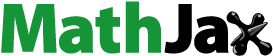
Abstract
This study presents a green and convenient approach for synthesising pure and Tb3+doped ZnO nanoparticles using naturally available Syzygium cumini fruit extract as fuel. The nanoparticles were thoroughly characterised for size, morphology, optical properties, photocatalytic, and cyclic voltammetric studies using various characterisation techniques. Powder X-ray diffraction (PXRD) analysis confirmed their high purity and crystallinity, with average sizes around 25 nm. Effective incorporation of Tb3+ ions into the ZnO lattice was verified. Scanning electron microscopy (SEM) images demonstrated the porous nature of the material. The prepared Tb3+-doped ZnO nanoparticles exhibited promising photocatalytic degradation performance of Titan yellow (TY) dye under UV light. Electrochemical studies using a modified electrode showed enhanced performance for sensing paracetamol. These results highlight the material’s potential for applications in sensors, catalysis, supercapacitors, batteries, and other fields.
1. Introduction
The escalating field of nanotechnology has sparked significant interest among researchers and industries, driving exploration into the unique characteristics of both emerging and traditional materials on the nanoscale. In particular, oxide semiconductors like ZnO, TiO2, SnO2, In2O3, and others have gathered considerable attention due to their promising potential for diverse future applications. In this context, we have chosen to employ zinc oxide nanoparticles for our study, as they exhibit semiconducting properties at the nanoscale (Happy, Kumar, and Rajeshkumar Citation2017).
Zinc oxide nanoparticles are one of the great potential semiconductors owing to their advanced properties, low cost, abundance, high surface activity, and environmental friendliness. ZnO is a wide-band gap semiconductor (3.37 eV). There are three unit structures, hexagonal wurtzite, zinc blende, and rock salt in ZnO, among the various structures, hexagonal wurtzite is the most recognised owing to its superior properties (Abderrahim et al. Citation2022). It has various applications such as gas sensors, biosensors, solar cells, organic LEDs, batteries, optoelectronic, spintronic, photocatalytic and antibacterial, nanomedicine, photochemical devices, technological and industrial domains, etc. However, undoped ZnO nanoparticles are affected by Photo-induced electron-hole pairs recombination and low light translation efficiency. The semiconducting properties of metal oxides can be altered by providing defects with the help of atomic/molecular manipulations through nanotechnology (Daksh and Agrawal Citation2016).
Moreover, the incorporation of dopants into ZnO nanomaterials has become a crucial focus of research. Currently, extensive investigations are underway employing rare earth ion dopants to enhance the photocatalytic degradation capabilities of ZnO. Notably, Tb3+ doping has been found to exert a substantial influence on crystal defects, surface morphology, as well as electrical and optical properties. These dopants effectively reduce the recombination rate of electron-hole pairs and introduce energy levels within the band gap, thereby extending the material’s light absorption capacity (Zhang et al. Citation2015).
Exploring the realm of energy storage and catalytic studies for future applications, one encounters two pivotal areas of interest: batteries and supercapacitors. Batteries are essential in our modern world, powering an array of devices from smartphones to electric vehicles. The research into batteries continues to evolve, with a strong emphasis on improving energy density, cycle life, and safety. Advancements in battery technology are pivotal for the widespread adoption of renewable energy sources, such as solar and wind power, as well as for the development of electric transportation (Manjunatha Citation2020: Vinod et al. Citation2017).
Catalytic studies play a pivotal role in addressing environmental and industrial challenges. Catalysts are substances that facilitate chemical reactions, enabling them to occur at lower temperatures and with higher efficiency. They are vital in fields ranging from energy production, and conversion to pollution control and pharmaceuticals. Ongoing research endeavours are aimed at discovering novel catalysts, understanding their mechanisms, and optimising their performance for various applications (Nalla Muthuchamy et al. Citation2018: Ashokkumar and Muthukumaran Citation2014).
Electrochemical sensors have garnered significant interest in the scientific community due to their rapid response, simple design, portability, high sensitivity, and selectivity. In complex sample matrices, various electrochemical sensors based on nanomaterials like graphene, conducting polymers (CPs), and metal nanoparticles have been developed in recent years (Amrutha et al. Citation2021; Manjunatha, Deraman, and Basri Citation2015; Chandrashekar et al. Citation2010). Paracetamol is a widely used analgesic and antipyretic drug, that contains an active phenolic hydroxyl group that can be electrochemically oxidised (Deepa et al. Citation2024). Creatinine is generally considered safe, but at high doses, it can cause serious side effects, such as kidney damage, and may inhibit the body’s natural creatinine production. Developing a commercially affordable sensor with superior sensitivity and selectivity would benefit both social and economic sectors. Consequently, there is considerable interest in developing various electrochemical techniques for detecting acetaminophen(paracetamol) (Chu et al. 2020; Charithra and Manjunatha Citation2019).
The synergy between advancements in batteries, sensors, supercapacitors, and catalytic studies offers tremendous potential for tackling global energy and environmental challenges. This synergy opens avenues for cleaner energy sources, improved energy storage efficiency, and sustainable chemical processes. These developments are crucial for realising a greener, more technologically advanced future. (Taghavi et al. Citation2023, Farzaneh et al. Citation2020, Taghavi et al. Citation2023). Numerous techniques, both chemical and physical, are employed in the production of nanoparticles (Chu et al. Citation2008). Many of these methods are associated with high energy consumption and present environmental challenges. In comparison to other methodologies, the combustion method has very good advantages over other preparative routes. This method involves the exothermic reaction between fuel and oxidiser sources, typically involving a metal or metal-containing compound as the precursor. The combustion process generates high temperatures and can lead to the rapid formation of desired products. rapid synthesis, homogeneity, relative simplicity, high purity, energy efficiency scalability, etc. are the advantages of the combustion method over other preparative routes (Bomila et al. Citation2017).
Here we presented a simple synthesis technique to fabricate pure and Tb3+ ions doped ZnO nanoparticles and the prepared materials were tested for photocatalytic degradation, battery, supercapacitor, and other applications.
2. Experimental
2.1. Materials
Terbium nitrate hexahydrate (Tb (NO3)3.6H2O, 99.99%) and zinc nitrate hexahydrate (Zn(NO3)2.6H2O, 99.99%) of analytical grade.
2.2. Extraction of fuel
Fresh Jamun fruit was collected from a botanical garden. These fruits were washed several times thoroughly using running tap water and then again washed with distilled water, dried in air. Washed Syzygium cumini portions were chopped into fine pieces, pulp of the fruit was separated from the seeds. 100 gm of chopped pulp was taken and crushed using a pestle and mortar, juice was extracted by filtering, and the same was used for the synthesis.
2.3. Synthesis of ZnO: Tb3+ (0-5mol %) nanoparticles
The stochiometric ratio of Tb(NO3)3.6H2O, Syzygium cumini fruit extract, and Zn(NO3)2.6H2O was put into a beaker containing 10 ml of distilled water kept at 450 °C in a muffle furnace for 30 min. the sample is calcined at the temperature 600 °C for 2 hr. The white solid was cooled down, pulverised, and then stored in a sample holder for further experiments and the corresponding procedure illustrated in . Undoped ZnO NPs were also prepared in the mentioned above procedure. Tb doped ZnO with different Mol % of Tb3+ concentrations ranging from 1 to 5 mol % were denoted by ZnO: 1 mol % Tb3+, ZnO: 3 mol % Tb3+, Zno:5 mol % Tb3+ respectively.
2.4. Photo catalytic experimental procedure
20 ppm of 250 ml aqueous solution of Titan Yellow(TY) and 60 mg of synthesised photocatalysts were taken in a circular glass reactor with an area of about 176.6 cm2. The aqueous (20 ppm of 250 ml) solution of the Titan Yellow dye with the synthesised photocatalyst (60 mg) was added into a circular glass reactor with an area of about 176.6 cm2and allowed to reach an adsorption–desorption equilibrium before UV light irradiation and the reaction mixture was stirred using a magnetic stirrer for the entire period of an experiment to ensure the establishment of adsorption/desorption.
2.5. Preparation of electrode
In typical Cyclic Voltammetry (CV), the carbon paste electrode was used as a working electrode. During the preparation of the working electrode, 75%, 15%, and 10% of prepared nanomaterial, graphite powder, and polytetrafluoroethylene respectively were mixed thoroughly for about 30 min after which the mixture was inserted into nickel mesh and crushed well at 20 MPa for 5 min to get an electrode. The current produced was measured using Ag/AgCl as the reference electrode and platinum wire as the counter electrode.
3. Results and discussions
To confirm the structure and crystal phase of pristine and doped ZnO nanomaterials, the samples were analysed by Powder X-ray diffractometer (PXRD). displays the PXRD patterns of pristine and doped ZnO. All the obtained peaks and their relative intensities of the prepared samples are well-matched with JCPDS No. 89-1397. The sharp peaks in PXRD indicate the high crystalline nature of prepared materials (Bomila et al. Citation2017). The average crystallite size of all the pristine and Tb3+ doped ZnO nanomaterials is estimated using the Scherrer equation and W-H methods () (Girish, Prashantha, et al. Citation2018, Kaini, Ali, and Saeid Citation2023).
(1)
(1)
Where, D = Crystallite size, = wavelength of X-rays K = Scherrer constant,
= Full width half maximum (Fardood et al. Citation2022). Also, the strain in the Tb3+ doped ZnO nanomaterials was evaluated using the W-H plots.
(2)
(2)
Dislocation density and strain associated with the material were estimated using the following relations and all the estimated values for the synthesised nanomaterials are given in the .
(3)
(3)
(4)
(4)
Table 1. Estimated crystallite parameters of prepared materials.
The morphology of the prepared nanomaterials was studied using Scanning Electron Microscopy(SEM) analysis, and the resulting SEM images are illustrated in . The observations from indicate the porous and agglomerated nature of the prepared material, also, revealed an interconnected network structure with a high rate of agglomeration and this kind of morphology is attributed to the rapid evolution of gases during the combustion process (Girish, Prashantha, and Nagabhushana Citation2017; Girish et al. Citation2016).
Diffused Reflectance Spectroscopic (DRS) studies were performed to analyse the optical properties of the synthesised Pristine and Tb3+ doped ZnO, the recorded diffused reflectance spectrum shown in (inset). The band gap energy of the pristine and Tb3+ doped ZnO was calculated from the DRS using the Kubelka-Munk Equationequation (5)(5)
(5) .
(5)
(5)
Here R∞ is the absolute reflectance and F(R∞) is the Kubelka-Munk function. The optical band gap values are estimated by plotting the variation of Kubelka-Munk function with photon energy through the following equation (Kubelka and Munk Citation1931).
(6)
(6)
Here ‘Eg’ is the optical band gap energy, hν: incident photon energy and n: the nature of the sample transition depends on the type of optical transition triggered by photon absorption. Obtained characteristic Kubelka-Munk plots of prepared nanomaterial shown in and the energy gap values for the prepared ZnO samples was found to be in range of 3.14 -3.20 eV, it was lesser than the reported value (∼3.3 eV). The reduction in the band gap energy might be owing to ZnO lattice crystal defects and also the presence of excess Zn in interstitial wurtzite lattice (Girish et al. Citation2016).
To investigate the effect of Tb3+ in ZnO structure, photocatalytic studies were performed on TY dye for a total duration of 60 mins with a time interval of 15mins and the corresponding absorption spectra of Tb3+ (3 mol %): ZnO catalyst presented in the and the efficiency of degradation was evaluated by equation.
(7)
(7)
The degradation of TY dye tracks the Langmuir-Hinshel wood first order kinetics model and can be expressed as ln (C/Co) = −k t, where k: constant of apparent reaction rate, C0: initial concentration of aqueous dye, t: reaction time and C: is the concentration of aqueous FOR dye at the reaction time of t (Yen et al. Citation2021).
The percentage of TY dye degradation with Tb3+ (1-5 mol %) activated ZnO catalyst illustrated in the and Tb3+ (3 mol %) activated ZnO catalyst reveals that highest % of TY dye was degraded and the detailed mechanism of photocatalytic degradation process () under UV light irradiation are presented below.
During UV irradiation, the electrons present in the valence band excited to conduction band and interacts with Tb3+ ions present in the ZnO, the Tb3+acts as an electron scavenger reduces the recombination of the charge carriers that increase the degradation activity by generating the O2-. and .OH. The Tb2+ ions produced from Tb3+ are less stable. The Tb2+ ions act on O2 and promotes the generation of O2-. The degradation mechanism for Tb3+ -doped ZnO is shown in the following equations (Girish et al. Citation2023).
The synthesised catalyst underwent a reusability test to assess its photostability, durability, and reusability. Even after five successive runs, there was only a slight decrease in degradation efficiency, with the decomposition efficiency remaining high at 73.30% by the end of the 5th cycle as shown in . The physical properties of the new and used photocatalyst were found to be nearly identical, indicating the catalyst’s stability over multiple uses. This established stability enhances its practical utility as a catalyst for dye photodecomposition. Therefore, the prepared material demonstrated both stability and reusability, making it a promising candidate for catalytic applications.
Electrochemical studies were performed to examine electrochemical properties of Tb3+ doped ZnO for battery and supercapacitor applications, the obtained CV curve of Tb3+ (3 mol%): ZnO electrodes with various scan rates are shown in . It was noticed the pseudo-capacitive nature of Tb3+: ZnO electrode (Sulciute et al. Citation2021).
To understand the charge transfer resistance, electrochemical impedance studies (EIS) were performed, and the obtained spectra of Tb3+ (0-5 mol%): ZnO electrode are seen in . Z′ is the real component, discloses the ohmic properties, and Zʺ is the virtual component reveals the capacitive properties. EIS spectra show an elevated arc (semicircle), generally, arc with larger radii indicates the higher charge transfer resistance (Raghupathy, Ramgopal, and Ravikumar Citation2022; Kumar, Ranjith, and Kumar Citation2018; Karthik et al. Citation2018). the results indicates semicircle at the higher frequency region and a straight line at the lower frequency region, representing that the electrodes has a superior capacitive behaviour, pristine, 1 mol % and 5 mol % doped ZnO electrodes show semicircle with larger radii compared to Tb3+ (3 mol %) represents the higher charge transfer resistance and 3 mol % of Tb3+ doped ZnO electrode shows semicircle with smaller radii denotes the smaller charge transfer resistance and thus Tb3+ (3 mol %) doped ZnO electrode possess superior capacitive properties than the pristine and other doped electrodes (Girish, Prashantha, et al. Citation2018; Kumar et al. Citation2021; Kusuma et al. Citation2023).
Furthermore, to evaluate the suitability of the suggested method as an electrochemical sensor, the electrodes that were prepared were utilised to detect the paracetamol content in the available Dolo-650 tablets. presents the CV plots of the pure and Tb3+ doped ZnO electrodes. The noticeable change in the CV profile after the introduction of paracetamol clearly indicates that, this observed and modified CV profile is indeed a result of the presence of paracetamol. This demonstration confirms that the proposed method (Kumar et al. Citation2023), can effectively detect the presence of paracetamol in pharmaceutical medications. All the obtained results highlights that the prepared material is potential for multifunctional applications, including wastewater purification, decoloration, supercapacitors, batteries, and sensors (Lavanya, Ramakrishnappa, Girish, Suresh Kumar, Basavarajur, et al. Citation2024).
The enhanced performance of photocatalytic and sensing properties of prepared materials is attributed to the introduction of Tb3+ ions, which create defects that serve as photo-electronic traps, enhancing the separation of charge carriers and reducing recombination and also modify the redox chemistry of the materials and altering the charge transfer kinetics at the electrode-electrolyte interface. However, it is crucial to note that the optimal doping concentration of Tb3+ ions is critical, as excessive doping can lead to the formation of recombination sites, reducing catalytic efficiency (Lavanya, Ramakrishnappa, Girish, Suresh Kumar, Basavarajur, et al. Citation2024). As can be seen from the Nyquist plot, the diameter of the semicircle of the Tb3+ (3 mol %) doped ZnO electrode is comparatively smaller than that of the other samples, thereby evidencing the increase in the separation of photogenerated electrons and holes. Remarkably, this shows that Tb3+ (3 mol %): ZnO has very low charge transfer resistance as it promotes migration of electrons and interfacial charge separation together with an efficient reduction of exciting quenching and energy dissipation. Also from the crystallite size for the Tb3+ (3 mol %): ZnO is comparatively less. As a result of this process, the Tb3+ (3 mol %): ZnO material sample provides good degradation efficiency and sensor properties compared to other prepared materials. As a result, prepared material is a prominent candidate for applications requiring enhanced photocatalysis and electrochemical sensing capabilities and other applications.
4. Conclusion
We successfully synthesised pure and Tb3+activated ZnO nanomaterials with various dopant concentrations using Syzygium cumini fruit extract as fuel via combustion technique. PXRD analysis confirmed the effective integration of Tb3+ ions into the ZnO crystal lattice. Scanning electron microscopy images revealed the porous nature of the material. The prepared catalysts demonstrated excellent catalytic activity for the degradation of TY dye under UV light, attributed to their small particle size and effective separation of charge carriers. Among the prepared catalysts, the 3 mol% Tb3+ doped ZnO catalyst exhibited the highest catalytic activity during the degradation process. The optimised Tb3+ concentration on the ZnO electrode significantly influenced its electrochemical activities and showed better sensitivity for the detection of paracetamol. These results highlight the potential of the synthesised material for multifunctional applications, including wastewater purification, decoloration, supercapacitors, batteries, and sensors.
Disclosure statement
No potential conflict of interest was reported by the author(s).
Data availability statement
The data that support the findings of this study are available on request from the corresponding author.
References
- Abderrahim, M., K. Navpreet, P. Nicola, Z. Dario, and C. Elisabetta. 2022. “One Dimensional ZnO Nanostructures: Growth and Chemical Sensing Performances.” Nanomaterials 10: 1940.
- Amrutha, B. M., G. Jamballi, Gowda Manjunatha, S. B. Aarti, and N. Hareesha. 2021. “Sensitive and Selective Electrochemical Detection of Vanillin at Graphene-Based Poly (Methyl Orange) Modified Electrode.” Journal of Science: Advanced Materials and Devices 6 (3): 415–424. https://doi.org/10.1016/j.jsamd.2021.05.002
- Ashokkumar, M., and S. Muthukumaran. 2014. “Microstructure, Optical and FTIR Studies of Ni, Cu co-Doped ZnO Nanoparticles by co-Precipitation Method.” Optical Materials 37: 671–678. https://doi.org/10.1016/j.optmat.2014.08.012
- Bomila, R., S. Srinivasan, A. Venkatesan, B. Bharath, and K. Perinbam. 2017. “Structural, Optical and Antibacterial Activity Studies of Ce-Doped ZnO Nanoparticles Prepared by Wet-Chemical Method.” Materials Research Innovations 1: 1–8. https://doi.org/10.1080/14328917.2017.1324379
- Chandrashekar, B. N., B. E. Kumara Swamy, M. Pandurangachar, S. Sharath Shankar, Ongera Gilbert, J. G. Manjunatha, and B. S. Sherigara. 2010. “Electrochemical Oxidation of Dopamine at Polyethylene Glycol Modified Carbon Paste Electrode: A Cyclic Voltammetric Study.” International Journal of Electrochemical Science 5 (4): 578–592. https://doi.org/10.1016/S1452-3981(23)15307-7
- Charithra, M. M., and J. G. Manjunatha. 2019. “Enhanced Voltammetric Detection of Paracetamol by Using Carbon Nanotube Modified Electrode as an Electrochemical Sensor.” Journal of Electrochemical Science and Engineering 10 (1): 29–40. https://doi.org/10.5599/jese.717
- Chu, Q., L. Jiang, X. Tian, and J. Ye. 2008. “Rapid Determination of Acetaminophen and Paminophenolin Pharmaceutical Formulationsusing Miniaturized Capillary Electrophoresis with Amperometric Detection.” Analytica Chimica Acta 606 (2): 246–251. https://doi.org/10.1016/j.aca.2007.11.015
- Daksh, Daksh, and Yadvendra Kumar Agrawal. 2016. “Rare Earth-Doped Zinc Oxide Nanostructures: A Review.” Reviews in Nanoscience and Nanotechnology 5 (1): 1–27. https://doi.org/10.1166/rnn.2016.1071
- Deepa, H. M., D. M. Tejashwini, H. V. Harini, H. P. Nagaswarupa, N. Ramachandra, and N. Basavaraju. 2024. “Multifunctional Applications of Hybrid CaMgTi2O4 Nanoparticles for Electrochemical Sensors and Photocatalysis.” Sensing Technology 2 (1): 2296890. https://doi.org/10.1080/28361466.2023.2296890
- Fardood, S. T., F. Moradnia, R. Forootan, R. Abbassi, R. Jalalifar, A. Ramazani, and, M. Sillanpӓӓ. 2022. “Facile Green Synthesis, Characterization and Visible Light Photocatalytic Activity of MgFe2O4@CoCr2O4 Magnetic Nanocomposite.” Journal of Photochemistry and Photobiology A: Chemistry 423: 113621. https://doi.org/10.1016/j.jphotochem.2021.113621
- Farzaneh, M., T. F. Saeid, R. Ali, O. Samira, and A. Ilnaz. 2020. “Green Sol–Gel Synthesis of CoMnCrO4 Spinel Nanoparticles and Their Photocatalytic Application.” Micro Nano Letters 15: 674–677. https://doi.org/10.1049/mnl.2020.0189
- Girish, K. M., S. G. Burragoni, N. Ramachandra, S. C. Prashantha, H. Nagabhushana, R. Lavanya, G. V. Ashok Reddy, and Jae Hong Kim. 2023. “Photoluminescence and Photocatalytic Properties of Zn2TiO4: Cr3+ Nanophosphors.” Inorganic Chemistry Communications 151: 110628. https://doi.org/10.1016/j.inoche.2023.110628
- Girish, K. M., S. C. Prashantha, and H. Nagabhushana. 2017. “Facile Combustion Based Engineering of Novel White Light Emitting Zn2TiO4:Dy3+ Nanophosphors for Display and Forensic Applications.” Journal of Science: Advanced Materials and Devices 2 (3): 360–370. https://doi.org/10.1016/j.jsamd.2017.05.011
- Girish, K. M., S. C. Prashantha, H. Nagabhushana, C. R. Ravikumar, H. P. Nagaswarupa, Ramachandra Naik, H. B. Premakumar, and B. Umesh. 2018. “Multi-Functional Zn2TiO4: Sm3+ Nanopowders: excellent Performance as an Electrochemical Sensor and an UV Photocatalyst.” Journal of Science: Advanced Materials and Devices 3 (2): 151–160. https://doi.org/10.1016/j.jsamd.2018.02.001
- Girish, K. M., S. C. Prashantha, Ramachandra Naik, H. Nagabhushana, H. P. Nagaswarupa, H. B. Premakumar, S. C. Sharma, and K. S. Anantha Raju. 2016. “Visible Photon Excited Photoluminescence; Photometric Characteristics of a Green Light Emitting Zn2TiO4: Tb3+ Nano Phosphor for wLEDs.” Materials Research Express 3 (7): 075015–075030. https://doi.org/10.1088/2053-1591/3/7/075015
- Girish, K. M., N. Ramachandra, S. C. Prashantha, H. Nagabhushana, H. P. Nagaswarupa, K. S. Anantha Raju, H. B. Premkumar, S. C. Sharma, and B. M. Nagabhushana. 2018. “Zn2TiO4: Eu3+ Nanophosphor: self Explosive Route and Its near UV Excited Photoluminescence Properties for WLEDs.” Spectrochimica Acta. Part A, Molecular and Biomolecular Spectroscopy 138: 857–865. https://doi.org/10.1016/j.saa.2014.10.097
- Happy, A., S V.. Kumar, and S. Rajeshkumar. 2017. “A Review on Green Synthesis of Zinc Oxide Nanoparticles –an Eco-Friendly Approach.” Resource-Efficient Technologies 3: 406–413. https://doi.org/10.1166/rnn.2016.1071
- Kaini, M. T., Ali, R., and Saeid T. F. 2023. “Green Synthesis and Characterization of Ni0.25Zn0.75Fe2O4 Magnetic Nanoparticles and Study of Their Photocatalytic Activity in the Degradation of Aniline.” Applied. Organometallic. Chemistry 37: e7053. https://doi.org/10.1002/aoc.7053
- Karthik, K., S. Dhanuskodi, C. Gobinath, S. Prabukumar, and S. Sivaramakrishnan. 2018. “Multifunctional Properties of Microwave Assisted CdO–NiO–ZnO Mixed Metal Oxide Nanocomposite: enhanced Photocatalytic and Antibacterial Activities.” Journal of Materials Science: Materials in Electronics 29 (7): 5459–5471. https://doi.org/10.1007/s10854-017-8513-y
- Kubelka, P., and F. Munk. 1931. “Reflection Characteristics of Paints.” Zeitschrift Fur Technische Physik 12: 593–601.
- Kumar, A. Naveen, Ramachandra Naik, V. Revathi, H. C, Ananda Murthy, H. P. Nagaswarupa, C. R. Ravikumar, G. V. Ashok Reddy, et al. 2023. “Multifunctional La10Si6O27:Tb3+ Tailored Material for Photoluminescence, Photocatalysis, and Electrochemical Sensing Applications.” Applied Surface Science Advances 14: 100392. https://doi.org/10.1016/j.apsadv.2023.100392
- Kumar, Deepak, C. Hepsibah Priyadarshini, V. Sudha, Jositta Sherine, S. Harinipriya, and Samanwita Pal. 2021. “Investigation of Adsorption Behavior of Anticancer Drug on Zinc Oxide Nanoparticles: A Solid-State NMR and Cyclic Voltammetry (CV) Analysis.” Journal of Pharmaceutical Sciences 110 (11): 3726–3734. https://doi.org/10.1016/j.xphs.2021.08.003
- Kumar, D. R., K. S. Ranjith, and R. T. R. Kumar. 2018. “Structural, Optical, Photocurrent and Solar Driven Photocatalytic Properties of Vertically Aligned Samarium Doped ZnO Nanorod Arrays.” Optik 154: 115–125. https://doi.org/10.1016/j.ijleo.2017.10.004
- Kusuma, K. B., M. Manju, C. R. Ravikumar, N. Raghavendra, T. Naveen Kumar, M. R. Anilkumar, H. P. Nagaswarupa, T. R. Shashi Shekhar, H. C. Ananda Murthy, and K. U. Aravind. 2023. “Synthesis of Strontium Oxide Nanoparticles by Probe Sonication Method: Its Photocatalytic Activity and Electrochemical Sensor Studies.” Sensors International 4: 100231. https://doi.org/10.3390/jcs8020047
- Lavanya, R., T. Ramakrishnappa, K. M. Girish, K. Suresh Kumar, N. Basavarajur, and B. M. Shilpa. 2024. “Blackberry Gel-Assisted Combustion Modified MgO: Sm3+ Nanoparticles for Photocatalytic, Battery, Sensor and Antibacterial Applications.” Results in Chemistry 7: 101417. https://doi.org/10.1016/j.rechem.2024.101417
- Lavanya, R., T. Ramakrishnappa, K. M. Girish, K. Suresh Kumar, M. Radhakrishna Reddy, and H. R. Prakash. 2024. “Synthesis and Characterization of Hydrothermally Derived Cerium-Doped TiO2@MoS2 Nanocomposites for Waste-Water Treatment and Super Capacitor Applications.” Bulletin of Materials Science 47 (1): 34. https://doi.org/10.1007/s12034-023-03112-0
- Manjunatha, J. G. 2020. “A Surfactant Enhanced Graphene Paste Electrode as an Effective Electrochemical Sensor for the Sensitive and Simultaneous Determination of Catechol and Resorcinol.” Chemical Data Collections 25: 100331. https://doi.org/10.1016/j.cdc.2019.100331
- Manjunatha, J. G., M. Deraman, and N. H. Basri. 2015. “Electrocatalytic Detection of Dopamine and Uric Acid at Poly (Basic Blue b) Modified Carbon Nanotube Paste Electrode.” Asian Journal of Pharmaceutical and Clinical Research 8 (5): 48–53.
- Muthuchamy, N., A. Raji, N. Thomas, Immanuel E. Jebakumar, P. Suguna, and R. L. Yong. 2018. “High-Performance Glucose Biosensor Based on Green Synthesized Zinc Oxide Nanoparticle Embedded Nitrogen-Doped Carbon Sheet.” Journal of Electroanalytical Chemistry 816: 195–204. https://doi.org/10.1016/j.jelechem.2018.03.059
- Raghupathy, D. A., G. Ramgopal, and C. R. Ravikumar. 2022. “Photocatalytic Degradation of Direct Green & Fast Orange Red Dyes: Electrochemical Sensor of Lead Using Cupric Oxide Nanoparticles Synthesized via Sonochemical Route.” Sensors International 3: 100204. https://doi.org/10.1016/j.sintl.2022.100204
- Sulciute, A., K. Nishimura, E. Gilshtein, F. Cesano, G. Viscardi, A. G. Nasibulin, Y. Ohno, and S. Rackauskas. 2021. “ZnO Nanostructures Application in Electrochemistry: Influence of Morphology.” The Journal of Physical Chemistry C 125 (2): 1472–1482. https://doi.org/10.1021/acs.jpcc.0c08459
- Taghavi, F. S., F. Moradnia, S. Heidarzadeh, and A. Naghipour. 2023. “Green Synthesis, Characterization, Photocatalytic and Antibacterial Activities of Copper Oxide Nanoparticles of Copper Oxide Nanoparticles.” Nano Chemistry Research 8 (2): 134–140. https://doi.org/10.22036/ncr.2023.02.006
- Vinod, K., O. M. Ntwaeaborwa, T. Soga, Viresh Dutta, and H. C. Swart. 2017. “Rare Earth Doped Zinc Oxide Nano Phosphor Powder: A Future Material for Solid-State Lighting and Solar Cells.” ACS Photonics. 4 (11): 2613–2637. https://doi.org/10.1021/acsphotonics.7b00777
- Yen, X., H. Nguyen, H. L. Thi Viet, and D. D. Van. 2021. “Green Synthesis of Ce-Doped ZnO Nanoparticles Using Hedyotis Capitellata Leaf Extract for Efficient Photocatalytic Degradation of Methyl Orange.” Vietnam Journal of Chemistry 59 (5): 648–659.
- Zhang, X., D. Shanshan, Z. Xian, Y. Lei, C. Guojun, D. Shuangshi, et.al. 2015. “A Facile One-Photosynthesis of Er–Al co-Doped ZnO Nanoparticles with Enhanced Photocatalytic Performance under Visible Light.” Materials Letters 143: 312–314.