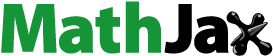
Abstract
The Nickel-Niobium Pentoxide Composite Coating is formed by electrochemically depositing nickel (Ni) and niobium pentoxide (Nb2O5). An investigation was conducted on corrosion resistance, microhardness, roughness, and deposit morphology. A comparison was made between composites and pure nickel coatings in scratch and contact angle tests. There is a homogeneous particle distribution with a maximum particle size of 99.27 µm in the coating. Adding Nb2O5 particles to the composite coating improved corrosion resistance with a maximum inhibitory efficiency of 87.9%. An increase of 44% in microhardness was observed for the Ni-Nb2O5 composite coating. The scratch test revealed no adhesion cracks and delamination at the surface of the cracks. The composite coating exhibited a hydrophobic nature in the contact angle measurement.
1. Introduction
Scientists and engineers have long striven to develop new materials with improved properties. The development of new and non-equilibrium (metastable) processing methods has recently resulted in improved performance of existing materials. Researchers claimed that the lack of superior properties among conventional coatings opened up avenues for nanostructured coatings, and found extremely promising for a variety of applications (Gurrappa and Binder Citation2008). In the development of nanocomposite films of metal matrix materials, particles of pure metals, polymers, ceramics, and organic materials such as Cu, Ni, Co, Cr, and others have been added to submicron sizes to 100 mm sizes (Zhou et al. Citation2017). Nanocomposite materials exhibit high tailorability, making them an ideal material for a variety of applications including marine, aerospace, sensors, automotive, electronics, memory devices, construction, energy, and biomedicine (Torabinejad et al. Citation2017; Naveen Kumar et al. Citation2021; Naveen Kumar et al. Citation2022; Kumar et al. 2022; Naveen Kumar et al. Citation2023; Naveen Kumar et al. Citation2018). The composite coating offers substrate protection through the combination of two or more substances. Several reinforcing particles form the matrix of this coating, giving it its unique properties. To meet the varied needs of the industry, numerous techniques are used. Additionally, protecting against corrosion, wear, and mechanical qualities are the functions of these composite coatings (Chen Citation2011; Muller and Ferkel Citation1998; Chen et al. Citation2006; Lampke et al. Citation2006; Shrestha et al. Citation2001; Sasi et al. Citation2015; Wang and Wei Citation2003). A composite coating based on Ni has excellent corrosion resistance, tribological properties, hardness, and bonding strength, which makes them an excellent choice for a wide range of industrial applications (He et al. Citation2014; Flores et al. Citation2009; Manohar et al. Citation2021; Rodrı́guez et al. Citation2003). Various techniques have been employed by researchers to coat including gas tungsten arc cladding, plasmas, laser cladding, high-velocity oxygen fuel spraying (HVOF), thermal spraying, electrodeposition, electroplating, electroless plating, detonation spraying, electrophoretic deposition (EPD), pack cementing, cold spraying, and hydrothermal deposition (Karmakar, Maji, and Ghosh Citation2021). In addition to its wide range of configurable production parameters, electrodeposition is a preferred technique for producing coatings, as well as its low cost, control of the deposit thickness and structure even on complex shapes, high purity, rapidity, lack of heat treatment, and ease of production (Koch Citation2007; Ma, Wang, and Walsh Citation2015). The reduction of metallic ions from an electrolyte occurs when metal, alloy, or composite films are electrodeposited on conductive substrates by supplying a current or potential (Zhou et al. Citation2017). In electrodeposition, metal ions are reduced with undissolved oxide particles, such as (SiO2) (Rathod et al. Citation2022), (TiO2) (Huang, Hu, and Pan Citation2011; Lajevardi and Shahrabi Citation2010), (CeO2) (Junli, Ruidong, and Zhang Citation2012), (Al2O3) (Sasi et al. Citation2015; Jeyaraj, Arulshri, and Sivasankaran Citation2016; Feng et al. Citation2008), (ZrO2) (Kavirajwar, Basavanna, and Devendra Citation2022), (MoS2) (Cardinal et al. Citation2009), (SrSO) (Liang et al. Citation2009), (Gr) (Varadaraj et al. Citation2022) carbides (SiC, B4C) (Kılıç et al. Citation2013; Devaneyan and Senthilvelan Citation2014; Shrestha, Kawai, and Saji Citation2005), nitrides (Si3N4) (Krishnaveni, Narayanan, and Seshadri Citation2008; Eslami et al. Citation2014), polymers (PTFE) (Ger and Hwang Citation2002; Wang et al. Citation2010), and even metals (Cr, Co) (Saravanan and Mohan Citation2009; Srivastava, Grips, and Rajam Citation2007). These composite coatings have been reported to provide improved substrate protection against physical and corrosive damage.
Metal, alloy, or composite films are electrodeposited onto conductive surfaces using a current or potential to reduce metallic ions in the electrolyte. Since niobium pentoxide has surface valence cations, it is a semiconductor with a greater surface acidity. Furthermore, Nb2O5 exhibits polymorphism, resulting in different physicochemical properties at different temperatures (de Oliveira, de Souza, and Lopes-Moriyama Citation2020). The resistance of Niobium to corrosion in nearly all aqueous environments is greater than that of nickel (ASM Handbook Citation1993). Brazil has 80.2% of the world’s niobium reserves, accounting for more than 88% of global output (Smrithi et al. Citation2022).
R.Q. Fratari et al. (Fratari and Robin Citation2006) performed electrocoating of Ni-Nb on carbon steel. There was a study of microhardness and volume fraction of co-deposited Nb particles in relation to cathodic current density, electrolyte stirring rate, and Nb particle concentration in the bath. A Ni-Nb composite coating obtained at 20 or 40 mAcm−2 exhibits a higher microhardness than a pure Ni coating. An improvement in corrosion resistance was achieved by incorporating Nb particles into Ni coatings in NaCl and H2SO4 solutions. Alain Robin et al. (Robin and Fratari Citation2007) developed Ni-Nb composite coatings for carbon steel through galvanostatic electrolysis of nickel baths containing suspended niobium powders. An evaluation of the effects of cathodic current density, electrolyte stirring rate, and Nb particle concentration in the bath was conducted for the purpose of determining the deposit morphology, texture, fractions of co-deposited Nb particles, and microhardness of the deposit. The morphology of composite layers of Ni-Nb is rough with Ni grains oriented randomly. The corrosion and the microhardness characteristics have improved with the addition of Nb. HCR Moreira et al. (Moreira et al. Citation2022) examined the tribology of electrodeposited Zn-Ni coatings using nanoparticles of niobium pentoxide (1 gl−1) as a dispersant. It was found that Niobium pentoxide nanoparticles produced significantly denser coatings, which incorporated some Nb into the coating surface. A more durable and adherent coating was developed. T Oluyori et al. (Oluyori et al. Citation2017) developed composite coatings incorporating Zn-Nb2O5 for corrosion resistance and wear resistance using electrodeposition from a sulphate bath.
Nb2O5 exhibits excellent mechanical flexibility, durability, and optical properties (Rani et al. Citation2014). Despite its application as an electrodeposition process for composite strengthening, Nb2O5 has not been extensively tested for corrosion resistance and structural performance. Corrosion testing is carried out to improve the reliability and longevity of coated products and structures by evaluating the coating performance (Shashanka, Chaira, and Be Citation2018). This study aims to develop an improved coating that will offer enhanced service life, as well as mechanical and corrosion resistance. In this research, Ni-Nb2O5 composite coatings are electrodeposited on mild steel substrates using a sulphate electrolyte to achieve substantial corrosion resistance and structural integrity.
2. Experimental procedure
2.1. Materials preparation and electrodeposition
A nickel bath solution containing Nb2O5 particles generated a nickel-Nb2O5 composite using mild steel specimens in the size of 2 × 3 cm2. In addition to distilled water, analytical-grade chemicals were used for the preparation of the electrolyte. A description of the constituents of the bath and deposition parameters is provided in . Magnetic stirrers are used to stir the electrolyte prior to electrodeposition at 300 RPM. The cathode of electrodeposition consisted of mild steel, while the anode consisted of nickel.
Table 1. Bath composition and deposition conditions.
Pre-treatment of mild steel specimens prior to electrodeposition is fundamental to ensuring proper adhesion, corrosion resistance, and overall performance of the deposited coating. The following methods were deployed to clean the mild steel substrate
2.1.1. Cleaning
Mild steel surfaces are often contaminated with oils, greases, dust, and other impurities that can hinder proper coating adhesion. Cleaning methods such as solvent cleaning, alkaline cleaning, or ultrasonic cleaning are used to remove these contaminants.
2.1.2. Degreasing
Solvent or alkaline degreasing processes are employed to remove oils, grease, and other organic substances that can inhibit proper adhesion of the coating. This step is particularly important to ensure good bonding between the substrate and the electrodeposited coating.
2.1.3. Rinsing
After cleaning and activation steps, thorough rinsing is essential to remove any residual cleaning agents or acids. The residue left on the surface can negatively impact coating adhesion and performance.
2.1.4. Drying
Proper drying of the pre-treated surface is crucial to prevent the formation of water spots, residual chemicals, or rust. Thorough drying ensures that the substrate is ready to receive the electrodeposited coating (Yang et al. Citation2014; Hyie et al. Citation2022).
Several grades of Emery paper were used to polish the specimen, including 80, 800, 1000, and 2000. The mild steel plate was first immersed in HCl 10% solution and then in acetone solution to polish it. During the electrodeposition procedure, the temperature and current density were maintained at 50 °C and 5 amps/second, respectively. There were four Nb2O5 concentrations investigated in electro-deposition trials i.e. 0.25, 0.50, 0.75, and 1 gl−1.
2.2. Characterisation of coating
The X-ray diffractogram was obtained using a Malvern Pananalytical Empyren 3rd Generation diffractometer (Cu = 1.5405998 Ao). Images of the surface structure were obtained using a Carl Zeiss Sigma FESEM. Tests of microhardness were conducted using the Vicker’s tester Matsuzowa MMT-X7A. Innova SPM atomic force microscope was used for the acquisition of the AFM images. An electrochemical workstation CHI 608D was used to perform the corrosion test in a conventional glass cell at a temperature of 27 °C. The coating profile was evaluated using a nano new ST 400 profilometer. For scratch testing, the DUCOM unitest 750 scratch tester was used. The contact angle was found using a Drop shape analyzer-100.
3. Results and discussion
3.1. The microstructure of Ni-Nb2O5 composite coating
A rough morphology with homogeneous particle distribution has been observed (). The Nb2O5 particles are evenly distributed on the mild steel interface, resulting in a smooth, undistorted surface. The gaps and recessions are largely filled by the particles (Moreira et al. 2022). A majority of the particles are covered in metallic Ni, but only a few Nb2O5 particles are visible. These particles are visible in the form of solid flakes and nodular agglomerates.
3.2. XRD analysis
shows an XRD plot of the Nickel and Ni-Nb2O5 deposited coatings by the DC method. Nickel peaks with varying intensities are observed in deposits in crystallographic planes (111), (200), and (220). It is evident that the two deposits exhibit nickel peaks at the (111), (200), and (220) planes in varying intensities. The Fe peaks were visible in both the deposits at (020) and (121) planes. For determining the peak index, a nickel card (01-070-1849), Nb2O5 card (00-018-0910) and Fe card (96-900-6602) of ICDD were used. The Nb2O5 peak does not appear in this coating, because it has a lower percentage of Nb2O5 than nickel coatings. On XRD, there is no sign of the Nb2O5 peak, which is less intense than nickel (García et al. Citation2003). shows a composite coating that consists of nickel and Nb2O5 according to EDAX analysis. represents the elemental composition of the EDAX analysis.
Table 2. Elemental composition of Nickel- Nb2O5 coated specimen.
XRD data were used to estimate the texture coefficient (TC) of a Ni-Nb2O5 composite coating. Based on Be’rube and L'Esperance’s methodology, the texture coefficient is calculated using the following relation,
A peak intensity, I(hkl), is represented by Ni electrodeposits, and diffraction peaks are added together in this equation. In the ICDD card number (01-070-1849), index 0 refers to the intensities of peaks from the standard Ni sample. In , the texture coefficient of Ni composite coatings and Ni-Nb2O5 composite coatings are shown graphically. There was a preferred orientation with nickel coating having the highest texture coefficient (TC) value at (200) plane. An orientation change occurs at the (220) plane for Ni-Nb2O5 coatings. The results indicate that adding Nb2O5 to Ni electrodeposition changes the preferred orientation. When nickel was coated with Nb2O5, the coating morphology also changed. This is primarily due to the presence of Nb2O5 in the coating.
3.3. Corrosion test
In this study, corrosion resistance was evaluated for pure Ni and Ni-Nb2O5 steel substrates in corrosive solution (3.5% NaCl). The working electrodes were uncoated pure Ni and Ni- Nb2O5 steel substrates with 1 cm2 surface area that were submerged in the corrosive liquid for five minutes. In this measurement, saturated calomel electrodes served as the primary electrode and platinum wire served as the secondary electrode. The technique of tafel extrapolation and impedance spectroscopy was used to evaluate corrosion resistance in detail.
shows a Tafel plot of Ni-Nb2O5 coating (1 gl−1) at low Icorr values with high voltage. According to , the inclusion of Nb2O5 particles raised the Ni coating’s inhibitory efficacy from 44.3% to 87.9%.
Table 3. Tafel extrapolation data.
An instant, non-destructive method to evaluate the corrosion resistance of a wide range of materials is Electrochemical Impedance Spectroscopy (EIS). The sinusoidal voltage signal included an amplitude of 0.01 V. shows Nyquist plots. shows an electrical equivalent circuit that is fitted to the impedance parameters. In electrochemical impedance (EI) spectra, a larger surface area semicircle was witnessed for a greater Nb2O5 content coating, whereas the smaller surface area semicircles appeared for Ni coating, without particles or with fewer particles as shown in . represents the impedance spectroscopy data. This indicates a better corrosion resistance characteristic of Ni- Nb2O5 (1 gl−1) coating.
Figure 7. Equivalent circuit for the Nyquist plots. R: Resistance; CR: Current Rectifier; QR: Quick Response.

Table 4. Impedance spectroscopy data.
Ni-Nb2O5 composites exhibit a lower corrosion rate than pure Ni, owing to a number of factors. Further, deposits may contain Nb particles, which are intrinsically more corrosion-resistant than Ni in most aqueous environments. In addition, the microstructure of a coating can change from columnar to non-columnar. In columnar microstructures, the coatings have a high aspect ratio, with their growth direction being perpendicular to the substrate surface. This type of microstructure can create pathways for the corrosive medium to penetrate and reach the substrate, leading to higher corrosion rates. On the other hand, non-columnar microstructures are characterised by a lower aspect ratio, with their growth direction being parallel to the substrate surface (R and Chaira Citation2014; Chaira Citation2015; Shashanka Citation2019; Shashanka and Chaira Citation2017). This type of microstructure can create a more uniform coating with fewer pathways for the corrosive medium to penetrate and reach the substrate, leading to lower corrosion rates (R and Chaira Citation2014).
The incorporation of Nb2O5 particles acts as a nucleation site for crystal growth with smaller grain size composite coating and further adds to the corrosion resistance of the substrates (Mohan Reddy, Praveen, and Kumar Citation2017). As a result, Ni-Nb2O5 composites strongly decrease the corrosion rate of pure Ni compared to Ni-Nb2O5 composites because they have Nb particles in the deposits and a non-columnar coating microstructure.
3.4. Microhardness of Ni-Nb2O5 composite coating
An indenter equipped with diamonds from Vicker was used to test the microhardness of two load segments of 50 and 100 g over a dwell time of five seconds. shows the microhardness of nickel and nickel-Nb2O5 coatings (1 gl−1). Ni-Nb2O5 composite coatings exhibit higher microhardness than pure nickel coatings. Ni-Nb2O5 (1 gl−1) coatings are reported to have 321.3 HV and 270 HV hardness, respectively, compared to the nickel counterpart’s 213.6 HV and 195.78 HV. The Nb2O5 has a positive impact on the hardness in both the 50 and 100 g load segments. The inclusion of Nb2O5 particles in the composites disturbs the growth mechanism of nickel and reduces the grain boundary size and therefore requires more energy required for deformation (García et al. 2003).
3.5. Tribological characteristics of Ni-Nb2O5 composite coating
3.5.1. Atomic force microscopy
and show AFM images of nickel and nickel-Nb2O5(1 gl−1) coatings. Granular grains are present on the surface of the composite thin films that resemble flakes. A 3D image of the surface coatings shows a bulgy-like spherical structure with microscopic grain boundaries. The boundaries of larger grains appear to contain a good number of grains. Nickel-Nb2O5 coatings had a smaller particle size, seemed uniform, and were tougher than nickel coatings alone, indicating that nickel and Nb2O5 were dispersed evenly in the coating. As viewed in , Nb2O5 particles did not have much impact on the roughness characteristics.
Table 5. Roughness data.
3.6. Profilometry
The Ni-Nb2O5 (1 gl−1) electrodeposited coating surface profile is shown in and . The coating profiles typically range from 20 to 70 µm.
The coating texture appears to have fewer dense spikes, as can be observed in the surface profile of the Ni-Nb2O5 coated sample. A maximum height of 99.27 µm was measured for the particles.
3.7. Scratch test
The scratch test measures the coating’s adhesion to the substrate and its micromechanical properties. Rockwell diamond indent was used to test the scratch resistance of Ni-Nb2O5 coating (1 gl−1) at an incremental load of (0–20 N) at 0.1 mm/sec and a scratch length of 5 mm.
During scratch testing, as the indenter load increases, friction between the indentor and coating will increase plastic and elastic deformation. The scratch path is illustrated in by a SEM image. In , load vs. stroke is represented by a graph. There is no noticeable material piling at the scratch’s edges. The plastic deformation has occurred for the coating material during the scratch test and a small portion is found at the edges of the scratch. Neither the tested coating nor the substrate material has visible adhesion cracks or delamination.
3.8. Contact angle measurement
The measured contact angle of the coatings is given in and . The wetting behaviour of Ni-Niobium oxide (1gl−1) coatings can be determined by measuring contact angles following the incorporation of Niobium Pentoxide into the Ni matrix. Wettability is improved by the less porous nature of the composite coating, its low or no stress and its lack of agglomeration of particles on the outer coating. The improvement in contact angle can be found from 86.4° to 107.6°.
4. Conclusion
In this study, the effects of niobium pentoxide on the morphology, mechanical characteristics, and corrosion resistance of Ni electrodeposited coatings were researched. The following conclusions were drawn based on the results obtained in the study:
Smooth, compact and undistorted surfaces were obtained for the coatings with the flake-like visibility of the Nb2O5 particles.
XRD studies indicate that Nb2O5 modulates crystal size and orientation. The maximum TC was observed for 200 for Ni element.
As Nb2O5 concentration increased, corrosion resistance increased, with a maximum corrosion resistance observed for Nb2O5 (1 gl−1)
With the incorporation of Nb2O5 particles in a sulphate solution, the microhardness value has increased by about 44% when compared to pure nickel.
The inhibitor efficiency almost doubled with the inclusion of Nb2O5 particles in the coating (1 gl−1)
Nb2O5 particles produced a more steady and tougher coating with reduced particle sizes, with a maximum particle size of 99.27 µm.
The Ni-Nb2O5 (1 gl−1) coating material noticed a plastic deformity during the scratch test with the least heaping at the edges of the crack.
The hydrophobic nature of the protective coating was revealed by the inclusion of Nb2O5 (1 gl−1). As a result of these enhanced corrosion and mechanical properties, Ni-Nb2O5 electrodeposited coating could be utilised as an alternative protective coating to cater for the needs of the marine industry.
Disclosure statement
The authors declare that they have no known competing financial interests or personal relationships that could have appeared to influence the work reported in this article.
References
- ASM Handbook. 1993. Corrosion, vol 13. ASM International, Materials Park https://doi.org/10.1108/acmm.2003.12850daf.001
- Cardinal, M. F., P. A. Castro, J. Baxi, H. Liang, and F. J. Williams. 2009. “Characterization and Frictional Behavior of Nanostructured Ni–W–MoS2 Composite Coatings.” Surface and Coatings Technology 204 (1-2): 85–90. https://doi.org/10.1016/j.surfcoat.2009.06.037
- Chaira, D. 2015. “Development of Nano-Structured Duplex and Ferritic Stainless Steels by Pulverisette Planetary Milling Followed by Pressureless Sintering.” Materials Characterization 99: 220–229. https://doi.org/10.1016/j.powtec.2014.03.061
- Chen, Jinsong. 2011. “Characteristic of Ni-Al2O3 Nanocomposition Coatings.” Procedia Engineering 15: 4414–4418. https://doi.org/10.1016/j.proeng.2011.08.829
- Chen, Li., Liping Wang, Zhixiang Zeng, and Tao Xu. 2006. “Influence of Pulse Frequency on the Microstructure and Wear Resistance of Electrodeposited Ni–Al2O3 Composite Coatings, J.” Surface and Coatings Technology 201 (3-4): 599–605. https://doi.org/10.1016/j.surfcoat.2005.12.008
- de Oliveira, T. F., C. P. de Souza, and A. L. Lopes-Moriyama. 2020. “Acid Leaching and Thermal Treatments in the Obtaining of Mixed Oxides of Nb and Ta from Ferrocolumbite.” Minerals Engineering 147: 106157. https://doi.org/10.1016/j.mineng.2019.106157
- Devaneyan, S. P., and T. Senthilvelan. 2014. “Electro Co-deposition and Characterization of SiC in Nickel Metal Matrix Composite Coatings on Aluminium 7075.” Procedia Engineering 97: 1496–1505. https://doi.org/10.1016/j.proeng.2014.12.433
- Eslami, M., H. Saghafian, F. Golestani-Fard, and A. Robin. 2014. “Effect of Electrodeposition Conditions on the Properties of Cu–Si3N4 Composite Coatings.” Applied Surface Science 300: 129–140. https://doi.org/10.1016/j.apsusc.2014.02.021
- Feng, Q., T. Li, H. Teng, X. Zhang, Y. Zhang, C. Liu, and J. Jin. 2008. “Investigation on the Corrosion and Oxidation Resistance of Ni–Al2O3 Nano-Composite Coatings Prepared by Sediment Co-deposition.” Surface and Coatings Technology 202 (17): 4137–4144. https://doi.org/10.1016/j.surfcoat.2008.03.001
- Flores, J. F., A. Neville, N. Kapur, and A. Gnanavelu. 2009. “An Experimental Study of the Erosion–Corrosion Behavior of Plasma Transferred Arc MMCs.” Wear 267 (1–4): 213–222. https://doi.org/10.1016/j.wear.2008.11.015
- Fratari, R. Q., and A. Robin. 2006. “Production and Characterization of Electrolytic Nickel–Niobium Composite Coatings.” Surface and Coatings Technology 200 (12–13): 4082–4090. https://doi.org/10.1016/j.surfcoat.2005.02.168
- García, I., A. Conde, G. Langelaan, J. Fransaer, and J. P. Celis. 2003. “Improved Corrosion Resistance through Microstructural Modifications Induced by Codepositing SiC-Particles with Electrolytic Nickel.” Corrosion Science 45 (6): 1173–1189. https://doi.org/10.1016/s0010-938x(02)00220-2
- Ger, M. D., and B. J. Hwang. 2002. “Effect of Surfactants on Codeposition of PTFE Particles with Electroless Ni-P Coating.” Materials Chemistry and Physics 76 (1): 38–45. https://doi.org/10.1016/s0254-0584(01)00513-2
- Gurrappa, I., and L. Binder. 2008. “Electrodeposition of Nanostructured Coatings and Their Characterization: A Review.” Science and Technology of Advanced Materials 9 (4): 043001. https://doi.org/10.1088/1468-6996/9/4/043001
- He, L., Y. Tan, H. Tan, Y. Tu, and Z. Zhang. 2014. “Microstructure and Tribological Properties of WC-CeO2/Ni-Base Alloy Composite Coatings.” Rare Metal Materials and Engineering 43 (4): 823–829. https://doi.org/10.1016/s1875-5372(14)60092-8
- Huang, S., Y. Hu, and W. Pan. 2011. “Relationship between the Structure and Hydrophobic Performance of Ni–TiO2 Nanocomposite Coatings by Electrodeposition.” Surface and Coatings Technology 205 (13-14): 3872–3876. https://doi.org/10.1016/j.surfcoat.2011.01.065
- Hyie, K. M., S. Budin, H. Yusoff, N. Roseley, N. Nawawi, and S. Suhaimi. 2022. “Surface Activation Pretreatment for Cobalt Alloys Electroplating.” Jurnal Tribologi 32: 108–117. https://doi.org/10.1007/978-981-16-9949-8_33
- Jeyaraj, S., K. P. Arulshri, and S. Sivasankaran. 2016. “Investigations on Effect of Process Parameters of Electrodeposited Ni-Al2O3 Composite Coating Using Orthogonal Array Approach and Mathematical Modeling.” Archives of Civil and Mechanical Engineering 16 (1): 168–177. https://doi.org/10.1016/j.acme.2015.07.004
- Junli, W., X. Ruidong, and Y. Zhang. 2012. “Study on Characteristics of Ni-WB Composites Containing CeO2 Nano-Particles Prepared by Pulse Electrodeposition.” Journal of Rare Earths 30 (1): 43–47. https://doi.org/10.1016/s1002-0721(10)60636-9
- Karmakar, R., P. Maji, and S. K. Ghosh. 2021. “A Review on the Nickel Based Metal Matrix Composite Coating.” Metals and Materials International 27 (7): 2134–2145. https://doi.org/10.1007/s12540-020-00872-w
- Kavirajwar, J. S., S. Basavanna, and B. K. Devendra. 2022. “An Investigation on Corrosion Properties of Bright Zn‐Ni Alloy Coated Mild Steel.” Electrochemical Science Advances 2 (5): e2100097. https://doi.org/10.1002/elsa.202100097
- Kılıç, F., H. Gül, S. Aslan, A. Alp, and H. Akbulut. 2013. “Effect of CTAB Concentration in the Electrolyte on the Tribological Properties of Nanoparticle SiC Reinforced Ni Metal Matrix Composite (MMC) Coatings Produced by Electrodeposition.” Colloids and Surfaces A: Physicochemical and Engineering Aspects 419: 53–60. https://doi.org/10.1016/j.colsurfa.2012.11.048
- Koch, C. C. 2007. “Structural Nanocrystalline Materials: An Overview.” Journal of Materials Science 42 (5): 1403–1414. https://doi.org/10.1007/s10853-006-0609-3
- Krishnaveni, K., T. S. Narayanan, and S. K. Seshadri. 2008. “Electrodeposited Ni–B–Si3N4 Composite Coating: Preparation and Evaluation of Its Characteristic Properties.” Journal of Alloys and Compounds 466 (1-2): 412–420. https://doi.org/10.1016/j.jallcom.2007.11.104
- Kumar, J. N., A. S. Almalki, B. M. Prasanna, P. Prasad, N. Hebbar, and A. Alsubaie. 2022. “Polyethyleneimine–Chromium Oxide Nanocomposite Sensor with Patterned Copper Clad as a Substrate for CO2 Detection.” Journal of Electronic Materials 51 (11): 6416–6430. https://doi.org/10.1007/s11664-022-09879-y
- Lajevardi, S. A., and T. Shahrabi. 2010. “Effects of Pulse Electrodeposition Parameters on the Properties of Ni–TiO2 Nanocomposite Coatings.” Applied Surface Science 256 (22): 6775–6781. https://doi.org/10.1016/j.apsusc.2010.04.088
- Lampke, Thomas, Bernhard Wielage, Dagmar Dietrich, and Annette Leopold. 2006. “Details of Crystalline Growth in Co-deposited Electroplated Nickel Films with Hard (Nano) Particles.” Applied Surface Science 253 (5): 2399–2408. https://doi.org/10.1016/j.apsusc.2006.04.060
- Liang, Xue-Song, Jia-Hu Ouyang, Yu-Feng Li, and Ya-Ming Wang. 2009. “Electrodeposition and Tribological Properties of Ni-SrSO {Sub 4} Composite Coatings.” Applied Surface Science 255 (7): 4316–4321. https://doi.org/10.1016/j.apsusc.2008.11.043
- Ma, C., S. C. Wang, and F. C. Walsh. 2015. “Electrodeposition of Nanocrystalline Nickel–Cobalt Binary Alloy Coatings: A Review.” Transactions of the IMF 93 (2): 104–112. https://doi.org/10.1179/0020296714Z.000000000218
- Manohar, R., S. K. Rajappa, B. M. Praveen, and D. K. Bharath. 2021. “Investigation of Dolichandra Unguis-Cati Leaves Extract as a Corrosion Inhibitor for Mild Steel in Acid Medium.” Current Research in Green and Sustainable Chemistry 4(2021): 100113. https://doi.org/10.1016/j.crgsc.2021.100113
- Mohan Reddy, R., B. M. Praveen, and P. Kumar. 2017. “Corrosion Behaviour and Characterisation of Ni–Nb2O5 Composites Prepared by Pulse Electrodeposition.” Surface Engineering and Applied Electrochemistry 53 (2): 179–185. https://doi.org/10.3103/S1068375517020090
- Moreira, H. C., J. A. Oliveira, N. L. Carreño, R. M. Silva, I. T. Garcia, H. L. Costa, and J. H. Alano. 2022. “Effects of Niobium Pentoxide Nanoparticles on the Tribological Properties of Electrodeposited ZnNi Coatings.” Surface Topography: Metrology and Properties 10 (2): 024003. https://doi.org/10.1088/2051-672X/ac4ab6
- Muller, B., and H. Ferkel. 1998. “Al2O3-Nanoparticle Distribution in Plated Nickel Composite Films.” Nanostructured Materials 10 (8): 1285–1288. https://doi.org/10.1016/s0965-9773(99)00008-2
- Naveen Kumar J. R., P. Prasad, M. B. Savitha, K. S. Lokesh, S. Bharath Kumar, N. Navaneeth Gowda, and H. V. Rohith. 2022. “CO2 Detection: using the Polyethyleneimine–Cerium Oxide Nanocomposite Sensing Film Coated on Interdigitated Electrode Prepared from Copper Clad.” Materials Research Innovations 26 (1): 16–24. https://doi.org/10.1080/14328917.2020.1864943
- Naveen Kumar, J. R., P. Prasad, N Hebbar, M. B. Savitha, K. S. Lokesh, and N. Navaneeth Gowda. 2021. “A Potential Application of Polyethyleneimine-Reduced Graphene Oxide Nanocomposite Sensing Film Coated on Interdigitated Electrode Prepared from Copper-Clad for Carbon Dioxide Detection.” Materials Research Innovations 25 (6): 363–371. https://doi.org/10.1080/14328917.2020.1826674
- Naveen Kumar, J. R., D. Shrinivasa Mayya, B. M. Praveen, K. S. Lokesh, and P. Prasad. 2023. “Carbon Dioxide Sensing Using a PEI-Cr2O3-rGO Nanocomposite Sensor with Patterned Copper Clad as a Substrate.” Materials Research Innovations : 1–15. https://doi.org/10.1080/14328917.2023.2250634
- Naveen Kumar, J. R., D. Shrinivasa Mayya, M. B. Savitha, and P. Prasad. 2018. “Graphene-Based Metal Oxide Nanocomposites for Gas Sensing Application.” Int. J. Appl. Eng. Manage. Lett.(IJAEML) : 2.
- Oluyori, T., O. E. Olorunniwo, O. S. I. Fayomi, P. O. Atanda, and A. P. I. Popoola. 2017. “Performance Evaluation Effect of Nb2O5 Particulate on the Microstructural, Wear and Anti-corrosion Resistance of Zn–Nb2O5 Coatings on Mild Steel for Marine Application.” Journal of Bio- and Tribo-Corrosion 3 (4): 1–6. https://doi.org/10.1007/s40735-017-0108-x
- R, Shashanka, and D. Chaira. 2014. “Phase Transformation and Microstructure Study of Nano-Structured Austenitic and Ferritic Stainless Steel Powders Prepared by Planetary Milling.” Powder Technology 259: 125–136. https://doi.org/10.1016/j.powtec.2014.03.061
- Rani, R. A., A. S. Zoolfakar, A. P. O'Mullane, M. W. Austin, and K. Kalantar-Zadeh. 2014. “Thin Films and Nanostructures of Niobium Pentoxide: fundamental Properties, Synthesis Methods and Applications.” Journal of Materials Chemistry A 2 (38): 15683–15703. https://doi.org/10.1039/C4TA02561J
- Rathod, M. R., S. K. Rajappa, R. L. Minagalavar, B. M. Praveen, and A. A. Devendra Kittur. 2022. “Investigation of African Mangosteen Leaves Extract as an Environment-Friendly Inhibitor for Low Carbon Steel in 0.5M H2SO4.” Inorganic Chemistry Communications 140: 109488. https://doi.org/10.1016/j.inoche.2022.109488
- Robin, A., and R. Q. Fratari. 2007. “Deposition and Characterization of Nickel–Niobium Composite Electrocoatings.” Journal of Applied Electrochemistry 37 (7): 805–812. https://doi.org/10.1007/s10800-007-9315-3
- Rodrı́guez, J., A. Martı́n, R. Fernández, and J. E. Fernández. 2003. “An Experimental Study of the Wear Performance of NiCrBSi Thermal Spray Coatings.” Wear 255 (7–12): 950–955. https://doi.org/10.1016/s0043-1648(03)00162-5
- Saravanan, G., and S. Mohan. 2009. “Corrosion Behavior of Cr Electrodeposited from Cr (VI) and Cr (III)-Baths Using Direct (DCD) and Pulse Electrodeposition (PED) Techniques.” Corrosion Science 51 (1): 197–202. https://doi.org/10.1016/j.corsci.2008.10.005
- Sasi, A., M. Mondal, S. Dayal, and S. Kumar. 2015. “Electro Deposition of Nickel-Alumina Composite Coating.” Materials Today: Proceedings 2 (4–5): 3042–3048. https://doi.org/10.1016/j.matpr.2015.07.292
- Shashanka, R. 2019. “Non-Lubricated Dry Sliding Wear Behavior of Spark Plasma Sintered Nano-structured Stainless Steel.” Journal of Materials and Environmental Science. 10 (8). https://doi.org/10.17265/2161-6221/2016.5-6.001
- Shashanka, R., and D. Chaira. 2017. “Effect of Sintering Temperature and Atmosphere on Nonlubricated Sliding Wear of Nano-Yttria-Dispersed and Yttria-Free Duplex and Ferritic Stainless Steel Fabricated by Powder Metallurgy.” Tribology Transactions 60 (2): 324–336. https://doi.org/10.1080/10402004.2016.1168897
- Shashanka, R., D. Chaira, and K. S. Be. 2018. “Effect of Y2O3 Nanoparticles on Corrosion Study of Spark Plasma Sintered Duplex and Ferritic Stainless Steel Samples by Linear Sweep Voltammetric Method.” Archives of Metallurgy and Materials. https://doi.org/10.17265/2161-6221/2016.5-6.001
- Shrestha, N. K., M. Kawai, and T. Saji. 2005. “Co-Deposition of B4C Particles and Nickel under the Influence of a Redox-Active Surfactant and anti-Wear Property of the Coatings.” Surface and Coatings Technology 200 (7): 2414–2419. https://doi.org/10.1016/j.surfcoat.2004.08.192
- Shrestha, Nabeen K., Kazuaki Sakurada, Masabumi Masuko, and Tetsuo Saji. 2001. “Composite Coatings of Nickel and Ceramic Particles Prepared in Two Steps.” Surface and Coatings Technology 140 (2): 175–181. https://doi.org/10.1016/s0257-8972(01)01045-3
- Smrithi, S. P., N. Kottam, A. Narula, G. M. Madhu, R. Mohammed, and R. Agilan. 2022. “Carbon Dots Decorated Cadmium Sulphide Heterojunction-Nanospheres for the Enhanced Visible Light Driven Photocatalytic Dye Degradation and Hydrogen Generation.” Journal of Colloid and Interface Science 627: 956–968. https://doi.org/10.1016/j.jcis.2022.07.100
- Srivastava, M., V. W. Grips, and K. S. Rajam. 2007. “Electrochemical Deposition and Tribological Behaviour of Ni and Ni–Co Metal Matrix Composites with SiC Nano-Particles.” Applied Surface Science 253 (8): 3814–3824. https://doi.org/10.1016/j.apsusc.2006.08.022
- Torabinejad, V., M. Aliofkhazraei, A. S. Rouhaghdam, and M. H. Allahyarzadeh. 2017. “Tribological Properties of Ni-Fe-Co Multilayer Coatings Fabricated by Pulse Electrodeposition.” Tribology International 106: 34–40. https://doi.org/10.1016/j.triboint.2016.10.025
- Varadaraj, S., B. Shreeprakash, B. M. Praveen, and B. K. Devendra. 2022. “The Effect of Graphene Content on the Corrosion and Mechanical Properties of an Electrodeposited Ni-Graphene Coating.” Applied Surface Science Advances 11: 100310. https://doi.org/10.1016/j.apsadv.2022.100310
- Wang, Sheng-Chang, and Wen-Cheng J. Wei. 2003. “Kinetics of Electroplating Process of Nano-Sized Ceramic Particle/Ni Composite.” Materials Chemistry and Physics 78 (3): 574–580. https://doi.org/10.1016/s0254-0584(01)00564-8
- Wang, Z., L. Wu, Y. Qi, W. Cai, and Z. Jiang. 2010. “Self-Lubricating Al2O3/PTFE Composite Coating Formation on Surface of Aluminium Alloy.” Surface and Coatings Technology 204 (20): 3315–3318. https://doi.org/10.1016/j.surfcoat.2010.03.049
- Yang, H., X. Guo, X. Chen, and N. Birbilis. 2014. “A Homogenisation Pre-Treatment for Adherent and Corrosion-Resistant Ni Electroplated Coatings on Mg-Alloy AZ91D.” Corrosion Science 79: 41–49. https://doi.org/10.1016/j.corsci.2013.10.024
- Zhou, Y., F. Q. Xie, X. Q. Wu, W. D. Zhao, and X. Chen. 2017. “A Novel Plating Apparatus for Electrodeposition of Ni-SiC Composite Coatings Using Circulating-Solution Co-deposition Technique.” Journal of Alloys and Compounds 699: 366–377. https://doi.org/10.1016/j.jallcom.2016.12.331