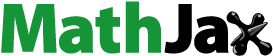
Abstract
The synthesis of transition molybdenum metal oxide (MoO3 nanoparticle) was achieved by bio-mediated (Aegle marmelos leaves) combustion process. The characteristics of prepared nanoparticle were well analysed by Powder-X-ray diffraction (PXRD), Scanning electron microscopy-Energy dispersive X-ray (SEM-EDAX), Fourier transform infra-red (FT-IR) spectroscopy, and UV–Visible absorption spectroscopy. The formation of orthorhombic phase structure and average crystallite size (23.6 nm) of MoO3 nanoparticle was confirmed by PXRD studies. The energy-band gap of achieved sample was found to be 3.1 eV recorded by UV–Visible absorption spectroscopy. This electron transition property directly influences the prepared sample as an excellent photo-catalyst for textile industrial Bromophenol Blue (BPB) dye under irradiation of UV light. The excellent photocatalytic performance on BPB dye was observed with 89.7% at 105 min, which is supported by its lower kinetic constants 17.42 × 10−3 min−1. The electrochemical measurements for investigating capacitance and resistance of modified graphite-MoO3 nanoparticle electrode under three-electrode system using 0.1 M HCl in the different scan rates 0.01–0.05 V/s by cyclic-voltammetry (CV) and electrochemical impedance spectroscopic (EIS) analysis. This investigation confirms the good sensing actions towards Lead, mercury, and Paracetamol molecules by impact of alteration in redox peak potentials.
1. Introduction
The nanotechnology has gaining increased attention due to tailoring the physico-chemical properties, such as surface area, size, and composition of samples on nano-scale for efficient actions on necessary multiple applications. The enhanced properties of nanomaterial like chemical stability, conductivity, catalytic and gas adsorption properties were achieved based on its larger surface area to volume ratio of nanoparticles (Jeevanandam et al. Citation2018; Malleshappa et al. Citation2015). Nowadays, the various benign of metal oxides, metal composites, and hybrid heterogeneous nanomaterials have drawn the attention of young researcher for its new multiple-application in almost all the areas (Ijaz et al. Citation2020; Malleshappa et al. Citation2015). The nanomaterials have played a significant role in novel multiple applications, such as drug transport, bio-therapeutics, pharmaceuticals, electronics, cosmetics, biotechnology, nano-fertilizers, ointments, lotions, water treatment, and electrochemical sensing applications (Baliga et al. Citation2020; Gudikandula and Charya Maringanti Citation2016; Iqbal et al. Citation2021). Generally, the nanoparticles are majorly prepared by physical, chemical, and biological methods as primarily utilised procedures. However, the bio-synthesis is the most cost-effective, simple, and eco-friendly process for providing an notable platform for the preparation of environmentally benign nanoparticle (Mahadevaswamy et al. Citation2022; Taghavi Fardood et al. Citation2023). Thus, the presented study focused on green mediated preparation of MoO3 nanomaterial using solution combustion process.
In the last few decades, transition metal oxides have attracted the research community in great deal due to their diversified applications. According to the survey, the great consideration of nanomaterials has been paid to the fabrication of various kinds of nanometal oxides like TiO2, ZnO, ZrO2, CuO, MgO, etc., semiconductors from the last few decades (Gurushantha et al. Citation2022; Mylarappa et al. Citation2022; Surendra et al. Citation2022; Taghavi Fardood et al. Citation2018; Uma et al. Citation2022). However, these metal oxides exhibit poor photocatalytic efficiency in the presence of visible light because of their wider energy band gap (Bhuvan Raj et al. Citation2021; Foroughi et al. Citation2014; Garkani Nejad et al. Citation2021; Tajik et al. Citation2020, Citation2021). Among these metal oxide, MoO3 nanomaterial finds the most fascinating materials due to its low-cost, higher the chemical stability, distinct, unique structural, optical, electrical, and mechanical properties. Thus, this kind of material was applied successfully in multiple applications of various fields, such as photocatalysis, light emitting diodes, therapies, gas sensing, and in many medical protocols (Barzinjy et al. Citation2020; Mazloum-Ardakani et al. Citation2012; Sneha et al. Citation2011). Photocatalysis process plays a vital role towards industrial waste water treatment in the presence of suitable photocatalyst having low band gap energy under light irradiation. The achieved nanomaterial having smaller crystallite size and larger surface is directly corresponding to higher the recombination rate of photo-generated electron-hole (e- -h) pairs and influences its larger photocatalytic efficiency (Chen et al. Citation2021; Gour and Jain Citation2019; Umadevi, Bindhu, and Sathe Citation2013).
Currently, the nanoparticles have drawn great interest towards sensors activities due to their physico-chemical properties ideal for different kinds of applications in electroanalytical devices (Rao et al. Citation2021; Sowmyashree et al. Citation2021). The prepared nanoparticle is a class of special anticipated due to their specific structural and optical properties. Thus, the MoO3 nanoparticles are cost-effective, conductive nature, and employed as an excellent nanosensor for electroanalytical sensing actions. The present study focused on medicinal plant leaf extracts assisted synthesis of MoO3 nanoparticle via combustion process and successfully applied in electrochemical sensing actions on Paracetamol, lead, and mercury heavy metals detection in 0.1 M HCl. Additionally, the photocatalytic performance of MoO3 nanoparticle on dye degradation studies and antibacterial properties were discussed in detail.
2. Materials and methods
2.1. Materials
The reagent used for the experimental synthesis of MoO3 NPs is ammonium molybdate tetrahydrate ((NH4)6Mo7O24.5H2O) as a starting material purchased from SD fine-chem Ltd. and were used as received without further purification.
2.2. Synthesis of MoO3 NPs
Stoichiometric ratios of 3.089 g of ammonium molybdate tetrahydrate as a starting material and 4 ml of Aegle marmelos extract were taken in a silica crucible and heated on a hot plate with continuous stirring for solidification (to form gel like consistency). The obtained homogeneous mixture was placed in muffle furnace for the combustion process maintained temperature of 495 ± 5 °C. The final product obtained by combustion reaction in the presence of green fuel preheated under muffle furnace for about 3–5 min was subjected to calcination at temperature of 500 ± 5 °C for about 3 h to attain high purity of sample (). Further, the yielded fine, grey green MoO3 sample was confirmed by several spectral characterisations.
2.3. Characterisation
The prepared MoO3 NP was done by the following characterisation techniques to confirm the material size, shape, surface properties, and morphology. X-ray diffraction studies confirm the specific shape and structure of prepared compound under ambient atmosphere at 30 °C by the X’pert Pro diffractometer (Cu-Kα radiation, λCu = 1.5148 Å). The MoO3 NPs were degassed under vacuity at 300 °C for 3 h before the measurement. The effective absorption study was estimated by UV–vis spectrophotometer (Agilent Cary 60 UV–visible spectrophotometer).
3. Results and discussions
3.1. P-XRD analysis
PXRD studies are a characteristic technique for measuring the crystallite size, nature of crystallinity, and purity with specific phase formation of prepared nanomaterial. The existence of sharp diffraction peaks in XRD pattern of MoO3 nanoparticle confirms its fine crystal-like particles as depicted in . The obtained diffraction planes at different angles (2θ) values of 12.68, 23.29, 25.68, 27.52, 29.78, 33.88, 38.82, 39.81, 45.51, 46.32, 50.14, 52.96, 57.62, 58.76, 62.76, 164.98, and 12.68° were corresponds to (020), (110), (040), (021), (130), (111), (060), (150), (141), (210), (161), (211), (171), (081), (251), and (190) peaks, respectively with orthorhombic structure with higher the purity due to its lack of impurity peaks associated MoO3 phases were noticed. The reported diffraction peaks of MoO3 nanoparticle are well-matched with JCPDS Card No.-05-0508 (Chen et al. Citation2009; Krishnamoorthy et al. Citation2014). However, the extreme intensity of the deflection points was witnessed in the plane (040) and its familiar crystalline dimension of the MoO3 nanoparticle was found to be 23.6 nm calculated with Scherrer’s formula (EquationEquation 1(1)
(1) ) (Nagabhushana, Samrat, and Chandrappa Citation2014; Surendra et al. Citation2021; Swamy et al. Citation2021).
(1)
(1)
Where β is full width half maxima of the peak, D is crystal size, λ is X-ray wavelength. Lesser the constituent dimension of MoO3 NPs illustrate the greater the micro straining and lesser displacement compactness in place of fine crystalline particles.
3.2. Scanning electron microscopy (SEM) examination
SEM analysis shows the effectiveness of internal surface morphology of MoO3 nanoparticle prepared bio mediated solution combustion technique at different magnifications as depicted in , respectively. These SEM micrographs confirm the existence of rod shaped structures and flake like with agglomeration morphology of MoO3 nanoparticle. According to the PXRD patterns, there are no impurities present in prepared nanomaterial and further, it was confirmed by determination of elemental composition using Energy dispersive X-ray (EDX) spectroscopy technique as shown in . This EDX spectral report revealed that the synthesised MoO3 nanoparticle incorporated by only Mo and O elements without any impurities. The atomic and weight percentage of these existed elements in synthesised MoO3 nanoparticle was presented in the table (inset ).
EDX technique is based on the emission of a specimen characteristic of X-rays by the impact of beam of high energy charged particles (electrons or protons) on examining material. An electron from a higher binding energy electron level falls into the core hole and an X-ray with the energy of the difference of the electron level binding energies is emitted. EDX analysis gives a spectrum that displays the peaks correlated to the elemental composition of the investigated sample. In addition, the elemental mapping of a sample can be created with this characterisation method to confirm the synthesised MoO3 nanoparticle consisting of only Mo and O elements as shown in .
3.3. Diffused reflectance spectroscopy (DRS) study
The optical examination of synthesised MoO3 NPs was achieved by measuring its energy band gap using DRS analysis carried out at wavelength ranges of 200–800 nm as shown in . The energy-band gap and relation between the reflectance of achieved nanomaterials (R), the absorption coefficient (K), and scattering coefficient (S) were calculated according to the reflectance spectral data by Kubelka-Munk function (EquationEquation 2(2)
(2) ).
(2)
(2)
Tauc relation for the band gap-energy and the linear absorption coefficient (α) of synthesised MoO3 NPs are given by
(3)
(3)
(4)
(4)
From the graphical plot of [F(R)hν]2 on y-axis versus incident photon energy (hγ) on x-axis, the energy-band gap (Eg) values of achieved MoO3 NPs were found to be 3.1 eV by the extrapolations of linearly dotted line as shown in . The lower band gap value shows higher photocatalytic action due to the quantum confinement effect and oxygen vacancies (Dinamani et al. Citation2023; Meenakshi et al. Citation2023).
3.4. Functional group analysis by FT-IR spectroscopy
The symmetric and asymmetric vibrations of functional groups present in synthesised MoO3 NPs were recorded by FT-IR spectral technique. The FT-IR spectrum of prepared MoO3 NPs from green (A. marmelos leaves) mediated combustion process was observed in between 400 and 4000 cm−1 as depicted in . The absence of broad peak around 3400–3600 cm−1 indicating the absence of water content in the crystal lattice of material and confirms its higher purity by the absence of additional peaks. The bands appeared in low frequency region representing the bending vibrations of metal-oxygen (M-O) linkages observed in the range between 1000 and 500 cm−1. The ascertained band at 987 and 867 cm−1 in finger-print area corresponding to the bending vibrations of co-ordination linkage of Mo-O-Mo group and the band at 641 cm−1 for Mo-O linkage (Sanchez et al. Citation2018; Xia et al. Citation2006).
3.5. Raman spectroscopy
The existence of functional groups and stretching modes of achieved MoO3 nanomaterial was examined by Raman spectral technique. The Raman peaks of synthesised MoO3 nanoparticle were recorded in the region of 500–4000 cm−1 as displayed in . The Raman peaks appeared at 995, 820, 666, 287, 157, and 126 cm−1 corresponding to octahedral stretching modes of synthesised MoO3 NPs. The existence of very sharp and high intense Raman band at 820 cm−1 is well-matched to the octahedral stretching modes of synthesised MoO3 nanoparticle recorded in the spanning region of 1000–600 cm−1. These major Raman bands at 995 and 820 cm−1 confirms the symmetric stretching vibrations of M=O and Mo–O–Mo linkages, respectively in synthesised MoO3 nanoparticle. Similarly, the octahedral bending vibrations and lattice modes of achieved MoO3 nanomaterial were observed in the region from 400 to 200 cm−1 and lower modes <200 cm−1.
4. Photocatalytic dye degradation activity
The current scenario of management of waste water discharged into surrounding water-bodies causing serious problems all over the world. Hence, the presented research work focused on photo-dye degradation activity using the prepared photo-catalyst under UV-Light irradiation. The photo-catalytic process is a significant cost-effective tool and has attracted greater attention towards degradation of various dye-contaminated water released from different sections (Gurushantha et al. Citation2023; Hegde et al. Citation2022; Moradnia et al. Citation2020; Pompapathi et al. Citation2023; Shanbhag et al. Citation2021). This process has covered a wide variety of chemical reactions, such as pollutant degradation, high catalytic reaction due to its larger surface area, hydrogen transfer by dehydrogenation process, and photo-oxidation routes. Thus, the MoO3 nanoparticle synthesised from green mediated combustion method has extensive semiconductor that is widely exploited as heterogeneous photocatalyst due to its intrinsic physio-chemical properties that distinguish them from metals and prevent the electron hole recombination caused by photo-activation.
The synthesised MoO3 nanoparticle has played a vital role in photocatalytic dye-degradation action on BB dye carried out under UV-Light irradiation with 20 ppm stock solution and 20 mg of photocatalyst. The photocatalytic dye degradation efficiency of achieved MoO3 nanoparticle on BB dye was examined by measuring the absorbance of light treated dye-samples (15 min) using UV-Visible spectroscopy as shown in . The photo-dye-degradation action of prepared MoO3 nanoparticle on dye under UV-Light irradiation was recorded to be 89.7% at 105 min as shown in . Additionally, the photo-dye-degradation stability actions of synthesised MoO3 nanoparticle were recorded by carried out with five cycles under above constant conditions. shows the photocatalytic action measurement of MoO3 photocatalyst towards recycle-ability performance on dye degradation activity. This obtained data reveals that MoO3 photocatalyst has excellent stability on photocatalytic action up to five cycles. Further, the half-time photo-dye degradation studies of MoO3 photocatalyst were examined by plotting (C/C0) v/s time under UV-light irradiation as shown in . The photo-dye degradation studies revealed that BPB dye degradation takes place 89.7% at 105 min and its half-time photo-dye degradation occurs at 42.6 min under UV-light irradiation (). The standard error bar plot of BPB dye degradation spectral studies is carried out under UV-light irradiation as shown in . The chemical kinetic studies for photo-dye degradation activity using MoO3 photocatalyst were studied as shown in . The comparative investigation of various nanomaterials in photocatalytic studies was discussed and its report has been mentioned in (Raghavendra et al. Citation2021; Surendra et al. Citation2018, Citation2020).
Figure 8. (a) Spectral absorbance of MoO3 nanoparticle with the variation of irradiation time under UV-light irradiation on BPB dye; (b) percentage decomposition of and (c) stability performance; (d) half-time dye-decolouration study; and (e) kinetics studies of MoO3 nanoparticle.
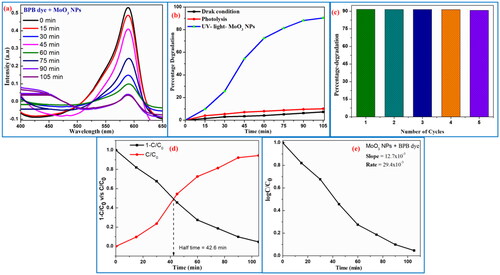
Table 1. The standard error of spectral absorbance plots for BPB dye.
Table 2. Comparative study of photocatalytic performance of various nanomaterials.
4.1. Photocatalytic dye degradation mechanism
The possible photocatalytic dye degradation mechanism of MoO3 photocatalyst on BB dye under UV-light irradiation is displayed in . The influences of light energy on synthesised photocatalyst surface show a photo-sensitization of BB dye under irradiated UV-light (EquationEquation 5(5)
(5) ). This process comprises equal numbers of electrons (
) and holes (
) by moving from valence band to the conduction band (EquationEquation 6
(6)
(6) ). In the photocatalytic dye decolouration reaction, the water and oxygen molecules are directly influencing the formation of superoxide radicals (
) by the presence of
in conduction band (EquationEquation 7
(7)
(7) ) and hydroxyl radicals
(EquationEquation 8
(8)
(8) ) by the presence of holes in valence band, respectively. The generated radicals are played a vital role in the photocatalytic dye decolouration activities by breaking of complex dye structures into eco-friendly by-products (Raghavendra et al. Citation2021; Surendra et al. Citation2018, Citation2020, Citation2023).
(5)
(5)
(6)
(6)
(7)
(7)
(8)
(8)
5. Electrochemical investigations of MoO3 NPs
The qualitative potential analysis of achieved MoO3 nanomaterial was examined by electrochemical studies using CV and EIS techniques in three electrode systems under 0.1 M HCl media as depicted in . The CV investigation of MoO3 NPs displays an effective action towards investigation of thermodynamics of reduction and oxidation reactions and specific-capacitance of achieved MoO3 nanomaterial was carried out by using prepared working electrode in scan rate of 0.01–0.05 V/s. The cycling potential of achieved MoO3 nanomaterial with graphite paste electrode was recorded by CV plot across a potential range of +1.0 to –1.3 V with a scan rate of 0.01–0.05 V/s as represented in .
Figure 10. (a) CV graph and (b) EIS-Nyquist plot of MoO3 NPs electrodes at the scan rate of 0.01–0.05 V/s in 0.1 M HCl.
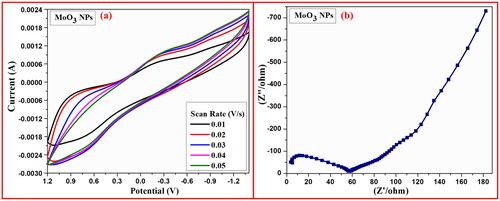
The specific capacitance of achieved MoO3 nanomaterial was determined by influence of encircled area received from CV plots using the following formula (EquationEquation 9(9)
(9) ).
(9)
(9)
Where; CSP is denotes the specific-capacitance (F/g), m is the mass of sample (g), I is current (A), v is scan rate (mV/s) and ΔV corresponds to potential windows (V). The measured lower specific-capacitance value from above mentioned formula for MoO3-Graphite paste electrode has been found to be 44.8 F/g. EIS-Nyquist plot of MoO3-Graphite paste working electrode revealed the nature of resistance and capacitance of synthesised MoO3 nanoparticle due to the impact of semicircle arc liable towards X-axis and straight line towards Y-axis. The EIS-Nyquist plot shows lower capacitance value found to be 58 Ω and its line more liable towards X-axis, indicating the higher capacitance synthesised MoO3 nanoparticle as shown in (Hareesha et al. Citation2021; Jamballi Manjunatha Citation2020; Jamballi Manjunatha 2013; Manjunatha Citation2018, Citation2020; Manjunatha et al. Citation2011; Prinith and Manjunatha Citation2023). The electrochemical examination of synthesised MoO3 nanoparticle is compared with other reported studies as shown in .
Table 3. Comparison of electrochemical analysis of different nanomaterials.
5.1. Cyclic voltammetry sensor actions of MoO3 NPs
Cyclic voltammetric curves of graphite-MoO3 NPs electrode show characteristic sensing activities towards Lead, mercury, and Paracetamol molecules under 0.1 M HCl at a specific scan rate of 0.03 V/s as shown in . shows the CV plot of prepared graphite-MoO3 NPs electrode for sensing action on Lead detection with different concentrations of 1–5 mM in 0.1 M HCl medium. Therefore, this analysis confirms that the good sensing performance of graphite-MoO3 NPs electrode on Lead ions due to its current density for oxidation potential peak appears at 0.9 V and reduction potential peaks at 0.6 V (). Similarly, the sensing analysis of prepared graphite-MoO3 NPs electrode on mercury and paracetamol molecules was recorded and its changing current density and redox potential peaks are observed at 0.9 and 0.6 V as shown in .
6. Conclusion
The present reported work revealed the preparation of MoO3 nanoparticle by bio-mediated (A. marmelos leaves) combustion process. The structural examinations of achieved sample were well studied by specific spectral techniques. The structural orthorhombic phase formation and purity of MoO3 nanoparticle was confirmed by PXRD spectra studies. The crystalline dimensions of MoO3 nanoparticle were found to be 23.6 nm calculated by Scherer’s method based on the existence of extreme intensity of the deflection points witnessed in the plane (040). Its energy-band gap was found to be 3.1 eV measured by UV–Visible absorption spectroscopy. This electron transition property has directly influenced the prepared sample act as an excellent photo-catalyst for textile industrial Bromophenol Blue (BPB) dye degradation with 89.7% at 105 min under irradiation of UV light, which is supported by its lower kinetic constants 17.42 × 10−3 min−1. The electrochemical measurements for investigating capacitance and resistance of modified graphite-MoO3 nanoparticle electrode under three-electrode system using 0.1 M HCl in the different scan rates of 0.01–0.05 V/s by CV and EIS analysis. This investigation confirms the good sensing action on characteristic sensing activities towards Lead, mercury, and Paracetamol molecules under 0.1 M HCl at specific scan rate of 0.03 V/s by its alteration of redox peak potentials. This study provides a cost-effective, eco-friendly, and potential new insight into multiple practical applications.
Disclosure statement
No potential conflict of interest was reported by the author(s).
Data availability statement
The data is available on request from the authors.
References
- Baliga, M. S., P. P. Mane, N. Joseph, and R. Jimmy. 2020. “Review on the Protective Effects of the Indigenous Indian Medicinal Plant, Bael (Aegle marmelos Correa), in Gastrointestinal Disorders.” In Bioactive Food as Dietary Interventions for Liver and Gastrointestinal Disease, 313–324. Elsevier.
- Barzinjy, A. A., S. M. Hamad, S. Aydın, M. H. Ahmed, and F. H. S. Hussain. 2020. “Green and Eco-Friendly Synthesis of Nickel Oxide Nanoparticles and Its Photocatalytic Activity for Methyl Orange Degradation.” Journal of Materials Science: Materials in Electronics 31 (14): 11303–11316. https://doi.org/10.1007/s10854-020-03679-y
- Bhuvan Raj, N., N. T. Pavithra Gowda, O. S. Pooja, S. K. Sukrutha, B. Purushotham, H. P. Nagaswarupa, M. R. Anil Kumar, B. S. Surendra, T. R. Shashi Shekhar, and S. C. Prashantha. 2021. “Eco-Friendly Synthesis of CeO2 NPs Using Aloe barbadensis Mill Extract: Its Biological and Photocatalytic Activities for Industrial Dye Treatment Applications.” Journal of Photochemistry and Photobiology 7: 100038. https://doi.org/10.1016/j.jpap.2021.100038
- Chen, Jun Song, Yan Ling Cheah, Srinivasan Madhavi, and Xiong Wen Lou. 2021. “Fast Synthesis of r-MoO3 Nanorods with Controlled Aspect Ratios and Their Enhanced Lithium Storage Capabilities.” The Journal of Physical Chemistry C 114 (18): 8675–8678. https://doi.org/10.1021/jp1017482
- Chen, Xiaohui, Weiwei Lei, Dan Liu, Jian Hao, Qiliang Cui, and Guangtian Zou. 2009. “Synthesis and Characterization of Hexagonal and Truncated Hexagonal Shaped MoO3 Nanoplates.” The Journal of Physical Chemistry C 113 (52): 21582–21585. https://doi.org/10.1021/jp908155m
- Dinamani, M., B. S. Surendra, H. C. Ananda Murthy, N. Basavaraju, and V. V. Shanbhag. 2023. “Green Engineered Synthesis of PbxZn1−xO NPs: An Efficient Electrochemical Sensor and UV Light-Driven Photocatalytic Applications.” Environmental Nanotechnology, Monitoring & Management 20: 100822. https://doi.org/10.1016/j.enmm.2023.100822
- Foroughi, Mohammad Mehdi, Hadi Beitollahi, Somayeh Tajik, Ali Akbari, and Rahman Hosseinzadeh. 2014. “Electrochemical Determination of N-Acetylcysteine and Folic Acid in Pharmaceutical and Biological Samples Using a Modified Carbon Nanotube Paste Electrode.” International Journal of Electrochemical Science 9 (12): 8407–8421. https://doi.org/10.1016/S1452-3981(23)11056-X
- Garkani Nejad, F., S. Tajik, H. Beitollahi, and I. Sheikhshoaie. 2021. “Magnetic Nanomaterials Based Electrochemical (Bio)Sensors for Food Analysis.” Talanta 228: 122075. https://doi.org/10.1016/j.talanta.2020.122075
- Gour, A., and N. K. Jain. 2019. “Advances in Green Synthesis of Nanoparticles.” Artificial Cells, Nanomedicine, and Biotechnology 47 (1): 844–851. https://doi.org/10.1080/21691401.2019.1577878
- Gudikandula, K., and S. Charya Maringanti. 2016. “Synthesis of Silver Nanoparticles by Chemical and Biological Methods and Their Antimicrobial Properties.” Journal of Experimental Nanoscience 11 (9): 714–721. https://doi.org/10.1080/17458080.2016.1139196
- Gurushantha, K., B. N. Swetha, S. Chinnam, K. Keshavamurthy, S. Meena, B. S. Surendra, S. Malini, and K. P. Roopa. 2023. “Structural, Optical, Photocatalytic, and Antimicrobial Attributes of Niobium Substituted Copper Nanoferrites.” Inorganic Chemistry Communications 156: 111162. volume https://doi.org/10.1016/j.inoche.2023.111162
- Gurushantha, K., K. S. Anantharaju, N. Kottam, K. Keshavamurthy, C. R. Ravikumar, B. S. Surendra, A. Murugan, and H. C. A. Murthy. 2022. “Synthesis of ZrO2:Dy3+ Nanoparticles: Photoluminescent, Photocatalytic and Electrochemical Sensor Studies.” Adsorption Science & Technology 2022: 1–13. https://doi.org/10.1155/2022/5664344
- Hareesha, N., J. G. Manjunatha, B. M. Amrutha, P. A. Pushpanjali, M. M. Charithra, and N. Prinith Subbaiah. 2021. “Electrochemical Analysis of Indigo Carmine in Food and Water Samples Using a Poly(Glutamic Acid) Layered Multi-Walled Carbon Nanotube Paste Electrode.” Journal of Electronic Materials 50 (3): 1230–1238. https://doi.org/10.1007/s11664-020-08616-7
- Hegde, S. S., R. S. C. Bose, B. S. Surendra, S. Vinoth, P. Murahari, and K. Ramesh. 2022. “SnS-Nanocatalyst: Malachite Green Degradation and Electrochemical Sensor Studies.” Materials Science and Engineering: B 283: 115818. https://doi.org/10.1016/j.mseb.2022.115818
- Ijaz, I., E. Gilani, A. Nazir, and A. Bukhari. 2020. “Detail Review on Chemical, Physical and Green Synthesis, Classification, Characterizations and Applications of Nanoparticles.” Green Chemistry Letters and Reviews. 13 (3): 223–245. https://doi.org/10.1080/17518253.2020.1802517
- Iqbal, A., A. ul Haq, G. A. Cerrón-Calle, S. A. R. Naqvi, P. Westerhoff, and S. Garcia-Segura. 2021. “Green Synthesis of Flower-Shaped Copper Oxide and Nickel Oxide Nanoparticles via Capparis Decidua Leaf Extract for Synergic Adsorption-Photocatalytic Degradation of Pesticides.” Catalysts 11 (7): 806. https://doi.org/10.3390/catal11070806
- Jamballi Manjunatha, G. 2020. “Electrochemical Fabrication of Poly (Niacin) Modified Graphite Paste Electrode and Its Application for the Detection of Riboflavin.” Open Chem Engineering 14: 52–62.
- Jeevanandam, J., A. Barhoum, Y. S. Chan, A. Dufresne, and M. K. Danquah. 2018. “Review on Nanoparticles and Nanostructured Materials: History, Sources, Toxicity and Regulations.” Beilstein Journal of Nanotechnology 9: 1050–1074. https://doi.org/10.3762/bjnano.9.98
- Krishnamoorthy, Karthikeyan, Mariappan Premanathan, Murugan Veerapandian, and Sang Jae Kim. 2014. “Nanostructured Molybdenum Oxide-Based Antibacterial Paint: Effective Growth Inhibition of Various Pathogenic Bacteria.” Nanotechnology 25 (31): 315101. https://doi.org/10.1088/0957-4484/25/31/315101
- Mahadevaswamy, M., S. R. Paniyadi, A. Lakshmikanthan, S. A. Swamirayachar, M. P. G. Chandrashekarappa, and K. Giasin. 2022. “Plant-Mediated Synthesis of NiO(II) from Lantana Camara Flowers: A Study of Photo-Catalytic, Electrochemical, and Biological Activities.” Journal of Materials Research and Technology.
- Malleshappa, J., H. Nagabhushana, S. C. Sharma, Y. S. Vidya, K. S. Anantharaju, S. C. Prashantha, B. Daruka Prasad, H. Raja Naika, K. Lingaraju, and B. S. Surendra. 2015. “Leucas aspera Mediated Multifunctional CeO2 Nanoparticles: Structural, Photoluminescent, Photocatalytic and Antibacterial Properties.” Spectrochimica Acta. Part A, Molecular and Biomolecular Spectroscopy 149: 452–462. https://doi.org/10.1016/j.saa.2015.04.073
- Manjunatha, J. G. 2013. “Electrochemical Studies of Dopamine, Ascorbic Acid and Their Simultaneous Determination at a Poly (Rosaniline) Modified Carbon Paste Electrode: A Cyclic Voltammetric Study.” Analytical and Bioanalytical Electrochemistry 5 (4): 426–438.
- Manjunatha, J. G. 2018. “Highly Sensitive Polymer Based Sensor for Determination of the Drug Mitoxantrone.” Journal of Surface Science and Technology 34 (1–2): 74–80. https://doi.org/10.18311/jsst/2018/15838
- Manjunatha, J. G. 2020. “A Surfactant Enhanced Graphene Paste Electrode as an Effective Electrochemical Sensor for the Sensitive and Simultaneous Determination of Catechol and Resorcinol.” Chemical Data Collections 25: 100331. https://doi.org/10.1016/j.cdc.2019.100331
- Manjunatha, J. G., Swamy, K. Mamatha, G. Gilbert, and O. S. Bailure. 2011. “Poly(Maleic Acid) Modified Carbon Paste Electrode for Simultaneous Detection of Dopamine in the Presence of Uric Acid: A Cyclic Voltammetric Study.” Analytical and Bioanalytical Electrochemistry 3 (2): 146–159.
- Mazloum-Ardakani, Mohammad, Zahra Taleat, Alireza Khoshroo, Hadi Beitollahi, and Hossein Dehghani. 2012. “Electrocatalytic Oxidation and Voltammetric Determination of Levodopa in the Presence of Carbidopa at the Surface of a Nanostructure Based Electrochemical Sensor.” Biosensors & Bioelectronics 35 (1): 75–81. https://doi.org/10.1016/j.bios.2012.02.014
- Meenakshi Giridhar, B. C., Manjunath, Surendra, B. S. K. N. Harish, S. C. Prashantha, T. Kiran, B. Uma, and H. C. Ananda Murthy. 2023. “Sustainable Approach of La Doped CuFe2O4 Nanomaterial for Electrochemical Lead and Paracetamol Sensing Action with Multiple Applications, Scientific Report.” Nature 13: 17821.
- Meenakshi, Giridhar, B. C. Manjunath, S. C. Prashantha, T. Prashanth, and B. S. Surendra. 2023. “Super Capacitor, Electrochemical Measurement and Sun Light Driven Photocatalytic Applications of CuFe2O4 NPs Synthesized from Bio-Resource Extract.” Sensors International 4: 100237. https://doi.org/10.1016/j.sintl.2023.100237
- Moradi, Sajjad, and Saeid Taghavi Fardood. 2019. “Eco-Friendly Synthesis and Characterization of α-Fe2O3 Nanoparticles and Study of Their Photocatalytic Activity for Degradation of Congo Red Dye.” Nanochemistry Research 4 (2): 140–147.
- Moradnia, Farzaneh, Saeid Taghavi Fardood, Ali Ramazani, Samira Osali, and Ilnaz Abdolmaleki. 2020. “Green Sol–Gel Synthesis of CoMnCrO4 Spinel Nanoparticles and Their Photocatalytic Application.” Micro & Nano Letters 15 (10): 674–677. https://doi.org/10.1049/mnl.2020.0189
- Mylarappa, M., N. Raghavendra, B. S. Surendra, K. N. Shravana Kumar, and S. Kantharjau. 2022. “Electrochemical, Photocatalytic and Sensor Studies of Clay/MgO Nanoparticles.” Applied Surface Science Advances 10: 100268. https://doi.org/10.1016/j.apsadv.2022.100268
- Nagabhushana, G., D. Samrat, and G. Chandrappa. 2014. “α-MoO3 Nanoparticles: Solution Combustion Synthesis, Photocatalytic and Electrochemical Properties.” RSC Advances 4 (100): 56784–56790. https://doi.org/10.1039/C4RA05135A
- Pompapathi, K., K. S. Anantharaju, B. S. Surendra, S. Meena, B. Uma, A. P. Chowdhury, and H. C. A. Murthy. 2023. “Synergistic Effect of a Bi2Zr2O7 and Hydroxyapatite Composite: Organic Pollutant Remediation, Antibacterial and Electrochemical Sensing Applications.” RSC Advances 13 (40): 28198–28210. https://doi.org/10.1039/d3ra05222b
- Prinith, N. S., and Manjunatha, J. G. 2020. “Polymethionine Modified Carbon Nanotube Sensor for Sensitive and Selective Determination of L-Tryptophan.” Journal of Electrochemical Science and Engineering 10 (4): 305–315. https://doi.org/10.5599/jese.774
- Raghavendra, N., H. P. Nagaswarupa, T. R. Shashi Shekhar, M. Mylarappa, B. S. Surendra, S. C. Prashantha, N. Basavaraju, C. R. Ravi Kumar, and M. R. Anil Kumar. 2021. “Electrochemical Sensor Studies and Optical Analysis of Developed Clay Based CoFe2O4 Ferrite NPs.” Sensors International 2: 100083. https://doi.org/10.1016/j.sintl.2021.100083
- Rao, S., R. Suhas, M. Mahadevaswamy, C. B. Pradeep Kumar, J. Gururaj Kudur, A. S. Sowmyashree, K. Shwetha, T. Aravinda, and S. Kumar. 2021. “Experimental and DFT Explorations of Tert-Butyl(1-(2-(4-Nitrobenzylidene)-Hydrazinyl)-1-Oxopropan-2yl)-Carbamate on CRCA Metal in 1M HCl Solution.” Results in Surfaces and Interfaces 5: 100023. https://doi.org/10.1016/j.rsurfi.2021.100023
- Sanchez, C., M. Nigen, V. Mejia Tamayo, T. Doco, P. Williams, C. Amine, and D. Renard. 2018. “Acacia Gum: History of the Future.” Food Hydrocolloids 78: 140–160. https://doi.org/10.1016/j.foodhyd.2017.04.008
- Shanbhag, V. V., S. C. Prashantha, C. R. Ravi Kumar, P. Kumar, B. S. Surendra, H. Nagabhushana, D. M. Jnaneshwara, et al. 2021. “Comparative Analysis of Electrochemical Performance and Photocatalysis of SiO2 Coated CaTiO3:RE3+ (Dy, Sm), Li+ Core Shell Nano Structures.” Inorganic Chemistry Communications 134: 108960. https://doi.org/10.1016/j.inoche.2021.108960
- Sneha, K., M. Sathishkumar, S. Y. Lee, M. A. Bae, and Y.-S. Yun. 2011. “Biosynthesis of Au Nanoparticles Using Cumin Seed Powder Extract.” Journal of Nanoscience and Nanotechnology 11 (2): 1811–1814. https://doi.org/10.1166/jnn.2011.3414
- Sowmyashree, A. S., A. Somya, C. B. P. Kumar, and S. Rao. 2021. “Novel Nano Corrosion Inhibitor, Integrated Zinc Titanate Nano Particles: Synthesis, Characterization, Thermodynamic and Electrochemical Studies.” Surfaces and Interfaces 22: 100812. https://doi.org/10.1016/j.surfin.2020.100812
- Surendra, B. S., H. P. Nagaswarupa, M. U. Hemashree, and J. Khanum. 2020. “Jatropha Extract Mediated Synthesis of ZnFe2O4 Nanopowder: Excellent Performance as an Electrochemical Sensor, UV Photocatalyst and an Antibacterial Activity.” Chemical Physics Letters 739: 136980. https://doi.org/10.1016/j.cplett.2019.136980
- Surendra, B. S., K. Gurushantha, K. S. Anantharaju, M. Rudresh, N. Basavaraju, N. Raghavendra, A. A. Jahagirdar, H. M. Somashekar, and H. C. A. Murthy. 2023. “Effective Paracetamol Sensor Activity, Thermal Barrier Coating (TBCs), and UV-Light Driven Photocatalytic Studies of ZrxO2:Mg2+(1−x) Nanoparticles.” New Journal of Chemistry 47 (8): 3978–3992. https://doi.org/10.1039/D2NJ06049C
- Surendra, B. S., M. M. Swamy, T. Shamala, S. Rao, A. S. Sowmy Shree, C. Mallikarjuna Swamy, and S. Pramila. 2022. “Development of Enhanced Electrochemical Sensor and Antimicrobial Studies of ZnO NPs Synthesized Using Green Plant Extract.” Sensors International 3: 100176. https://doi.org/10.1016/j.sintl.2022.100176
- Surendra, B. S., M. Veerabhdraswamy, K. S. Anantharaju, H. P. Nagaswarupa, and S. C. Prashantha. 2018. “Green and Chemical-Engineered CuFe2O4: Characterization, Cyclic Voltammetry, Photocatalytic and Photoluminescent Investigation for Multifunctional Applications.” Journal of Nanostructure in Chemistry 8 (1): 45–59. https://doi.org/10.1007/s40097-018-0253-x
- Surendra, B. S., T. Kiran, M. V. Chethana, H. S. Savitha, and M. S. Paramesh. 2021. “Cost-Effective Aegle marmelos Extract-Assisted Synthesis of ZnFe2O4:Cu2+ NPs: Photocatalytic and Electrochemical Sensor Applications.” Journal of Materials Science: Materials in Electronics 32 (20): 25234–25246. https://doi.org/10.1007/s10854-021-06981-5
- Swamy, M., B. S. Surendra, C. Mallikarjunaswamy, S. Pramila, and N. Rekha. 2021. “Bio-Mediated Synthesis of ZnO Nanoparticles Using Lantana Camara Flower Extract: Its Characterizations, Photocatalytic, Electrochemical and Anti-Inflammatory Applications.” Environmental Nanotechnology, Monitoring & Management 15: 100442. https://doi.org/10.1016/j.enmm.2021.100442
- Taghavi Fardood, S., A. Ramazani, P. A. Asiabi, and S. W. Joo. 2018. “A Novel Green Synthesis of Copper Oxide Nanoparticles Using a Henna Extract Powder.” Journal of Structural Chemistry 59 (7): 1737–1743. https://doi.org/10.1134/S0022476618070302
- Taghavi Fardood, S., F. Moradnia, S. Heidarzadeh, and A. Naghipour. 2023. “Green Synthesis, Characterization, Photocatalytic and Antibacterial Activities of Copper Oxide Nanoparticles.” Nanochemistry Research 8 (2): 134–140.
- Tajik, Somayeh, Hadi Beitollahi, Fariba Garkani Nejad, Mohadeseh Safaei, Kaiqiang Zhang, Quyet Van Le, Rajender S. Varma, Ho Won Jang, and Mohammadreza Shokouhimehr. 2020. “Developments and Applications of Nanomaterial-Based Carbon Paste Electrodes.” RSC Advances 10 (36): 21561–21581. https://doi.org/10.1039/d0ra03672b
- Tajik, Somayeh, Hadi Beitollahi, Ho Won Jang, and Mohammadreza Shokouhimehr. 2021. “A Screen Printed Electrode Modified with Fe3O4@polypyrrole-Pt Core-Shell Nanoparticles for Electrochemical Detection of 6-Mercaptopurine and 6-Thioguanine.” Talanta 232: 122379. https://doi.org/10.1016/j.talanta.2021.122379
- Uma, B., K. S. Anantharaju, S. Malini, S. S. More, Y. S. Vidya, S. Meena, and B. S. Surendra. 2022. “Synthesis of Novel Heterostructured Fe-Doped Cu2O/CuO/Cu Nanocomposite: Enhanced Sunlight Driven Photocatalytic Activity, Antibacterial and Supercapacitor Properties.” Ceramics International 48 (23): 35834–35847. https://doi.org/10.1016/j.ceramint.2022.08.032
- Umadevi, M., M. R. Bindhu, and V. Sathe. 2013. “A Novel Synthesis of Malic Acid Capped Silver Nanoparticles Using Solanum lycopersicums Fruit Extract.” Journal of Materials Science and Technology 29 (4): 317–322. https://doi.org/10.1016/j.jmst.2013.02.002
- Xia, T., Q. Li, X. Liu, J. Meng, and X. Cao. 2006. “Morphology-Controllable Synthesis and Characterization of Single-Crystal Molybdenum Trioxide.” The Journal of Physical Chemistry. B 110 (5): 2006–2012. https://doi.org/10.1021/jp055945n