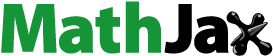
Abstract
The current study aimed to investigate multiple applications of synthesised cobalt oxide (Co3O4) nanoparticles (CONPs) via Aloe-Vera latex as green fuel by solution combustion approach. The structural parameters of prepared NPs were confirmed by several spectral methods, such as PXRD study shows the existence of cubic phases and crystalline behaviour of synthesised CONPs. Surface morphology and elemental analysis of prepared sample were illustrated by SEM-EDX and TEM techniques. The optical measurement of CONPs was discussed by UV-Visible absorbance spectral technique using Kubelka–Monk function and its energy band-gap is 1.77 eV. This study explored excellent photo-dye-degradation action (98.8% at 135 min) of synthesised photocatalyst on AR-88 dye (40 ppm) under the effect of irradiation of UV-light. Electrochemical behaviour of achieved CONPs was effectively examined by cyclic voltammetry (CV) and electrochemical impedance methods in 1 M KOH. Further, the excellent sensor performance on paracetamol chemical and glucose bio-molecules was examined using CO carbon paste electrode (COCPE). These analyses were revealed that CONPs is an effective and promising material for electrochemical sensor and photocatalytic studies.
1. Introduction
The development and remarkable applications of noble metal nanomaterials in recent years have benefitted many fields (Basnet et al. Citation2018; Ghosh Chaudhuri and Paria Citation2011; Ghotekar Citation2019; Sridevi et al. Citation2023; Vinayagam et al. Citation2023). These fields include solar cells, space technology, biomedicines, sensors, catalysis, cosmetics, healthcare, fuel cells, electrochemistry, biotechnology, energy devices, agriculture, imagination, food technology, optics and optical devices, pharmaceuticals, textiles, and water treatment. Among metal oxide nanoparticles, Co3O4 NPs have attracted a lot of attention because of their low price and great electroactivity. However, being a transition metal, cobalt may exist in a variety of oxidation states (including Co2+, Co3+, and Co4+). Because of its spinel crystal structure and several functionalities, Co3O4 is an established p-type semiconductor with antiferromagnetic properties (Diallo et al. Citation2015). Co3O4 NPs may be employed as photocatalysts to break down a variety of organic contaminants when exposed to visible light due to their direct optical band gaps of 1.48 and 2.19 eV (Shet et al. Citation2023).
Oxides of cobalt may be found in a variety of spin states, from low to high to intermediate. Co3O4’s physics is appealing from a basic perspective and for spintronic applications because of these spin states. Therefore, Co3O4 NPs are widely used in field effect transistors (Shet et al. Citation2023), energy storage (Shinde et al. Citation2006), catalysis (Sivachidambaram et al. Citation2017), anode material in Li-ion rechargeable batteries (Li, Xu, and Chen Citation2005), electrochromic sensors (Han, Yang, and Liu Citation2015), solar-cells (Sharma et al. Citation2015), and photocatalysts (Dewi, Ulizar, and Apriandanu Citation2019). Because of its high-quality biological applications, Co3O4 NPs have been shown to have antimicrobial, anticancer, antioxidant, antifungal, and enzyme-inhibiting characteristics (Anuradha and Raji Citation2019). Hydrothermal (Manjunatha Citation2022), Thermal decomposition (Manjunatha et al. Citation2020), Solution combustion (Shaalan et al. Citation2016), Microwave assisted (Ahmed et al. Citation2008), Microemulsion method (Kim et al. Citation2006), Spray pyrolysis (Askarinejad, Bagherzadeh, and Morsali Citation2010), Sonochemical (Pal and Chauhan Citation2010), Co-precipitation (Vikas et al. Citation2012), Sol gel (He et al. Citation2004), and solvothermal (Pallavolu et al. Citation2022) methods are just a few of the physical and chemical methods that have been used to synthesise Co3O4 NPs.
However, the aforementioned synthetic approaches have several drawbacks that may have a detrimental effect on the environment (Basnet et al. Citation2018). These include being expensive, time- and energy-consuming, requiring specialised tools for experimental work, and being unfriendly to the environment (Mahadeva Swamy et al. Citation2021; Manjunatha Citation2018; Puspalak et al. Citation2022). Some greener and more eco-accommodating approaches have been offered as a means of addressing the issue of energy imbalance and hazardous waste. Safer synthetic processes need to be developed and used; ideally, these would be low-cost, simple, non-toxic, clean, and efficient. The necessary Co3O4 NPs may be made rapidly, effectively, simply, and cheaply using biological resources like plants. Fabricating NPs from plant-derived materials is a viable alternative to traditional physical and chemical approaches (Ghotekar Citation2019). Here, we used Aloe Vera latex as a fuel in an effort to create Co3O4 NPs in an environmentally friendly manner. Co3O4 NPs’ photocatalytic and electrochemical sensor properties were studied.
2. Materials and methods
2.1. Preparation
The combustion technique was used in the production of CONPs utilising the eco-friendly fuel of Aloe Vera latex. The Forest Department of Bangalore University in India, collected the Aloe Vera leaves from the neighbouring agricultural areas. The gel was made by dissolving 5 g of cleaned, chopped Aloe Vera leaves in 10 mL of double-distilled water and agitating the mixture for 30 min. Aloe Vera plant extract fuel was produced using the resulting solution. Because plant extracts include biomolecules with the ability to reduce metal ions, such as proteins, carbohydrates, and coenzymes, their usage as reducing agents has also shown promise. These biomolecules also serve as the produced nanoparticles’ capping and stabilising agents. Compared to microbe-mediated synthesis, plant-mediated synthesis is faster and more scalable. It has also been shown to be effective, affordable, and widely accessible (Danish et al. Citation2022). Cobalt (II) nitrate hexahydrate (Co(NO3)2.6H2O) (Sigma Aldrich) was mixed with 10 mL of Aloe Vera at the stoichiometric ratio. The mixture was stirred well using a magnetic stirrer for 15 min. Before testing, the mixture was heated to 400 °C in a muffle furnace. When the solution is heated, a clear gel is produced. White foam developed from the gel, and then the foam caught fire. The sample’s rapid combustion filled the room with white powder residue. The whole thing only took around 5 min to finish. The final product was calcined at 500 °C for 3 h so that structural and other analyses could be performed. shows that the pictorial representation of the synthesis of CO NPs.
2.2. Photocatalytic analysis
The photo-degradation of AR-88 dye was performed under the effect of UV-light irradiation using synthesised CO NPs. This experimental analysis was conducted by using 40 mg of prepared CO NPs which was treated with 250 ml of AR-88 dye (40 ppm) solution taken in a glass reactor. During the experiment, 5 ml of dye solution was pipetted out at regular intervals until complete decomposition of dye solution and finally adsorption was monitored using UV–Visible spectrophotometer. To check the endurance of synthesised CO NPs, the experiment was repeated using the same photocatalyst after washing and drying with fresh dye.
3. Result and discussions
3.1. PXRD analysis
displays the XRD pattern of CO NPs synthesised by combustion utilising green (Aloe Vera latex) fuel. Powder X-ray diffraction investigation verified the structural characteristics of the produced NPs, demonstrating the presence of cubic phases and the crystalline behaviour of the synthesised CO NPs. The diffraction peaks of CO NPs appearing at 2θ = 19, 32, 37, 45, 59.9, and 66° are corresponds to (111), (220), (311), (222), (400), (511), (440), and (533) planes. When compared to cubic phase on standard JCPDS card number 042-1467 (Mahadeva Swamy et al. Citation2021), these diffraction peaks of CONPs are a good match. In CONPs, the highest intensity line occurs at 37°, which is in accordance with their increased crystallinity and lattice strain. The Debye-Scherrer formula (EquationEquation 1(1)
(1) ) was used to determine that typical crystallite size of CONPs is 36 nm. The lower particle size having larger surface area, which directly corresponds to enhanced photo-catalytic activity and electrochemical studies (Manjunatha Citation2018; Puspalak et al. Citation2022).
(1)
(1)
3.2. Surface morphology investigations
The structural modification with elemental composition and internal-surface morphology of synthesised CO NPs was examined by SEM-EDX techniques (). SEM micro-images of synthesised CO NPs clearly show a non-uniform, similarly cubic, and rod shape with well agglomerations as given in . These micrographs of CO NPs show characteristics of well agglomerated along with observable voids and pores due to impact of solution combustion method. The confirmation of synthesised CO NPs was proved by its elemental compositions using EDAX analysis ().
Further, it was extended to confirm the formation of CO NPS via TEM, HRTEM, and SAED investigations. displays TEM micrographs of CO NPs at different magnification, which is distinctly shows regularly cubic shaped with agglomerated in nature. Correspondingly, the HR-TEM micro-image with considering specific region, it shows d spacing values of 0.245 nm for crystal lattice of (111) plane as depicted in . The SAED patterns of CONPs showed a major superimposed bright spot-on ring patterns indicating the characteristics of polycrystalline in the nature of nanoparticles ().
3.3. Functional group examination
The existence of molecular bonding behaviour for synthesised CONPs was examined through the Fourier Transform Infra-red Spectroscopy (FT-IR) process recorded at wavelength ranges of 4000 and 400 cm−1 (). The existence of sharp and broad intensity peak at 3444 cm−1 specifies to stretching vibration of hydroxyl (OH–) ions that is assigned to the adsorbed H2O from atmosphere. Additionally, the smaller intensity bands at 1638 and 1385 cm−1 are assigned to asymmetric vibrations of unsaturated carbon-carbon (C = C) or carboxyl (C = O) functional groups. The presence of peak in finger-print region corresponds to metal–oxygen vibration linkages. Thus, bending vibrational peaks of Co-O linkage in CO nanoparticle was found to be 650 and 556 cm−1 (Charithra and Manjunatha Citation2021; Peng et al. Citation2017; Tang, Wang, and Chien Citation2008). Therefore, the appearance of two peaks at lower frequency region confirms its characteristic of CO NPs formation.
3.4. Diffused reflectance spectroscopy (DRS) analysis
The characteristic optical property of synthesised CONPs was investigated by DRS studies recorded at wavelength ranges of 800–200 nm (). The physical property (Eg-energy band-gap) of synthesised CONPs was found via Kubelka-Munk method (EquationEquation 2(2)
(2) ) with the correlation between the diffuse reflectance of the CF NPs (R), the absorption coefficient (K) and the scattering coefficient (S) (Manjunatha Citation2020);
(2)
(2)
Tauc equation used for Eg value and linear absorption coefficient (α) of synthesised CO nanomaterial, as expressed by EquationEquations (3)(3)
(3) and Equation(4)
(4)
(4) ;
(3)
(3)
(4)
(4)
The Eg value of the synthesised CONPs was measured to be 1.77 eV (Inset of ), which was derived by projecting the linearly fitted areas to [F(R)hν]2 = 0 from the [F(R)hν]2 vs. hν plot. Since visible light causes a greater photo-response, the resulting finding of rather narrow Eg values supports this hypothesis. The following formulae can be used to determine the band edge locations of synthesised CONPs:
(5)
(5)
(6)
(6)
Here, X-electronegativity of sample, E0-energy with respect to SHE for about 4.5 eV, and ECB and EVB indicates valence-band and conduction-band edge potential, respectively. Edge potential EVB and ECB of synthesised CONPs were found to be −1.73 and −0.04 eV, respectively with the help of electronegativity values of prepared material found at 1.88 eV. These recorded data confirm its excellent photo-catalytic application for lower Eg value of green fuel assisted synthesised CONPs (Basavaraju et al. Citation2021; Dinamani et al. Citation2023).
3.5. Photocatalytic degradation studies on dyes
The waste water that was disposed into surrounding water system released from many sectors like industrial or domestic regions are currently causing serious issues all over the world. To treat the diverse dye-contaminated water, photo-catalytic activity is a useful method that has recently attracted greater attention. It was a field that covered a wide range of reactions, including surface modification, water decontamination, and toxin elimination, dehydrogenation with hydrogen transfer, and complete or light oxidation processes. Due to their extensive range of electronic states and band energies, semiconductors are the most widely used heterogeneous photocatalysts because they possess intrinsic physical and chemical properties that distinguish them from metals and prevent the electron hole recombination caused by photo-activation. The band-gap from the full valence band to empty conduction-band is also taken into account.
The photocatalytic dye-degradation activity of synthesised CO NPs was explored on AR-88 dye under effect of irradiation of UV-light. Photocatalytic analysis on dye decolouration was carried out for 40 ppm AR-88 dye in presence of 20 mg of CO photocatalyst, which is measured its absorbance of dye under UV-Visible spectroscopy (). Under UV-light irradiation, CO photocatalyst showed a maximum photodegradation performance of 97.6% for the AR-88 dye after 135 min (). Also, shows that the dark and photolysis photo-degradation performances of AR-88 dye were 9.3 and 13.8%, respectively. Furthermore, by plotting (C/C0) vs. time under UV-light irradiation, we discovered that the half-time of photodegradation activity of AR-88 dye in the occurrence of the produced CO photocatalyst was 29.8 min (). After five cycles were completed under constant settings, displays an analysis of the CO photocatalyst’s capacity to recycle its performance on the discolouration of AR-88 dye.
Figure 7. (a) Spectral absorbance of CONPs with the variation of irradiation time under visible-light irradiation of AR-88 dye; (b) percentage decomposition of AR-88 dye under visible-light irradiation; (c) half time and (d) stability performance of CO NPs.

3.5.1. Photocatalytic studies of AR-88 dye
Under UV irradiation, CO photocatalyst demonstrates a strong catalytic activity in the photodegradation of AR-88 dye. The photocatalysis can be elucidated based on the appearance of chromophore groups and azo linkages (–N = N–) in dyes, which exhibit a prominent peak at 502 nm wavelength due to the n → π* transition that is photocatalytically degraded in the presence of UV radiation. Thus, the CO photo-catalyst was absorbed an energy from light radiation and produces several holes and electrons before the deterioration began at room temperature (EquationEquation 7(7)
(7) ). these resulting charges are directly responsible to creation of several free active radicals, such as OH•, and O2•− groups by gaining holes and electrons (EquationEquations 8
(8)
(8) and Equation9
(9)
(9) ). Hence, these radicals are identified as major contributors to the degradation process in the textile dyes (EquationEquation 10
(10)
(10) ) () (Lakshmi Ranganatha et al. Citation2020; Raghavendra, Nagaswarupa, Shashi Shekhar, Mylarappa, Surendra, Prashantha, Ravikumar, et al. Citation2021; Surendra et al. Citation2018; Surendra and Veerabhadraswamy Citation2017)
(7)
(7)
(8)
(8)
(9)
(9)
(10)
(10)
3.5.2. Scavengers analysis
This scavenging investigation reveals that the reactive oxidative species are involved directly in dye degradation process by the impact of various scavengers, like isopropanol (IP, 5 mM), ascorbic acid (AA, 5 mM), and ammonium oxalate (AO, 5 mM). The conducting experiment was very similar to the photocatalytic process, but before the photo-catalyst (CO NPs) was added to the AR-88 dye solution, the specific quantity of scavengers was added. This experimental photo-degradation analysis was shows 77.6, 47.4, and 19.6% for ascorbic acid, ammonium oxalate, and isopropanol scavengers, respectively (). This result confirms that isopropanol scavenger shows excellent photocatalytic activity by blocking the holes. Hence, photo-degradation performance of AR-88 dye was successfully achieved by holes as an effective tool under UV-light irradiation.
3.6. Electrochemical analysis
Observing the redox reaction of the completed cobalt oxide carbon paste electrode (COCPE) at scan rates ranging from 0.01 to 0.05 V/s in 1 M KOH with a 3-electrode system and CV and EIS methods yielded insight into the electrode’s electrochemical characteristics (). The good current measured in the potential range of −0.35–0.6 V yielded a CV voltammogram of COCPE. The reversibility of a redox reaction is measured by observing how anodic peak (Epa) and cathodic peak (peak Epc) potentials shift with time. The Randles-Sevecik equation (Raghavendra, Nagaswarupa, Shashi Shekhar, Mylarappa, Surendra, Prashantha, Basavaraju, et al. Citation2021; Shruthi et al. Citation2017) is used to determine the peak current (ip) and the potential difference (Ea,c), which both point to a lower value of 0.45 V, signifying more reversibility of COCPE.
Figure 10. (a) CV plot of COCPE at different scan rates in 1 M KOH solution; (b) EIS spectra; (c) relationship between the square root of the scan rate and the cathodic peak current (ip); (d) amperometry spectra of COCPE.

As can be shown in , the frequency range for the EIS analysis of the COCPE was 1 Hz to 1 MHz, and the amplitude was held constant at 5 mV. Research using the EIS-Nyquist method shows that the semicircle arises at high frequencies, where charge transfer resistance is strong, and at low frequencies, when material capacitance is high (Rashmi, et al. Citation2020). High capacitance and high charge-transfer resistance (Rct) are shown by EIS-Nyquist analysis of COCPE, which reveals that its smaller semicircle arc liable towards the X-axis is 32Ω.
In the high-frequency portion of the Nyquist plot, the semicircle represents charge transport through the electrode-electrolyte interface, while the straight line represents electrode capacitance in the low-frequency zone. The diameter of the semicircle arc on the real axis can be determined from the amount of the Rct (Ravi Kumar et al. Citation2017). For a reversible process, the Randles-Sevcik-Sevcikon utilises the current height (EquationEquation 11(11)
(11) ) (Raghavendra, Nagaswarupa, Shashi Shekhar, Mylarappa, Surendra, Prashantha, Basavaraju, et al. Citation2021).
(11)
(11)
The notations n, A, D, v, and Co are used to represent reaction parameters. These figures represent the quantity of electrons moved throughout the reaction. Electrode size and scanning rate (For the COCPE
(12)
(12)
also affects the chemical’s initial concentration.
In , we can see that the cathodic peak current (ip) for a COCPE is proportional to the square root of the scan rate (v1/2). Hydrogen diffusion limits the COCPE’s electrode reactivity, as seen by the tighter linear connection between ip and v1/2, as stated in (Ravi Kumar et al. Citation2017). Based on the slope of fitted line in and Equation (12), we get a value of 2.0165 × 10−5 cm2s−1 for the COCPE’s hydrogen diffusion co-efficient. displays amperometric i–t curve found for COCPE during potential sweep of −0.4–0.6 V, it shows current linearity with respect to time which corresponds to the prepared COCPE is stable in 1 M KOH solution.
3.6.1. Sensor activity of COCPE
The chemical and bio-molecules sensor characteristics of modified COCPE were carried out with variety of concentrations of analyte at specific scan-rate of 3 mV/s in 1 M KOH. displays the CV plot of COCPE for paracetamol detection with various concentrations of 1–5 mM in 1 M KOH. The stronger potential variations in redox peak potentials and appearance of extra peaks at 0.127, 0.057, and −0.093 V confirm sensing action of paracetamol chemical (Uma et al. Citation2023). Similarly, the sensing activity of COCPE towards glucose bio-molecule was reported in 1 M KOH electrolyte as shown in , it confirms the presence of peaks at different potentials at 0.183, −0.09, and −0.241 V, respectively. Even also the terminal peak in the + ve side move towards down (−ve current side), these distinctions confirm that our prepared COCPE senses both paracetamol and glucose in higher impact.
Figure 11. CV plot of COCPE (a) paracetamol (b) glucose bio-molecule sensors, amperometric i–t curve COCPE for the sensing of (a) paracetamol and (b) glucose bio-molecule using 1 M KOH electrolyte.

Amperometric i-t curves for paracetamol and glucose biomolecule sensing throughout a −0.4–0.6 V potential sweep are displayed in , respectively. displays the first current response of COCPE at paracetamol and glucose concentrations of 0 mM. In addition, the current response grew and reached a constant current under 3s after repeated injections of 1 mM of paracetamol or glucose at a sampling interval of 50 s. Rapid amperometric reaction to the oxidation of paracetamol and glucose demonstrated by the sensor is clear from this sort of activity. The current manufactured sensor has been discovered to have a sensitivity of 0.0102 A for paracetamol and 0.0103 A for glucose (Avinash et al. Citation2019).
shows the calibration curve, and variation of peaks current as a function of concentration of Paracetamol and glucose using CO electrode with fitted data (inset), respectively. The limit of detection (LOD) (Kusuma et al. Citation2020) of paracetamol and glucose are as low as 1.00 × 10−3 mol/L. The linear characteristic of the calibration curve confirms that the activity is a diffusion controlled in the measured concentration range.
4. Conclusions
The summary of the reported research encloses the systematic analysis of prepared CONPs by Aloe-Vera latex mediated solution combustion approach. An Examination of the Structure by using PXRD methods, it was determined that the crystallite size of CONPs was 36 nm and that it exhibits a cubic, crystalline structure. Using the UV-Visible absorbance spectrum method and the Kubelka-Monk function, the optical tests revealed a band-gap of 1.77 eV. It was reported that AR-88 dye (40 ppm) degraded by 98.8 percent in 135 min when exposed to UV light in the presence of CO photocatalyst. Enhanced electrochemical behaviour and sensor performance on paracetamol chemical and glucose bio-molecule of COCPE was recorded by CV and EIS methods in 1 M KOH. These analyses revealed that CONPs is an effective and promising material for electrochemical sensor and photocatalytic studies.
Code availability statement
Not applicable.
Disclosure statement
No potential conflict of interest was reported by the author(s).
Data availability statement
The data and materials that support the findings of this study are available with the corresponding author.
Additional information
Funding
References
- Ahmed, J., T. Ahmad, K. V. Ramanujachary, S. E. Lofland, and A. K. Ganguli. 2008. “Development of a Microemulsion-Based Process for Synthesis of Cobalt (Co) and Cobalt Oxide (Co3O4) Nanoparticles from Submicrometer Rods of Cobalt Oxalate.” Journal of Colloid and Interface Science 321 (2): 434–441. https://doi.org/10.1016/j.jcis.2008.01.052
- Anuradha, C. T., and P. Raji. 2019. “Effect of Annealing Temperature on Antibacterial, Antifungal and Structural Properties of Bio-Synthesized Co3O4 Nanoparticles Using Hibiscus rosa-sinensis.” Materials Research Express 6 (9): 095063. https://doi.org/10.1088/2053-1591/ab2f9e
- Askarinejad, A., M. Bagherzadeh, and A. Morsali. 2010. “Catalytic Performance of Mn3O4 and Co3O4 Nanocrystals Prepared by Sonochemical Method in Epoxidation of Styrene and Cyclooctene.” Applied Surface Science 256 (22): 6678–6682. https://doi.org/10.1016/j.apsusc.2010.04.069
- Avinash, B., C. R. Ravikumar, M. R. Anil Kumar, H. P. Nagaswarupa, M. S. Santosh, Aarti S. Bhatt, and Denis Kuznetsov. 2019. “Nano CuO: Electrochemical Sensor for the Determination of Paracetamol and D-Glucose.” Journal of Physics and Chemistry of Solids 134: 193–200. https://doi.org/10.1016/j.jpcs.2019.06.012
- Basavaraju, N., S. C. Prashantha, B. S. Surendra, T. R. Shashi Shekhar, M. R. Anil Kumar, C. R. Ravikumar, N. Raghavendra, and T. S. Shashidhara. 2021. “Structural and Optical Properties of MgNb2O6 NPs: Its Potential Application in Photocatalytic and Pharmaceutical Industries as Sensor.” Environmental Nanotechnology, Monitoring & Management 16: 100581. https://doi.org/10.1016/j.enmm.2021.100581
- Basnet, P., T. L. Chanu, D. Samanta, and S. Chatterjee. 2018. “A Review on Bio-Synthesized Zinc Oxide Nanoparticles Using Plant Extracts as Reductants and Stabilizing Agents.” Journal of Photochemistry and Photobiology. B, Biology 183: 201–221. https://doi.org/10.1016/j.jphotobiol.2018.04.036
- Charithra, M. M., and J. G. Manjunatha. 2021. “Electrochemical Sensing of Adrenaline Using Surface Modified Carbon Nanotube Paste Electrode.” Materials Chemistry and Physics 262: 124293. https://doi.org/10.1016/j.matchemphys.2021.124293
- Danish, Mir Sayed Shah, Liezel L. Estrella-Pajulas, I. M. Alemaida, M. L. Grilli, A. Mikhaylov, and T. Senjyu. 2022. “Green Synthesis of Silver Oxide Nanoparticles for Photocatalytic Environmental Remediation and Biomedical Applications.” Metals 12 (5): 769. https://doi.org/10.3390/met12050769
- Dewi, N. O. M., Y. Ulizar, and D. O. B. Apriandanu. 2019. “Green Synthesis of Co3O4 Nanoparticles Using Euphorbia heterophylla L. leaves Extract: Characterization and Photocatalytic Activity.” IOP Conference Series: Materials Science and Engineering 509: 012105.
- Diallo, A., A. C. Beye, T. B. Doyle, E. Park, and M. Maaza. 2015. “Green Synthesis of Co3O4 Nanoparticles via Aspalathus Linearis: Physical Properties.” Green Chemistry Letters and Reviews 8 (3–4): 30–36. https://doi.org/10.1080/17518253.2015.1082646
- Dinamani, M., B. S. Surendra, H. C. Ananda Murthy, N. Basavaraju, and Vijaya V. Shanbhag. 2023. “Green Engineered Synthesis of PbxZn1−xO NPs: An Efficient Electrochemical Sensor and UV Light-Driven Photocatalytic Applications.” Environmental Nanotechnology, Monitoring & Management 20: 100822. https://doi.org/10.1016/j.enmm.2023.100822
- Ghosh Chaudhuri, R., and S. Paria. 2011. “Core/Shell Nanoparticles: Classes, Properties, Synthesis Mechanisms, Characterization, and Applications.” Chemical Reviews 112 (4): 2373–2433. https://doi.org/10.1021/cr100449n
- Ghotekar, S. 2019. “A Review on Plant Extract Mediated Biogenic Synthesis of CdO Nanoparticles and Their Recent Applications.” Asian Journal of Green Chemistry 3: 187–200.
- Han, L., D. P. Yang, and A. Liu. 2015. “Leaf-Templated Synthesis of 3D Hierarchical Porous Cobalt Oxide Nanostructure as Direct Electrochemical Biosensing Interface with Enhanced Electrocatalysis.” Biosensors & Bioelectronics 63: 145–152. https://doi.org/10.1016/j.bios.2014.07.031
- He, T., D. Chen, X. Jiao, Y. Xu, and Y. Gu. 2004. “Surfactant-Assisted Solvothermal Synthesis of Co3O4 Hollow Spheres with Oriented-Aggregation Nanostructures and Tunable Particle Size.” Langmuir 20 (19): 8404–8408. https://doi.org/10.1021/la0488710
- Kim, D. Y., S. H. Ju, H. Y. Koo, S. K. Hong, and Y. C. Kang. 2006. “Synthesis of Nanosized Co3O4 Particles by Spray Pyrolysis.” Journal of Alloys and Compounds 417 (1–2): 254–258. https://doi.org/10.1016/j.jallcom.2005.09.013
- Kusuma, K. B., M. Manju, C. R. Ravikumar, H. P. Nagaswarupa, M. A. Shilpa Amulya, M. R. Anilkumar, B. Avinash, K. Gurushantha, and N. Ravikantha. 2020. “Photocatalytic and Electrochemical Sensor for Direct Detection of Paracetamol Comprising γ-Aluminium Oxide Nanoparticles Synthesized via Sonochemical Route.” Sensors International 1: 100039. https://doi.org/10.1016/j.sintl.2020.100039
- Lakshmi Ranganatha, V., S. Pramila, G. Nagaraju, B. S. Surendra, and C. Mallikarjunaswamy. 2020. “Cost-Effective and Green Approach for the Synthesis of Zinc Ferrite Nanoparticles Using AegleMarmelos Extract as a Fuel: Catalytic, Electrochemical, and Microbial Applications.” Journal of Materials Science: Materials in Electronics 31 (20): 17386–17403. https://doi.org/10.1007/s10854-020-04295-6
- Li, W. Y., L. N. Xu, and J. Chen. 2005. “Co3O4 Nanomaterials in Lithium‐Ion Batteries and Gas Sensors.” Advanced Functional Materials 15 (5): 851–857. https://doi.org/10.1002/adfm.200400429
- Mahadeva Swamy, M., B. S. Surendra, C. Mallikarjuna Swamy, S. Pramila, and N. D. Rekha. 2021. “Bio-Mediated Synthesis of ZnO Nanoparticles Using Lantana Camara Flower Extract: Its Characterizations, Photocatalytic, Electrochemical and anti-Inflammatory Applications.” Environmental Nanotechnology, Monitoring & Management 15: 100442. https://doi.org/10.1016/j.enmm.2021.100442
- Manjunatha, J. G. 2018. “A Novel Poly (Glycine) Biosensor towards the Detection of Indigo Carmine: A Voltammetric Study.” Journal of Food and Drug Analysis 26 (1): 292–299. https://doi.org/10.1016/j.jfda.2017.05.002
- Manjunatha, J. G. 2020. “A Surfactant Enhanced Graphene Paste Electrode as an Effective Electrochemical Sensor for the Sensitive and Simultaneous Determination of Catechol and Resorcinol.” Chemical Data Collections 25: 100331. https://doi.org/10.1016/j.cdc.2019.100331
- Manjunatha, J. G. 2022. Electrochemical Sensors Based on Carbon Composite Materials, Fabrication, Properties and Applications (IOP Series in Sensors and Sensor Systems). Bristol, UK: IOP Science.
- Manjunatha, J. G., C. Raril, N. Hareesha, M. M. Charithra, P. A. Pushpanjali, Girish Tigari, D. K. Ravishankar, S. C. Mallappaji, and Jayarame Gowda. 2020. “Electrochemical Fabrication of Poly (Niacin) Modified Graphite Paste Electrode and Its Application for the Detection of Riboflavin.” The Open Chemical Engineering Journal 14 (1): 90–98. https://doi.org/10.2174/1874123102014010090
- Pal, J., and P. Chauhan. 2010. “Study of Physical Properties of Cobalt Oxide (Co3O4) Nanocrystals.” Materials Characterization 61 (5): 575–579. https://doi.org/10.1016/j.matchar.2010.02.017
- Pallavolu, M. R., K. G. Krishna, G. Nagaraju, P. S. Babu, S. Sambasivam, and A. Sreedhar. 2022. “Rational Design of Cu-Doped Co3O4, Carbon Nanocomposite and Agriculture Crop-Waste Derived Activated Carbon for High-Performance Hybrid Supercapacitors.” Journal of Industrial and Engineering Chemistry 116: 428–437. https://doi.org/10.1016/j.jiec.2022.09.033
- Peng, Z. W., D. Yuan, Z. W. Jiang, and Y. F. Li. 2017. “Novel Metal-Organic Gels of Bis(Benzimidazole)-Based Ligands with Copper (II) for Electrochemical Selectively Sensing of Nitrite.” Electrochimica Acta. 238: 1–8. https://doi.org/10.1016/j.electacta.2017.03.121
- Puspalak, A., P. Chinnadurai, R. Prathibha, M. P. Kumar, S. G. Manjushree, V. UdayaKumar, and P. S. Adarakatti. 2022. “Cobalt Oxide Nanoparticles-Based Carbon Electrode for the Detection of Residual Nitrite in the Soil of Agricultural Fields.” Materials Research Innovations 27 (2): 100–109. https://doi.org/10.1080/14328917.2022.2085909
- Raghavendra, N., H. P. Nagaswarupa, T. R. Shashi Shekhar, M. Mylarappa, B. S. Surendra, S. C. Prashantha, C. R. Ravikumar, M. R. Anil Kumar, and N. Basavaraju. 2021. “Development of Clay Ferrite Nanocomposite: Electrochemical, Sensors and Photocatalytic Studies.” Applied Surface Science Advances 5: 100103. https://doi.org/10.1016/j.apsadv.2021.100103
- Raghavendra, N., H. P. Nagaswarupa, T. R. Shashi Shekhar, M. Mylarappa, B. S. Surendra, S. C. Prashantha, N. Basavaraju, C. R. Ravi Kumar, and M. R. Anil Kumar. 2021. “Electrochemical Sensor Studies and Optical Analysis of Developed Clay Based CoFe2O4 Ferrite NPs.” Sensors International 2: 100083. https://doi.org/10.1016/j.sintl.2021.100083
- Rashmi, B. N., Sujatha F. Harlapur, B. Avinash, C. R. Ravikumar, H. P. Nagaswarupa, M. R. Anil Kumar, K. Gurushantha, and M. S. Santosh. 2020. “Facile Green Synthesis of Silver Oxide Nanoparticles and Their Electrochemical, Photocatalytic and Biological Studies.” Inorganic Chemistry Communications 111: 107580. https://doi.org/10.1016/j.inoche.2019.107580
- Ravi Kumar, C. R., P. Kotteeswaran, V. Bheema Raju, A. Murugan, M. S. Santosh, H. P. Nagaswarupa, S. C. Prashantha, M. R. Anil Kumar, and M. S. Shivakumar. 2017. “Influence of Zinc Additive and pH on the Electrochemical Activities of β-Nickel Hydroxide Materials and Its Applications in Secondary Batteries.” Journal of Energy Storage 9: 12–24. https://doi.org/10.1016/j.est.2016.11.001
- Shaalan, N. M., M. Rashad, A. H. Moharram, and M. A. Abdel-Rahim. 2016. “Promising Methane Gas Sensor Synthesized by Microwave-Assisted Co3O4 Nanoparticles.” Materials Science in Semiconductor Processing 46: 1–5. https://doi.org/10.1016/j.mssp.2016.01.020
- Sharma, J. K., P. Srivastava, G. Singh, M. S. Akhtar, and S. Ameen. 2015. “Green Synthesis of Co3O4 Nanoparticles and Their Applications in Thermal Decomposition of Ammonium Perchlorate and Dye-Sensitized Solar Cells.” Materials Science and Engineering: B 193: 181–188. https://doi.org/10.1016/j.mseb.2014.12.012
- Shet, V. B., P. Senthil Kumar, V. Ramesh, Raja Selvaraj, C. Vibha, Shravya Rao, S. M. Pawan, et al. 2023. “Cocoa Pod Shell Mediated Silver Nanoparticles Synthesis, Characterization, and Their Application as Nanocatalyst and Antifungal Agent.” Applied Nanoscience 13 (6): 4235–4245. https://doi.org/10.1007/s13204-023-02873-8
- Shinde, V. R., S. B. Mahadik, T. P. Gujar, and C. D. Lokhande. 2006. “Supercapacitive Cobalt Oxide (Co3O4) Thin Films by Spray Pyrolysis.” Applied Surface Science 252 (20): 7487–7492. https://doi.org/10.1016/j.apsusc.2005.09.004
- Shruthi, B., B. J. Madhu, V. Bheema Raju, S. Vynatheya, B. Veena Devi, G. V. Jayashree, and C. R. Ravikumar. 2017. “Synthesis, Spectroscopic Analysis and Electrochemical Performance of Modified β-Nickel Hydroxide Electrode with CuO.” Journal of Science: Advanced Materials and Devices 2 (1): 93–98. https://doi.org/10.1016/j.jsamd.2016.12.002
- Sivachidambaram, M., J. J. Vijaya, K. Kaviyarasu, L. J. Kennedy, H. A. Al-Lohedan, and R. J. Ramalingam. 2017. “A Novel Synthesis Protocol for Co3O4 Nanocatalysts and Their Catalytic Applications.” RSC Advances 7 (62): 38861–38870. https://doi.org/10.1039/C7RA06996K
- Sridevi, H., M. Ramananda Bhat, P. Senthil Kumar, N. Manoj Kumar, and Raja Selvaraj. 2023. “Structural Characterization of Cuboidal α-Fe2O3 Nanoparticles Synthesized by a Facile Approach.” Applied Nanoscience 13 (8): 5605–5613. https://doi.org/10.1007/s13204-023-02780-y
- Surendra, B. S., and M. Veerabhadraswamy. 2017. “Microwave Assisted Synthesis of Polymer via Bioplatform Chemical Intermediate Derived from Jatropha Deoiled Seed Cake.” Journal of Science: Advanced Materials and Devices 2 (3): 340–346. https://doi.org/10.1016/j.jsamd.2017.07.004
- Surendra, B. S., M. Veerabhdraswamy, K. S. Anantharaju, H. P. Nagaswarupa, and S. C. Prashantha. 2018. “Green and Chemical-Engineered CuFe2O4: Characterization, Cyclic Voltammetry, Photocatalytic and Photoluminescent Investigation for Multifunctional Applications.” Journal of Nanostructure in Chemistry 8 (1): 45–59. https://doi.org/10.1007/s40097-018-0253-x
- Tang, C. W., C. B. Wang, and S. H. Chien. 2008. “Characterization of Cobalt Oxides Studied by FT-IR, Raman, TPR and TG-MS.” Thermochimica Acta 473 (1–2): 68–73. https://doi.org/10.1016/j.tca.2008.04.015
- Uma, B., K. S. Anantharaju, B. S. Surendra, K. Gurushantha, Sunil S. More, S. Meena, B. Hemavathi, and H. C. Ananda Murthy. 2023. “Influence of Ag on the Structural, Electrochemical, Antibacterial, and Photocatalytic Performance of the (CuO–Cu2O) Cu Nanocomposite.” ACS Omega 8 (11): 9947–9961. https://doi.org/10.1021/acsomega.2c07124
- Vikas, P., J. Pradeep, C. Manik, and S. Shashwati. 2012. “Synthesis and Characterization of Co3O4 Thin Film.” Soft Nanoscience Letters 2: 1–7.
- Vinayagam, Ramesh, Akshatha Hebbar, P. Senthil Kumar, Gayathri Rangasamy, Thivaharan Varadavenkatesan, Gokulakrishnan Murugesan, Shikhar Srivastava, Louella Concepta Goveas, N. Manoj Kumar, and Raja Selvaraj. 2023. “Green Synthesized Cobalt Oxide Nanoparticles with Photocatalytic Activity towards Dye Removal.” Environmental Research 216 (Pt 4): 114766. https://doi.org/10.1016/j.envres.2022.114766