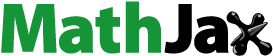
Abstract
In the present work, a polymeric thin layer that is poly(nigrosine) modified carbon paste electrode (PNMCPE) was established for the electrochemical analysis of catechol (CC) and hydroquinone (HQ) simultaneously and individually by using cyclic voltammetric (CV) technique. The PNMCPE shows a great electrocatalytic activity towards the oxidation of CC and HQ in phosphate buffer solution (PBS) of pH 7.4 than the BCPE. All experimental parameters are studied such as scan rate effect, effect of concentration, stability and interference study. With these studies optimised result was obtained at PNMCPE. The limit of detection (LOD) of CC and HQ was found to be 1.917 µM and 1.902 µM. and limit of quantification (LOQ) of CC and HQ was found to be 6.39 µM and 6.34 µM. The real sample analysis was successfully applied in tap water sample with a satisfactory recovery. Ultimately, this method can be used for the development of electrochemical sensor for sensitive analysis of CC and HQ.
1. Introduction
Nowadays, for the analysis of analytes voltammetric methods were used because of its high selectivity and faster response. Among other voltammetric methods such as linear sweep voltammetry, square wave voltammetry, differential pulse voltammetry, the Cyclic voltammetric method has proved to be good, simple, cost effective and very popular voltammetric method in their ability to detect the analytes at a very low concentration. Hence a cyclic voltammetry (CV) has become one of the most important methods in electroanalytical chemistry and it is moderately inexpensive and also the evaluation of obtained result is easy and simple (Rajendrachari et al. Citation2022, Citation2023; Rajendrachari and Kumaraswamy Citation2020). CV consists of three electrodes such as reference electrode (Ag/Ag+), counter electrode (Platinum wire) and working electrode (Carbon paste electrode, glassy carbon electrode, pencil graphite electrode). Over the past decades, carbon paste electrode is widely used for the analysis of oxidisable compounds. Generally, the bare carbon paste electrode (BCPE) mainly depends on the surface structure and the catalytic activity is quietly less in BCPE. Hence modification of BCPE is one of the important parameter. The modified carbon paste electrode shows very good sensitivity than the BCPE (Jayaprakash et al. Citation2021; Vajdle et al. Citation2020; Kumara et al. Citation2022).
The catechol (CC) and hydroquinone (HQ) are the phenolic compounds and isomers of dihydroxybenzene and abundantly used in industrial and agricultural fields. These compounds are very hazardous to environment and which is having many poisonous effects of health of human beings such as high toxicity of these two isomers will affect central nervous system, cause kidney damage and also low concentration can cause cancerous alterations. The CC and HQ are related to Induline and it is a mixture of phenazine based compounds. During the analytical resolution and quantification of CC and HQ produces an inevitable interference due to their closely analogues physical properties and chemical structure. Hence the detection of these compounds and also estimation of the danger and destruction of these phenolic compounds is very much necessary. Therefore, the development of electrochemical sensors for the detection of these two pollutants was developed for this cause (Hassan et al. Citation2018; Meskher and Achi Citation2022; Du et al. Citation2011; Sun et al. Citation2022; Ramya et al. Citation2021; Goulart and Mascaro Citation2016). Many analytical methods are done for the simultaneous analysis of CC and HQ. Some of them are mass spectrometry, pH based flow injection analysis, spectrophotometry, synchronous fluorescence, electro chemiluminescence, chromatography, electrochemical methods (Afkhami and Khatami Citation2001; Zhao et al. Citation2017; Marrubini et al. Citation2005; Harisha, Kumara Swamy, and Ebenso Citation2018; Nagaraja, Vasantha, and Sunitha Citation2001; Sun et al. Citation2000; Mesa and Mateos Citation2007; Pistonesi et al. Citation2006; Kuskur, Kumara Swamy, and Jayadevappa Citation2017; Ahammad et al. Citation2010; Gupta, Karimi-Maleh, and Sadegh Citation2015; Jiang et al. Citation2019). From the above listed data some of these have disadvantages because of its low sensitivity, high cost, more time consuming and difficult handling techniques. Electrochemical methods are preferred because of its easy handling, high sensitivity, feasibility and fast way in environmental analysis (Hu et al. Citation2012; Zheng et al. Citation2019; Huang, Cao, et al. Citation2020; Umar et al. Citation2020; Wang et al. Citation2022; Liu et al. Citation2023; T. Bhamer Jaafer Citation2017).
Nigrosine dye is also called as artificial synthetic black dye. This dye is used as negative staining of bacteria, fungus, yeast in the biological field. The main industrial application in making pen inks and used as colourant for varnishes, and also used as lacquer-used to shiny coating or furnishing on wood or metal like materials. This dye is also called by another name as solvent black 5 (Raril and Manjunatha Citation2018; Green Citation1990; Clark Citation1981).
In the present work poly(nigrosine) dye was used for the fabrication of working carbon paste electrode by electrochemical method by cyclic voltammetry method. For the detection of CC and HQ this modified electrode has good capacity to resolve the voltammetric peaks of CC and HQ. This fabricated electrode shows large surface area and ability of electron transfer is good. According to literature survey many modifiers are used for the detection of CC and HQ. Some of them are Silsesquioxane/MCPE (Silva et al. Citation2013), Influence of micelles/GCE (Peng and Gao Citation2006), Poly(brilliant blue) MCPE (Ganesh and Swamy Citation2015), Co3O4/MWWCNTs/GCE (Song et al. Citation2019), Poly(benzoguanamine)/MCPE (Chetankumar, Swamy, and Sharma Citation2019), Pt/C60/PGE (Zhu et al. Citation2020), etc. Compared to all these works poly (nigrosine) modified electrode shows low detection limit and good sensitivity and no work has reported for the analysis of CC and HQ by poly(nigrosine) modified carbon paste electrode. A very simple method was reported for the analysis of these two phenolic compounds.
2. Experimental sections
2.1. Reagents and chemicals
Poly (Nigrosine) was purchased from Sigma Aldrich. Catechol (CC) and Hydroquinone (HQ) was obtained from Himedia. 25 × 10−4 M of poly(nigrosine) and 25 × 10−4 M of CC were prepared by dissolving it in distilled water. The phosphate buffer Na2HPO4 and NaH2PO4.H2O was obtained from NICE chemicals. The required pH solution was prepared by taking mixture of Na2HPO4 and NaH2PO4.H2O of appropriate ratio.
2.2. Apparatus
The electrochemical studies were carried out in a conventional three electrode cell of electrochemical work station instrument CH-660c. The three electrodes were reference electrode, counter electrode and working electrode. The carbon paste electrode was used as a working electrode in this study.
2.3. Preparation of bare carbon paste electrode
Bare carbon paste electrode was prepared by hand-mixing 70:30 ratio of graphite powder and silicon oil in mortar for about 30–40 min till it becomes homogeneous mixture. Then the prepared carbon paste was filled to the teflon tube cavity which is present at the one end of teflon tube and it was rubbed on the butter paper to get uniformly shining surface.
3. Optimisation of the experimental parameters
3.1. Effect of supporting electrolyte
Supporting electrolyte shows a very important role on the chemical reactions and chemical substances which is to be measured in the process of development of electrochemical sensors. Therefore, the choice of supporting electrolyte is very important. In this work PBS (phosphate buffer solution) is used as a supporting electrolyte because of apparent redox peaks are shown. According to the study, compared to other supporting electrolyte that is formic acid, sodium acetate, PBS shows clear redox peaks and also because of the higher negative oxidation peak potential the oxidation reaction is accessible. Hence PBS is choosen as supporting electrolyte in this work.
3.2. Electropolymerisation of poly(nigrosine) on BCPE
The poly(nigrosine) polymer film was coated on bare carbon paste electrode by lectropolymerization method. The fabrication of BCPE was done by cyclic voltammetric technique. Then the poly(nigrosine) MCPE was prepared by taking 0.1 mM nigrosine with 0.1 M NaOH in the electrochemical cell. The potential range of -0.6 V to 1.4 V with sweep rate of 50 mVs−1 for 20 cycles was maintained. During the process the voltammogram decreases with the increase in number of cycles, shown in and later became constant, which suggests that polymer thin film of poly(nigrosine) was neatly deposited on the surface of BCPE.
3.3. Effect of multiple cycles
The electrocatalytic oxidation of 25 × 10−4 M CC (10 μM) in PBS of pH 7.4 was lookovered by varying multiple cycles from 5 to 25 cycles on the BCPE. The 20 multiple cycles show increased both anodic and cathodic peak current compared to other multiple cycles study, shown in . Therefore, 20 cycles were selected as ideal for the electropolymerisation of poly(nigrosine).
3.4. Influence of pH on the determination of CC at PNMCPE
The influence of pH of phosphate buffer solution on the investigation of CC at PNMCPE was precisely estimated from the pH range of 5.8–7.8. The change in the pH value has compelling contribution on the electrocatalytic oxidation of CC at PNMCPE by affecting both the peak current with reduction and oxidation peak potentials. The CV reported for 25 × 10−4 M CC at PNMCPE with different pH solution with sweep rate of 50 mVs−1. With the increase in pH, the oxidation peak potential shift towards more negative potential shown in . The graph of Epa versus pH was plotted which shows that Epa depends linearly on the pH value from the range 5.8–7.8. (inset ). Linear regression can be expressed as Epa (V) = 0.5841–0.0593 (pH), (r2 = 0.9859). The obtained slope was 59 mV/pH (inset ) nearer to the nearest theoretical value, i.e. 59 mV/pH (Raril and Manjunatha Citation2018; Green Citation1990; Clark Citation1981), which recommends equal number of protons and electrons are involved in reduction and oxidation mechanism. The graph of anodic peak current versus pH was plotted (). Maximum peak was procured at pH 7.4. Hence physiological pH 7.4 was choosen for further electrochemical studies in this work.
3.5. Electrochemical characterisation of poly(nigrosine) MCPE in standard K4[Fe(CN)6] system
A newly prepared solution of 1 mM K4[Fe(CN)6] in 1 M KCl (supporting electrolyte) was taken in an electrochemical cell and the CV experiment was performed with a three electrode system, PNMCPE as working electrode, Platinum as reference electrode and Ag/AgCl as counter electrode. The obtained cyclic voltammogram for the 1 mM K4[Fe(CN)6] at both BCPE (coloured red) and PNMCPE (coloured blue) and in KCl without potassium ferrocyanide (dotted line) at a scan rate of 50 mVs−1 was recorded (). The BCPE displays a sluggish electron transfer phenomenon, low redox peak current response was obtained. In the same identical condition, the PNMCPE displays good increment of redox peak currents with improvement in the electron transfer kinetics. This shows that the surface property of the PNMCPE changed significantly and it proves the electrocatalytic activity of PNMCPE. The active surface area of BCPE and MCPE can be calculated by Randles-Sevcik equation (EquationEquation (1)(1)
(1) ) (Arpitha, Kumara Swamy, and Shashikumara Citation2023; Banu, Swamy, and Ebenso Citation2022; Chetankumar and Swamy Citation2022). When compared to BCPE the active surface area of PNMCPE is maximum, which is tabulated in .
(1)
(1)
where A is area of electrode, Ipa is anodic peak current, C0 is concentration, n is number of electron changes, D0 is diffusion coefficient, ʋ is scan rate in mV/s.
Figure 3. Cyclic voltammogram of 1 mM potassium ferrocyanide at BCPE (coloured red), poly(nigrosine) MCPE (coloured blue) in 1 M KCl at scan rate of 0.05 Vs−1 and in KCl without potassium ferrocyanide (dotted line) at scan rate of 0.05 Vs−1.

Table 1. Comparison of area of BCPE and PNMCPE.
3.6. Electrochemical determination of individual CC and HQ at PNMCPE
To achieve optimum condition for the preparation of PNMCPE different parameters are studied such as potential range, number of cycles, solvent selection and scan rate were studied. By using cyclic voltammetric method, the cyclic voltammogram at a sweep rate of 50 mVs−1 for 10 μM of CC and HQ individually at BCPE and MCPE in 0.2 M PBS of 7.4 pH respectively. For these two isomers the reduction and oxidation peak potentials was calculated for both BCPE and MCPE media. At BCPE of CC (coloured red) and HQ (coloured red), the oxidation and reduction peak potential difference (ΔEp) was found to be 0.12 V and 0.08 V. At PNMCPE the CC (coloured blue) and HQ (coloured green) revealed the redox systems with ΔEp value 0.04 V and 0.02 V. The acquired result specify the oxidation of CC and HQ are irreversible and at BCPE it shows very slow transfer of electrons. (). The peak potential was higher for BCPE than PNMCPE. This shows there is increase of current response confirms the electrocatalytic activity of fabricated PNMCPE towards the electrochemical oxidation of CC and HQ. The oxidation mechanism of CC and HQ was shown in Schemes 1 and 2. The mechanism of CC and HQ followed by three main steps in which first step includes deprotonation by addition of electron and second step includes shifting of negative charge as followings and in the third step by the removal of proton due to simultaneous shifting of negative charge the final product 1,2-benzoquinone and 1,4-benzoquinone was obtained.
Figure 4. (a) Cyclic voltammograms of 10 µM CC in 0.2 M PBS solution of pH 7.4 at BCPE (coloured red) and poly(nigrosine) MCPE (coloured blue) at scan rate of 0.05 Vs−1. (b) Cyclic voltammograms of 10 µM HQ in 0.2 M PBS solution of pH 7.4 at BCPE coloured red) and poly(nigrosine) MCPE (coloured green) at scan rate of 0.05 Vs−1.

3.7. The scan rate effect on the peak current of CC and HQ
For the determination of the redox behaviour of CC and HQ on PNMCPE was either adsorption controlled or diffusion controlled, the effect of scan rate of CC (10 µM) and HQ (10 µM) on the surface of PNMCPE in pH 7.4 PBS was examined from the range 50–500 mV/s by cyclic voltammetric technique as shown in and , respectively. The redox peak current of CC and HQ increases with increase in the scan rate according to Randles-Sevcik equation. The oxidation peak potentials of both the analytes were shifted to slight positive side and reduction peak potentials was shifted towards negative side. To investigate the electrode process, the graph of peak current (Ipa) versus scan rate (ʋ) of CC and HQ was plotted, (CC) and (HQ) subsequently. A good linear relationship was attained for both CC and HQ, Ipa (µA) = 5.22 ʋ (mV/s) + 1.250 and Ipa (µA) = 6.11 ʋ (mV/s) + 3.09, with the correlation coefficient (r2) 0.994 (CC) and 0.998 (HQ) (inset and ) and graph of Ipa versus square root of scan rate (ʋ1/2) was plotted (CC) and (HQ) subsequently, Ipa (µA) = 4.92 ʋ (mV/s) + 8.69 and Ipa (µA) = 5.13 ʋ (mV/s) + 6.95 with the correlation coefficient (r2) 0.995 (CC) and 0.998 (HQ) (inset and ). This result affirms that the kinetics at the electrode surface is adsorption controlled at PNMCPE.
Figure 5. (a) Cyclic voltammogram of 10 µM CC in 0.2 M PBS of pH 7.4 at poly(nigrosine) MCPE with varied scan rate. (b) Graph of anodic peak current versus scan rate. (c) Graph of anodic peak current versus square root of scan rate.

Figure 6. (a) Cyclic voltammogram of 10 µM HQ in 0.2 M PBS of pH 7.4 at poly(nigrosine) MCPE with varied scan rate. (b) Graph of anodic peak current versus scan rate. (c) Graph of anodic peak current versus square root of scan rate.

The heterogeneous rate constant (k0) of CC and HQ was deceived by taking the peak potential difference (ΔΕp) from the experimental data and by using EquationEquation (2)(2)
(2) (Huang, Cao, et al. Citation2020; Chetankumar et al. Citation2021).
This equation was used for the experimental data when values of ΔΕp was found to be greater than 10 mV.
(2)
(2)
The k0 values for the oxidation of CC and HQ was tabulated in .
Table 2. the heterogeneous rate constant estimated from potential scan rate.
3.8. The effect of concentration and detection limit
The effect of concentration was studied for both CC and HQ at PNMCPE by employing cyclic voltammetric technique. The concentration of CC and HQ was varied from 10–100 μM. and revealed that, by increase in the concentration increases the current response. The inset and showcased the linearity of CC and HQ. The corresponding linear regression was explicit as follows:
for CC and HQ, respectively.
Figure 7. (a) Cyclic voltammogram of 10 µM CC in 0.2 M PBS of pH 7.4 at poly(nigrosine) MCPE with varied concentration at a scan rate of 0.05 Vs−1. (b) Graph of anodic peak current versus concentration.

Figure 8. (a) Cyclic voltammogram of 10 µM HQ in 0.2 M PBS of pH 7.4 at poly(nigrosine) MCPE with varied concentration at a scan rate of 0.05 Vs−1. (b) Graph of anodic peak current versus concentration.

The limit of detection and limit of quantification was calculated for both the analytes at PNMCPE by using EquationEquations (3)(3)
(3) and Equation(4)
(4)
(4) .
(3)
(3)
(4)
(4)
where M is the slope and is the standard deviation. The LOD for CC and HQ was found to be 1.92 µM and 1.91 µM and LOQ of CC and HQ was found to be 6.39 µM and 6.34 µM. The LOD and LOQ values obtained in this work was lower than the earlier noted literature, which was tabulated in .
Table 3. Comparison of the LOD of PNMCPE with other reported voltammetric methods.
3.9. Simultaneous analysis of CC and HQ
The successive determination of CC and HQ has been set down at poly(nigrosine) MCPE by cyclic voltammetric technique. The main purpose of this examination was to appeal the PNMCPE for the selective and sensitive determination of CC in the presence of HQ. The simultaneous analysis of these two analytes is quite difficult because of almost same oxidation peak potentials. In BCPE, the separation of oxidation peak of CC and HQ was not clearly occurred, they are overlapped (coloured black) oxidation peak potential was showed at 0.155 V. But in the case of PNMCPE, two well defined peaks of CC and HQ are observed (coloured red) with different peak potentials detected at 0.129 V and 0.032 V respectively with a potential difference between CC and HQ is 0.097 V. This result was enough to identify CC in the presence of HQ at poly(nigrosine) MCPE.
3.10. Stability of the poly(nigrosine) MCPE
The method of preparation of electrode is easy and fast. However, some of the electrodes shows low stability over lengthened time. Therefore, the stability of the electrode for a lengthened time is more important. The stability of the PNMCPE for the determination of CC (10 mM) and HQ (10 mM) in PBS of pH 7.4 for 40 successive cycles was examined (). Even after the 40 cycles there is a little decrease in peak current and there is no change in the peak potential. So there is no change in the peak potential. This examination tells that the PNMCPE shows excellent stability towards the electroactive species.
3.11. Interference study and the practical analytical application
The cyclic voltammetric technique was used for the interference study of the binary samples having CC (25 × 10−4 M), HQ (25 × 10−4 M) at PNMCPE. The peak current of CC increases with increase in the concentration (10–60 µM) by keeping steady concentration of HQ (). Similarly, by differing the concentration of HQ (40–60 µM), the peak current of HQ was also increased by keeping stable concentration of CC (). The obtained result confirms that by differing the concentration of one analyte does not change the Ipa and Ipc of the another analyte. This reveals that the PNMCPE shows excellent selectivity and sensitivity towards CC and HQ.
Figure 11. (a) the cyclic voltammogram obtained for CC (10–80 µM) in PBS of pH 7.4 at a scan rate of 0.05 Vs−1 in the presence of HQ (10 µM) at poly(nigrosine) MCPE. (b) The cyclic voltammogram obtained for HQ (10–90 µM) in PBS of pH 7.4 at a scan rate of 0.05 Vs−1 in the presence of CC (10 µM) at poly(nigrosine) MCPE.

Without any pre-treatment the tap water sample was taken as real sample for the evaluation of the viable applications of the PNMCPE for the simultaneous analysis of CC and HQ. To execute the recovery investigation, the tap water samples and known concentration of CC and HQ were added into the PBS of pH 7.4 by cyclic voltammetric technique. The corresponding cyclic voltammogram was recorded and tabulated in . The result obtained shows that the PNMCPE depicts admissible recovery for each addition of sample and having very good efficiency of PNMCPE for the determination of CC and HQ in real sample analysis.
Table 4. Detection of CC and HQ in tap water.
4. Conclusion
The present study exemplified an electropolymerisation of poly (nigrosine) on the surface of bare carbon paste electrode and also its application towards the electrochemical resolution of catechol and hydroquinone and this PNMCPE modified MCPE resolves the problem of overlapping oxidation peaks of CC and HQ into two well resolved separate peaks.
This modified electrode having the ability to determine CC and HQ individually and also in binary mixture. For the separation of analytes the PNMCPE showed very good sensitivity and selectivity. Furthermore, effect of scan rate, effect of concentration and pH study, interference study was examined. Stability of the working electrode was also checked and showed very good stability. Analytical application was also noted for the determination of these two phenolic compounds in a tap water sample with good recovery was obtained. Overall, the modified electrode showed great sensitivity, selectivity, stability for the determination of the examined biological molecules.
Disclosure statement
No potential conflict of interest was reported by the author(s).
References
- Afkhami, A., and H. A. Khatami. 2001. “Indirect Kinetic – Spectrophotometric Determination of Resorcinol, Catechol and Hydroquinone.” Journal of Analytical Chemistry 56 (5): 429–432. https://doi.org/10.1023/A:1016670818466
- Ahammad, A., S. Sarker, M. Rahman, and J. Lee. 2010. “Simultaneous Determination of Hydroquinone and Catechol at an Activated Glassy Carbon Electrode.” Electroanalysis 22 (6): 694–700. https://doi.org/10.1002/elan.200900449
- Arpitha, S. B., B. E. Kumara Swamy, and J. K. Shashikumara. 2023. “An Efficient Electrochemical Sensor Based on ZnO/Co3O4 Nanocomposite Modified Carbon Paste Electrode for the Sensitive Detection of Hydroquinone and Resorcinol.” Inorganic Chemistry Communications 152: 110656. https://doi.org/10.1016/j.inoche.2023.110656
- Banu, Rukaya, Kumara Swamy, and Eno E. Ebenso. 2022. “Voltammetric Analysis of Serotonin and Epinephrine in Presence of Guanine and Adenine at Bismarck Brown R Amplified Pencil Graphite Electrode.” Inorganic Chemistry Communications 144 (1): 109868. https://doi.org/10.1016/j.inoche.2022.109868
- Chetankumar, K., and Kumara Swamy. 2022. “Poly(6-Phenyl-1,3,5-Triazine-2,4-Diamine) Modified Carbon Paste Electrode for Uric Acid in Presence of Dopamine: A Voltammetric Study.” Analytical and Bioanalytical Electrochemistry 14 (8): 753–767.
- Chetankumar, K., B. E. Kumara Swamy, and S. C. Sharma. 2019. “Poly (Benzoguanamine) Modified Sensor for Catechol in Presence of Hydroquinone: A Voltammetric Study.” Journal of Electroanalytical Chemistry 849: 113365. https://doi.org/10.1016/j.jelechem.2019.113365
- Chetankumar, K., B. E. Kumara Swamy, S. C. Sharma, and S. A. Hariprasad. 2021. “An Efficient Electrochemical Sensing of Hazardous Catechol and Hydroquinone at Direct Green 6 Decorated Carbon Paste Electrode.” Scientific Reports 11 (1): 15064. https://doi.org/10.1038/s41598-021-93749-w
- Clark, G. 1981. Staining Procedures Used by the Biological Stain Commission. 4th ed., 412. Baltimore, London: Williams & Wilkins.
- Du, Haijun, Jianshan Ye, Jiagi Zhang, Xiaodan Huang, and Chengzhong Yu. 2011. “A Voltammetric Sensor Based on Grapheme Modified Electrode for Simultaneous Determination of Catechol and Hydroquinone.” Journal of Electroanalytical Chemistry 650 (2): 209–213. https://doi.org/10.1016/j.jelechem.2010.10.002
- Ganesh, P. S., and B. E. K. Swamy. 2015. “Simultaneous Electroanalysis of Hydroquinone and Catechol at Poly(Brilliant Blue) Modified Carbon Paste Electrode: A Voltammetric Study.” Journal of Electroanalytical Chemistry 756: 193–200. https://doi.org/10.1016/j.jelechem.2015.08.027
- Goulart, Lorena Athie, and Lucia Helena Mascaro. 2016. “Lucia Helena Mascaro, Glassy Carbon Electrode Modified with Carbon Nanotubes and NiO for the Determination of bisphenol-A, Hydroquinone and Catechol.” Electrochimica Acta 196: 48–55. https://doi.org/10.1016/j.electacta.2016.02.174
- Green, F. J. 1990. The Sigma Aldrich Handbook of Dyes, Stains and Indicators, 513–515. Milwaukee Wis: Aldrich Chemical.
- Gupta, V. K., H. Karimi-Maleh, and Roya Sadegh. 2015. “Simultaneous Determination of Hydroxylamine, Phenol and Sulphite in Water and Waste Water Samples Using a Voltammetric Nano Sensor.” International Journal of Electrochemical Science 10 (1): 303–316. https://doi.org/10.1016/S1452-3981(23)04994-5
- Harisha, K. V., B. E. Kumara Swamy, and Eno E. Ebenso. 2018. “Poly (Glycine) Modified Carbon Paste Electrode for Simultaneous Determination of Catechol and Hydroquinone: A Voltammetric Study.” Journal of Electroanalytical Chemistry 823: 730–736. https://doi.org/10.1016/j.jelechem.2018.07.021
- Hassan, Khalid Mahmoud, Abla Ahmed Hathoot, Mohamed Fathi Abo Oura, and Magdi Abdel Azzem. 2018. “Simultaneous and Selective Electrochemical Determination of Hydroquinone, Catechol and Resorcinol at Poly(1,5-Diaminonaphthalene)/Glassy Carbon-Modified Electrode in Different Media.” RSC Advances 8 (12): 6346–6355. https://doi.org/10.1039/c7ra13665j
- Hu, Fangxin, Shihong Chen, Chengyan Wang, Ruo Yuan, Dehua Yuan, and Cun Wang. 2012. “Study on the Application of Reduced Grapheme Oxide and Multiwall Carbon Nanotubes Hybrid Materials for Simultaneous Determination of Catechol, Hydroquinone and p –Cresol and Nitrite.” Analytica Chimica Acta 724: 40–46. https://doi.org/10.1016/j.aca.2012.02.037
- Huang, Liangliang, Yuanyuan Cao, and Dongfeng Diao. 2020. “Electrochemical Activation of Graphene Sheets Embedded Carbon Films for High Sensitivity Simultaneous Determination of Hydroquinone, Catechol and Resorcinol.” Sensors and Actuators B: Chemical 305: 127495. https://doi.org/10.1016/j.snb.2019.127495
- Huang, R., D. Liao, S. Chen, J. Yu, and X. Jiang. 2020. “A Strategy for Effective Electrochemical Detection of Hydroquinone and Catechol: Decoration of Alkalization-Intercalated Ti3C2 with MOF-Derived N-Doped Porous Carbon.” Sensors and Actuators B: Chemical 320: 128386. https://doi.org/10.1016/j.snb.2020.128386
- Jayaprakash, Gururaj Kudur, B. E. Kumara Swamy, Shashanka Rajendrachari, S. C. Sharma, and Roberto Flores-Moreno. 2021. “Dual Descriptor Analysis of Cetylpyridinium Modified Carbon Paste Electrodes for Ascorbic Acid Sensing Applications.” Journal of Molecular Liquids 334 (9): 116348. https://doi.org/10.1016/j.molliq.2021.116348
- Jiang, Danfeng, Jun Pang, Qiannan You, Tao Liu, Zhenyu Chu, and Wanqin Jin. 2019. “Simultaneous Biosensing of Catechol and Hydroquinone via a Truncated Cube-Shaped Au/PBA Nanocomposite.” Biosensors & Bioelectronics 124–125: 260–267. https://doi.org/10.1016/j.bios.2018.09.094
- Kumara, J. K. Shashi, B. E. Kumara Swamy, G. K. Jayaprakash, S. C. Sharma, Roberto Flores-Moreno, Kaustubha Mohanty, and S. A. Hariprasad. 2022. “Effect of TX-100 Pretreatment on Carbon Paste Electrode for Selective Sensing of Dopamine in Presence of Paracetamol.” Scientific Reports 12 (1): 20292. https://doi.org/10.1038/s41598-022-24387-z
- Kuskur, Chethan M., B. E. Kumara Swamy, and H. Jayadevappa. 2017. “Poly (Naphthol Green B) Modified Carbon Paste Electrode Sensor for Catechol and Hydroquinone.” Journal of Electroanalytical Chemistry 804: 99–106. https://doi.org/10.1016/j.jelechem.2017.09.039
- Liu, Guanying, Jun Liu, Peng Pan, Zhiyong Wang, Zhengchun Yang, Jun Wei, Peng Li, et al. 2023. “Electrochemical Sensor Based on Laser-Induced Preparation of MnOx-rGO Composites for Simultaneous Recognition of Hydroquinone and Catechol.” Microchemical Journal 185: 108234. https://doi.org/10.1016/j.microc.2022.108234
- Marrubini, G., E. Calleri, T. Coccini, A. F. Castoldi, and L. Manzo. 2005. “Direct Analysis of Phenol, Catechol and Hydroquinone in Human Urine by Coupled Column HPLC with Fluorimetric Detection.” Chromatographia 62 (1–2): 25–31. https://doi.org/10.1365/s10337-005-0570-3
- Mesa, J. A. G., and R. Mateos. 2007. “Direct Automatic Determination of Bitterness and Total Phenolic Compounds in Virgin Olive Oil Using a pH-Based Flow Injection Analysis System.” Journal of Agricultural and Food Chemistry 55 (10): 3863–3868. https://doi.org/10.1021/jf070235v
- Meskher, Hicham, and Fethi Achi. 2022. “Electrochemical Sensing Systems for the Analysis of Catechol and Hydroquinone in the Aquatic Environments: A Critical Review.” Critical Reviews in Analytical Chemistry 25: 1–14. https://doi.org/10.1080/10408347.2022.2114784
- Mohammed T. Bhamer Jaafer. 2017. “UV-a Activated ZnO Mediated Photocatalytic Decolourisation of Nigrosine (Acid Black 2) Dye in Aqueous Solution.” Journal of Geoscience and Environment Protection 11: 5.
- Nagaraja, P., R. A. Vasantha, and K. R. Sunitha. 2001. “A New Sensitive and Selective Spectrophotometric Method for the Determination of Catechol Derivatives and Its Pharmaceutical Preparations.” Journal of Pharmaceutical and Biomedical Analysis 25 (3–4): 417–424. https://doi.org/10.1016/s0731-7085(00)00504-5
- Peng, J., and Z. N. Gao. 2006. “Influence of Micelles on the Electrochemical Behaviours of Catechol and Hydroquinone and Their Simultaneous Determination.” Analytical and Bioanalytical Chemistry 384 (7–8): 1525–1532. https://doi.org/10.1007/s00216-006-0329-1
- Pistonesi, M. F., M. S. D. Nezio, M. E. Centurion, M. E. Palomeque, A. G. Lista, and B. S. F. Band. 2006. “Determination of Phenol, Resorcinol and Hydroquinone in Air Samples by Synchronous Fluorescence Using Partial Least-Squares.” Talanta 69 (5): 1265–1268. https://doi.org/10.1016/j.talanta.2005.12.050
- Rajendrachari, Shashanka, Nagaraj Basavegowda, Vinayak M. Adimule, Baris Avar, Prathap Somu, Saravana Kumar R M, and Kwang-Hyun Baek. 2022. “Assessing the Food Quality Using Carbon Nanomaterial Based Electrodes by Voltammetric Techniques.” Biosensors 12 (12): 1173. https://doi.org/10.3390/bios12121173
- Rajendrachari, Shashanka, Nagaraj Basavegowda, R. Vinaykumar, Damanapeta Narsimhachary, Prathap Somu, and Meng-Jen Lee. 2023. “Electrocatalytic Determination of Methyl Orange Dye Using Mechanically Alloyed Novel Metallic Glass Modified Carbon Paste Electrode by Cyclic Voltammetry.” Inorganic Chemistry Communications 155: 111010. https://doi.org/10.1016/j.inoche.2023.111010
- Rajendrachari, Shashanka, and B. E. Kumaraswamy. 2020. “Biosynthesis of Silver Nanoparticles Using Leaves of Acacia Melanoxylon and Their Application as Dopamine and Hydrogen Peroxide Sensors.” Physical Chemistry Research 8: 1–18. https://doi.org/10.22036/PCR.2019.205211.1688
- Ramya, R., D. Nathiya, P. Thivya, and J. Wilson. 2021. “Functionally Anchored Ag-TiO2 Nanoparticles on Guar Gum Based Nanocomposite for Simultaneous Determination of Hydroquinone, Catechol, Resorcinol and Nitrite.” Microchemical Journal 170: 106734. https://doi.org/10.1016/j.microc.2021.106734
- Raril, Chenthattil, and Jamballi G. Manjunatha. 2018. “Sensitive and Selective Analysis of Nigrosine Dye at Polymer Modified Electrochemical Sensors.” Analytical Journal of Bioanalytical and Electrochemistry 10: 372–382.
- Silva, P. S. D., B. C. Gasparini, H. A. Magosso, and A. Spinelli. 2013. “Electrochemical Behaviour of Hydroquinone and Catechol at a Silsequioxane-Modified Carbon Paste Electrode.” Journal of Brazilian Chemistry Society 24 (4): 695–699.
- Song, Yawen, Minggang Zhao, Xingtao Wang, Huiyan Qu, Ying Liu, and Shougang Chen. 2019. “Simultaneous Electrochemical Determination of Catechol and Hydroquinone in Seawater Using Co3O4/MWCNTs/GCE.” Materials Chemistry and Physics 234: 217–223. https://doi.org/10.1016/j.matchemphys.2019.05.071
- Sun, Y. G., H. Cui, Y. H. Li, and X. Q. Lin. 2000. “Determination of Some Catechol Derivatives by a Flow Injection Electrochemiluminescent Inhibition Method.” Talanta 53 (3): 661–666. https://doi.org/10.1016/s0039-9140(00)00550-6
- Sun, Lei., Hao Guo, Zhilan Pan, Bingqing Liu, Tingting Zhang, Meng Yang, Ning Wu, Junye Zhang, Fan Yang, and Wu Yang. 2022. “In Situ Reducing Platinum Nanoparticles on Covalent Organic Framework as a Sensitive Electrochemical Sensor for Simultaneous Detection of Catechol, Hydroquinone and Resorcinol.” Colloids and Surfaces A: Physicochemical and Engineering Aspects 635: 128114. https://doi.org/10.1016/j.colsurfa.2021.128114
- Sunil Kumar Naik, T. S., and B. E. Kumara Swamy. 2017. “Modification of Carbon Paste Electrode by Electrochemical Polymerization of Neutral Red and Its Catalytic Capability towards the Simultaneous Determination of Catechol and Hydroquinone: A Voltammetric Study.” Journal of Electroanalytical Chemistry 804: 78–86. https://doi.org/10.1016/j.jelechem.2017.08.047
- Umar, Ahmad, Ahmed A. Ibrahim, Rajesh Kumar, Tubia Almas, Priyanka Sandal, M. S. Al-Assiri, Mater H. Mahnashi, B. Z. Alfarhan, and S. Baskoutas. 2020. “Fern Shaped La2O3 Nanostructures as Potential Scaffold for Efficient Hydroquinone Chemical Sensing Application.” Ceramics International 46 (4): 5141–5148. https://doi.org/10.1016/j.ceramint.2019.10.258
- Umasankar, Yogeswaran, Arun Prakash Periasamy, and Shen-Ming Chen. 2011. “Electrocatalysis and Simultaneous Determination of Catechol and Quinol by Poly(Malachite Green) Coated Multi-Walled Carbon Nanotue Film.” Analytical Biochemistry 411 (1): 71–79. https://doi.org/10.1016/j.ab.2010.12.002
- Vajdle, Olga, Sanja Šekuljica, Valéria Guzsvány, László Nagy, Zoltán Kónya, Milka Avramov Ivić, Dušan Mijin, Slobodan Petrović, and Jasmina Anojčić. 2020. “Use of Carbon Paste Electrode and Modified by Gold Nanoparticles for Selected Macrolide Antibiotics Determination as Standard and in Pharmaceutical Preparations.” Journal of Electroanalytical Chemistry 873: 114324. https://doi.org/10.1016/j.jelechem.2020.114324
- Wang, Qi, Jinjin Zhang, Nannan Dou, and Jianying Qu. 2022. “Sensitive Simultaneous Determination of Catechol and Hydroquinone Based on Iron and Nitrogen Doped Carbon Nanonets Derived from MOFs.” Journal of Electroanalytical Chemistry 913: 116290. https://doi.org/10.1016/j.jelechem.2022.116290
- Zhao, L. J., B. Lv, H. Yuan, Z. Zhou, and D. Xiao. 2017. “A Sensitive Chemiluminescence Method for Determination of Hydroquinone and Catechol.” Sensors 7 (4): 578–588. https://doi.org/10.3390/s7040578
- Zheng, Gehua, Yuanyuan Zhang, Tianming Nie, Xingmao Jiang, Qijin Wan, Yawei Li, and Nianjun Yang. 2019. “Expanded Graphite Decorated with PdO@C Nanoparticles for Individual and Simultaneous Sensing of Multiple Phenols.” Sensors and Actuators B: Chemical 291: 362–368. https://doi.org/10.1016/j.snb.2019.04.072
- Zhu, Yun., Shuangshuang Huai, Jinglong Jiao, Qinqin Xu, Hai Wu, and Hong Zhang. 2020. “Fullerene and Platinum Composite-Based Electrochemical Sensor for the Selective Determination of Catechol and Hydroquinone.” Journal of Electroanalytical Chemistry 878: 114726. https://doi.org/10.1016/j.jelechem.2020.114726