at 8 cm depth during dry conditions. However, upon rewetting, the cracks close again, and as a result of this expansion and contraction, fluted shimmering pedfaces known as slickensides are observed at depths of 70–120 cm. The Vertisols of the command area are predominantly characterized by weak to moderate grade, fine to coarse size, and angular blocky structure type, with prominent color and pressure face features observed with the Vertisols of the command site (Table ).
3.2 Soil physical characteristics
3.2.1 Soil texture
The major particle size distribution of the research site was demonstrated in Table . Clay, sand, and silt percentages were classified by Hazelton and Murphy (Citation2016) as very high, high, moderate, low, and very low categories, respectively, >50, 040–50, 25–40, 10–25, and <10. According to this classification, Nitisols and Luvisols had sand and silt contents that ranged from low to moderate, and clay contents that ranged from very high to moderate, respectively. Vertisols were low in sand, moderate in silt, and high in clay content. Except for pedon 3, the clay content showed increased somewhat with the depth of the profiles. The largest portion of subsurface horizons was found to be argic and created by the eluviation of clay mineral deposits from the outer layers (Dinssa & Elias, Citation2021). This finding was consistent with research by El Ghonamey et al. (Citation2020), who found that clay particles may have been inherited from the parent material among disintegrating and the breakdown of primary minerals. Most of the examined pedons showed a declining tendency in the coarse-size particles namely sand and silt distributions across the soil depth (Table ).
Table 3. Selected soil physical characteristics of the pedons at Soro district
3.2.2 The ratio of silt to clay
The examined pedons’ surface and subsurface soils had silt/clay ratios ranging from 0.12 to 4.82 in pedon 1 and pedon 4, respectively (Table ). This index is crucial to examine the weathering rates of soil and determining the comparative stage of growth of a particular solum (Debele et al., Citation2018). In the four pedons of the research site, there was a definite tendency toward a decline in the clay/silt along the depth of a given soil (Nitisols, Luvisols, and Vertisols). With regard to soil types, the silt/clay showed decreasing order in Vertisols, Luvisols, and Nitisols, respectively (Table ). Ahukaemere et al. (Citation2017) who reported that soils with silt-to-clay ratio of less than 0.15 are deliberated to be greatly weathered soils. Nitisols are weathered soils, whereas Luvisols and Vertisols with silt/clay greater than 0.15 are relatively younger with a greater concentration of weathering likely (Dinssa & Elias, Citation2021). A similar result is reported by Giday et al. (Citation2015) in Southern Tigray and found that silt-to-clay ratios were greater than 0.38.
3.2.3 Bulk density (BD) and total porosity (TP)
In this study, surface horizons BD range from 1.36 g/cm3 to 1.45 g/cm3, being the smallest in pedon 1 and largest in pedon 2. Consequently, the subsurface bulk densities varied from 1.36 g/cm3 to 1.39 g/cm3 (Table ). The BD of the research area was favorable for crop production, which is in line with the finding of Lelago and Buraka (Citation2019), who found bulk densities of the Ambukuna catchment ranged from 1.02 to 1.23 g/cm3. In a similar way, the range values of bulk densities obtained by Hazelton and Murphy (Citation2016) which was 1.3–1.6 g/cm3 were suggested for agricultural production.
In the subsurface soil, the total porosity (TP) ranged from 44.53% (pedon 3) to 49.43% (pedon 1), whereas the TP of the surface soil ranged from 45.66% (pedon 2) to 48.62% (pedon 1). Therefore, the achieved TP amount falls within the usual range reported elsewhere (Hazelton & Murphy, Citation2007). Similar results, where the soil total porosity was less than 50%, were informed by Sebnie et al. (Citation2021) and Fekadu et al. (Citation2018). The recognized soil profiles’ total porosity values in the top horizon and underlying horizons fall within the allowable limit for yields of crops.
3.2.4 Soil moisture content
For pedons 1 and 2, surface soils had a relatively larger water content at FC than underlying horizon soils, whereas the contrary results were obtained for PWP in four pedons. Surface soil at FC of the soils at the research site fluctuated from 124 mm/m at pedon 3 to 299 mm/m at pedon 1, while in the subsurface layers, it ranged from 125 mm/m in pedon 2 to 300 mm/m in pedon 4 (Table ). Pedon 2 had a substantially higher clay content than other pedons, which contributed to its considerably higher water retention (Sebnie et al., Citation2021).
In all soil profiles, the available water content (AWC) had an un-symmetric trend along with depth; this could be due to differences in organic carbon, particle size distribution, soil structure, and rooting depth (Miller & Donahue, Citation1995). AWC values in surface soils ranged from 121 to 184.44 mm/m, whereas values in subsurface soils ranged from 122 to 186 mm/m (Table ).
The highest AWC (184 mm/m) for pedon 4 was most probably due to its lowest sand particle, the maximum silt content, and low bulk density (1.37 g/cm3). Similar to this, according to Reichert et al. (Citation2009), AWC was highest in silt proportion and lowest in sand textural percentage. The quantity of water retained at the permanent wilting point and field capacity was positively correlated with clay content (Mbah, Citation2012; Nagaraju & Gajbhiye, Citation2014). The AWC of the surface and subsurface soils was in the medium range (McIntyre, Citation1974), which is adequate for crop cultivation (Sebnie et al., Citation2021).
3.3 Soil chemical characteristics
3.3.1 Soil pH
The soil pH (H2O) value mostly showed irregular variations with depth in pedons 2 & 3, whereas an increasing trend was observed in pedons 1 and 4. Except for pedon 4, the soil’s surface and subsurface horizons have pH values of less than 7. The pH of pedons 1 and pedon 4 in the topsoil layers ranges from 5.81 to 6.69, respectively (Table ). Due to the utilization of basic cations from crops and the comparatively higher amount of organic matter in the surface horizons, the pH was low. In pedons 1 and 4, respectively, the pH ranges from 5.75 to 8.4 in subsurface horizons. At each site, the surface soils had the lowest pH values, whereas the depths had greater pH (Assen & Yilma, Citation2010; Sharu et al., Citation2013; Yitbarek et al., Citation2018).
Table 4. Soil pH, OC, TN, available P, and EC of the pedons at Soro district
According to Ayalew and Beyene (Citation2012), lower organic matter content with depth results in less H+ ion discharge from the breakdown of organic matter, and a rise in soil pH along soil depth may suggest the existence of vertical explorations of exchangeable bases. The majority of crops can grow in all soil pH levels recorded in the research area (Landon, Citation1991).
3.3.2 Soil organic carbon, total nitrogen, and carbon-to-nitrogen ratio
The organic carbon (OC) content varied along the soil depth at the research location Pedons (Table ). The examined soils’ low levels of organic carbon ranged from 1.71% to 2.31% in the subsurface soils and from 1.82% to 2.54% in the surface soils (Hazelton & Murphy, Citation2016). However, the profiles of all soils showed that the organic carbon content declined along soil depth, demonstrating that top soils obtain more crops left over as litter (Sebnie et al., Citation2021). The reason for the high OC value in the surface soil was the heavy rainfall, which encourages a high level of biomass establishment. In different parts of Ethiopia, low organic carbon values were reported by scholars (Beyene, Citation2017; Fekadu et al., Citation2018; Hailu et al., Citation2015; Zewide et al., Citation2018). The cultivation of crops may have sped up the oxidation of organic matter, and the total clearance of crop residue may have contributed to the study area’s low levels of organic carbon. Additionally, this result is consistent with findings made by Negassa and Gebrekidan (Citation2003), who investigated Ethiopia’s cultivated land had low levels of organic carbon.
The examined surface soils’ TN contents ranged from 0.15% to 0.16%, and the underlying soils for all soil profiles ranged from low to medium (0.09% to 0.15%) (Table ). A significant correlation between TN and soil OC is suggested by the fact that the amount of TN in the surface soil was greater than that in the underlying layers and that it trended similarly to that of OC in all the pedons investigated (Dinssa & Elias, Citation2021). In Pedons 1 and 3, there is a trend toward decreasing total nitrogen along with soil depth. This finding is in line with other scholars who discovered that TN content declined with increasing depth (Mulugeta & Beyene, Citation2010; Sebnie et al., Citation2021; Yitbarek et al., Citation2018).
Likewise, Mulugeta and Beyene (Citation2010) and Hailu et al. (Citation2015) found low TN in southern Ethiopia and Wollo area, respectively. Because there is a lack of livestock feed, it is typical practice in the research area to completely remove crop waste, which reduces the quantity of OM in the top horizon and results in lower total nitrogen levels.
In the top soils, the carbon-to-nitrogen ratio (C/N) ranged from 11.38 in pedon 1 to 16.93 in pedon 2, while in the underlying horizon, it varied from 12.20 in pedon 3 to 19.56 in pedon 4 (Table ). In comparison to their underlying horizons, the C/N ratio values of all the examined pedon surfaces were consistently higher in pedons 1 and 2 as soil depth increased. The research area’s C/N ratio is generally larger than 10:1, which implies a considerably better decomposition rate, improved nitrogen availability to plants, and potential for incorporating agricultural leftovers into the soil (Lelago & Buraka, Citation2019). For both the surface and sub-surface soils, it is less than 24:1, which encourages the net transformation of organic matter by micro-organisms (Sebnie et al., Citation2021). Optimal microbial activity for the humification and hydrolysis of organic wastes is indicated by the research region’s C/N ratio, which is within the range where there is nitrogen excess for microbial demands (Landon, Citation1991).
3.3.3 Available phosphorous
The four pedons of research irrigation schemes’ surface horizons have low levels of available phosphorus (). In the subsurface horizons, the range of available phosphorus was 15.69 to 24.11 ppm, whereas it ranged from 18.52 to 34.50 ppm in the surface soils. The available phosphorus found in all topsoil horizons is rated as having medium to high levels (Olsen et al., Citation1954). This finding is in line with Debele et al. (Citation2018) in Muger sub-watershed, which found that the available phosphorus ranged from medium to high. Due to the diminishing soil OM content, the available phosphorus showed a declining tendency for all soil profiles across the depth of the soil (Ayalew et al., Citation2015; Debele et al., Citation2018). This conclusion is in line with that of Ali et al. (Citation2010), who found that the surface horizon had the maximum concentration of soil’s available phosphorus. Similarly, Ayalew (Citation2016) in selected areas of southern Ethiopia reported that the surface soils’ available phosphorus content was medium (5–15 mg/kg).
3.3.3 Electrical conductivity
Subsurface soils in Pedon 4 had the greatest electrical conductivity (EC) value of 0.22 dSm−1, while surface soils in Pedons 1 and 2 had the lowest value (0.04 dSm−1) (Table ). All the surface and subsurface EC values are less than 2 dSm−1 revealing that the soils were non-saline. The electrical conductivity content determined in the examined soils showed that the contents of soluble salts are lower than the levels at which most crops do not experience any negative effects from soil salinity on growth and productivity (Landon, Citation1991; Sebnie et al., Citation2021).
3.3.4 Exchangeable bases, base saturation, and cation exchange capacity
In decreasing sequence, Ca2+ > Mg2+ > K+ > Na+ were the predominant cations filling the exchange sites. With increasing soil depth in pedons 1 and 3, the calcium (Ca2+) concentration dropped consistently (Table ). The surface layer of Pedon 4 (Vertisols) had the uppermost Ca2+ value (18.18 cmol(+) kg−1) while Pedon 2 (Luvisols) had the lowest (14.91 cmol(+) kg−1). Across all profiles, there were no discernible trends in the value of exchangeable calcium related to soil depth. This can be a result of the parent materials that contain calcium being distributed irregularly throughout the soil profiles (Sebnie et al., Citation2021). The pH value of 5 to 9 was the most ideal pH assortment for the obtainability of Ca2+, and the largest concentration of Ca2+ indicated the status of weathering (Dinssa & Elias, Citation2021; Hazelton & Murphy, Citation2016).
Table 5. CEC, exchangeable bases, and BS percent of Soro district
In the surface horizons, the exchangeable Mg2+ contents ranged from 4.31 to 6.72 cmol(+) kg−1 in pedon 4 and pedon 2, respectively, while in the underlying layers, they ranged from 3.9 to 13.85 cmol(+) kg−1 in pedon 2 and pedon 4, respectively (Table ). According to Hazelton and Murphy (Citation2016), the exchangeable magnesium content of the soil pedons at the study site ranged from low to high.
The exchangeable K+ concentrations in the surface horizon were lowest in pedon 3 (0.61 cmol(+)kg−1) and greatest in pedon 2 (3.78 cmol(+)kg−1). In the underlying layer, the smallest K+ concentration (0.46 cmol(+)kg−1) was reported under pedon 2 (Luvisols), and the greatest (5.12 cmol(+)kg−1) was obtained in pedon 4 (Vertisols).
The exchangeable K+ fluctuated from medium to very high in top soils and varies from very low to very high in the underlying horizons of all pedons (Hazelton & Murphy, Citation2016), and its variation along soil depth is not uniform. Hence, the exchangeable potassium does not influence crop cultivation in the research area. Due to the differences in the parent material and the substantial rainfall, exchangeable sodium (Na+) is not accumulated in the examined soils since it is present at low concentrations across the profiles of the soils.
The highest CEC values in the surface soils (42.77 cmol (+)/kg) and the lowest (35.67 cmol (+)/kg) were found in pedon 1 and pedon 3, respectively. In the subsurface, CEC ranged from 40.16 cmol (+)/kg in pedon 3 to 64.30 cmol (+)/kg in pedon 4 (Table ). The CEC values of the soils that had been studied were typically in the medium to extremely high range (Hazelton & Murphy, Citation2016) indicating strong nutrient retention and buffering capability. However, the cation exchange capacity under the four pedons showed an unsystematic tendency along soil depths.
The surface horizons’ Ca:Mg ratios range from 2.2:1 to 4:1, with pedons 2 and 3, respectively, falling within the (1–4:1) range except pedon 4 which has a ratio greater than 4:1 (Table ). The pedons 1 and 2 of the surface soils, according to Hazelton and Murphy (Hazelton & Murphy, Citation2016), had a Ca:Mg ratio that was less than 4:1, which resulted in a restricted supply of Ca (Hazelton & Murphy, Citation2016). This could be due to a lack of Ca absorption brought on by an excess of Mg or the excessive rain washing out the basic cations (Sebnie et al., Citation2021). In the research area, it is unlikely that exchangeable base values will constrain crop growth and production.
The surface layer of pedon 3 had the greatest BS value (61.99%), whereas pedon 1’s surface horizon had the lowest value (56.96%). Pedon 4 had the highest base saturation percentage (95.28%) and pedon 2 had the lowest (49.61%) in the subsurface soils (Table ). Except for Pedon 2, all analyzed profiles had base saturation percentages for all horizons of soils that were above 50% and graded as moderate to extremely high (Hazelton & Murphy, Citation2016). The leaching of basic cations by rainfall was determined by variation in BS and utilized as a diagnostic characteristic to categorize soils (Meena, Citation2014).
3.4 Relationship among various soil properties
The analysis’s findings revealed that while some soil characteristics did not exhibit links among themselves, others did exhibit substantial relationships with one another. Significant correlations were found between the following factors: total porosity with FC, PWP, pH, and exchangeable bases (Ca, Mg, and K), whereas TN with AWC and PWP and pH with EC and CEC were positively correlated (Table ), respectively. On the contrary, clay with OC and TN; BD with pH, EC, CEC, Ca, and Mg; TP with pH, EC, BS, K, and BD were correlated negatively (Table ).
Table 6. Correlation analysis for physical and chemical properties of soils in Soro district
3.5 Soil classification
3.5.1 Soil classification based on WRB legend
Pedon 1 was characterized as being at the top slope, deep, with clay content and a strongly developed angular blocky structure, >30% clay, and a silt/clay of 0.4. It was also described as being medium in OC. These characteristics of the pedon meet the requirements for the nitric underlying layer diagnostic criteria. Additionally, soil that had a weighted average of greater than 1% soil organic carbon, 50 cm below the mineral soil surface, qualified for humic. The use of the Eutric additional qualifier is required when the BS is greater than 50% and is between 20 and 50 cm below the surface. Accordingly, the soil is categorized as Eutric Nitisols (Humic) which is presented in Figure .
Pedon 2 was described as being located on farmed land in the middle of the slope. The subsurface included more clay than the surface soils, and an alluvial deposit of clay created an argic subsoil horizon. As a reference soil group, Luvisols are defined as soils with high activity clays (CEC >24 cmol (+) kg−1) throughout the argic horizon and high base saturation in the depth range of 50–100 cm. The soils have a layer that is 30 cm thick, with a Munsell color hue redder moist and a value of 4 (moist) prefixed as Rhodic, between 25 and 150 cm below the soil surface. Nevertheless, the application of the Eutric extra qualifier to designate the soil as Rhodic Luvisols (Eutric) is necessitated by the existence of a BS of >50%, between 20 and 50 cm from the surface soil.
On a medium slope of cultivated ground, pedon 3 was dug up 4% slope, 160 cm of very deep effective soil depth, and moderate to good drainage are all present. When the ratio of clay in the top layer to the overlying horizon is more than 1.2, it indicates the presence of an argic horizon. The pedon has a subterranean layer with higher clay than an overlaying layer. This is due to the illuvial buildup of clay, pedogenetic creation of clay in the subsoil, and selective removal of clay. Additionally, the entire argic horizon contains CEC bigger than 24 cmol(+) kg−1. Based on these standards, the soil satisfied the necessities for the Luvisols reference soil group. This pedon was categorized as Haplic Luvisols (Humic) due to its high base saturation (up to 83%).
Pedon 4 was described as being situated on cultivated land that was on a moderate slope. It has a 160 cm effective soil depth, clays that swell and expand through wet and dry seasons, and cracks that regularly open and close. Additionally, it has a vertic layer that begins within 100 cm of the soil’s surface and contains more than 30% clay. The soils represented by this pedon meet the criteria for Vertisols when taking into account the aforementioned factors. Vertisols with a Munsell color value of 3 and a Chroma of 2, both moist, are found in the upper 30 cm of the soil and are prefixed with the pellic main qualifier. The pedon matched the criteria for the hypereutric suffix qualifier and had a base saturation of more than 80% throughout the lower subsurface horizon. The soils were categorized as Pellic Vertisols (hypereutric).
3.5.2 Soil classification based on soil taxonomy
Pedon 1 had had argillic diagnostic horizons with a base saturation (by sum of cations) of less than 35%, and hence categorized under the order Ultisols. The pedons were further grouped under Ustults at suborder level due to their ustic soil moisture regime and Haplustults and Typic Haplustults, at great group and subgroup levels, respectively.
Pedons 2 and 3 also had argillic diagnostic horizons with a base saturation (by sum of cations) of greater than 35%, and hence categorized under the order Alfisols (Soil survey Staff, Citation2014). The pedons were further grouped under Ustalfs at suborder level due to their ustic soil moisture regime and Haplustalfs and Typic Haplustalfs, at great group and subgroup levels, respectively
Pedon 4 had 30% and more clay, exhibiting slickensides and cracks that open and close periodically. Thus, the pedons were classified under Vertisols. If not irrigated during the year, the cracks remained opened for 90 or more cumulative days per year, qualifying them for the Usterts suborder; and Haplusterts and Typic Haplusterts for the great group and subgroup, respectively.
4. Conclusion
For the purpose of creating efficient land use plans, managing soil fertility, and increasing agricultural crop output exhaustive evidence on the soil parameters used for soil characterization and categorization is crucial. To estimate productivity, enable knowledge transfer, and increase information sharing among soil professionals, decision-makers, designers, academicians, and agricultural workers, soil classification is useful.
It is extremely significant and has proven crucial to building an efficient land management approach for boosting agricultural productivity on an ecological balance where soil resources are systematically assessed and understood concerning their scope, dissemination, and features.
Based on their morphological, physical, and chemical characteristics, the soils in this study were categorized as follows: pedon 1, Eutric Nitisols (Humic); pedon 2, Rhodic Luvisols (Eutric); pedon 3, Haplic Luvisols (Humic); and pedon 4, Pellic Vertisols (Hyperdystric) according to WRB and with their USDA Soil Taxonomy equivalent as Ultisols (Typic Haplustults), Alfisols (Typic Haplustalfs) and Vertisols (Typic Haplusterts).
Overall, the differences in topography, soil depth, and among soils of the irrigation scheme are morphological, physical, and chemical indicators of the existence of several elements that influence soil development. The re-establishment and augmentation of the OM and TN values of the soils should be the main goals of soil productivity management techniques. The use of appropriate organic and inorganic fertilizers should also be raised and spread out over different terrains, supported by activities that conserve soil and water and are dependent on the amount of soil fertility specific to each site.
In addition to these soils’ chemical and physical characteristics, erosion is a risk in the examined area. It has been discovered that some irrigation scheme locations experience sheet and rill erosion, a moderate-to-severe erosion danger. The danger of soil erosion is more considerable in the Nitisols and Luvisols of the study area occurring mainly on gentle and undulating slopes due to its sloping topographic nature
The results revealed that the soil had low total nitrogen, available phosphorus, and organic carbon. Therefore, organic fertilizer addition should be the primary goal of agricultural land management, and crop residue removal should be avoided. Hence, the overall soil nutrient status of all the soils (Nitisols, Vertisols, and Luvisols) of the command area indicates that a soil constraint related to nutrient availability will be the limiting factor of the soils in the future expansion of the proposed irrigation development. Besides, surface drainage problems (such as Vertisols) and erosion hazards (particularly in Nitisols) resulting from terrain of command area, the project command area could be limited if its slope greater than 5%.
The application of fertilizers and manure is required to replenish nutrients depleted from the soil by crops to maintain soil fertility. Even in soil that is extremely productive, nutrient reserves are depleted as crops are continuously grown and harvested, necessitating replacement. Mulching of crop residues after harvesting should be used, together with the use of manure and compost, to maintain organic carbon.
Utilizing plant varieties that can fix atmospheric nitrogen can increase soil fertility and lessen a region’s reliance on artificial fertilizers. Additionally, modern agricultural techniques like crop rotation and the use of green manure offer an additional source of nutrients for plant growth. It is advised to rotate your crops because doing so will increase the productivity of the soil.
Author contributions
All authors contributed to the different stages of this study. MM and MK were involved in the design of the study, investigation, and Methodology. MK has participated in data collection, analysis, writing—review & editing of the manuscript. MM has made substantial contributions in reviewing & editing the manuscript. All authors have read and agreed to the published version of the manuscript.
Acknowledgements
The authors would like to acknowledge the South Design, Construction, and Supervision Enterprise for financial support of data collection and soil laboratory analysis.
Disclosure statement
No potential conflict of interest was reported by the author(s).
Data availability statement
The data presented in this study are available on request from the first author.
Additional information
Funding
References
- Abate, N., Kibret, K., Gebrekidan, H., & Esayas, A. (2014). Characterization and classification of soils along the toposequence at the wadla delanta massif, north central highlands of Ethiopia. Journal of Ecology and the Natural Environment, 6(9), 304–16. https://doi.org/10.5897/JENE2014.0463
- Abebe, F., (1988). Need for soil survey studies. In: D. Beyene (Ed.), Soil Science Research in Ethiopia: A Review. In: Proceedings of the First Soil Science Research Review Workshop 11-14 February 1986. Addis Ababa, Ethiopia.
- Ahukaemere, C. M., Osujieke, D., & Ndukwu, B. (2017). Horizon differences in micronutrient contents of soils of the coastal plain sands in Imo state, South-East Nigeria, micronutrient contents of pedons formed under coastal plain sands. Bulgarian Journal of Soil Science, 2(2), 112–122.
- Alemu, L., & Buraka, T. (2018). Soil classification and agricultural potentials of soils of Tembaro district, Kembata Tembaro Zone, Southern Ethiopia. Environment Science, 10(6), 75–91.
- Ali, A., Esayas, A., & Beyene, S. (2010). Characterizing soils of Delbo Wegene watershed, Wolaita Zone, Southern Ethiopia for planning appropriate land management. Journal of Soil Science and Environmental Management, 1(8), 184–199.
- Assen, M., & Yilma, S. (2010). Characteristics and classification of the soils of gonde micro-catchment, Arsi highlands, Ethiopia. Ethiopia Journal of Science, 32(2), 101–116.
- Ayalew, A. (2016). Assessment of soil fertility status of different types of soils in selected areas of Southern Ethiopia. Journal of Natural Sciences Research, 6(1), 105–110.
- Ayalew, A., & Beyene, S. (2012). Characterization of soils at Angacha district in Southern Ethiopia. Journal of Biology, Agriculture and Healthcare, 2(1), 6–17.
- Ayalew, A., Beyene, S., & Walley, F. (2015). Characterization and classification of soils of selected areas in Southern Ethiopia. Journal of Environment and Earth Science, 5(11), 116–137.
- Beyene, S. (2017). Topographic positions and land use impacted soil properties along Humbo Larena-Ofa Sere. Journal of Soil Science and Environmental Management, 8(8), 135–147. https://doi.org/10.5897/JSSEM2017.0643
- Blake, G. R., & Hartge, K. H. (1986). Bulk Density. In A. Klute (Ed.), Methods of soil analysis, Part 1—Physical and Mineralogical Methods, 2nd Edition, Agronomy Monograph 9 (pp. 363–375). American Society of Agronomy—Soil Science Society of America.
- Bouyoucos, G. J. (1962). Hydrometer method improved for making particle size analyses of soils 1. Agronomy Journal, 54(5), 464–465. https://doi.org/10.2134/agronj1962.00021962005400050028x
- Bray, R. H., & Kurtz, L. T. (1945). Determination of total, organic and available forms of phosphorus in soils. Soil Science, 59(1), 39–46. https://doi.org/10.1097/00010694-194501000-00006
- Buol, S. W., Graham, R. C., McDanieL, P. A., & Southard, R. J. (2011). Soil genesis and classification. John Wiley & Sons.
- Buraka, T., & Lelago, A. (2016). Physico-chemical properties and agricultural potentials of soils of Tembaro Woreda, Kembata Tembaro Zone, Southern Ethiopia. Chemistry and Materials Research, 8(7), 64–75.
- Debele, M., Bedadi, B., Beyene, S., & Mohammed, M. (2018). Characterization and classification of soils of muger sub-watershed, Northern Oromia. East African Journal of Sciences, 12(1), 11–28.
- Dengiz, O., Saglam, M., Sarioglu, F. E., Saygin, F., & Atasoy, C. (2012). Morphological and physico-chemical characteristics and classification of vertisol developed on deltaic plain. Open Journal of Soil Science, 2(1), 20. https://doi.org/10.4236/ojss.2012.21004
- Deressa, A., Bote, B., & Legesse, H. (2013). Evaluation of soil cations in agricultural soils of east wollega zone in south western Ethiopia. Science, Technology and Arts Research Journal, 2(1), 10–17. https://doi.org/10.4314/star.v2i1.98836
- Dessalegn, D., Beyene, S., Ram, N., Walley, F., & Gala, T. S. (2014). Effects of topography and land use on soil characteristics along the toposequence of ele watershed in southern Ethiopia. Catena, 115, 47–54. https://doi.org/10.1016/j.catena.2013.11.007
- Dinssa, B., & Elias, E. (2021). Characterization and classification of soils of Bako Tibe district, West Shewa, Ethiopia. Heliyon, 7(11), e08279. https://doi.org/10.1016/j.heliyon.2021.e08279
- El Ghonamey, Y., Abdellatif, A., & Abdel Ghaffar, M. (2020). Soil mineralogy of North Western desert, Egypt. Egyptian Journal of Soil Science, 60(4), 485–500.
- Elias, E. (2017). Characteristics of Nitisol profiles as affected by land use type and slope class in some Ethiopian highlands. Environmental Systems Research, 6(20), 1–15. https://doi.org/10.1186/s40068-017-0097-2
- Erango, E., Bersisa, M., Shewangizaw, M., Etensa, T., & Tolera, T. (2017). Economic valuation of soil conservation in highlands of Hadiya Zone of Ethiopia: The case of Soro Woreda Ajacho Watershed. Journal of Resources Development and Management, 37(10), 16–23. https://doi.org/10.7176/JRDM
- FAO. (2006). Guidelines for soil description (4th ed.). Food and Agriculture Organization of the United nations.
- Fekadu, E., Kibret, K., Bedadi, B., & Melese, A. (2018). Characterization and classification of soils of yikalo subwatershed in Lay Gayint district, northwestern highlands of Ethiopia. Eurasian Journal of Soil Science (EJSS), 7(2), 151–166. https://doi.org/10.18393/ejss.376267
- Getahun, M. (2015). Characterisation of agricultural soils in CASCAPE intervention woredas of Amhara region. Bahir Dar, No. BDU-CASCAPE working paper 12.
- Giday, O., Gibrekidan, H., & Berhe, T. (2015). Soil fertility characterization in vertisols of southern. Advances in Plants & Agriculture Research, 2(1), 7–13. https://doi.org/10.15406/apar.2015.02.00034
- Hailu, A. H., Kibret, K., & Gebrekidan, H. (2015). Characterization and classification of soils of Kabe Subwatershed in South Wollo Zone, Northeastern Ethiopia. African Journal of Soil Science, 3(7), 134–146.
- Hazelton, P., & Murphy, B. (2007). Interpreting soil test results: What do all the numbers mean?. CSIRO Publishing.
- Hazelton, P., & Murphy, B. (2016). Interpreting soil test results what do all the numbers mean? European Journal of Soil Sciences, 58(5), 1219–1220. https://doi.org/10.1111/j.1365-2389.2007.00943_8.x
- IUSS Working Group WRB. (2015). World reference base for soil resources 2014, update 2015 international soil classification system for naming soils and creating legends for soil maps. World soil resources reports(Vol. 106, p. 203). FAO.
- Jackson, M. L. (1958). Soil chemical analysis. Prentice-Hall Inc.
- Landon, J. R. (1991). Booker tropical soil manual: A handbook for soil survey and agricultural land evaluation in the tropics and subtropics (2nd ed.). Longman Scientific and Technical.
- Lelago, A., & Buraka, T. (2019). Determination of physico-chemical properties and agricultural potentials of soils in Tembaro District. KembataTembaro Zone Eurasian Journal of Soil Science, 8(2), 118–130. https://doi.org/10.18393/ejss.533454
- Lufega, S., & Msanya, B. (2017). Pedological characterization and soil classification of selected soil units of Morogoro district, Tanzania. International Journal of Plant & Soil Science, 16(1), 1–12. https://doi.org/10.9734/IJPSS/2017/32681
- Mbah, C. (2012). Determining the field capacity, wilting point and available water capacity of some Southeast Nigerian soils using soil saturation from capillary rise. Nigerian Journal of Biotechnology, 24(35), 41–47.
- McIntyre, D. S. (1974). Water retention and moisture characteristics. In J. Love Day. (Eds.), Methods for analysis of irrigated soils (pp. 43–62).
- Meena, R. S. (2014). Characterization and classification of lowland soils of Chikkarsinkere Hobli, maddur taluk, Mandya district of Karnataka. Agropedology, 24(1), 95–101.
- Mengistu, A. (2003). Country pasture/forage resources profiles: Ethiopia. Food and Agriculture Organization of the United Nations (FAO).
- Miller, R. W., & Donahue, R. L. (1995). Soils in our environment (7th ed.). Prentice-Hall Inc.
- Moshago, S., Regassa, A., & Yitbarek, T. (2022). Characterization and classification of soils and land suitability evaluation for the production of major crops at Anzecha Watershed, Gurage Zone, Ethiopia. Applied & Environmental Soil Science, 2022, 1–22. https://doi.org/10.1155/2022/9733102
- Mulugeta, D., & Beyene, S. (2010). Characterization and classification of soils along the toposequence of Kindo Koye watershed in Southern Ethiopia. East African Journal of Sciences, 4(2), 65–77. https://doi.org/10.4314/eajsci.v4i2.71528
- Munsell, S. (2000). Munsell soil colour company. Gretagmacbeth.
- Nagaraju, M. S. S., & Gajbhiye, K. S. (2014). Characterization and evaluation of soils of Kukadi Command (minor-25) in Ahmednagar district of Maharashtra for land resource management. Agropedology, 24(2), 157–165.
- Negassa, W., & Gebrekidan, H. (2003). Influence of land management on morphological, physical and chemical properties of some soils of Bako, Western Ethiopia. Agropedology, 13(2), 1–9.
- Okalebo, J. R., Gathua, K. W., & Womer, P. L. (2002). Laboratory methods of soil and plant analysis: A working manual (2nd ed.). TSBF.
- Olsen, S. R., Cole, C. V., Watanabe, F. S., & Dean, L. A. (1954). Estimation of available phosphorus in soils by extraction with sodium bicarbonate (Vol. 939). US Department of Agriculture.
- Onyekanne, C. F., Akamigbo, F. O., & Nnaji, G. U. (2012). Characterization and classification of soils of Ideato North local government area. Nigerian Journal of Soil Science, 22(1), 11–17.
- Reichert, J., Albuquerque, J., & Rodrigo, D. (2009). Estimation of water retention and availability in soils of Rio Grande do sul. Rev Bras Ciênc Solo, 33(6), 1547–1560. https://doi.org/10.1590/S0100-06832009000600004
- Richards, L. (1965). Part 1 Physical and Mineralogical Properties, including Statistics of Measurement and Sampling (Vol. 9, pp. 128–152). American Society of Agronomy.https://doi.org/10.2134/agronmonogr9.1
- Sanchez, P. A., Palm, C. A., & Buol, S. W. (2003). Fertility capability soil classification: A tool to help assess soil quality in the tropics. Geoderma, 114(3), 157–185. https://doi.org/10.1016/S0016-7061(03)00040-5
- SAS. (2003). Statistical analysis system (version 9.1). SAS Institute.
- Schoonover, J. E., & Crim, J. F. (2015). An introduction to soil concepts and the role of soils in watershed management. Journal of Contemporary Water researCh & Eduacation, 154(1), 21–47. https://doi.org/10.1111/j.1936-704X.2015.03186.x
- Sebnie, W., Adgo, E., & Kendie, H. (2021). Characterization and classification of soils of zamra irrigation scheme, Northeastern Ethiopia. Air, Soil and Water Research, 14, 1–12. https://doi.org/10.1177/11786221211026577
- Sharu, M. B., Yakubu, M., Noma, S. S., & Tsafe, A. I. (2013). Characterization and classification of soils on an agricultural landscape in Dingyadi district, Sokoto state, Nigeria. Nigerian Journal of Basic and Applied Science, 21(2), 137–147. https://doi.org/10.4314/njbas.v21i2.9
- Soil survey staff. (2014). Keys to soil taxonomy (12th ed.). United States Department of Agriculture.
- Van Reeuwijk, L. (2002). Procedures for soil analysis (6th ed.). ISRIC, FAO.
- Walkley, A., & Black, I. A. (1934). An examination of the degtjareff method for determining soil organic matter and a proposed modification of the chromic acid titration method. Soil Science, 37, 29–38. https://doi.org/10.1097/00010694-193401000-00003
- Yacob, A., & Nigussie, A. (2022). Characterization and classification of soils along the toposequence of medo sub-watershed at Wondo Genet. International Journal of Natural Resource Ecology and Management, 7(2), 73–85.
- Yitbarek, T., Beyene, S., & Kibret, K. (2016). Characterization and classification of soils of Abobo area, Western Ethiopia. Applied and Environmental Soil Science, 2, 1–16. https://doi.org/10.1155/2016/4708235
- Yitbarek, T., Jembere, A., & Kerebeh, H. (2018). Characterization and classification of soils of Wolkite university research sites, Ethiopia. Eurasian Journal of Soil Science (EJSS), 7(4), 292–299. https://doi.org/10.18393/ejss.436186
- Zewide, I., Tana, T., L, W., & Ibrahim, M. (2018). Soil morphology, physico-chemical properties and classification of typical soils of Abelo Area Masha district South Western Ethiopia. Advances in Crop Science and Technology, 6(2), 1–9. https://doi.org/10.4172/2329-8863.1000341