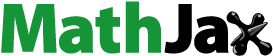
ABSTRACT
Due to the numerous infrastructure development projects taking place in developing nations, large amounts of construction and demolition waste (C&DW) are unavoidable. Most C&DW management strategies, however, involve ‘isolated’ operations with high overhead. Waste data transfers are difficult to watch over. Important information needed for decision-making is, therefore, challenging to obtain. This paper’s goal is to discuss C&DW management from an integrative standpoint. Using GIS functions and a fuzzy analytical hierarchy approach, the best sites for waste management facilities can be found. A mixed integer optimization model is created to identify optimal location and allocation of waste management facilities. The objective is to reduce waste collection costs under different operational scenarios. By incorporating ‘constructs elements’ and ‘connected elements’ that are linked, isolated C&DW management ventures are transformed into successful C&DW management ventures. The effectiveness and efficiency of waste management strategies are evaluated using GIS analysis. A dashboard for tracking waste data flows is created to show how stakeholder decision making can be made easier. The proposed integrative approach differs from other approaches since it re-purposes location-allocation models and GIS data to optimize ‘isolated’ C&DW management practices. In addition, the notion of ‘construct elements’ that can be time-shared is outlined. Moreover, the notion of ‘connected elements’ that are dynamic, time-limited, and yet transient enough to provide the necessary interconnectivity is developed to support and complement the seamless function of a network of C&DW management facilities. The usefulness of the integrative approach is illustrated by a case study. Analysis of case studies demonstrates that waste management strategies in developing countries can be made more effective and waste collection efficiency can be increased. According to comparative studies, updating current waste management facilities can cut operating costs by 26%, while implementing an integrative strategy can cut costs by 52%. The discussions in this paper can help towns reevaluate C&DW management and put useful, unified C&DW management techniques into practice.
1. Introduction and background
Construction projects are significant economic activities that generate substantial amounts of construction and demolition waste (Aslam et al., Citation2020; Bao & Lu, Citation2020; Kabirifar et al., Citation2021). This trend is unavoidable since the amount of waste generated is usually related to the expansion of national economies (Islam et al., Citation2019; Manowong, Citation2012; Urio & Brent, Citation2006; Wang et al., Citation2022; Yuan, Citation2013). For example, a quick observation shows that this trend is true especially in quickly developing countries like Qatar, where most of the construction and demolition waste is generated by multiple construction projects running concurrently (Egbelakin et al., Citation2021). These projects are typically undertaken to address, (i) infrastructure development, (ii) rising urbanization, and (iii) the requirement to satisfy strategic development objectives (Bao & Lu, Citation2020). Despite this, it is critical to monitor the development, storage, and disposal of construction and demolition waste (C&DW) to prevent the negative repercussions and burdens associated with a reactive approach to C&DW management (Zhao, Citation2021). While some of the cities in developing countries aspire quick upgrades to smart city developments, it is critical to balance infrastructural development and construction waste management to avoid, for example, visual effects of waste generation and accumulation—a situation that is not amenable to urbanization and smart city development (Bao et al., Citation2023; Szpilko et al., Citation2023).
In the published literature, researchers have developed a waste hierarchy to help in positioning waste management strategies towards sustainable waste management systems (Kabirifar et al., Citation2020, Citation2021; Mahayuddin et al., Citation2008; Manowong, Citation2012; Urio & Brent, Citation2006; Zhang et al., Citation2022). These strategies can help in curbing the rising volumes and complexity of generated waste. The increase in the volume and complexity of waste implies that waste prevention and reduction strategies are not effectively implemented (Fitri et al., Citation2019; Shahid et al., Citation2023; Urio & Brent, Citation2006). Nevertheless, lack of effective C&DW management strategies in developing countries is most probably due to lack of funding mechanisms that leave construction contractors with profit challenges against investments in effective waste management practices. With contractors focusing more on business and profit, waste generation will continue to escalate until or unless sustainable integrated solutions are put in place.
In cases of countries that can afford to implement sound sustainable waste management practices, the reason for increases in volume of waste generated could be lack of understanding of concepts in the high end of the waste hierarchy or lack of enough case studies that thoroughly address specific issues and barriers to effective implementations of waste management principles (Lu et al., Citation2021; Manowong, Citation2012, Yuan Citation2013; Kabirifar et al., Citation2021). It could also be due to waste management deficiencies and/or lack of proper governance (Aslam et al., Citation2020). Albeit it all boils down to implementing effective waste management principles and strategies (Kabirifar et al., Citation2020; Wu et al., Citation2020). The starting point would be to identify the critical success factors in C&DW management and use these factors as countermeasures to develop sound waste management strategies (Lu et al., Citation2021; Newaz et al., Citation2022; Oluleye et al., Citation2022).
Poor waste management strategies often result in environmental problems in addition to human health and safety. For example, it is common practice in developing countries to dump C&DW in illegal dump sites, riverbanks, and landfills (Abdelhamid, Citation2014; Mahayuddin et al., Citation2008; Ramos and Martinho, Citation2023; Thives et al., Citation2022). These practices are usually the cause of water and soil pollution, which eventually affect the environment and/or human health and safety (Alsheyab, Citation2022; Chen et al., Citation2021; Liu et al., Citation2020). Therefore, proper waste management plans at construction sites, municipal and national levels should be developed and implemented to avoid the pitfalls of pollution (Ramos et al., Citation2023).
In most developing countries, disposal methods, techniques, and tools for C&DW are not properly developed (Rondinel-Oviedo, Citation2023). Little governance and less stringent legislation will mean that C&DW will continue to rise to unacceptable levels (Eghbali-Zarch et al., Citation2022; Huang et al., Citation2018; Manowong, Citation2012). This is even more complicated by inadequate waste management facilities and inefficient waste collection mechanisms and networks (Hu, Citation2011; Manowong, Citation2012).
Another prominent challenge is how to manage C&DW in a more sustainable manner to accrue social, environmental, and economic benefits (Al-Otaibi et al., Citation2022; Hasan et al., Citation2023; Manowong, Citation2012; Ratnasabapathy et al., Citation2019). One solution could be to apply principles of sustainable C&DW waste management as discussed in (Al-Otaibi et al., Citation2022; Cheng et al., Citation2022; Ramos et al., Citation2023). Another solution would be to view waste management in the perspective of circular economy principles as discussed in (Zhang et al., Citation2022; Nadazdi et al., Citation2022, Bao Citation2023, Bao et al, Citation2023). Albeit sustainable C&DWM and circularity of C&DWM can be factored into the development and implementation of integrated waste management systems. Such systems can go a long way in positioning waste management practices towards leading value indicators in C&DWM practices.
Proactive approaches can also help in implementing sound waste management strategies (Aslam et al., Citation2020; Banawi et al., Citation2020). A proactive strategy entails rethinking the function of waste management facilities by (a) improving facilities to encourage waste management integration and (b) upgrading facilities to improve connectivity. One way of realizing such changes is to integrate multiple smart digital technologies into waste management practices (Hu et al., Citation2022; Sartipi, Citation2020; Sepasgozar et al., Citation2021). These technologies can be harnessed to enable implementation of integrated C&DW management systems through digitalization and automation (Jain et al., Citation2023; Rahigude et al., Citation2022). This is expected to provide benefits such as waste estimation, waste reporting, data management, and waste diversion—all of which are currently missing in most C&DW management ventures in developing countries (Jain et al., Citation2023; Rahigude et al., Citation2022; Ratnasabapathy et al., Citation2019).
Unlike municipal solid waste management, smart digital technology applications in C&DW management are relatively few and most reported applications are at prototype stages (Hu et al., Citation2022; Kang et al., Citation2022; Ratnasabapathy et al., Citation2019; Sartipi, Citation2020; Sepasgozar et al., Citation2021). However, a prominent challenge in implementing smart digital technologies in developing countries lies in that most of the nations are still struggling to realize a significant level of digitalization.
For example, it has been shown that smart BIM, i.e. BIM coupled with smart digital technologies can be effective in managing demolition waste by providing quantitative assessment of waste, enabling demolition process planning, and identifying optimal disposal route selection (Kang et al., Citation2022). However, very few developing countries are fully implementing BIM in construction projects thus nullifying implementation of smart BIM.
Other supporting digital technologies on the market include sensors, actuators, the internet of things (IoTs), Industry 5.0 and 5 G networks. Research has shown that these technologies can be harnessed in one way or another to provide a fully automated C&DW management solution with benefits (Hu et al., Citation2021; Islam et al., Citation2019; Khoshand et al., Citation2020; Sartipi, Citation2020; Sepasgozar et al., Citation2021).
Despite the contribution that smart digital technologies can provide, most C&DW management strategies in developing countries do not utilize them. In addition, most C&DW management practices can be characterized as ‘isolated’ ventures that lack connectivity, communication, and connectedness. Nevertheless, simple inexpensive technologies such as sensors, actuators, common operating systems such as windows 11, and IT networking through the internet can be configured to enable and support development of improved C&DW management systems. As such, smart digital technologies can provide the missing links in ‘isolated’ ventures that can improve and advance C&DW management strategies in developing countries.
Isolated ventures miss out on the benefits of integrated C&DW management systems, such as (a) high system level effectiveness of networked facilities, (b) high operating efficiencies, and (c) lower total operating costs. Total operating costs can be lowered due to economies of scale that allow for waste management facility sharing and re-invention (Purchase et al., Citation2021; Yeheyis et al., Citation2013). Furthermore, because of ‘disconnects’ inherent in isolated waste management practices, it is difficult to track, analyze, and manage waste data. As a result, despite the availability of methodologies for estimating waste data quantities, C&DW data flow patterns are poorly understood and waste quantities are not exactly known (Guerra et al., Citation2019, Citation2020; Islam et al., Citation2019). This leads to sub-optimal operations with low operating efficiencies.
One of the reasons for low operating efficiencies is lack of waste data for stakeholder decision making in addition to isolated waste management practices that lack communication, interconnectedness, and interconnectivity (Gupta et al., Citation2022; Rondinel-Oviedo, Citation2023; Zeller et al., Citation2019). Besides improving communication, interconnectedness, and interconnectivity, digital technologies can play a critical role in providing and tracking the required waste flow data thereby advancing C&DW management practices.
While implementing integrated C&DW management systems can be too expensive for some developing countries, this paper describes how a set of simple, low-cost tools can be used to position C&DW management practices on a route to integrated C&DW management systems.
On another note, development of integrated waste management systems requires applications of a number of tools. One of the popular tools in waste management is location-allocation models for finding the optimal number and optimal location of waste management facilities (de Souza Melaré et al., Citation2017; Lin, Citation2020; Tirkolaee et al., Citation2020).
Despite the importance of these tools, most location-allocation solutions in published literature are mathematical in nature, necessitating greater research and understanding before they can be put into practice. Most of the time, these technologies do not offer enough information to directly affect waste management procedures (Bolouri et al., Citation2020; Duan et al., Citation2019; Paz et al., Citation2018).
Despite these shortcomings, optimal waste management facility location and allocation have an impact on several factors, including: (a) waste collection efficiency, (b) waste management effectiveness, (c) socioeconomic factors related to site location, (d) environmental factors related to site location, and (e) operating cost factors (Yazdani et al., Citation2020; Yu & Solvang, Citation2017).
To improve the practical significance of location allocation models, several researchers have used GIS analytic tools and improvements of the practicality of solutions have been reported (Bolouri et al., Citation2020; Li et al., Citation2020; Madi & Srour, Citation2019; Vu et al., Citation2018). This is because the optimal location and allocation of waste management facilities in the GIS environment considers practical issues of concern that impact efficiency and effectiveness by taking into account the presence of existing road networks, locations and relative locations of existing structures, buildings, and other infrastructures (ESRI, Citation2015, Citation2020).
Besides GIS functions and tools, Multi-Criteria Decision Analysis (MCDA) is another technique widely used for waste management analysis. This technique has been used to gather expert opinions in making decisions such as the location of waste management facilities or identifying optimal waste management practices (Abd El Karim et al., Citation2020).
The benefit of using MCDA methods for site selection for waste management facilities is that they consider crucial variables including geography, politics, the environment, and economics. To enhance the quality of the suggested solution, MCDA approaches are often typically employed in combination with other tools like GIS (Oyenuga, Citation2015) or other decision-making techniques.
Another motivation for combining tools is to create more realistic, ready-to-implement solutions when one tool compensates for the shortcomings of another (Paz et al., Citation2018; Bolouri et al., Citation2020, Abd El Karim et al., Citation2020).
This study has a systems level perspective of a network of ‘connected’ waste management facilities, in contrast to most methods to C&DW management. A networked configuration/architecture that includes waste management facilities for managing three types of waste simultaneously, namely (1) waste from building construction sites, (2) waste from building demolition sites, and (3) waste from road construction, connects isolated waste management practices through necessary ‘construct elements’. Such a networked configuration provides opportunities for system level analysis and control towards desired outcomes of a C&DW management system.
Since most rapidly developing nations have started initiatives to demolish outdated cities to upgrade to modern living, in line with strategic objectives or sustainable development, the inclusion of building construction, demolition, and road construction waste is of strategic importance. In addition, as most drainage systems are designed to follow road networks, upgrades of roads and respective demolition projects present chances to update both roadways and drainage systems both of which produce C&D waste. Additionally, the waste portions of these three categories of C&DW mentioned above are similar thereby opening opportunities to reconfigure and share waste management facilities. Moreover, debris from the construction or demolition of buildings can be repurposed to provide aggregates for roads.
Likewise, demolition waste can be recycled into new buildings, especially when deconstruction principles are applied to the demolition process (Saghafi & Teshnizi, Citation2011). Furthermore, waste management procedures typically provide comparable paths for waste from building demolition, road construction, and other sources, thus providing another opportunity for economies of scale in C&DWM.
While most location allocation research has centered on identifying and/or developing optimal location-allocation algorithms, this study focuses on demonstrating methodologies and approaches for reconsidering the use of location-allocation models in conjunction with GIS data to enhance C&DW management practices. Along with this logic, location-allocation models can be created as a black box, with a user interface for entering necessary data and information, after which the purported solution is displayed in a way that stakeholders can understand. Such a user interface may be part of a trash dashboard, which stakeholders could use to establish alternative waste flow networks to leverage the current state of C&DW management scenarios, practices and/or methods of implementation.
This study suggests the usage of ‘construct elements’ and ‘connected elements’ to improve the functionality of isolated C&DW management practices. These elements are critical missing links that can enable integration and optimization of C&DW management practices. According to this approach, the low-level C&DW management capabilities suggested in this research are dynamic, time-limited, and thus transient while the high-level management capabilities can be used to leverage economies of scale.
Temporary structures, i.e. temporary in nature, are described in this sense as structures that can be quickly erected or disassembled to a new location when the geographical center point of construction projects changes for whatever cause. The change in location could be because of the end of a construction project and/or the start of new construction projects. Either way, the impact of the change in the geographical center point of construction projects and/or any other changes can be immediately assessed using a location-allocation user interface and/or GIS functions or GIS analysis depending on the details required at hand.
Temporary facilities are time sensitive because their existence is dependent on the duration of a construction project (building, demolition, or road construction). This ‘temporary’ classification enables low-level waste management facilities to be shared and reconfigurable (configurable to adopt numerous functionalities and/or several capacities), while still strategically positioned to leverage total waste transfer costs. Facilities like recycling plants and landfills are regarded as ‘permanent’ infrastructure that could be shared with similar waste fractions from other waste streams within a planning zone or municipality at higher levels of the hierarchy. This is intended to benefit from economies of scale as well as reduced operating costs. A case study is used to demonstrate the practical value of the proposed strategy.
To summarize, C&DW management practices in developing countries need to be improved in many aspects. Waste management practices should implement sound strategies that can position C&DW management towards leading value indicators and best practice in waste management. In other parts of the world, such as the EU, a lot of guidelines, policies, and directives have been developed to help manage construction and demolition waste. In such cases, waste management plans are developed with reference to these guidelines, policies, and directives. Although some of the developing countries have prepared guidelines, policies, and directives it is usually difficult to conduct follow-ups and in many cases, there is no enforcement. As a result, dump sites and landfills are still the final resting place of mixed unrecycled C&DW. There is also a general lack of digital technologies to support C&DW management in developing countries since the digitalization levels are very low. Although smart digital technologies can assist in C&DW management, these are probably too expensive for some of the developing countries. Without methods, tools, and techniques to support C&DW management practices, waste data flow is difficult to track, manage or control. This leads to poor waste management practices and uninformed decision making. Lack of enough case studies also leaves practitioners and contractors with no references to guide decisions in C&DW management. Therefore, more research must be done to improve and advance C&DW management practices in developing countries.
The contribution of this paper to the needs summarized above lies in developing an integrative approach for optimizing ‘isolated’ C&DW management practices. This development is based on re-purposing the roles of location-allocation models and GIS data from mathematical orientations to ‘black box’ implementations that provide valuable and practical information to optimize C&DW management practices. Simple inexpensive digital tools are harnessed into a networking system to provide opportunities for integrating ‘isolated’ C&DW management practices. The notion of re-thinking the role of C&DW management facilities is explored and outlined by perceiving waste management facilities to consist of ‘construct elements’ that can be time-shared in a C&DW management system. In addition, the notion of ‘connected elements’ that are dynamic, time-limited, and yet transient enough to provide the necessary interconnectedness and interconnectivity is developed to support and complement the seamless function of a network of C&DW management facilities. The contribution and novelty of the proposed integrative approach can be summarized as follows:
Location-allocation models and GIS data are re-purposed to simple to use ‘black box’ functions that can be used to analyze, simulate, and optimize C&DW management networks
A simple excel-based dashboard to display waste flow quantities (current and predicted), waste flow patterns and C&DW fractions at all project sites and yet visible to all stakeholders is developed to demonstrate how knowledge-informed decision making can be done
The notions of ‘construct elements’ as time shared facilities that can receive waste from building construction, road construction, and demolition projects is introduced. In this way, the time-shared facilities can leverage economies of scale in C&DW management
The notion of dynamic ‘connected elements’ that provide interconnectivity as a robust way of communicating the locations of accumulated waste and waste in transit, waste accumulation rates and quantities, waste generation rates and quantities is introduced as an integral part of an integrated C&DW management system. To achieve these functionalities the ‘connected elements’ are facilitated with simple, inexpensive sensors, actuators and communications are enabled through smart phones, internet, social media, and a common operating system available to all stakeholders
The integrative approach promotes the use of networking as a fundamental requirement to support and complement the seamless function of a heterogenous collection of networked C&DW management facilities.
The paper is structured as follows: section 2 discusses the various methods that are harnessed to develop and explore techniques for optimizing C&DW management systems and a brief description of the case study is provided. Section 3 describes the development of the integrative approach. Section 4 demonstrates the usefulness of the integrative approach under three scenarios configured to implement the integrative approach at varying degrees of integration depending on the available budget. Finally, conclusions and recommendations are provided in section 5.
2. Methodology
2.1. GIS analysis
The GIS Network Analyst Tool can be used to locate and allocate C&DW management facilities. In comparison to very complicated mathematical methodologies and algorithms for location-allocation, this tool is inexpensive and straightforward to use. The advantage of utilizing ArcGIS over other tools is that it incorporates topographical and road network attributes such as road width, speed limit, obstacles, turn limitation, one-way restrictions, and traffic conditions at various times (ESRI, Citation2020).
Such inclusions provide a more realistic solution that decision makers can employ (ESRI, Citation2020). Other useful GIS tools are service area analysis (SAA), closest facility analysis (CFA), and the origin destination (OD) matrix (ESRI, Citation2020). The term ‘service area analysis’ refers to the area that comprises all accessible roadways within a certain impedance cut-off distance from a waste management facility (Indriasari et al., Citation2010). As a result, service area analysis gives information about the level of service associated with a network of waste management facilities. The closest facility analysis reveals which facilities are the closest to each other (Awaghade et al., Citation2014). In an emergency, such information can be useful to discover alternative routes. The origin destination (OD) matrix is used to locate and measure the lowest-cost paths along a network from one C&DW origin to another C&DW destination. This shows the relative costs of transporting waste between waste management facilities.
2.2. Fuzzy AHP
A pairwise assessment of criteria for selecting waste management facility sites is performed using a fuzzy Analytical Hierarchy Process (Fuzzy AHP). The relevant criteria and sub-criteria utilized in this paper are shown in (Figure ). Figure also shows how expert criteria are used in conjunction with GIS analysis to create a suitability map indicating probable waste management facility sites.
Figure 1. Criteria and eight sub-criteria used in the analysis for waste management site selections.

The criteria employed are consistent with sustainable development and include socioeconomic factors, environmental factors, and morphological issues. Fuzzy logic is used to address uncertainties in expert judgments by incorporating triangular fuzzy number input from experts. Thus, triangular fuzzy memberships were utilized to obtain the final relative weights for the distinct criterion and sub-criteria by calculating the relative importance of different criteria and sub-criteria.
The following equation is used to do pair-wise comparisons of different criteria:
where n is the number of criteria compared, is the ratio between criteria i and criteria j and wi and wj are the priority weights for criteria i and j respectively. A matrix for comparison is used in which normalization for the columns is done by dividing each element by the largest value in the column as per the following equation:
A random number generator based on Saaty’s scale is used to calculate the consistency index from which the consistency ratio is determined and checked for consistency (Khoshand et al., Citation2020). Fuzzy vector weight values are calculated using Equationequation 3(3)
(3) and each comparison value is transformed into fuzzy value using a fuzzy scale.
Where fuzzy value, and
is the summation of all values in column i and row j in the comparison matrix. To determine the weights, defuzzification is done in which the corresponding crisp value is determined based on the membership function using the following logic:
The fuzzy AHP was created with Excel, a popular tool in the Windows operating system. To determine the final relative weights for the various criterion and sub-criteria, an excel spreadsheet was created. When possible, sites are chosen based on socioeconomic, environmental, and morphological characteristics, as well as their sub-categories, cost variables are considered in the deployment of the GIS Network Analyst Tools outlined in section 2.1.
Shape file formats for GIS analysis are developed based on data for sub-criteria. They are transformed into raster images. In raster computations, different raster maps are multiplied using weights determined from the F-AHP. The result is a raster map with color-coded areas that the user can use to locate potential waste management facility sites. Using this map, candidate waste management sites are seeded in appropriate areas that meet the criteria and sub-criteria for picking waste management sites rather than being randomly populated in the location allocation analysis.
2.3. Optimization model development
The Network Analyst tool is supplemented by an optimization model. The next subsections cover the nomenclature and mathematical models.
2.3.1. Optimization model
The optimization goal is to reduce the total expenses associated with waste transfer between waste management facilities. Waste management facilities in the perspective of this paper consist of temporary and stationary facilities. Key was management facilities include Temporary Waste Generation Points (TWGPs), Temporary Centralized Collection Points (TCCPs), Stationary Recycling Plants (SRPs), and Stationary Landfills (SLFs).
Temporary Waste Generation Points (TWGs) are location points conveniently situated at waste generations sites such as building construction, road construction, or building demolitions. They are temporary because they are only active for the duration of the project. They are important location points were generated waste is accumulated and separated into recyclable, hazardous and non-hazardous waste. Therefore, they are specific points of reference that facilitate easy location for waste pick-up at a construction project site. For example, a connected vehicle can easily navigate to the precise location of separated waste using GPS to pick up the waste. To avoid delays and misappropriation of C&DW, the separated waste must be classified, and an accurate description of the waste fraction must be provided. In addition, the location for TWGs must be suitable and safe for workers.
Temporary Centralized Collection Points (TCCPs) are location specific points that accept separated waste from TWGs. They are conveniently located to serve a number of TWGs within a specified distance (impedance cut-off). They are temporary because their geographic position changes with changes in the centroid of construction sites, i.e. building construction, road construction, and demolition sites. They are dynamic and time-limited because the construction projects have different durations. Moreover, new projects can start at any time in the vicinity of other on-going projects thereby changing the centroid of construction sites and hence shifting the precise location of TCCPs. The shifting is done to minimize costs and distance of transporting waste from TWGPs to TCCPs. Besides accepting separated waste, TCCPs are also involved in segregation, sorting, and compacting of waste in preparation for long distance transportation to recycling plants.
Stationary Recycling Plants (SRPs) are permanent facilities that are used for recycling C&DW. Their position is fixed, and they can operate for many years. Hence careful location and allocation of these facilities must be done in a holistic and sustainable manner. Therefore, the siting of SRPs must take into account socio-economic factors, environmental factors as well as morphological factors as discussed in section 2.2. In this way, their operations will minimize land, soil and/or water pollution. Stationary recycling plants receive segregated waste from TCCPs for recycling.
Stationary Landfills (SLFs) are structures that are used to dispose C&DW that cannot be reused nor recycled. Most developing countries are still using dump sites and controlled landfills, while developed countries now advocating sanitary, engineered, and sustainable landfills to reduce the impact of landfills on the environment, human health, and safety (Ozbay et al., Citation2021). SLF receives non-recyclable waste from either SRPs or TCCPs.
In the operations of the integrative approach, one or more TCCPs are optimally assigned to the project sites. SRPs are long-term structures that are strategically placed to serve TCCPs. SLFs are strategically placed to service TCCPs and SRPs. EquationEquations 4(4)
(4) , Equation5
(5)
(5) , and Equation6
(6)
(6) depict three cost functions utilised in the optimization process, whereas Equationequation 7
(7)
(7) shows the objective function.
The limitations are EquationEquations 9(9)
(9) and 10. In Equationequation 9
(9)
(9) , waste from TWGPs to TCCPs can result in either insufficiency or overcapacity, whereas Equationequation 10
(10)
(10) demonstrates that extra waste or less waste transported from TCCP to SRP can result in either insufficiency or overcapacity.
In Equationequation 11(11)
(11) , all waste generated from TWGPs is equal to waste quantities in TCCPs.
In Equationequations 12(12)
(12) and Equation13
(13)
(13) waste disposed should not exceed the capacities of the TCCPs and waste that exceed TCCPs capacity will be diverted to landfills.
EquationEquation 14(14)
(14) helps in achieving a balanced system that will ensure that all generated wastes from TCCP ‘i’ will be disposed in recycling facilities and designated landfills.
EquationEquations 15(15)
(15) and Equation16
(16)
(16) show that the total number of TCCPs and recycling facilities should not exceed the maximum number of available facilities and centres.
EquationEquation 17(17)
(17) ensures that wastes from TWGPs should be disposed to TCCPs.
EquationEquations 18(18)
(18) and Equation19
(19)
(19) ensures that there will be a connection between TCCPs and SLFs and/or SRP when they are only opened.
EquationEquations 20(20)
(20) , Equation21
(21)
(21) , and Equation22
(22)
(22) ensures that waste is transferred only when there is connection in the waste management system.
Although the optimization model looks complex, it is simplified by implementing it in GAMS software and a simple to use and user-friendly interface is created for use by management and stakeholders. In this way, the mathematical details are contained in a ‘black box’ and the user provide inputs and the user interface provide data displayed in a simple to understand form such as details of a simulated network.
2.4. Case study
Qatar is the case study location. Qatar has a total area of 11,651 km2, with a width of 85 km and a length of 185 km (Environment Statistics Annual Report, Citation2017). In the case study, C&DW management practises include transporting mixed trash generated on construction sites to a recycling plant and approved landfills.
The case study’s C&DW management was impacted by two major building construction standards, LEED and GSAS (Ferwati et al., Citation2019; Ismaeel, Citation2020; Saleh & Al-Swidi, Citation2019). At the time of the study, waste management methods were in the hands of the project’s contractors. Because each contractor is free to use waste management recommendations from green grading systems at his or her discretion, there will undoubtedly be disparities in waste management practises and successes (Mohamed & Musharavati, Citation2016). As a result, positioning waste management strategies toward leading value indicators in C&DW management best practise is problematic.
In the case study, qualitative and quantitative methodologies were employed to determine baseline data for C&DW management. Waste is classified into four categories based on its origin: (i) construction site waste materials, (ii) demolition material (debris) from demolition work sites, (iii) road planning, construction, and maintenance materials, and (iv) bulk excavation materials.
The primary materials that make up C&DW are concrete, wood, sand, bricks, and metals. The composition of C&DW was verified using published data and discussions with subject-matter specialists. In the case study, interviews with the primary construction contractors helped to characterise C&DW.
The case study has launched many projects to: (a) establish new cities and new residential areas, (b) upgrade old cities and residential areas, (c) construct infrastructure development projects to meet strategic goals, (d) construct road tunnels, interchanges, underpasses, and bridges, (e) upgrade and enhance road networks, (f) construct Gulf Organisation for Research and Development (GORD) (Citation2022) stadiums, and (g) construct Light Rail Transit. An integrative approach is unavoidable given the large number of such projects and the requirement to meet sustainable development goals. Because the authorities in the case study designated four landfills for bulk excavation waste, bulk excavation materials were not considered in this analysis.
Quantified C&DW data comprised information gathered from various types of building construction and demolitions, infrastructure developments, and road construction projects. These statistics were gathered from the case study between 2012 and 2021. Contractors employed multiple quantification approaches in accordance with agreements with relevant authorities due to differences in the circumstances of project sites (i.e. construction, demolition, and road).
Interviews with authorities revealed that the following methods were used to collect these data: estimations based on field measurements and approximations, site visits (Hu et al., Citation2021; Lam et al., Citation2019; Wu et al., Citation2014, Citation2019), direct measurements (Hu et al., Citation2021; Lam et al., Citation2019; Wu et al., Citation2014, Citation2019).
displays data samples from building construction, road construction, and demolition projects, as well as samples of percentage of waste diverted from landfills.
Table 1. Sample of data collected from different types of c& dw projects in the case study
The amount of waste that is kept out of landfills is a key component in establishing how effective waste management is. At the time of conducting this research, relevant contractors and industry experts in the construction sector evaluated the proportion of waste diverted from landfills, which is shown in (Table ). The construction of the stadiums for the Gulf Organisation for Research and Development (GORD) (Citation2022) achieved the highest proportion of waste diversion from landfills, according to data in (Table ). This was mostly because of policies and practises for managing trash that were based on the GSAS green rating system, which was monitored and applied by authorities in the case study (FIFA World Cup Case Study Report, Citation2022).
3. Results and discussion
3.1. C&DW management facilities
3.1.1. Candidate waste management site locations
The following waste management facilities have been recommended for the case study, Temporary Waste Generation Points (TWGPs), Temporary Centralized Collection Points (TCCPs), Stationary Recycling Plants (SRPs), and Stationary Landfills (SLFs). Temporary Waste Generation Points (TWGPs) are location points conveniently situated at waste generations sites such as building construction, road construction, or building demolitions. They are temporary because they are only active for the duration of the project. The main role is to separate C&DW into recyclable, hazardous and non-hazardous waste. They facilitate easy location for waste pick-up at a construction project site.
Temporary Centralized Collection Points (TCCPs) are location specific points that accept separated waste from TWGs. They are conveniently located to serve a number of TWGPs within a specified distance (impedance cut-off). They are temporary because their geographic position changes with changes in the centroid of construction sites, i.e. building construction, road construction, and demolition sites. They are dynamic and time-limited because the construction projects have different durations. Besides accepting separated waste, TCCPs are also involved in segregation, sorting, and compacting of waste in preparation for long distance transportation to recycling plants.
Stationary Recycling Plants (SRPs) are permanent facilities that are used for recycling C&DW. Stationary recycling plants receive segregated waste from TCCPs for recycling.
Stationary Landfills (SLFs) are structures that are used to dispose C&DW that cannot be reused nor recycled. SLF receives non-recyclable waste from either SRPs or TCCPs.
TWGs, TCCPs, SRPs, and SLFs are the key waste management facilities. TWGs are located on the project site and are determined by the local contractor. Other facilities, such as TCCPs, SRPs, and SLFs, were identified using a mix of GIS analysis and the F-AHP approach. Figure shows a model builder used to come up with a suitability map for locating waste management facilities, while Figure depicts the color-coded output suitability map.
The suitability map was used to seed candidate facilities in the location-allocation study for TCCPs, SRPs, and SLFs. The requirement to locate industrial facilities in regions that the government has classified as industrial, which are shown on the suitability map, had an impact on the site of SRPs as well.
3.1.2. Optimal location of waste management facilities
The Network Analyst Tool was used to determine the best number and location of TCCPs. TWGPs were used as demand points for finding and allocating TCCPs at various project sites. Figure depicts the locations of the selected waste management facilities. Figure depicts the selection of 15 TCCPs as centralized trash collection stations for accepting separated waste from TWGPs (i.e. the demand). In Figure , the exploded view shows that most of the demand points are within reach of the requisite waste management facilities.
Figure , the exploded view also depicts the assignment of demand points to various facilities. It is also worth noting that in the exploded view the TCCPs do not cover all demand points.
Figure shows the selection of SRPs accepting waste from TCCPs (i.e. the demand) and locations of an existing Thermal Treatment (TT) facility, a secured stationary landfill (secured SLF) and sanitary stationary landfills (sanitary SLF). Figure also shows that 3 new stationary recycling plants are required to operationalize the proposed integrative approach to C&DW management system in addition to the one existing recycling plant. In addition, two sanitary landfills are required to operationalize the proposed integrative approach to C&DW management system in addition to one existing landfill. These recommended options are costly, but the long-term benefits are huge and appropriate particularly because the case study has already announced the closure of the existing landfill, and to leverage sustainable development millennium goals described in the most recent Qatar 2030 vision.
3.2. Operating conditions
Table summarizes the operational conditions of the C&DW management system for the proposed integrative method. Table shows the average waste collection effectiveness and waste collection efficiencies. The waste collection efficiency represents the best way (based on optimal location-allocation analysis) of collecting waste from demand points to destination, i.e. ratio of the number of demand points served to the total number of available demand points within a specified impedance cut-off. This is essentially an operational efficiency that is determined based on the total demand that must be served by a network of waste management facilities as an output indicator.
Table 2. Typical operating conditions of an integrative approach to C&DW management practices
From Table , the average waste collection efficiencies for TCCPs, SRPs, and SLFs are 77.99%, 80%, and 83.33%, respectively. The average waste collection efficiencies of various C&DW management facilities for impedance cut-offs of 10 km, 20 km, 30 km, and 40 km can be similarly determined using data in Table .
The average waste collection effectiveness is calculated as the service level provided by the network of waste management facilities using the service area analysis function in the Network Analyst tool. The waste collection effectiveness determines how well facilities in the network are served (ESRI, Citation2010). However, the calculation of the average waste collection effectiveness includes geographic aspects such as the presence of existing buildings, road networks and conditions, as well as barriers and street details such as traffic lights, road travel restrictions.
The average effectiveness of waste management facilities, namely TCCPs, SRPs, and SLFs, in meeting their respective demands is 68.9%, 73.33%, and 78.95%, respectively. In fact, authorities can choose their preferred collection efficiency or effectiveness and then use GIS to generate the relevant networks and facility data (e.g. the number of required facilities to fulfil a given efficiency or effectiveness). By defining the networks in the location allocation user interface, the overall operating expenses can be calculated. Alternatively, less prosperous economies might utilize ArcGIS’s minimum facilities feature to identify the minimal number and location of facilities capable of handling construction and demolition trash. This, however, may jeopardise the efficiency and efficacy of waste management techniques.
3.3. Integrating isolated ventures
Extra facilities, equipment, methods, and approaches are necessary to ‘connect’ separate (‘isolated’) C&DW management operations. The proposed integrative approach consists of ‘construct elements’ and ‘connected elements’. Construct elements serve as the foundation for operating waste management facilities. Connected elements, on the other hand, give a means of connecting waste management facilities into a working network. The ‘construct elements’ are as follows: (i) optimally situated Temporary Waste Generation Points (TWGPs), (ii) optimally located Temporary Centralized Collection Points (TCCPs), (iii) optimally located Stationary Recycling Plants (SRPs), and (iv) optimally located Stationary Landfills (SLFs). Figure depicts the proposed framework and hierarchy for combining discrete initiatives.
Figure 4. Hierarchy for an integrative approach to C&DW management practices in developing countries.

The layout of the architectural elements in Figure provides a well-designed structure and hierarchy of waste management facilities, the path of which is highly repeatable, allowing stakeholders to understand waste flow patterns and identify bottlenecks in the resulting system.
All construction parts in Figure are outfitted with the required IT equipment, such as sensors, data collecting systems, smart phones, iPads/tablets, laptops, and/or desktops, thereby making it simple to regulate and account for waste transfer and transportation via connected elements.
Besides TWGPs, TCCPs, SRPs, and SLFs, other connected elements include: (i) Connected Waste Transfer and Transportation Trucks (CWTTTs), which transport waste among waste management facilities, and (ii) Connected Mobile Truck Stations (CMTS), which perform trash segregation, sorting, and compacting of sorted waste at TCCPs. Mobile truck stations are a noble idea since they may be shifted (change locations) to dynamically follow the density of construction projects, which are specifically identified as TWGP at construction project sites.
Ordinary ICTs like sensors, GPS and GPS trackers, data acquisition systems (for weighing waste aboard a connected truck), smart phones, iPads/tablets, and laptops connected through a common operating system like Windows 10 further increase connected elements in the C&DW management system. The many stakeholders can share and exchange waste data amounts and flows thanks to this connectedness.
Most developing nations have access to waste collection trucks. It is necessary to have connected trucks, or vehicles that can connect to and communicate with neighbouring C&DW facilities, tools, and equipment via networks. The GPS notifies managers of the precise location of waste in transportation, and they can exchange and access waste flow data through wireless internet. Operating economies, communication, and accurate data recording and display are all projected to increase as a result.
The missing links necessary to integrate discrete waste management practises are construct elements and connected elements. They aid in C&DW management by improving connectivity, communication, and coordination. They can also increase waste management efficiency and effectiveness, as well as lower operating costs in the long run, in addition to boosting sustainability metrics. Most national economies, and particularly the case study, can afford these IT tools and equipment.
3.4. Construction projects visualization dashboard
Tracking waste flows and volumes is a fundamental component in an integrated C&DW management system. Equally critical is the ability to accurately predict C&DW one day ahead. Such forecasts can be used to control the functional performance of the waste management system, making it more effective. Predictions can also play an important role in proactively guiding waste collection vehicles to regions with high waste generation and build up.
The capacity to predict waste is projected to lower the percentage of visual waste on roadways, construction sites, and demolition sites that are unacceptable to wealthy residents and intolerable in smart city living (one of the millennium targets for the case study nation). The availability of one-day forecasts gives authorities estimates on trash volumes at each site of building construction, road construction, and demolition site. These estimates can be calculated and entered into computers at construction sites. As a result, waste data will be available at all waste management facilities. Furthermore, actual waste data at each site should be measured and entered locally, allowing for a comparison of anticipated and actual data for future C&DW management system enhancements.
Managers and stakeholders can use waste data patterns to optimise waste collection, waste transfer, and waste transportation plans if waste data is entered into a common platform such as the Windows 10 operating system. This is easily accomplished with smart phones, emails, or walkie-talkies, such as Google Play walkie-talkie communications.
To describe the performance of waste management methods in a zone, district, or municipality, a simple excel dashboard can be utilised. The image below is a typical waste management dashboard generated in Excel (Figure ). Figure depicts waste data recorded on a local computer as both actual and expected. When measured data is available at waste management facilities, predicted data can be utilised for planning, and plans can be readjusted.
Despite its simplicity, this dashboard offers information that can be used to make decisions. For example, the dashboard can be configured to display a live dashboard of waste quantities, waste types, waste fractions, and waste flows at each of the following locations: (i) each waste management facility, (ii) each project site, (iii) municipality level, and (iv) physical planning districts or zones. To display the same information, a more advanced dashboard, such as Live GIS Maps, can be created in the GIS environment (Sepasgozar et al., Citation2021).
Each project site has a dashboard display of building up trash, as seen in Figure . A user interface for a GAMS model for location-allocation analysis was developed and is included in the waste management dashboard to enable flexibility and ease of use in conjunction with a GIS simulation tool. By defining the preferred network and entering input data as prompted by the user interface, the user interface can be used, as a ‘black box’ to find optimal routes for waste and the corresponding costs in the waste management network.
4. Performance comparison
To demonstrate the impact of the proposed integrative approach to waste management systems, three waste management scenarios are defined, i.e. scenario 1-base scenario, scenario 2 – upgrade of existing practices, and scenario 3 – applications of the integrative approach.
4.1. Scenario (1) base scenario
In the case study, the current waste management practice includes the following elements: construction project sites (roads and buildings), demolition project sites, waste management vehicles for transferring and transporting trash, one recycling plant, and two C&DW landfills. This scenario depicts the case study as it existed at the time this project was carried out. The GIS Network Analyst Tool is used in this scenario to simulate the current network and determine the total operating costs. The following sections present two potential scenarios based on the research findings.
4.2. Scenario (2) upgrade
This is a proposed upgrade to the case study’s current waste management techniques. Existing waste management facilities are used, but with certain modifications to modernize and integrate waste management methods into a more effective C&DW management system. Additional elements required include: (a) temporary waste generation points (TWGPs) where waste is separated at the source of generation, (b) temporary centralised collection points (TCCPs) where waste is sorted, and (c) case study landfill sites recently approved due to the anticipated increase in C&DW generation. Scenarios 1 and 2 differ in that Scenario 2 contains construct elements and accessories, as detailed in Section 3, in addition to the optimal number and location of SRPs and SLFs. The GIS Network Analyst Tool is used to simulate the ideal position and allocation of the upgraded waste treatment facilities into a working C&DW management system.
4.3. Scenario (3) integrative approach
The suggested waste management system is represented by this scenario. Construction and demolition waste management facilities (C&DW management facilities) include construct elements and related accessories, i.e. (a) Temporary Waste Generation Points (TWGPs) where waste is separated, (b) optimally located and allocated Temporary Centralized Collection Points (TCCPs) where waste is sorted and rigorously segregated into well-defined and well characterized C&DW fractions, (c) optimally located and allocated C&DW Stationary Recycling Plants (SRPs), and optimally located stationary landfills (SLFs). The description of operations from scenario 3 has been provided in sections 3 and 4. The GIS Network Analyst Tool is used to simulate the ideal placement and distribution of the proposed waste management facilities into a useful C&DW management system.
To assess the performance of the scenarios, the optimization model outlined in section 3.3 was used. The calculations included various cost components that were obtained from the case study authorities through interviews to calculate the cost of C&DW collection. The optimization model was supplied with the outputs from the GIS simulated networks for scenarios 1, 2, and 3. Transportation expenses from various building project sites to landfill sites were considered when calculating the total costs connected with the three waste management scenarios.
Using Quantum GIS, the distances between the TWGPs, TCCPs, SRPs, and SLFs were determined using the Euclidean distance (QGIS). EquationEquations 4(4)
(4) , Equation5
(5)
(5) , and Equation6
(6)
(6) illustrate how various dynamic weights for each cost in the objective function were used to get the overall collection costs. On a personal computer running Windows 10 and powered by an Intel Core i5 processor clocked at 2.50 GHz, a GAMS model was run using the mixed integer linear programming algorithm. The various scenarios’ average run times were between 2 and 7 minutes, making it possible to make decisions, reclassify objects, relocate objects, and reassign objects within an average period of 10 minutes.
The total collection costs for moving waste from source generation to landfill sites were determined to be 1,842,240 USD/day, 1,356,000 USD/day, and 875,450 USD/day for the current practice—scenario 1, upgraded system—scenario 2, and the integrative approach—scenario 3. As a result, by improving waste management techniques (scenario 2), the case study can save 26% of total operating costs. More importantly, the case study can save 52% of total operating expenses by utilizing the proposed C&DW management system (i.e. scenario 3 in which a highly integrative method for all waste management facilities with interconnectivity is applied).
4.4. Practical implications
The study has provided an understanding of waste management practices in developing countries and how they can be improved. The reported findings can help to improve waste management practices, policies and regulations in developing countries and position waste management practices towards leading value indicators of integrated waste management systems. Improvements can be made at construction sites, municipal and national levels depending on the current state of waste management practices in a specific country. A simple way of understanding, managing, and controlling waste data flows and patterns has been demonstrated through an excel dashboard as an alternative to live GIS maps of C&DW management. In this way, the pattern of operating costs for C&DW management practices can be known and hence controlled. Availability of alternative waste routes among waste management facilities can provide useful alternatives according to the available budgets and waste management resources.
Towns, cities, and national economies can use this study to reevaluate C&DW management and put useful, unified C&DW management plans and techniques into practice. The findings can also help stakeholders and entrepreneurs to understand the status quo of waste management in developing countries particularly the case study. The study can also help construction professionals to identify loopholes in their C&DW management practices and find ways of saving money and implement more sustainable waste management practices. The research community can also use the discussions in this paper to advance theory and applications in waste management.
5. Conclusion
The primary purpose of this study is to develop an integrative strategy for optimizing Construction and Demolition Waste (C&DW) management methods in developing nations. To that goal, a variety of tools, methodologies, and techniques have been employed to illustrate how separate C&DW management practices may be successfully integrated into a complete C&DW management system. The main contribution of this paper lies in re-purposing location-allocation models and GIS data to provide opportunities for integrating ‘isolated’ C&DW management practices. The notion of re-thinking the role of C&DW management facilities is explored by perceiving waste management facilities to consist of ‘construct’ and ‘connected’ elements”. The connected elements provide the necessary interconnectivity required to transform isolated C&DW management practices into integrated waste management systems. Moreover, the integrative approach includes a mixture of ‘permanent’ and ‘temporary’ facilities. Temporary facilities are included to avoid unnecessary investments in waste management facilities that are used for the duration of road construction, building construction and demolition projects. The functionality of permanent facilities can be re-purposed as time-shared facilities to leverage economies of scale while ‘temporary’ facilities are dynamic, time-limited, and transient to reflect differences in construction projects durations that manifest as a change in the geographic location of building construction, road construction and demolition projects. Such considerations are expected to support and complement the seamless function of a network of C&DW management facilities.
The created optimization model can calculate the actual operating costs for a particular network of waste management facilities. Authorities can influence waste management activities based on known costs and how these costs affect the waste management budget because operating costs are known. Location-allocation simulations also offer management with statistics and information regarding the effectiveness and efficiency of the waste collection networks and routes that have been installed. Location allocation models are presented as black box functions that can be understood and used due by stakeholders to a simple and user-friendly interface linked to the mathematical optimization model and GIS analysis environment.
The optimization methodology employed is designed to reduce operating costs related to waste collection, transfer, and transportation across a diverse collection of C&DW management facilities. Two different scenarios for applying the proposed technique (scenarios 2 and 3) have been considered and compared to the current scenario (scenario 1). Authorities can choose the best scenario based on available resources; particularly budget constraints typical of problems in developing countries. The results demonstrate that by improving current waste management techniques, the case study can save 26% of total operating costs. However, by applying the integrative approach proposed in this paper, the case study can save 52% of the total operating costs. By implementing the proposed approach, ‘isolated’ waste management practices can be advanced into a beneficial waste management system. Stakeholder decision making is improved by the availability of precise trash data amounts and waste flow patterns visualized as a dashboard. While a fully integrated C&DW management system may be too expensive for some developing countries, authorities can choose the less expensive option (i.e. modernize waste management facilities as in scenario 2) with benefits.
Nomenclature
Acknowledgements
Open Access funding provided by the Qatar National Library.
Disclosure statement
No potential conflict of interest was reported by the author(s).
References
- Abdelhamid, M. S. (2014). Assessment of different construction and demolition waste management approaches. HBRC Journal, 10(3), 317–21. https://doi.org/10.1016/j.hbrcj.2014.01.003
- Abd El Karim, A., & Awawdeh, M. M. (2020). Integrating GIS accessibility and location-allocation models with multicriteria decision analysis for evaluating quality of life in Buraidah city, KSA. Sustainability, 12(4), 1412.
- Al-Otaibi, A., Bowan, P. A., Abdel Daiem, M. M., Said, N., Ebohon, J. O., Alabdullatief, A., Al-Enazi, E., & Watts, G. (2022). Identifying the barriers to sustainable management of construction and demolition waste in developed and developing countries. Sustainability, 14(13), 7532. https://doi.org/10.3390/su14137532
- Alsheyab, M. A. T. (2022). Recycling of construction and demolition waste and its impact on climate change and sustainable development. International Journal of Environmental Science and Technology, 19(3), 2129–2138. https://doi.org/10.1007/s13762-021-03217-1
- Aslam, M. S., Huang, B., & Cui, L. (2020). Review of construction and demolition waste management in China and USA. Journal of Environmental Management, 264, 110445. https://doi.org/10.1016/j.jenvman.2020.110445
- Awaghade, S., Dandekar, P., & Ranade, P. (2014). Site selection and closest facility analysis for automated teller machine (ATM) centers: Case study for Aundh (Pune), India. International Journal of Advancement in Remote Sensing, GIS and Geography, 2(1), 19–29.
- Banawi, A. A., Besne, A., Fonseca, D., & Ferrandiz, J. (2020). A three methods proactive improvement model for buildings construction processes. Sustainability, 12(10), 4335. https://doi.org/10.3390/su12104335
- Bao, L., Ding, X., Zhang, J., & Ma, D. (2023). Can new urbanization construction improve ecological welfare performance in the Yangtze River Economic Belt?. Sustainability, 15(11), 8758.
- Bao, Z., & Lu, W. (2020). Developing efficient circularity for construction and demolition waste management in fast emerging economies: Lessons learned from Shenzhen, China. Science of the Total Environment, 724, 138264. https://doi.org/10.1016/j.scitotenv.2020.138264
- Bolouri, S., Vafeainejad, A., Alesheikh, A., & Aghamohammadi, H. (2020). Environmental sustainable development optimizing the location of urban facilities using vector assignment ordered median problem-integrated GIS. International Journal of Environmental Science and Technology, 17(5), 3033–3054. https://doi.org/10.1007/s13762-019-02573-3
- Cheng, B., Huang, J., Guo, Z., Li, J., & Chen, H. (2022). Towards sustainable construction through better construction and demolition waste management practices: A SWOT analysis of Suzhou, China. International Journal of Construction Management, 23(15), 1–11. https://doi.org/10.1080/15623599.2022.2081406
- Chen, K., Wang, J., Yu, B., Wu, H., & Zhang, J. (2021). Critical evaluation of construction and demolition waste and associated environmental impacts: A scientometric analysis. Journal of Cleaner Production, 287, 125071. https://doi.org/10.1016/j.jclepro.2020.125071
- de Souza Melaré, A. V., González, S. M., Faceli, K., & Casadei, V. (2017). Technologies and decision support systems to aid solid-waste management: A systematic review. Waste Management, 59, 567–584. https://doi.org/10.1016/j.wasman.2016.10.045
- Duan, H., Miller, T. R., Liu, G., & Tam, V. W. (2019). Construction debris becomes growing concern of growing cities. Waste Management, 83, 1–5. https://doi.org/10.1016/j.wasman.2018.10.044
- Egbelakin, T., Ogunmakinde, O. E., Teshich, B., & Omotayo, T. (2021). Managing fast-track construction project in Qatar: Challenges and opportunities. Buildings, 11(12), 640. https://doi.org/10.3390/buildings11120640
- Eghbali-Zarch, M., Tavakkoli-Moghaddam, R., Dehghan-Sanej, K., & Kaboli, A. (2022). Prioritizing the effective strategies for construction and demolition waste management using fuzzy IDOCRIW and WASPAS methods. Engineering, Construction & Architectural Management, 29(3), 1109–1138. https://doi.org/10.1108/ECAM-08-2020-0617
- Environmental statistics. (2017). https://gccstat.org/images/gccstat/docman/state-of-qatar-1/2019/En Accessed October
- ESRI. (2010). ArcGIS Desktop: Release 10. Environmental Systems Research Institute.
- ESRI. (2015). ArcGIS Desktop: Release 10.3.1. Environmental Systems Research Institute.
- ESRI. (2020). ArcGIS [GIS software]. Version 10.8. Environmental Systems Research Institute,Inc.
- Ferwati, M. S., Al Saeed, M., Shafaghat, A., & Keyvanfar, A. (2019). Qatar sustainability assessment system (QSAS)-neighborhood development (ND) assessment model: Coupling green urban planning and green building design. Journal of Building Engineering, 22, 171–180. https://doi.org/10.1016/j.jobe.2018.12.006
- Fitri, L., Hatmoko, J. U. D., & Harman, F. (2019). November. Managing construction waste in developed countries: Lessons learned for Indonesia. IOP Conference Series: Earth and Environmental Science, 366(1), 012016. IOP Publishing: https://doi.org/10.1088/1755-1315/366/1/012016
- Guerra, B. C., Bakchan, A., Leite, F., & Faust, K. M. (2019). BIM-based automated construction waste estimation algorithms: The case of concrete and drywall waste streams. Waste Management, 87, 825–832. https://doi.org/10.1016/j.wasman.2019.03.010
- Guerra, B. C., Leite, F., & Faust, K. M. (2020). 4D-BIM to enhance construction waste reuse and recycle planning: Case studies on concrete and drywall waste streams. Waste Management, 116, 79–90. https://doi.org/10.1016/j.wasman.2020.07.035
- Gulf Organisation for Research & Development (GORD), wWaste management best practices for construction sites case studies from FIFA world cup Qatar 2022™ stadiums environment statistics annual report, 2014. State of Qatar Ministry of Development Planning and Statistics, retrieved 2020 from https://www.psa.gov.qa/en/knowledge/Publications/Environment/Env_Environmental_Statistic_Report_En_2013.pdf
- Gupta, S., Jha, K. N., & Vyas, G. (2022). Proposing building information modeling-based theoretical framework for construction and demolition waste management: Strategies and tools. International Journal of Construction Management, 22(12), 2345–2355. https://doi.org/10.1080/15623599.2020.1786908
- Hasan, M. R., Sagar, M. S. I., & Ray, B. C. (2023). Barriers to improving construction and demolition waste management in Bangladesh. International Journal of Construction Management, 23(14), 2333–2347. https://doi.org/10.1080/15623599.2022.2056804
- Hu, Y., (2011), May. Minimization management of construction waste. Proceedings of the 2011 International symposium on water resource and environmental protection, Xi’an, China (Vol. 4, pp. 2769–2772). IEEE.
- Huang, B., Wang, X., Kua, H., Geng, Y., Bleischwitz, R., & Ren, J. (2018). Construction and demolition waste management in China through the 3R principle. Resources, Conservation and Recycling, 129, 36–44. https://doi.org/10.1016/j.resconrec.2017.09.029
- Hu, R., Chen, K., Chen, W., Wang, Q., & Luo, H. (2021). Estimation of construction waste generation based on an improved on-site measurement and SVM-based prediction model: A case of commercial buildings in China. Waste Management, 126, 791–799. https://doi.org/10.1016/j.wasman.2021.04.012
- Hu, X., Zhou, Y., Vanhullebusch, S., Mestdagh, R., Cui, Z., & Li, J. (2022). Smart building demolition and waste management frame with image-to-BIM. Journal of Building Engineering, 49, 104058. https://doi.org/10.1016/j.jobe.2022.104058
- Indriasari, V., Mahmud, A. R., Ahmad, N., & Shariff, A. R. M. (2010). Maximal service area problem for optimal siting of emergency facilities. International Journal of Geographical Information Science, 24(2), 213–230. https://doi.org/10.1080/13658810802549162
- Islam, R., Nazifa, T. H., Yuniarto, A., Uddin, A. S., Salmiati, S., & Shahid, S. (2019). An empirical study of construction and demolition waste generation and implication of recycling. Waste Management, 95, 10–21. https://doi.org/10.1016/j.wasman.2019.05.049
- Ismaeel, W. S. E. (2020). LEED in the MENA Region…Chances and Challenges of Change. In Z. Gou(Ed.), Green Building in Developing Countries (pp. 237–252). Green Energy and Technology.
- Jain, M. S., Sudarsan, J. S., & Parija, P. P. (2023). Managing construction and demolition waste using lean tools to achieve environmental sustainability: An Indian perspective. Environmental Science and Pollution Research, 30(19), 57188–57200. https://doi.org/10.1007/s11356-023-26445-z
- Kabirifar, K., Mojtahedi, M., & Wang, C. C. (2021). A systematic review of construction and demolition waste management in Australia: Current practices and challenges. Recycling, 6(2), 34. https://doi.org/10.3390/recycling6020034
- Kabirifar, K., Mojtahedi, M., Wang, C. C., & WY, T. V. (2020). A conceptual foundation for effective construction and demolition waste management. Cleaner Engineering and Technology, 1, 100019. https://doi.org/10.1016/j.clet.2020.100019
- Kang, K., Besklubova, S., Dai, Y., & Zhong, R. Y. (2022). Building demolition waste management through smart BIM: A case study in Hong Kong. Waste Management, 143, 69–83. https://doi.org/10.1016/j.wasman.2022.02.027
- Khoshand, A., Khanlari, K., Abbasianjahromi, H., & Zoghi, M. (2020). Construction and demolition waste management: Fuzzy analytic hierarchy process approach. Waste Management & Research, 38(7), 773–782. https://doi.org/10.1177/0734242X20910468
- Lam, P. T., Ann, T. W., Wu, Z., & Poon, C. S. (2019). Methodology for upstream estimation of construction waste for new building projects. Journal of Cleaner Production, 230, 1003–1012. https://doi.org/10.1016/j.jclepro.2019.04.183
- Lin, Z., Xie, Q., Feng, Y., Zhang, P., & Yao, P. (2020). Towards a robust facility location model for construction and demolition waste transfer stations under uncertain environment: The case of Chongqing. Waste Management, 105, 73–83.
- Liu, J., Liu, Y., & Wang, X. (2020). An environmental assessment model of construction and demolition waste based on system dynamics: A case study in Guangzhou. Environmental Science and Pollution Research, 27(30), 37237–37259. https://doi.org/10.1007/s11356-019-07107-5
- Li, C. Z., Zhao, Y., Xiao, B., Yu, B., Tam, V. W., Chen, Z., & Ya, Y. (2020). Research trend of the application of information technologies in construction and demolition waste management. Journal of Cleaner Production, 263, 121458. https://doi.org/10.1016/j.jclepro.2020.121458
- Lu, W., Bao, Z., Lee, W. M., Chi, B., & Wang, J. (2021). An analytical framework of “zero waste construction site”: Two case studies of Shenzhen, China. Waste Management, 121, 343–353. https://doi.org/10.1016/j.wasman.2020.12.029
- Madi, N., & Srour, I. (2019). Managing emergency construction and demolition waste in Syria using GIS. Resources, Conservation and Recycling, 141, 163–175. https://doi.org/10.1016/j.resconrec.2018.10.018
- Mahayuddin, S. A., Pereira, J. J., Badaruzzaman, W. H. W., & Mokhtar, M. B. (2008). Construction waste management in a developing country: Case study of Ipoh, Malaysia. WIT Transactions on Ecology and the Environment, 109, 481–489. https://doi.org/10.2495/WM080491
- Manowong, E. (2012). Investigating factors influencing construction waste management efforts in developing countries: An experience from Thailand. Waste Management & Research, 30(1), 56–71. https://doi.org/10.1177/0734242X10387012
- Mohamed, F., & Musharavati, F. (2016). Green building rating systems: A comparison of LEED and GSAS systems on construction & demolition waste management performance in Qatar. Qatar University.
- Nadazdi, A., Naunovic, Z., & Ivanisevic, N. (2022). Circular economy in construction and demolition waste management in the western balkans: A sustainability assessment framework. Sustainability, 14(2), 871. https://doi.org/10.3390/su14020871
- Newaz, M. T., Davis, P., Sher, W., & Simon, L. (2022). Factors affecting construction waste management streams in Australia. International Journal of Construction Management, 22(13), 2625–2633. https://doi.org/10.1080/15623599.2020.1815122
- Oluleye, B. I., Chan, D. W., & Olawumi, T. O. (2022). Barriers to circular economy adoption and concomitant implementation strategies in building construction and demolition waste management: A PRISMA and interpretive structural modeling approach. Habitat International, 126, 102615. https://doi.org/10.1016/j.habitatint.2022.102615
- Oyenuga, A. A., & Bhamidiarri, R. (2015). Considering appropriate decision support models for construction and demolition waste management optimization: Possibilities and limitations. SSRG International Journal of Economics and Management Studies, 2(5), 15–24.
- Ozbay, G., Jones, M., Gadde, M., Isah, S., & Attarwala, T. (2021). Design and operation of effective landfills with minimal effects on the environment and human health. Journal of Environmental and Public Health, 2021, 1–13. https://doi.org/10.1155/2021/6921607
- Paz, D. H. F. D., Lafayette, K. P. V., & Sobral, M. D. C. (2018). GIS-based planning system for managing the flow of construction and demolition waste in Brazil. Waste Management & Research, 36(6), 541–549. https://doi.org/10.1177/0734242X18772096
- Purchase, C. K., Al Zulayq, D. M., O’Brien, B. T., Kowalewski, M. J., Berenjian, A., Tarighaleslami, A. H., & Seifan, M.(2021). Circular economy of construction and demolition waste: A literature review on lessons, challenges, and benefits. Materials, 15(1), 76.
- Rahigude, R., Khwairakpam, D., Rade, S., & Kadam, K. (2022). Construction waste management in the context of de-tools, industry 4.0 & circular economy, a critical review of pune metropolitan area, India. International Journal of Sustainable Building Technology and Urban Development, 13(4), 514–548.
- Ramos, M., & Martinho, G. (2023). An assessment of the illegal dumping of construction and demolition waste. Cleaner Waste Systems, 4, 100073. https://doi.org/10.1016/j.clwas.2022.100073
- Ramos, M., Martinho, G., & Pina, J. (2023). Strategies to promote construction and demolition waste management in the context of local dynamics. Waste Management, 162, 102–112. https://doi.org/10.1016/j.wasman.2023.02.028
- Ratnasabapathy, S., Perera, S., & Alashwal, A. (2019). A review of smart technology usage in construction and demolition waste management. In Y. G. Sandanayake, S. Gunatilake, & A. Waidyasekara (Eds.), Proceedings of the 8th World Construction Symposium, Colombo, Sri Lanka, 8-10 November (pp. 45–55). https://doi.org/10.31705/WCS.2019.5.
- Rondinel-Oviedo, D. R. (2023). Construction and demolition waste management in developing countries: A diagnosis from 265 construction sites in the lima metropolitan area. International Journal of Construction Management, 23(2), 371–382. https://doi.org/10.1080/15623599.2021.1874677
- Saghafi, M. D., & Teshnizi, Z. A. H. (2011). Building deconstruction and material recovery in Iran: An analysis of major determinants. Procedia Engineering, 21, 853–863. https://doi.org/10.1016/j.proeng.2011.11.2087
- Saleh, R. M., & Al-Swidi, A. (2019). The adoption of green building practices in construction projects in Qatar: A preliminary study. Management of Environmental Quality, 30(6), 1238–1255. https://doi.org/10.1108/MEQ-12-2018-0208
- Sartipi, F. (2020). Influence of 5G and IoT in construction and demolition waste recycling–conceptual smart city design. Journal of Construction Materials, 1(4), 1–9. https://doi.org/10.36756/JCM.v1.4.1
- Sepasgozar, S. M., Mair, D. F., Tahmasebinia, F., Shirowzhan, S., Li, H., Richter, A., Yang, L., & Xu, S. (2021). Waste management and possible directions of utilising digital technologies in the construction context. Journal of Cleaner Production, 324, 129095. https://doi.org/10.1016/j.jclepro.2021.129095
- Shahid, M. U., Thaheem, M. J., & Arshad, H. (2023). Quantification and benchmarking of construction waste and its impact on cost–a case of Pakistan. Engineering, Construction & Architectural Management, 30(6), 2304–2333. https://doi.org/10.1108/ECAM-07-2019-0375
- Szpilko, D., de la Torre Gallegos, A., Jimenez Naharro, F., Rzepka, A., & Remiszewska, A. (2023). Waste management in the Smart City: Current practices and future directions. Resources, 12(10), 115. https://doi.org/10.3390/resources12100115
- Thives, L. P., Ghisi, E., & Júnior, J. J. T. (2022). An outlook on the management of construction and demolition waste in Brazil. Cleaner Materials, 6, 100153. https://doi.org/10.1016/j.clema.2022.100153
- Tirkolaee, E. B., Mahdavi, I., Esfahani, M. M. S., & Weber, G. W. (2020). A robust green location-allocation-inventory problem to design an urban waste management system under uncertainty. Waste Management, 102, 340–350. https://doi.org/10.1016/j.wasman.2019.10.038
- Urio, A. F., & Brent, A. C. (2006). Solid waste management strategy in Botswana: The reduction of construction waste. Journal of the South African Institution of Civil Engineering= Joernaal van die Suid-Afrikaanse Instituut van Siviele Ingenieurswese, 48(2), 18–22.
- Vu, H. L., Ng, K. T. W., & Bolingbroke, D. (2018). Parameter interrelationships in a dual phase GIS-based municipal solid waste collection model. Waste Management, 78, 258–270. https://doi.org/10.1016/j.wasman.2018.05.050
- Wang, Z., Xie, W., & Liu, J. (2022). Regional differences and driving factors of construction and demolition waste generation in China. Engineering, Construction & Architectural Management, 29(6), 2300–2327. https://doi.org/10.1108/ECAM-10-2020-0887
- Waste Management Best Practices for Construction Sites Case Studies from FIFA World Cup Qatar. (2022). Stadiums. https://gsas.gord.qa/wp-content/uploads/2021/10/GSAS-SC-Waste-Management-Report.pdf Accessed October
- Wu, Z., Ann, T. W., & Poon, C. S. (2019). An off-site snapshot methodology for estimating building construction waste composition-a case study of Hong Kong. Environmental Impact Assessment Review, 77, 128–135. https://doi.org/10.1016/j.eiar.2019.03.006
- Wu, Z., Ann, T. W., Shen, L., & Liu, G. (2014). Quantifying construction and demolition waste: An analytical review. Waste Management, 34(9), 1683–1692. https://doi.org/10.1016/j.wasman.2014.05.010
- Wu, Z., Yu, A. T., & Poon, C. S. (2020). Promoting effective construction and demolition waste management towards sustainable development: A case study of Hong Kong. Sustainable Development, 28(6), 1713–1724. https://doi.org/10.1002/sd.2119
- Yazdani, M., Kabirifar, K., Frimpong, B. E., Shariati, M., Mirmozaffari, M., & Boskabadi, A. (2020). Improving construction and demolition waste collection service in an urban area using a simheuristic approach: A case study in Sydney, Australia. Journal of Cleaner Production, 280, 124138. https://doi.org/10.1016/j.jclepro.2020.124138
- Yeheyis, M., Hewage, K., Alam, M. S., Eskicioglu, C., & Sadiq, R. (2013). An overview of construction and demolition waste management in Canada: A lifecycle analysis approach to sustainability. Clean Technologies and Environmental Policy, 15, 81–91.
- Yuan, H. (2013). A SWOT analysis of successful construction waste management. Journal of Cleaner Production, 1–8.
- Yu, H., & Solvang, W. D. (2017). A multi-objective location-allocation optimization for sustainable management of municipal solid waste. Environment Systems and Decisions, 37(3), 289–308. https://doi.org/10.1007/s10669-017-9632-y
- Zeller, V., Towa, E., Degrez, M., & Achten, W. M. (2019). Urban waste flows and their potential for a circular economy model at city-region level. Waste Management, 83, 83–94. https://doi.org/10.1016/j.wasman.2018.10.034
- Zhang, C., Hu, M., DiMaio, F., Sprecher, B., Yang, X., & Tukker, A. (2022). An overview of the waste hierarchy framework for analyzing the circularity in construction and demolition waste management in Europe. Science of the Total Environment, 803, 149892. https://doi.org/10.1016/j.scitotenv.2021.149892
- Zhao, X. (2021). Stakeholder-associated factors influencing construction and demolition waste management: A systematic review. Buildings, 11(4), 149. https://doi.org/10.3390/buildings11040149