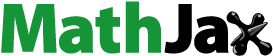
Abstract
The solution combustion process and environmentally acceptable green fuels, including plant root extracts from Flacortia indica and Aloe vera, were used to make a nanostructured nickel ferrite (NiFe2O4). We used X-ray diffraction (XRD) to find the crystallinity of the produced ferrite nanoparticles, Field Emission scanning electron microscopy (FE-SEM) to examine the surfaces, and energy dispersive X-ray diffraction (EDAX) to find the elements. The NiFe2O4 sample was subjected to electrochemical testing, which included electrostatic charge and discharge (GCD) experiments with electrodes submerged in a 0.1 M HCl electrolyte and measurements of AC impedance. The sample was used to build chemical sensors for arsenic trioxide and mercuric acetate. Thanks to their capacity to be magnetically retrieved, aloe vera-mediated combustion of NiFe2O4 NPs degraded 98.4 percent of the AR-88 dye after 90 min, whereas combustion of plant root extract under UV light degraded 84.4 percent. The increased emphasis on research that this comparison study sparked led to the production of high-quality, repeatable materials made of bio-composite reagents that are non-toxic and environmentally friendly.
1. Introduction
Emerging nanomaterials and related phenomena may be the focus of future nano-science research. In recent years, magnetic nano-materials have attracted a lot of attention due to the intriguing uses they have (Taghavi Fardood et al. Citation2023, Citation2018a, Citation2022; Moradnia et al. Citation2020; Taghavi Fardood et al. Citation2018b). The magnetic characteristics of spinel ferrites, which are described by the formula MFe2O4 (where M is an element such as Zn, Ni, Mn, Co, etc.), make them extremely adaptable in a wide range of applications (Taghavi Fardood et al. Citation2023, Citation2018a, Citation2022; Moradnia et al. Citation2020; Taghavi Fardood et al. Citation2018b). Spinel ferrites are essential in many applications, including data storage, magneto-optical recording, antennas, humidity sensors, energy storage devices, inductors, microwave devices, and magnetic sensing (Dhanda et al. Citation2023; Raghavendra et al. Citation2021; Manjunatha et al. Citation2023). Their extraordinary chemical, optical, electrical, and magnetic characteristics render them indispensable in these domains. Additionally, ferrofluids for targeted therapies, medical diagnostics, cancer therapy, hyperthermia treatment, drug delivery systems, MRI (Magnetic Resonance Imaging), and other medical applications have come to rely on magnetic nanoparticles, particularly ferrite nanoparticles (Raghavendra et al. Citation2021; Mylarappa et al. Citation2022).
The precise targeting and colloidal stability of nanostructures are essential for these applications, and nanoparticles’ sensitivity, influenced by their surface area-to-volume ratio, plays a crucial role (Basavaraju et al. Citation2021). Beyond medical and technological applications, ferrite nanoparticles have also demonstrated effectiveness in environmental applications, particularly in wastewater treatment and dye degradation (Surendra et al. Citation2023; Vasudha et al. Citation2021; Raghavendra et al. Citation2024; Al Asian, Olya, and Mahmoodi Citation2015; Mahmoodi et al. Citation2019). NiFe2O4 nanoparticles (NPs) are important because of their many uses. Due to their excellent permeability at high frequencies, electrical resistivity, and chemical and mechanical stability, they are widely used in electronics (Ishino and Narumiya Citation1987; Manjunatha Citation2022; Charithra and Manjunatha Citation2021; Manjunatha et al. Citation2020; Hareesha and Manjunatha Citation2020). These ferrites also exhibit interesting electrochemical properties and photocatalytic degradation that make them suitable for various applications due to their unique combination of properties, including high conductivity, catalytic activity, and stability.
These ferrites can be synthesised using several techniques like chemical methods, including combustion, coprecipitation, sol-gel, mechanical alloying and precipitation for the fabrication of stoichiometric and chemically pure spinel ferrite nanoparticles (Manjunatha Citation2022; Charithra and Manjunatha Citation2021; Manjunatha et al. Citation2020; Hareesha and Manjunatha Citation2020; Manjunatha Citation2020; Verma, Goel, and Mendiratta Citation2000; Chen and He Citation2001). However, the combustion process is commonly utilised to generate nanoparticles with specified properties for diverse purposes. Moreover, the process is relatively simple, scalable, and cost-effective, making it suitable for large-scale production. In summary, the combustion method for synthesising ferrite nanoparticles can align with green synthesis principles by minimising energy consumption, eliminating or reducing the use of harmful solvents, generating minimal waste, using sustainable starting materials, maintaining low toxicity, and offering scalability. Overall, the combustion technique is a versatile method for synthesising nanocrystalline nickel ferrite nanoparticles with specific characteristics tailored for various applications, including catalysis, magnetic storage, biomedical imaging, and environmental remediation.
Ferrites have also been studied as electrocatalysts for various reactions due to their high surface area, good conductivity, and catalytic activity (Chen and Liu Citation2012; Karakas et al. Citation2015; Maaz et al. Citation2009; Sue et al. Citation2011). They have shown potential in oxygen reduction reactions (ORR), hydrogen evolution reaction (HER), oxygen evolution reaction (OER), and other important electrochemical processes (Son et al. Citation2002; Wang et al. Citation2009; Qu et al. Citation2007; Can, Fırat, and Özcan Citation2011). These can be used as electrode materials in supercapacitors due to their high specific surface area, high sensitivity, high capacitance, and excellent electrochemical performance making them promising candidates for energy storage applications, electrochemical sensors for detecting various analytes such as heavy metals, gases, and biomolecules. Continued research in this area is essential for further understanding and optimising their performance for practical use in various electrochemical devices and systems. According to the literature, developing the solution combustion process to produce ferrites from a variety of eco-friendly fuels would be highly beneficial. In this work, naturally occurring, eco-friendly, and easily available green fuels such as aloe vera and plant root extract are used to synthesise and characterise NiFe2O4 nanoparticles via green approach. Different spectral characterisation methods, including XRD, EDAX, SEM, CV, sensor and photocatalytic studies, were used to confirm the synthesised NiFe2O4.
2. Experimental
2.1. Materials and method
In this work, all the chemicals were purchased from Merck as indicated in the following .
Table 1. List of chemicals, specifications and suppliers used.
2.2. Experimental procedure
2.2.1. Extraction of Aloe vera gel
The extract appears in the gel that is found in the canter of aloe vera leaves. The outer green coat of aloe vera was peeled (sliced) off in order to obtain the gel. The inner part of the gel, which was firmly crushed and ground to a thin, homogenous consistency, was used to create the jelly used in the synthesis. Aloe vera gel was employed as a sustainable substitute to fuel the NPs’ synthesis.
2.2.2. Synthesis NiFe2O4 by simple solution combustion method
NiFe2O4 NPs can be produced by dissolving 1.45 g of nickel nitrate and 2.415 g of ferric nitrate in distilled water in a crucible. 1.8 ml of aloe vera gel or 1.08 g of plant root extract are the next ingredients to be added. The crucible was put in a heated muffle furnace to maintain its temperature of 750 °C. When the combustion process is complete, the final product can be ground into a fine powder using a clean mortar and pestle. Utilising the traditional calculation approach, the stoichiometric compositions of aloe vera, nickel nitrate, and plant root extracts should be ascertained. A schematic representation of the aloe vera solution combustion method-based nickel ferrite synthesis process is presented in .
2.2.3. Characterisation
A nanocomposite of NiFe2O4-aloe vera and NiFe2O4-root extract was studied using an XRD Maxima-7000 instrument from Shimadzu to determine its structural characteristics. A JEOL JSM-5600LV and a SU-1500, HITACHI were used for morphology and EDX analyses of the samples. A UV-Vis absorption spectrum was recorded using an SL 159 ELICO UV-Visible spectrophotometer. Under UV light irradiation, the produced NPs were photocatalytically decoloured with Acid Red-88 (AR-88) dye. A CHI608E potentiostat tri-electrode configuration was utilised to conduct electrochemical activity. The setup included a modified electrode, platinum wire, and Ag/AgCl as the working, counter, reference electrodes, respectively and the electrolyte employed was a 0.1 M HCl solution.
3. Results and discussions
3.1. X-ray diffraction studies
The XRD (X-Ray Diffraction) pattern of synthesised NiFe2O4 NPs by solution combustion method as shown in . The peaks at {220},{311},{222},{400},{511} and {440} related to the 2θ values of 30.4°, 36.7°, 37.8°, 43.5°, 57.5° and 63.4° correspondingly. The sample can be indexed as inverse spinel NiFe2O4 with face centred cubic (fcc) structure corresponded to the standard reference (JCPDS Card No.10–0325) (Yousefi, Amiri, and Salavati-Niasari Citation2019). A secondary phase, haematite (𝛼-Fe2O3) was observed at 2θ values 33.4°, 40.9°, 49.6° (JCPDS Card No: 79–0007) (Karcıoğlu Karakaş Citation2022). Maintaining the ideal temperature and proper stochiometric ratios of both oxidants and fuels should reduce the secondary phase. reveals that the average crystalline size of the produced NiFe2O4 shows a range from 21.7 to 23.6 nm for NiFe2O4-aloe vera and NiFe2O4-root extract, respectively, in accordance to the Scherrer formula.
(1)
(1)
Table 2. Crystalline size and structural constraints of NiFe2O4 nanoparticles.
where k – Scherrer constant, λ – wave length, β – FWHM (Fullwidth at half-maximum) and θ – Bragg’s angle (Taghavi Fardood et al. Citation2019; Moradnia et al. Citation2020).
3.2. Field emission scanning electron microscopy (FE-SEM) studies
For examining the morphological characteristics of synthetic materials, FE-SEM is one of the best analytical techniques. By using FE-SEM testing, we can easily get images at both high and low magnification. High- and low-magnification images of NiFe2O4 nanoparticles are shown in , correspondingly. Nanoparticle accumulation could be drastically reduced by raising the ultrasonic intensity (M. S. Silva et al. Citation2023; Modak et al. Citation2009; Gharagozlou Citation2009; Gedanken Citation2004). shows that the NiFe2O4 nanoparticles have a closed-like shape, and shows that the particles are distributed evenly.
3.3. Energy dispersive X-ray analysis studies
The results of the analysis of the elemental compositions of the NiFe2O4-aloe vera and NiFe2O4-root extracts are shown in , respectively. Elements with mass percentages of 47.17%, 29.48%, and 23.35% for aloe vera and 45.17%, 33.51%, and 21.32% for plant root extract were present in the synthesised sample, respectively. However, quantitative chemical analysis confirmed the sample’s validity, and EDAX did not detect any impurities.
3.4. Electrochemical studies
3.4.1. CV Analysis
It was determined that the CV research of the electrochemical properties of the NiFe2O4-aloe vera and NiFe2O4-root extract nanoparticles was carried out, and the results are depicted in and , respectively. The potential window range was between −0.3 and +0.4 V and between −0.4 and +0.6 V ( and ) by using 0.1 M HCl as electrolyte. Electrode fabrication required a 30-min gentle grinding in an agate mortar containing NiFe2O4 NPs, graphite powder, and silicon oil in a weight ratio of 15:70:15 (Kumar et al. Citation2019). When compared to NiFe2O4-root extract, NiFe2O4-aloe vera had a greater degree of reversibility and possessed a better proton diffusion coefficient in the CV test. As can be seen from the enhanced D value in , the electrochemical activity of NiFe2O4-aloe vera is significantly more effective than that of NiFe2O4-root extract. In the CV of NiFe2O4 nanoparticles, the oxidation and reduction peaks are brought about by the transformation of Ni to Ni2+ and Fe2+ to Fe3+, respectively. There was an increase in the scan rate from 0.01 to 0.05 mV/s in each of the samples. With each scan rate, the area of the curves in the CV profiles increased. This is because the two sample electrodes undergo a rapid redox kinetic process (Ravikumar et al. Citation2018).
Figure 7. Proton deficient coefficient and linear fitting of (a) NiFe2O4-aloe vera and (b) NiFe2O4-root extract electrode.
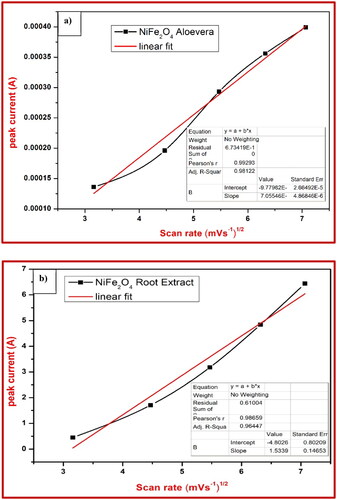
Table 3. Reversibility and proton deficient coefficient of NiFe2O4-aloe vera nanoparticle.
Table 4. Reversibility and proton deficient coefficient of NiFe2O4 root extract nanoparticle.
3.5. Electrochemical impedance spectral analysis (EIS)
With a three-electrode setup in 0.1 M HCl and a frequency range of 1 MHz to 100 MHz, we compared the EIS of the NiFe2O4 electrode to that of Ag/AgCl. shows the AC impedance of the NiFe2O4-aloe vera electrode and shows the AC impedance of the NiFe2O4-root extract electrode. The capacitive properties are related to the imaginary component Zimg (Z''), while the ohmic properties are revealed by the real component Zreal (Z'). In comparison to more streamlined shapes, the resistance to charge transfer is usually higher in rigid semicircles. According to the EIS data, the electrical interface conductivity is improved by using the NiFe2O4-aloe vera electrode in 0.1 M HCl as opposed to the control electrodes, which have a lower charge-transfer resistance (Rct). Because of this, they are an excellent material to use when building electrochemical sensors.
3.6. Electrochemical sensor studies
By employing the NiFe2O4 electrode’s CV measurement, arsenic trioxide was successfully detected. As seen in , the NiFe2O4-generated carbon paste electrode can detect arsenic trioxide concentrations ranging from 1–3 mM in an acidic medium (0.1 M HCl). shows that there is a noticeable shift in the oxidation peak, which falls within the range of 0.32 to 0.51 V, confirming this. shows that the peak of the rapid oxidation potential increased from 0.38 to 0.15 V when mercuric acetate was utilised as a chemical sensor, and shows that the peak of the reduction potential increased from 0.08 to 0.10 V. That the chemical sensor’s sensing capabilities were enhanced is evident from this.
Figure 10. CV response for the NiFe2O4 root extract electrode at 0.1 M HCl of sensor arsenic trioxide.
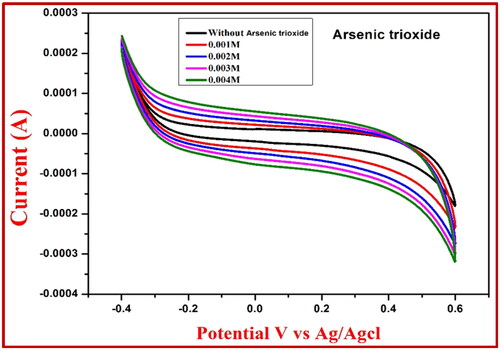
Table 5. Redox reaction values of NiFe2O4 extract using arsenic trioxide sensor.
Table 6. Redox reaction values of NiFe2O4 extract using mercuric acetate sensor.
3.7. Band gap analysis
The optical properties of the synthesised NiFe2O4 from aloe vera and Root extract methods investigated using UV–Visible Diffuse Reflectance spectrophotometric technique as shown in . the energy band gap data of synthesised NiFe2O4 nanoparticles were calculated by using the Kubelka-Munk equation: F(R) = (1−R)2/2R, with R representing % of reflected light (Moradnia et al. Citation2021; Mohammad Taghi Kiani and Saeid Citation2023; Meenakshi et al. Citation2023). The optical band gap (Eg) is determined from the observed UV-Visible spectral dependence of the absorption near the absorption edge.
Figure 12. Diffuse reflectance spectra of the synthesised NiFe2O4 from aloe vera and root extract methods.
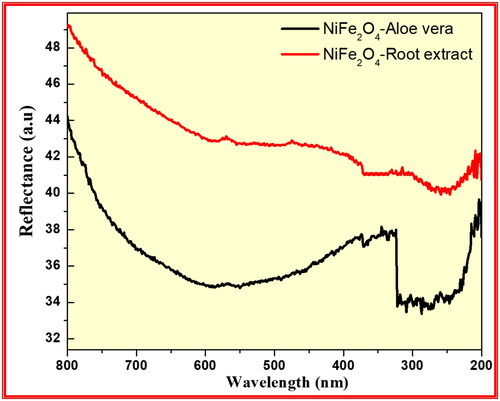
Based on Urbach rule the absorption coefficient (α) is calculated from the optical absorption spectrum. It is known that the absorption coefficient for non-crystalline materials varies with frequency and the relationship between the absorption coefficient (α) and the optical band gap Eg obeys the classical Tauc’s expression. Hence, the observed absorption spectrum is translated in to Tauc’s plot using the equation (Dinamani et al. Citation2023). For all samples a plot of the product of absorption coefficient (α) and photon energy (αhν)1/2 versus the photon energy hν at room temperature shows a linear behaviour, which can be considered as evidence for the indirect allowed transition. Extrapolation of the linear portion of this curve to a point (αhν) 1/2 = 0 gives the optical band gap Eg. shows the energy band gap of the synthesised NiFe2O4 from aloe vera and Root extract methods were found to be 3.53 and 4.56 eV respectively. This result confirms that synthesised NiFe2O4 from aloe vera method has low energy band gap than Root extract method showed excellent photocatalytic applications.
3.8. Photocatalytic activities
Photocatalysis is a light-initiated phenomenon that takes place in the presence of a catalyst. The NiFe2O4 NPs samples act as photocatalysts and release superoxide radicals (⋅O2−) when they are exposed to light. This happens because the electrons move from the valence band to the conduction band. At the same time, water molecules on the surface react with holes in the valence band to create hydroxyl radicals (OH). These reactive radicals can dissolve organic contaminants at the photocatalyst surface by adsorbing them (Anandan and Rajendran Citation2015). The research looked at what UV light does to AR-88 dye when it is mixed with NiFe2O4 nanoparticles from plant roots and the aloe vera-mediated combustion process (). When 30 ppm of AR-88 dye was mixed with 30 mg of NiFe2O4 nanoparticles made using the aloe vera-mediated combustion technique and plant roots, the results are shown in . The absorbance of the dye was recorded under UV-visible spectroscopy. As shown in , NiFe2O4 nanoparticles made from the aloe vera method were better at breaking down AR-88 dye under UV light, with a rate of 98.4% after 90 min compared to 84% for the plant root-mediated combustion method. Further, it was observed that the photo-degradation performance of AR-88 dye was 12.6% under dark conditions and 15.5% under photolysis ().
Figure 14. Absorbance of AR-88 (30 ppm) in presence of NiFe2O4 NPs by (a) aloe vera method; and (b) plant root mediated combustion method under UV-light. (c) Plot of % degradation vs time indicating the AR-88 dye photo-degradation capability (d) plot of % degradation vs no. of cycles of AR-88 dye.
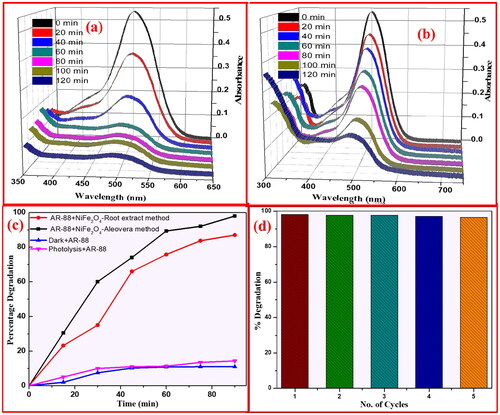
In addition, as demonstrated in , the recycle performance ability of NiFe2O4 NPs on AR-88 dye decoloration was assessed after each cycle under the same conditions for a total of 5 cycles. shows the NiFe2O4 NPs created using the plant root method and shows the NiFe2O4 NPs prepared using the aloe vera-mediated combustion method, both of which were subjected to UV radiation for the half-time for AR-88 dye decoloration. Results from the sample preparation process show that the degradation time of NiFe2O4 NPs mediated by aloe vera is 48.7 min, much shorter than the degradation period of NiFe2O4 NPs helped by plant roots 59.8 min. A linear relationship between log C/Co and k for NiFe2O4 NPs generated by plant root and aloe vera-mediated combustion techniques under UV light is shown in . For NiFe2O4 NPs produced utilising plant root and aloe vera-mediated combustion techniques, the measured values of slope k were 0.04756 and 0.0988 min−1, respectively, according to the equation.
(2)
(2)
where t is the testing duration, Co and C are the dye concentrations, and k is the first order rate constant.
Figure 15. (a) Half time analysis of AR-88 (30 ppm) in presence of NiFe2O4 NPs prepared by a aloe vera method; (b) plant root mediated combustion method under UV-light and (c) the kinetics studies of NiFe2O4 NPs.
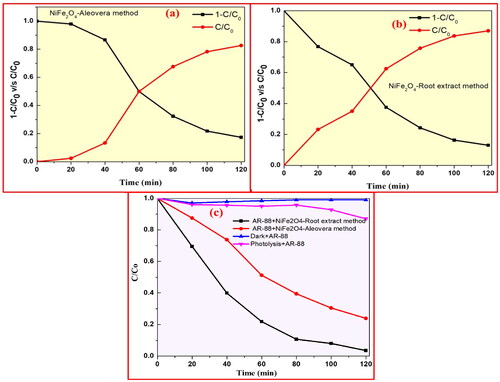
Dye colours are produced by chromophore groups and azo linkages (–N = N–), which have a maximum absorption at 502 nm due to the photocatalytic breakdown of the n → π* transition in ultraviolet light. The AR-88 solution with catalyst has its UV absorption spectra recorded from 0 to 90 min at room temperature. At room temperature, the beginning of the degradation was indicated by a rise in time and a fall in intensity. During the breakdown of AR-88 dye, the green NiFe2O4 NPs demonstrated effective catalytic activity. Using UV-Vis spectrophotometry, the process was monitored at room temperature. In addition to the catalysts, which are green NiFe2O4 NPs, there may be other factors that contribute to the rapid degradation of the AR-88 dye, as illustrated in . These include an increase in electron production by the reductant, aloe vera, and an efficient transfer of charges towards the dye.
4. Conclusion
In this article, the eco-friendly fuels aloe vera and root extract were combined with NiFe2O4 NP using the solution combustion method. It was determined that inverted and FCC NiFe2O4 NPs had formed by XRD examination. The NiFe2O4-root extract had an average particle size of 23.6 nm, whereas the NiFe2O4-aloe vera extract had an average particle size of 21.7 nm. The elemental analysis confirmed that the produced samples actually include Cu, Ni, Fe, and O. When compared to NiFe2O4-root extract, electrochemical studies on NiFe2O4-aloe vera showed a considerable improvement in proton diffusion rate, reaction reversibility, and electricity transfer. In addition, studies on chemical sensors evaluated how well NiFe2O4 measured CV for heavy metal detection, specifically mercuric acetate and arsenic trioxide. In particular, the NiFe2O4 carbon paste electrodes’ high peak mobility of oxidation and reduction states makes them very good sensors. Since NiFe2O4-aloe vera degrades more quickly than NiFe2O4-root extract, it may find usage in a variety of applications. These findings suggest that the synthesised NiFe2O4 with aloe vera could be useful in a variety of environmental remediation applications, including electrochemical detection of heavy metals and various biosensors for treating industrial effluent water.
Disclosure statement
No potential conflict of interest was reported by the author(s).
Additional information
Funding
References
- Basavaraju, N., S. C. Prashantha, B. S. Surendra, T. R. Shashi Shekhar, M. R. Anil Kumar, C. R. Ravikumar, N. Raghavendra, and T. S. Shashidhara. 2021. “Structural and Optical Properties of MgNb2O6 NPs: Its Potential Application in Photocatalytic and Pharmaceutical Industries as Sensor.” Environmental Nanotechnology, Monitoring & Management 16: 100581. https://doi.org/10.1016/j.enmm.2021.100581
- Al Asian, A., M. E. Olya, and N. M. Mahmoodi. 2015. “Preparation and Adsorption Behavior of Diethylenetriamine/Polyacrylonitrile Composite Nanofibers for a Direct Dye Removal.” Fibers and Polymers 16 (9): 1925–1934. (https://doi.org/10.1007/s12221-015-4624-3
- Anandan, K., and V. Rajendran. 2015. “Effects of Mn on the Magnetic and Optical Properties and Photocatalytic Activities of NiO Nanoparticles Synthesized via the Simple Precipitation Process.” Materials Science and Engineering: B 199: 48–56. https://doi.org/10.1016/j.mseb.2015.04.015
- Can, Musa Mutlu, Tezer Fırat, and Şadan Özcan. 2011. “Interparticle Interaction Effects on Magnetic Behaviors of Hematite (α-Fe2O3) Nanoparticles.” Physica B: Condensed Matter 406 (13): 2483–2487. https://doi.org/10.1016/j.physb.2011.01.002
- Charithra, M. M., and J. G. Manjunatha. 2021. “Electrochemical Sensing of Adrenaline Using Surface Modified Carbon Nanotube Paste Electrode.” Materials Chemistry and Physics 262 (1): 124293. https://doi.org/10.1016/j.matchemphys.2021.124293
- Chen, D. H., and X. R. He. 2001. “Synthesis of Nickel Ferrite Nanoparticles by Sol-Gel Method.” Materials Research Bulletin 36 (7–8): 1369–1377. https://doi.org/10.1016/S0025-5408(01)00620-1
- Chen, D., and H. Liu. 2012. “One-Step Synthesis of Nickel Ferrite Nanoparticles by Ultrasonic Wave-Assisted Ball Milling Technology.” Materials Letters 72: 95–97. https://doi.org/10.1016/j.matlet.2011.12.101
- Dhanda, N., P. Thakur, A.-C A. Sun, and A. Thakur. 2023. “Structural, Optical and Magnetic Properties along with Antifungal Activity of Ag-Doped Ni-Co Nano Ferrites Synthesized by Eco-Friendly Route.” Journal of Magnetism and Magnetic Materials 572: 170598. https://doi.org/10.1016/j.jmmm.2023.170598
- Dinamani, M., B. S. Surendra, H. C. Ananda Murthy, N. Basavaraju, and Vijaya V. Shanbhag. 2023. “Green Engineered Synthesis of PbxZn1-xO NPs: An Efficient Electrochemical Sensor and UV Light-Driven Photocatalytic Applications.” Environmental Nanotechnology, Monitoring and Management 20: 100822. https://doi.org/10.1016/j.enmm.2023.100822
- Gedanken, A. 2004. “Using Sonochemistry for the Fabrication of Nanomaterials.” Ultrasonics Sonochemistry 11 (2): 47–55. https://doi.org/10.1016/j.ultsonch.2004.01.037
- Gharagozlou, M. 2009. “Synthesis, Characterization and Influence of Calcination Temperature on Magnetic Properties of Nanocrystalline Spinel Co-Ferrite Prepared by Polymeric Precursor Method.” Journal of Alloys and Compounds 486 (1–2): 660–665. https://doi.org/10.1016/j.jallcom.2009.07.025
- Hareesha, N., and J. G. Manjunatha. 2020. “Fast and Enhanced Electrochemical Sensing of Dopamine at Cost-Effective Poly(DL-Phenylalanine) Based Graphite Electrode.” Journal of Electroanalytical Chemistry 878: 114533. https://doi.org/10.1016/j.jelechem.2020.114533
- Ishino, K., and Y. Narumiya. 1987. “Development of Magnetic Ferrites: control and Application of Losses.” Ceramic Bull 66: 1469–1475.
- Karakas, Z. K., R. B. Kcuogu, I. H. Karakas, and M. Ertugru. 2015. “The Effects of Heat Treatment on the Synthesis of Nickel Ferrite (NiFe2O4) Nanoparticles Using the Microwave Assisted Combustion Method.” Journal of Magnetism and Magnetic Materials 374: 298–306.
- Karcıoğlu Karakaş, Zeynep. 2022. “A Comprehensive Study on the Production and Photocatalytic Activity of Copper Ferrite Nanoparticles Synthesized by Microwave-Assisted Combustion Method as an Effective Photocatalyst.” Journal of Physics and Chemistry of Solids 170: 110927. https://doi.org/10.1016/j.jpcs.2022.110927
- Kumar, M. R. Anil, H. P. Nagaswarupa, C. R. Ravikumar, S. C. Prashantha, H. Nagabhushana, and Aarti S. Bhatt. 2019. “Green Engineered Nano MgO and ZnO Doped with Sm3+: Synthesis and a Comparison Study on Their Characterization, PC Activity and Electrochemical Properties.” Journal of Physics and Chemistry of Solids 127: 127–139. https://doi.org/10.1016/j.jpcs.2018.12.012
- M. S. Silva, Maitê, Rafael A. Raimundo, Thayse R. Silva, Allan J. M. Araújo, Daniel A. Macedo, Marco A. Morales, Carlson P. Souza, Andarair G. Santos, and André L. Lopes-Moriyama. 2023. “Morphology-Controlled NiFe2O4 Nanostructures: Influence of Calcination Temperature on Structural, Magnetic and Catalytic Properties towards OER.” Journal of Electroanalytical Chemistry 933: 117277. https://doi.org/10.1016/j.jelechem.2023.117277
- Maaz, K., S. Karim, A. Mumtaz, S. K. Hasanain, J. Liu, and J. L. Duan. 2009. “Synthesis and Magnetic Characterization of Nickel Ferrite Nanoparticles Prepared by co-Precipitation.” Journal of Magnetism and Magnetic Materials 321 (12): 1838–1842. https://doi.org/10.1016/j.jmmm.2008.11.098
- Mahmoodi, N. M., A. Taghizadeh, M. Taghizadeh, and M. A. S. Baglou. 2019. “Surface Modified Montmorillonite with Cationic Surfactants: Preparation, Characterization, and Dye Adsorption from Aqueous Solution.” Chemical Engineering. 7 (4): 103243. https://doi.org/10.1016/j.jece.2019.103243
- Manjunatha, J. G. 2020. “A Surfactant Enhanced Graphene Paste Electrode as an Effective Electrochemical Sensor for the Sensitive and Simultaneous Determination of Catechol and Resorcinol.” Chemical Data Collections 25: 100331. https://doi.org/10.1016/j.cdc.2019.100331
- Manjunatha, J. G. 2022. Electrochemical Sensors Based on Carbon Composite Materials, Fabrication, Properties and Applications. Bristol, UK: IOP Publishing,
- Manjunatha, J. G., C. Raril, N. Hareesha, M. M. Charithra, P. A. Pushpanjali, Girish Tigari, D. K. Ravishankar, S. C. Mallappaji, and Jayarame Gowda. 2020. “Electrochemical Fabrication of Poly (Niacin) Modified Graphite Paste Electrode and Its Application for the Detection of Riboflavin.” Open Chemical Engineering Journal 14 (1): 90–98. https://doi.org/10.2174/1874123102014010090
- Manjunatha, J. G., N. Prinith Subbaiah, N. Hareesha, C. Raril, Ammar M. Tighezza, and Munirah D. Albaqami. 2023. “Fabrication of Polymer-Modified Carbon Sensor and Its Application in the Electrochemical Determination of Indigo Carmine.” Monatshefte Für Chemie – Chemical Monthly 154 (11): 1235–1242. https://doi.org/10.1007/s00706-023-03129-5
- Meenakshi, Giridhar, B. C. Manjunath, S. C. Prashantha, T. Prashanth, and B. S. Surendra. 2023. “Super Capacitor, Electrochemical Measurement and Sun Light Driven Photocatalytic Applications of CuFe2O4 NPs Synthesized from Bio-Resource Extract.” Sensors International 4: 100237. https://doi.org/10.1016/j.sintl.2023.100237
- Modak, S., M. Ammar, F. Mazaleyrat, S. Das, and P. K. Chakrabarti. 2009. “XRD, HRTEM and Magnetic Properties of Mixed Spinel Nanocrystalline Ni–Zn–Cu-Ferrite.” Journal of Alloys and Compounds 473 (1–2): 15–19. https://doi.org/10.1016/j.jallcom.2008.06.020
- Mohammad Taghi Kiani, Ali Ramazani, and Taghavi Fardood Saeid. 2023. “Green Synthesis and Characterization of Ni0.25Zn0.75Fe2O4 Magnetic Nanoparticles and Study of Their Photocatalytic Activity in the Degradation of Aniline.” Applied Organometallic Chemistry 37: e7053.
- Moradnia, Farzaneh, Saeid Taghavi Fardood, Ali Ramazani, and Vinod Kumar Gupta. 2020. “Green Synthesis of Recyclable MgFeCrO4 Spinel Nanoparticles for Rapid Photodegradation of Direct Black 122 Dye.” Journal of Photochemistry and Photobiology A: Chemistry 392: 112433. https://doi.org/10.1016/j.jphotochem.2020.112433
- Moradnia, Farzaneh, Saeid Taghavi Fardood, Ali Ramazani, Bong-ki Min, Sang Woo Joo, and Rajender S. Varma. 2021. “Magnetic Mg0.5Zn0.5FeMnO4 Nanoparticles: Green Sol-Gel Synthesis, Characterization, and Photocatalytic Applications.” Journal of Cleaner Production 288: 125632. https://doi.org/10.1016/j.jclepro.2020.125632
- Moradnia, Farzaneh, Saeid Taghavi Fardood, Ali Ramazani, Samira Osali, and Ilnaz Abdolmaleki. 2020. “Green Sol-Gel Synthesis of CoMnCrO4 Spinel Nanoparticles and Their Photocatalytic Application.” Micro & Nano Letters 15 (10): 674–677. https://doi.org/10.1049/mnl.2020.0189
- Mylarappa, M., N. Raghavendra, B. S. Surendra, K. N. Shravana Kumar, and S. Kantharjau. 2022. “Electrochemical, Photocatalytic and Sensor Studies of Clay/MgO Nanoparticles.” Applied Surface Science Advances 10: 100268. https://doi.org/10.1016/j.apsadv.2022.100268
- Qu, S., J. Wang, J. L. Kong, P. Y. Yang, and G. Chen. 2007. “Magnetic Loading of Carbon Nanotube/nano-Fe3O4 Composite for Electrochemical Sensing.” Talanta 71 (3): 1096–1102. https://doi.org/10.1016/j.talanta.2006.06.003
- Raghavendra, N., H. P. Nagaswarupa, T. R. Shashi Shekhar, M. Mylarappa, B. S. Surendra, S. C. Prashantha, N. Basavaraju, C. R. Ravi Kumar, and M. R. Anil Kumar. 2021. “Electrochemical Sensor Studies and Optical Analysis of Developed Clay Based CoFe2O4 Ferrite NPs.” Sensors International 2: 100083. https://doi.org/10.1016/j.sintl.2021.100083
- Raghavendra, N., H. P. Nagaswarupa, T. R. Shashi Shekhar, M. Mylarappa, B. S. Surendra, S. C. Prashantha, C. R. Ravikumar, M. R. Anil Kumar, and N. Basavaraju. 2021. “Development of Clay Ferrite Nanocomposite: Electrochemical, Sensors and Photocatalytic Studies.” Applied Surface Science Advances 5: 100103. https://doi.org/10.1016/j.apsadv.2021.100103
- Raghavendra, N., H. P. Nagaswarupa, T. R. Shashi Shekhar, M. Mylarappa, N. Basavaraju, and B. S. Surendra. 2024. “Evaluation of Nb2O5/rGO Nanocomposites for Their Electrochemical Sensor & Photocatalytic Applications.” Sensing Technology 2 (1): 2299092.
- Ravikumar, C. R., M. R Anil Kumar, H. P. Nagaswarupa, S. C. Prashantha, Aarti S. Bhatt, M. S. Santosh, and Denis Kuznetsov. 2018. “CuO Embedded β-Ni(OH)2 Nanocomposite as Advanced Electrode Materials for Supercapacitors.” Journal of Alloys and Compounds 736: 332–339. https://doi.org/10.1016/j.jallcom.2017.11.111
- Son, S., M. Taheri, E. Carpenter, V. G. Harris, and M. E. McHenry. 2002. “Synthesis of Ferrite and Nickel Ferrite Nanoparticles Using Radio-Frequency Thermal Plasma Torch.” Journal of Applied Physics 91 (10): 7589–7591. https://doi.org/10.1063/1.1452705
- Sue, K., M. Aoki, T. Sato, D. N. Hamane, S. Kawasaki, Y. Hakuta, Y. Takebayashi, et al. 2011. “Continuous Hydrothermal Synthesis of Nickel Ferrite Nanoparticles Using a Central Collision-Type Micromixer: effects of Temperature, Residence Time, Metal Salt Molality, and Naoh Addition on Conversion, Particle Size, and Crystal Phase.” Industrial & Engineering Chemistry Research 50 (16): 9625–9631. https://doi.org/10.1021/ie200036m
- Surendra, B. S., K. Gurushantha, K. S. Anantharaju, M. Rudresh, N. Basavaraju, N. Raghavendra, A. A. Jahagirdar, H. M. Somashekar, and H. C. Ananda Murthy. 2023. “Effective Paracetamol Sensor Activity, Thermal Barrier Coating (TBC), and UV-Light-Driven Photocatalytic Studies of ZrxO2: Mg2þ(1 − x) Nanoparticles.” New Journal of Chemistry 47 (8): 3978–3992. https://doi.org/10.1039/D2NJ06049C
- Taghavi Fardood, S., A. Ramazani, P. A. Asiabi, and S. W. Joo. 2018a. “A Novel Green Synthesis of Copper Oxide Nanoparticles Using a Henna Extract Powder.” Journal of Structural Chemistry 59 (7): 1737–1743. https://doi.org/10.1134/S0022476618070302
- Taghavi Fardood, S., A. Ramazani, Z. Golfar, and S. W. Joo. 2018b. “Green Synthesis Using Tragacanth Gum and Characterization of Ni–Cu–Zn Ferrite Nanoparticles as a Magnetically Separable Catalyst for the Synthesis of Hexabenzylhexaazaisowurtzitane under Ultrasonic Irradiation.” Journal of Structural Chemistry 59 (7): 1730–1736. https://doi.org/10.1134/S0022476618070296
- Taghavi Fardood, Saeid, Farzaneh Moradnia, Reza Forootan, Rouzbeh Abbassi, Salman Jalalifar, Ali Ramazani, and Mika Sillanpӓӓ. 2022. “Facile Green Synthesis, Characterization and Visible Light Photocatalytic Activity of MgFe2O4@CoCr2O4 Magnetic Nanocomposite.” Journal of Photochemistry and Photobiology A: Chemistry 423: 113621. https://doi.org/10.1016/j.jphotochem.2021.113621
- Taghavi Fardood, Saeid, Farzaneh Moradnia, Sajjad Moradi, and Reza Forootan. 2019. “Fateme Yekke Zare, Maryam Heidari, Eco-Friendly Synthesis and Characterization of α-Fe2O3 Nanoparticles and Study of Their Photocatalytic Activity for Degradation of Congo Red Dye.” Nanochemistry Research 4 (2): 140–147.
- Taghavi Fardood, Saeid, Farzaneh Moradnia, Siamak Heidarzadeh, and Ali Naghipour. 2023. “Green Synthesis, Characterization, Photocatalytic and Antibacterial Activities of Copper Oxide Nanoparticles.” Nanochemistry Research 8 (2): 134–140.
- Vasudha, M., Akif Ahamed Khan, K. M. Bhumika, Devaraja Gayathri, H. P. Nagaswarupa, T. R. Shashi Shekhar, N. Raghavendra, et al. 2021. “Facile Chemical Synthesis of Ca3MgAl10O17 Nanomaterials for Photocatalytic and Non-Enzymatic Sensor Applications.” Sensors International 2: 100082. https://doi.org/10.1016/j.sintl.2021.100082
- Verma, A., T. C. Goel, and R. G. Mendiratta. 2000. “Low Temperature Processing of NiZn Ferrite by Citrate Precursor Method and Study of Properties.” Materials Science Technology 16 (6): 712–715. https://doi.org/10.1179/026708300101508324
- Wang, J., F. Ren, R. Yi, A. Yan, G. Z. Qiu, and X. Liu. 2009. “Solvothermal Synthesis and Magnetic Properties of Size-Controlled Nickel Ferrite Nanoparticles.” Journal of Alloys and Compounds 479 (1–2): 791–796. https://doi.org/10.1016/j.jallcom.2009.01.059
- Yousefi, Seyede Rahele, Omid Amiri, and Masoud Salavati-Niasari. 2019. “Control Sonochemical Parameter to Prepare Pure ZnO.35Fe2.65O4 Nanostructures and Study Their Photocatalytic Activity.” Ultrasonics Sonochemistry 58: 104619. https://doi.org/10.1016/j.ultsonch.2019.104619