Abstract
Replication conditional oncolytic human adenovirus has long been considered a promising biological therapeutic to target high-grade gliomas (HGG), a group of essentially lethal primary brain cancer. The last decade has witnessed initiation and some completion of a number of Phase I and II clinical investigations of oncolytic adenovirus for HGG in the US and Europe. Results of these trials in patients are pivotal for not only federal approval but also filling an existing knowledge gap that primarily derives from the stark differences in permissivity to human adenovirus between humans and preclinical mouse models. DNX-2401 (Delta-24-RGD), the current mainstream oncolytic adenovirus with modifications in E1A and the fiber, has been shown to induce impressive objective response and long-term survival (>3 years) in a fraction of patients with recurrent HGG. Responders exhibited initial enlargement of the treated lesions for a few months post treatment, followed by shrinkage and near complete resolution. In accord with preclinical research, post-treatment specimens revealed virus-mediated alteration of the immune tumor microenvironment as evidenced by infiltration of CD8+ T cells and M1-polarized macrophages. These findings are encouraging and together with further information from ongoing studies have a potential to make oncolytic adenovirus a viable option for clinical management of HGG. This review deals with this timely topic; we will describe both preclinical and clinical development of oncolytic adenovirus therapy for HGG, summarize updated knowledge on clinical trials and discuss challenges that the field currently faces.
Introduction
Glioma is a group of neoplasms that primarily arise within the central nervous system (CNS). The current 2016 World Health Organization (WHO) classification uses both histology and genetic molecular features to define gliomas into subtypes and malignancy grade: grades I–IV.Citation1 Grades III and IV are considered high-grade (i.e., malignant) gliomas (HGG). Glioblastoma (GBM), grade IV, is the most common glioma typically affecting adults, and includes GBM, IDH-wildtype and GBM, IDH-mutant that each show distinct clinical characteristics. GBM, in general, is characterized by aggressive and infiltrative growth and resistance to therapy. The prognosis of IDH-wildtype GBM is very poor with the median survival of 15 months despite the current standard treatment consisting of a combination of surgical resection, radiation and chemotherapy.Citation2 HGG also affect children, and include diffuse mid-line glioma, histone3 K27M mutant and HGG in the cerebral hemispheres. Recent large-scale genetic studies have vastly advanced our understanding of molecular background of both adult and pediatric HGG, and led to clinical testing of numerous molecular-targeted strategies. However, no molecular targeting agents have been shown to extend the overall survival of patients with HGG. The development of novel therapies for HGG is highly needed.
The concept of applying virus to the treatment of cancer is old and was clinically tested in the 1950–1970s.Citation3,Citation4 It was in the early 1990s when technology to engineer viral genome first enabled the generation of oncolytic virus (OV) as a cancer-selective therapeutic.Citation5 A variety of genetically modified viruses and some naturally occurring viruses have been developed as OV that possesses the ability to replicate in cancer cells and kill them but spare normal cells. The basic OV effect is a direct killing of host cancer cells following its selective replication in tumor cells. Upon cancer cell killing, many OVs induce inflammatory and anti-tumor immune responses that have been increasingly shown to play a major role in overall anti-cancer effects mediated by OV. In addition, genetic modification of OV allows loading of a new function such as therapeutic gene expression or selective infectability to increase efficacy and safety.Citation6 A number of virus species, both DNA and RNA viruses, have been studied as OV platforms for cancer therapy, such as herpes simplex virus (HSV), adenovirus, vaccinia virus, reovirus, poliovirus and measles virus. In 2015, FDA approved talimogene laherparepvec (T-VEC), a genetically modified HSV, for advanced melanoma as the first OV therapeutic in the US.Citation7
Extensive preclinical and clinical research conducted in the past three decades has accumulated a body of evidence that supports that HGG, in particular GBM, is an appropriate target of OV therapy. Along with oncolytic HSV, oncolytic adenovirus is one of the most studied and promising OVs in the treatment of HGG, with multiple oncolytic adenovirus clinical trials currently ongoing in patients with HGG.Citation8 In this review article, we will summarize preclinical and clinical development of oncolytic adenovirus therapies for HGG and discuss challenges and future directions to advance the field.
History Of The Development Of Conditionally Replicative Adenoviruses (CRAd)
Adenoviruses are non-enveloped viruses with an icosahedral capsid that contains a double-stranded DNA genome of about 36 kb in size. They have a broad range of vertebrate hosts, from fish to humans, showing species-specificity. In human adenovirus, 57 serotypes have been identified.Citation9 Depending on the serotype, human adenoviruses cause various disease conditions such as respiratory disease, conjunctivitis, gastroenteritis and myocarditis. The well-understood adenovirus biology allows opportunities for genetic manipulation and tailored gene modifications to generate oncolytic adenoviruses, i.e. conditionally replicative adenovirus (CRAd). With regard to manufacturing, CRAd can be produced at high titer and particles are of excellent physicochemical stability.Citation10,Citation11
1st Generation Of CRAd: E1B-55kD Deleted Onyx-015
dl1520 (Onyx-015) is the first genome-modified CRAd that is based on human adenovirus type 2/5 chimera.Citation12 Onyx-015 has a large deletion at the E1B locus, and does not express E1B-55kD. When wild-type adenovirus infects cells and forces entry into the S phase triggering p53-mediated apoptosis, the E1B-55kD protein binds and inactivates p53, abrogating p53-mediated apoptosis and enabling the virus to replicate. Onyx-015 is unable to replicate in normal cells due to the deletion of this E1B-55kD, and was initially shown to replicate only in tumor cells lacking p53. However, subsequent studies revealed that Onyx-015 was not selective to p53-defective cells as it replicated in cancer cells having wild-type p53.Citation13–Citation15 On the other hand, tumor cells exist that do not support replication of Onyx-015. Multi-functional E1B-55kD mediates export of late adenoviral mRNA and tumor cells resistant to Onyx-015 do not provide the RNA export functions of E1B-55kD.Citation16 Heat shock at 39.4°C rescued the mRNA export function of E1B-55kD and enabled efficient replication of E1B-55kD-deletion mutants.Citation17
Onyx-015 clinical trials have been conducted in several types of cancer, and a Phase I trial for malignant glioma was reported in 2004.Citation18 In this trial, Onyx-015 was injected into the brain that surrounded a surgically resected glioma. None of a total of 24 patients experienced serious adverse events related to Onyx-015. Although lymphocytic and plasma cell infiltrates were found within tumors histologically, no definite anti-tumor efficacy was demonstrated in this trial. After an unsuccessful Phase III trial of combination therapy of chemotherapy and Onyx-015 in head and neck cancer patients (NCT00006106),Citation19,Citation20 clinical development of Onyx-015 discontinued in the United States. In China, combination therapy of intratumoral injection of H101, a CRAd very similar to Onyx-015, and chemotherapy demonstrated promising efficacy for head and neck cancer.Citation21 In 2005, H101 (Oncorine) was approved as the world’s first OV in China.Citation22 Currently, H101 trials for hepatocellular carcinoma are underway in China (NCT03790059, NCT03780049).
2nd Generation CRAd: Delta-24-RGD (DNX-2401)
The presence of multiple adenoviral genes that target cellular cell cycle regulators provided the opportunity to develop oncolytic adenoviruses targeting new pathways. Since many cancers including gliomas have deficiencies in the Rb (retinoblastoma) tumor suppressor pathway, virus therapy targeting the Rb pathway was developed as the second generation of CRAd.Citation23 E1A-delta24 (Delta-24) was based on human adenovirus type 5 and had a 24-base pair deletion in the Rb-binding domain of the E1A gene. The major function of E1A protein is to bind to Rb protein and release transcription factor E2F from the Rb-E2F complex, promoting E2F-driven transcription of genes associated with the S phase (e.g. DNA metabolism) and thereby viral replication.Citation24 Delta-24 cannot replicate in cells with intact Rb due to the inability of the mutant E1A to bind to Rb protein. On the other hand, Delta-24 can replicate in tumor cells in which Rb is deleted, since E2F that exists in a free state promotes gene transcription and transition to the S phase. At about the same time, dl922-947, another oncolytic adenovirus containing a 24-base pair deletion within the Rb-binding region of E1A, was developed and showed better in vivo efficacy than dl1520 (Onyx-015).Citation25
Human adenovirus type 5 binds to Coxsackievirus and adenovirus receptor (CAR) on the cell surface and enters the host cells. Although CAR is expressed in a variety of normal cells,Citation26 several cancers including glioma express lower levels of CAR,Citation27,Citation28 causing poor infectivity of CRAd in cancer cell lines. In order to overcome this problem, Suzuki et al developed the approach that incorporates a sequence encoding an RGD (Arg-Gly-Asp) peptide in a cyclic conformation (RGD-4C) into the HI loop of the fiber knob.Citation29 The RGD sequence interacts with αv integrins on the cell surfaceCitation30 that many cancer cells express, including HGG known to highly express integrin αvβ3.Citation31 The fiber modification with RGD improved the infection rate of CRAd in cancer cell lines and enabled CAR independent infection. The resulting CRAd, Delta-24-RGD (DNX-2401) having a 24-bp deletion in the E1A gene and an RGD motif in fiber protein, became the prototype of a newer generation of oncolytic adenoviruses. Since the late 2000s, a number of clinical trials started testing DNX-2401 for HGG (), and the first report of a Phase I trial was published in 2018,Citation32 as detailed below.
Table 1 Current Clinical Trials Studying Oncolytic Adenovirus In High-Grade Glioma
3rd Generation CRAds
Continued efforts have been made to create CRAd that are more efficacious and cancer-selective. Many novel CRAds have been developed based on DNX-2401 and tested for their effects on HGGs in translational research. To express mutant E1A in a cancer-selective manner, Alonso et al generated the oncolytic adenovirus ICOVIR5 which contains an insulated E2F1-responsive promoter and a Kozak sequence preceding the E1A start codon.Citation33 DeltaE1A expression driven by a promoter consisting of four palindromic E2F-binding sites and one Sp-1 binding site in ICOVIR15 increased cytotoxicity and antitumor activity.Citation34 ICOVIR17 is a modified version of ICOVIR15 and is armed with cDNA for human hyaluronidase PH20.Citation35 Hyaluronidase-mediated degradation of hyaluronic acid in the tumor extracellular matrix enabled ICOVIR17 to spread better within orthotopic HGG xenografts and extended animal survival.Citation35,Citation36 Furthermore, VCN-01, in which RGD was inserted in the fiber shaft instead of fiber knob to improve the infectivity of ICOVIR17, similarly produced a significant survival prolonging effect in an HGG xenograft model.Citation37,Citation38 Although these viruses have not entered clinical trials for HGG, Phase I trials of VCN-01 are active for retinoblastoma (EudraCT 2016-001060-11) and pancreatic adenocarcinoma (NCT02045589 and NCT02045602) in Spain.
Delta-24-RGDOX was generated by arming DNX-2401 with mouse OX40L (OX40 ligand) cDNA to activate T cells through the activating receptor OX40 on the surface of T cells.Citation39 Intratumoral injection of Delta-24-RGDOX recruited more CD4+ and CD8+ T cells in tumor sites than Delta-24-RGD, and significantly prolonged survival time in an immuno-competent mouse GBM model. Combination therapy with anti-PD-L1 antibody further increased the therapeutic effect on survival. A Phase I trial of DNX-2440, which is armed with human OX40L, is currently ongoing for patients with recurrent glioblastoma (NCT03714334).
The genomic structures of 2nd and 3rd generations of CRAd are illustrated in .
Figure 1 Genomic structure of oncolytic adenoviruses currently used in pre-clinical and clinical studies on high-grade glioma. ITR, inverted terminal repeat. E1AΔ24: a deletion of 24 base pairs within the E1A region. DM: insulator DM-1. E2Fp: E2F-responsive promoter. K: a Kozak sequence. E2F-pal: E2F-responsive palindromes (8 E2F-binding sites). PH20: human sperm PH20 hyaluronidase cDNA. RGD: an RGD integrin-binding motif in the HI loop of the fiber. RGDK: RGDK in the putative heparin sulfate-glycosaminoglycans binding domain KKTK in the fiber shaft. pCMV: the cytomegalovirus promoter. mOX40L: mouse OX40L cDNA. BGH pA: bovine growth hormone poly-adenylation signal. The mOX40L expression cassette replaces the E3 region in Delta-24-RGDOX.
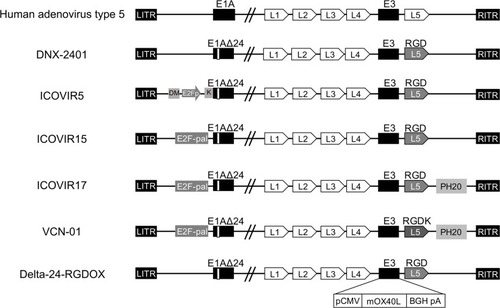
Induction Of Anti-Tumor Immune Response By CRAd
The CNS has long been considered the “immunologically privileged site” representing an immunologically distinct environment from other organs. Glioma in the brain induces angiogenesis and disruption of the blood brain barrier (BBB), which allows various drugs and immune cells to enter the tumor. However, the microenvironment of GBM is highly immunosuppressive characterized by secretion of immunosuppressive cytokines (TGF-β, IL-10, etc.), and the dominance of immuno-suppressive immune cells such as regulatory T cells, functionally exhausted T cells, myeloid-derived suppressor cells and M2-polarized tumor-associated macrophages.Citation40–Citation43 In recent years, immune checkpoint blockade has emerged as a novel immune-therapeutic modality for cancer, but unfortunately, the Phase III CheckMate-143 study (NCT02017717) investigating the anti-PD-1 nivolumab monotherapy in recurrent GBM patients did not extend overall survival compared with bevacizumab (Reardon et al, WFNOS2017Citation44). This result may be attributable to the immunosuppressive microenvironment of GBM and suggest that combination with other therapy that stimulates immune responses may enhance the efficacy of immune checkpoint inhibitors in GBM patients.Citation45
CRAd has been shown to alter the immune microenvironment in experimental GBM in mice.Citation46–Citation48 Delta-24-RGD (DNX-2401) treatment of GL261 murine GBM recruited F4/80+ macrophages, NK cells and CD4+ and CD8+ T cells to the tumor site in C57/BL6 mice, and induced T cells to react to the virus and tumor cells.Citation46,Citation47 These immune effects mediated the therapeutic efficacy of DNX-2401 as co-treatment with dexamethasone, an immunosuppressive steroid, abrogated the survival benefit in mice.Citation47 Thus, adenoviral modification of the immunological landscape within HGG can set a stage for enhancing the efficacy of immunotherapy for HGG. Indeed, Jiang et al showed that the combination therapy with Delta-24-RGDOX and anti-PD-L1 antibody, both administered intratumorally twice, effectively prolonged the survival of murine GL261 GBM model,Citation39 providing a rationale for clinical testing of this immunotherapeutic strategy.
Clinical Trial Of DNX-2401 For Malignant Gliomas
summarizes clinical trials of CRAd for HGGs that recently completed or are currently active.
Treatment With DNX-2401 In Recurrent High-Grade Gliomas
Lang et al reported the results of a Phase I trial of DNX-2401 for recurrent malignant gliomaCitation32 (NCT00805376). Thirty-seven patients were enrolled and divided into Group A (n = 25) and Group B (n = 12). In Group A, DNX-2401 at escalating doses (1 x 107 to 3 x 1010 viral particles) was stereotactically injected into the tumor after biopsy confirming tumor recurrence. Following treatment, 20% of patients (5 of 25) survived more than 3 years, and dramatic tumor reduction (95% or more, CR) was seen in 3 patients, whose progression-free survival (PFS) was over 3 years. To investigate the mechanism of virus treatment, tumors in Group B were injected with DNX-2401 (1 x 107 to 3 x 108 viral particles) through catheters inserted into the tumors and the tumors were resected 14 days later, along with a second DNX-2401 injection to the wall of the resection cavity. Interestingly, MRI of the 3 complete responders showed an initial increase in contrast enhancement within 4 months after injection of DNX-2401, suggesting an immune or inflammatory response, followed by regression over 1–1.5 years. Immunohistochemical analysis showed evidence of DNX-2401 replication within the tumor in 6 of 11 Group B tumors (55%) 2 weeks post-virus treatment. Furthermore, intratumoral infiltration of CD4+ T cells and T-bet+ cells, suggestive of a helper 1 (Th1) response, was observed. Among immune checkpoint proteins, expression of TIM3, but not PD-L1, was decreased after treatment with DNX-2401. Histological examination of a lesion that developed distant from the treated tumor and was resected in one of the complete responders revealed inflammatory cells, with no evidence of tumor cells, suggesting a response driven by adaptive immune memory. Thus, this clinical study for the first time showed direct oncolytic effects in human brain tumors and provided evidence for elicitation of anti-glioma immune responses. No dose-limiting toxicities were observed in this trial. Adverse effects were reported in 15% of patients, but no serious events of grade 3 or higher were noted that were considered to be related to the virus, supporting the safety.
In the Netherlands, a Phase I/II clinical trial was initiated in 2010 testing DNX-2401 for recurrent glioblastoma (NCT01582516). The virus is administered using convection-enhanced delivery via catheters targeting tumor mass as well as the surrounding infiltrated brain. Using tumor tissues and cerebrospinal fluid (CSF) collected from the study participants, van den Bossche et al studied the impact of DNX-2401 injection on tumor macrophages.Citation49 In some patients, DNX-2401 treatment increased the CSF concentrations of cytokines such as TNF, IL6 and IFNγ, and such CSF was able to increase the levels of CD64, a marker of M1-polarization, on macrophages in vitro. A tumor that re-recurred and was resected 26 months after DNX-2401 therapy provided a valuable research material that suggested a prolonged shift of a macrophage phenotype from M2 to M1. The feasibility and efficacy results of the trial have not been published.
DNX-2401 And Immunotherapy
Because of the ability of CRAd to alter the immune status by turning the cold tumor microenvironment to hot (inflamed), combining CRAd with immune-modulating therapies is a rational approach, which is supported by preclinical studies for GBMCitation39 and other cancers.Citation50–Citation52 Currently, a Phase II multicenter study is investigating a combination therapy of DNX-2401 and Pembrolizumab (anti-PD-1 antibody) for recurrent malignant glioma (CAPTIVE, NCT02798406). In this trial, a single intratumoral dose of DNX-2401 (5x108, 5x109 and 5x1010 virus particles) is injected via cannula inserted into the tumor, and followed 7 days later by intravenous injection of 200 mg pembrolizumab every 3 weeks for up to 24 months or until confirmed progression, intolerable toxicity or study withdrawal. Interim outcome was reported at the SNO 2018 annual meeting that 23 patients have been treated and DNX-2401 followed by pembrolizumab has been well tolerated (Zadeh et al,Citation53 SNO2018). Preliminary efficacy is encouraging as two partial responses and 100% 9-month survival for the first 7 patients treated were noted. Publication of longer follow-up data is eagerly awaited.
Addition Of IFNγ To DNX-2401 Did Not Improve Survival
Lang et al reported at ASCO 2017 the Phase Ib trial of DNX-2401 with or without interferon gamma (IFNγ) for recurrent glioblastoma (TARGET-ICitation54). Twenty-seven patients were enrolled and randomized to intratumoral DNX-2401 followed by subcutaneous IFNγ (n = 18) or to DNX-2401 alone (n = 9). Due to the poor tolerability of IFNγ, the median duration of treatment was only 6 weeks. Overall survival of 12 months and 18 months for all patients enrolled was 33% and 22%, respectively, regardless of treatment assignment. Three patients (DNX-2401, n=1; DNX-2401 with IFNγ, n=2) remain alive over 1.5 years after the treatments.
DNX-2401 Combination With TMZ
The alkylating agent Temozolomide (TMZ) is part of the standard treatment for newly diagnosed GBM.Citation2 TMZ chemosensitivity is known to depend on epigenetic silencing of O6-methylguanine-DNA methyltransferase (MGMT) by promoter methylation.Citation55 Pre-clinically, DNX-2401 infection was shown to reduce MGMT levels in human GBM cell lines (U87 and T98G) in vitro and decrease IC50 of TMZ.Citation56 In vivo, combination therapy of DNX-2401 and TMZ significantly extended survival in a GBM xenograft model in immunodeficient mouse.Citation56 Kleijn et al subsequently showed that combination was also efficacious in immunocompetent mouse GBM models.Citation57 These studies provided a rationale for the Phase I trial of the combination therapy of DNX-2401 and TMZ that is ongoing in Spain for recurrent glioblastoma (NCT01956734).
DNX-2440 – Oncolytic Adenovirus Armed With T Cell Stimulator
A Phase I trial of intratumoral injection of DNX-2440, a modified version of DNX-2401 armed with OX40L, for recurrent glioblastoma is ongoing in Spain (NCT03714334). This is the first clinical trial testing a 3rd generation CRAd for malignant glioma.
DNX-2401 In Pediatric Glioma
Pediatric high-grade gliomas (pHGG) are distinct from adult HGG as pHGG harbor genetic drivers that adult HGGs do not and arise at different CNS locations. Diffuse intrinsic pontine glioma (DIPG) is an aggressive pHGG that arises in the brainstem and is characterized by histone H3 K27M mutation that is also frequent in pediatric gliomas in the midline structures such as the thalamus. pHGG of the cerebral cortex typically harbors pG34R/V histone H3 mutations. In contrast, H3 mutations are extremely rare in adult HGGs.Citation58,Citation59 Because of the vital functions of the brainstem, resection is not a therapeutic option for DIPG. Radiotherapy is the standard of care for DIPG, but this only improves the quality of life for several months and is not curative.Citation60
Preclinically, Martinez-Velez et al reported that DNX-2401 viral infection and replication were observed in several human and murine DIPG cell lines. Intratumoral administration of DNX-2401 was safe in mice and resulted in a significant increase of survival rate in both immunodeficient and immunocompetent models of pHGG and DIPG.Citation61 The same group further showed that DNX-2401 treatment downregulated DNA damage repair proteins and when combined with radiotherapy mediated a synergistic anti-glioma effect in mouse models of pHGG and DIPG.Citation62 Currently, a Phase I trial of DNX-2401 for newly diagnosed DIPG is ongoing in Spain (NCT03178032Citation63). And a case report in this trial showed the tolerability of DNX-2401 in an 8-year-old DIPG patient. DNX-2401 was injected via a catheter into the tumor within the pons following biopsy and the procedure was not associated with safety problems or new neurological deficit.Citation64 After 3–4 weeks of virus administration, patients will start receiving standard radiation therapy.
Stem Cell Delivery Of CRAd To HGG
Because of their ability to migrate preferentially toward tumor cells, stem cells have been explored as vehicles to deliver antitumor agents including OV to brain tumors.Citation65–Citation67 Preclinically, combination of different types of stem cells and CRAd have been studied as experimental treatments of glioma; mesenchymal stem cell MSC-Delta24-RGD,Citation68 and MSC-ICOVIR17,Citation36 MSC-CRAd-CXCR4-5/3,Citation69 neural stem cells (NSC)-CRAd-survivin-pk7Citation69–Citation72 demonstrated anti-glioma activity. CRAd-CXCR4-5/3 and CRAd survivin-pk7 are transcriptionally targeted CRAd using tumor-specific C-X-C chemokine receptor 4 (CXCR4) and survivin promotor, respectively, to control E1A expression and target the viruses to GBM.Citation73,Citation74 CRAd-CXCR4-5/3 has a chimeric Ad 5/3 fiber (chimera of the shaft of human adenovirus type 5 and the knob of human adenovirus type 3) that targets CD46 or CD80/86 cellular receptors and exhibits increased transduction of malignant glioma compared with wild-type Ad5.Citation73,Citation75 CRAd-Survivin-pk7 has fiber modification with pk7 encoding polylysine that was designed to increase transduction of malignant glioma by direct binding to heparan sulfate and polyanionic cellular receptorsCitation74,Citation76,Citation77 Currently, a Phase I trial is ongoing in the US (NCT03072134) in which NSC loaded with CRAd-survivin-pk7 are injected into the resection cavity of newly diagnosed GBM.
Gene-Mediated Cytotoxic Immunotherapy Using Adenoviral Vectors
Adenovirus vectors that deliver the thymidine kinase gene of HSV (HSV-tk) have been long studied as a therapeutic strategy for GBM. Adenoviral transduction of HSV-tk in tumor cells followed by systemic administration of a nucleoside analog prodrug such as ganciclovir induces the phosphorylation of the prodrug, termination of DNA replication and death in dividing cells, as well as neighboring cells through the bystander effect.Citation78,Citation79 The death in cancer cells results in release of tumor neoantigens and elicitation of anti-tumor cellular immune responses.Citation78,Citation80 Although this modality, so-called gene-mediated cytotoxic immunotherapy (GMCI), is outside the field of OV therapy due to use of replication-defective vectors, induction of tumor-selective immunogenic cell death may underlie a mechanism-of-action common in GMCI and CRAd therapies.
In neuro-oncology, Ad-HSV-tk (AdV-tk) was first tested clinically in a Phase I study in patients with recurrent malignant glioma.Citation81 A subsequent Phase Ib dose-escalation study for newly diagnosed GBMCitation82 confirmed the tolerability and safety of the local injection of the vector and oral valacyclovir administration. A Phase II study conducted in parallel showed an increase in overall survival of patients receiving GMCI, as compared with those treated with the standard of care, with prominent efficacy observed when gross total resection was followed by GMCI. Recently, a potential efficacy of GMCI was reported in pediatric patients with glioma (NCT00634231).Citation83 Currently, a Phase I trial is investigating the combination of GMCI and immune checkpoint blockade with novolumab for newly diagnosed high-grade gliomas (NCT03576612). Another interesting clinical trial for newly diagnosed malignant gliomas involves a rational combination of two adenoviral vectors that express HSV-tk or Flt3L, a cytokine that differentiates precursors into dendritic cells and recruits those to the brain tumor environment (NCT01811992).Citation84
Discussion – Challenges And Future Directions
During the last 10 years, research on CRAd has been rapidly evolving as a potential novel treatment for patients with HGG. Extensive efforts to translate findings gained from preclinical research using animal models have resulted in a number of early-stage (Phase I and II) clinical trials that have completed or are active in the US and Europe (). The results of these clinical investigations, regarding feasibility, safety and efficacy, have largely been unpublished yet. As shown by the report by Lang et al,Citation32 survival and radiographic (MRI) follow-up as well as analysis of biospecimens including tumors, CSF, serum, sputum and urine all provide valuable information that helps us understand the complex biological processes and mechanisms-of-action of oncolytic adenovirus therapy (). Detailed reports of trials are therefore awaited by the large research community and patients alike. DNX-2401 is currently the mainstream CRAd that is under vigorous testing in multiple trials, and may become the first FDA-approved CRAd. That 20% of patients with recurrent HGGs (mostly IDH wildtype GBM) survived over 3 years is encouraging and such a long survival tail in a fraction of patients is consistent with the results with PVS-RIPO (poliovirus).Citation85
Figure 2 Dynamic changes that occur over time within the tumor microenvironment after oncolytic adenovirus therapy of glioblastoma. Upper, macroscopic and radiographic changes. Lower, microscopic-level changes. (A) Before treatment, the GBM tumor microenvironment is immune-suppressive as characterized by M2-polarized macrophages and few effector T cells. (B) A few days after virus administration, oncolytic adenovirus is actively replicating within the tumor, starting to induce the recruitment of effector T cells and macrophages with a shift from an M2 to M1 phenotype. (C) A few months later, the tumor exhibits an increase in size on magnetic resonance imaging (MRI). At this stage, virus has been cleared, but an active inflammatory reaction persists with the infiltration of immune cells such as T cells and M1-polarized macrophages. Tumor cells are essentially absent (necrosis), despite the worsening of imaging (pseudo-progression). (D) During the follow-up, inflammation gradually subsides and MRI shows progressive regression of the lesion and associated edema, which could take over a year.
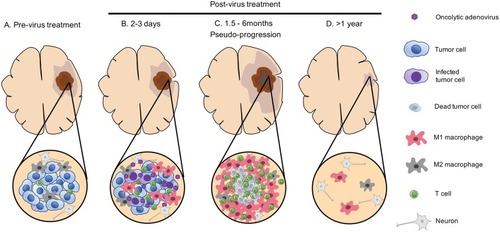
Since the clinical benefits of DNX-2401 were very variable between patients,Citation32 search of biomarkers of response is one of the critical tasks. One of the intriguing findings that Lang et al reported with the DNX-2401 trial was that, in all three patients who responded extremely well to DNX-2401 treatment, contrast enhancement of treated lesions showed an increase on MRI between 1 and 6 months after therapy, which then subsided and almost disappeared (). This initial enlargement of the lesions in responders is considered pseudo-progression (therapy-mediated tumor enhancement), a phenomenon frequently reported in OV therapy for other cancersCitation3 and explained as inflammatory responses triggered by the virus. The identification of pseudo-progression after clinical CRAd therapy for HGGs raises several questions. First, how can pseudo-progression and recurrence/progression be distinguished, and for this purpose if biospecimens useful or imaging modalities sufficient? Second, is pseudo-progression a reliable biomarker of response and clinical benefit? The Lang report seems to be supportive, but did not provide definitive yes to this question. Third, when pseudo-progression directly causes local brain symptoms or elevation of intracranial pressure, will the use of glucocorticosteroid be appropriate to noninvasively manage the conditions without negatively impacting anti-tumor immune responses and ultimate outcomes? Accumulation of clinical data may be able to address these questions.
The DNX-2401 trial validated prior preclinical research that injection of CRAd elicits the recruitment of immune cells, particularly T cells, to the tumor site. This is an indication that immunocompetent mouse model of HGGCitation39,Citation46,Citation47,Citation49,Citation57,Citation61,Citation86 was useful to predict, at least, some biological changes evoked in patients by CRAd therapy. However, all current CRAds are based on human adenovirus that replicates poorly in mouse cells.Citation87 To overcome this problem, multiple administration of high-dose CRAd has been the commonly used approach to mimic the situation of good virus replication.Citation39,Citation51 Alternative animal models such as Syrian hamster,Citation88 pigCitation89 or use of humanized mouse modelsCitation50,Citation90 are being developed and could be shown in the future to better represent clinical scenarios of CRAd therapy.
The field of CRAd is rapidly evolving. The 3rd generation of CRAd, designed to be more potent and maintain safety, are entering several cancer clinical trials as monotherapy or combination,Citation67 including a DNX-2440 trial for GBM (NCT03714334). We expect that knowledge on efficacy, feasibility, safety and mechanisms-of-action that will be gained from the current CRAd trails in neuro-oncology will boost efforts of developing newer generations of CRAd and help accelerate transition to their clinical evaluation.
Disclosure
The authors report no conflicts of interest in this work.
References
- Louis D, von Deimling A, Cavenee W 1. Disffuse astrocytic and oligodendroglial tumors. WHO classifivation of tumours of the central nervous system Revised 4th IARC; 2016; Lyon, France:15–78.
- Stupp R, Mason WP, van Den Bent MJ, et al. Radiotherapy plus concomitant and adjuvant temozolomide for glioblastoma. N Engl J Med. 2005;352(10):987–996. doi:10.1056/NEJMoa04333015758009
- Larson C, Oronsky B, Scicinski J, et al. Going viral: a review of replication-selective oncolytic adenoviruses. Oncotarget. 2015;6(24):19976–19989. doi:10.18632/oncotarget.511626280277
- Kelly E, Russell SJ. History of oncolytic viruses: genesis to genetic engineering. Mol Ther. 2007;15(4):651–659. doi:10.1038/sj.mt.630010817299401
- Alemany R. Viruses in cancer treatment. Clin Transl Oncol. 2013;15(3):182–188. doi:10.1007/s12094-012-0951-723143950
- Bell J, McFadden G. Viruses for tumor therapy. Cell Host Microbe. 2014;15(3):260–265. doi:10.1016/j.chom.2014.01.00224629333
- Andtbacka RH, Kaufman HL, Collichio F, et al. Talimogene laherparepvec improves durable response rate in patients with advanced melanoma. J Clin Oncol. 2015;33(25):2780–2788. doi:10.1200/JCO.2014.58.337726014293
- Cervera-Carrascon V, Havunen R, Hemminki A. Oncolytic adenoviruses: a game changer approach in the battle between cancer and the immune system. Expert Opin Biol Ther. 2019;19(5):443–455. doi:10.1080/14712598.2019.159558230905206
- Alemany R. Oncolytic adenoviruses in cancer treatment. Biomedicines. 2014;2(1):36–49. doi:10.3390/biomedicines201003628548059
- Niemann J, Kuhnel F. Oncolytic viruses: adenoviruses. Virus Genes. 2017;53(5):700–706. doi:10.1007/s11262-017-1488-128702840
- Baker AT, Aguirre-Hernandez C, Hallden G, Parker AL. Designer oncolytic adenovirus: coming of age. Cancers (Basel). 2018;10(6). doi:10.3390/cancers10110400
- Bischoff JR, Kirn DH, Williams A, et al. An adenovirus mutant that replicates selectively in p53-deficient human tumor cells. Science. 1996;274(5286):373–376. doi:10.1126/science.274.5286.3738832876
- Edwards SJ, Dix BR, Myers CJ, et al. Evidence that replication of the antitumor adenovirus ONYX-015 is not controlled by the p53 and p14(ARF) tumor suppressor genes. J Virol. 2002;76(24):12483–12490. doi:10.1128/jvi.76.24.12483-12490.200212438574
- Rothmann T, Hengstermann A, Whitaker NJ, Scheffner M, Zur Hausen H. Replication of ONYX-015, a potential anticancer adenovirus, is independent of p53 status in tumor cells. J Virol. 1998;72(12):9470–9478.9811680
- Geoerger B, Grill J, Opolon P, et al. Oncolytic activity of the E1B-55 kDa-deleted adenovirus ONYX-015 is independent of cellular p53 status in human malignant glioma xenografts. Cancer Res. 2002;62(3):764–772.11830531
- O’Shea CC, Johnson L, Bagus B, et al. Late viral RNA export, rather than p53 inactivation, determines ONYX-015 tumor selectivity. Cancer Cell. 2004;6(6):611–623. doi:10.1016/j.ccr.2004.11.01215607965
- O’Shea CC, Soria C, Bagus B, McCormick F. Heat shock phenocopies E1B-55K late functions and selectively sensitizes refractory tumor cells to ONYX-015 oncolytic viral therapy. Cancer Cell. 2005;8(1):61–74. doi:10.1016/j.ccr.2005.06.00916023599
- Chiocca EA, Abbed KM, Tatter S, et al. A phase I open-label, dose-escalation, multi-institutional trial of injection with an E1B-Attenuated adenovirus, ONYX-015, into the peritumoral region of recurrent malignant gliomas, in the adjuvant setting. Mol Ther. 2004;10(5):958–966. doi:10.1016/j.ymthe.2004.07.02115509513
- Kirn D. Clinical research results with dl1520 (Onyx-015), a replication-selective adenovirus for the treatment of cancer: what have we learned? Gene Ther. 2001;8(2):89–98. doi:10.1038/sj.gt.330137711313778
- Wildner O. Clinical trials: the sensitizing side of Onyx-015. Gene Ther. 2005;12(5):386–387.19202637
- Xia ZJ, Chang JH, Zhang L, et al. Phase III randomized clinical trial of intratumoral injection of E1B gene-deleted adenovirus (H101) combined with cisplatin-based chemotherapy in treating squamous cell cancer of head and neck or esophagus (Article in Chinese). Ai Zheng. 2004;23(12):1666–1670.15601557
- Garber K. China approves world’s first oncolytic virus therapy for cancer treatment. J Natl Cancer Inst. 2006;98(5):298–300. doi:10.1093/jnci/djj11116507823
- Fueyo J, Gomez-Manzano C, Alemany R, et al. A mutant oncolytic adenovirus targeting the Rb pathway produces anti-glioma effect in vivo. Oncogene. 2000;19(1):2–12. doi:10.1038/sj.onc.120325110644974
- Nevins JR. E2F: a link between the Rb tumor suppressor protein and viral oncoproteins. Science. 1992;258(5081):424–429. doi:10.1126/science.14115351411535
- Heise C, Hermiston T, Johnson L, et al. An adenovirus E1A mutant that demonstrates potent and selective systemic anti-tumoral efficacy. Nat Med. 2000;6(10):1134–1139. doi:10.1038/8047411017145
- Reeh M, Bockhorn M, Gorgens D, et al. Presence of the coxsackievirus and adenovirus receptor (CAR) in human neoplasms: a multitumour array analysis. Br J Cancer. 2013;109(7):1848–1858. doi:10.1038/bjc.2013.50924022195
- Miller CR, Buchsbaum DJ, Reynolds PN, et al. Differential susceptibility of primary and established human glioma cells to adenovirus infection: targeting via the epidermal growth factor receptor achieves fiber receptor-independent gene transfer. Cancer Res. 1998;58(24):5738–5748.9865732
- Fuxe J, Liu L, Malin S, Philipson L, Collins VP, Pettersson RF. Expression of the coxsackie and adenovirus receptor in human astrocytic tumors and xenografts. Int J Cancer. 2003;103(6):723–729. doi:10.1002/ijc.1089112516090
- Suzuki K, Fueyo J, Krasnykh V, Reynolds PN, Curiel DT, Alemany R. A conditionally replicative adenovirus with enhanced infectivity shows improved oncolytic potency. Clin Cancer Res. 2001;7(1):120–126.11205899
- Pasqualini R, Koivunen E, Ruoslahti E. Alpha v integrins as receptors for tumor targeting by circulating ligands. Nat Biotechnol. 1997;15(6):542–546. doi:10.1038/nbt0697-5429181576
- Gladson CL, Cheresh DA. Glioblastoma expression of vitronectin and the alpha v beta 3 integrin. Adhesion mechanism for transformed glial cells. J Clin Invest. 1991;88(6):1924–1932. doi:10.1172/JCI1155161721625
- Lang FF, Conrad C, Gomez-Manzano C, et al. Phase I study of DNX-2401 (Delta-24-RGD) oncolytic adenovirus: replication and immunotherapeutic effects in recurrent malignant glioma. J Clin Oncol. 2018;36(14):1419–1427. doi:10.1200/JCO.2017.75.821929432077
- Alonso MM, Cascallo M, Gomez-Manzano C, et al. ICOVIR-5 shows E2F1 addiction and potent antiglioma effect in vivo. Cancer Res. 2007;67(17):8255–8263. doi:10.1158/0008-5472.CAN-06-467517804740
- Rojas JJ, Guedan S, Searle PF, et al. Minimal RB-responsive E1A promoter modification to attain potency, selectivity, and transgene-arming capacity in oncolytic adenoviruses. Mol Ther. 2010;18(11):1960–1971. doi:10.1038/mt.2010.17320808288
- Guedan S, Rojas JJ, Gros A, Mercade E, Cascallo M, Alemany R. Hyaluronidase expression by an oncolytic adenovirus enhances its intratumoral spread and suppresses tumor growth. Mol Ther. 2010;18(7):1275–1283. doi:10.1038/mt.2010.7920442708
- Martinez-Quintanilla J, He D, Wakimoto H, Alemany R, Shah K. Encapsulated stem cells loaded with hyaluronidase-expressing oncolytic virus for brain tumor therapy. Mol Ther. 2015;23(1):108–118. doi:10.1038/mt.2014.20425352242
- Vera B, Martinez-Velez N, Xipell E, et al. Characterization of the antiglioma effect of the oncolytic adenovirus VCN-01. PLoS One. 2016;11(1):e0147211. doi:10.1371/journal.pone.014721126808201
- Bayo-Puxan N, Gimenez-Alejandre M, Lavilla-Alonso S, et al. Replacement of adenovirus type 5 fiber shaft heparan sulfate proteoglycan-binding domain with RGD for improved tumor infectivity and targeting. Hum Gene Ther. 2009;20(10):1214–1221. doi:10.1089/hum.2009.03819537946
- Jiang H, Rivera-Molina Y, Gomez-Manzano C, et al. Oncolytic adenovirus and tumor-targeting immune modulatory therapy improve autologous cancer vaccination. Cancer Res. 2017;77(14):3894–3907. doi:10.1158/0008-5472.CAN-17-046828566332
- Ma Q, Long W, Xing C, et al. Cancer stem cells and immunosuppressive microenvironment in glioma. Front Immunol. 2018;9:2924. doi:10.3389/fimmu.2018.0292430619286
- Ochs K, Sahm F, Opitz CA, et al. Immature mesenchymal stem cell-like pericytes as mediators of immunosuppression in human malignant glioma. J Neuroimmunol. 2013;265(1–2):106–116. doi:10.1016/j.jneuroim.2013.09.01124090655
- Sokratous G, Polyzoidis S, Ashkan K. Immune infiltration of tumor microenvironment following immunotherapy for glioblastoma multiforme. Hum Vaccin Immunother. 2017;13(11):2575–2582. doi:10.1080/21645515.2017.130358228362548
- Becker JC, Andersen MH, Schrama D, Thor Straten P. Immune-suppressive properties of the tumor microenvironment. Cancer Immunol Immunother. 2013;62(7):1137–1148. doi:10.1007/s00262-013-1434-623666510
- Renfrow JJ, Weller M, Strowd RE. Meeting update-world federation of neuro-oncology societies (WFNOS) meeting 2017. Neuro Oncol. 2017;19(11):1457–1459. doi:10.1093/neuonc/nox18429059448
- Filley AC, Henriquez M, Dey M. Recurrent glioma clinical trial, CheckMate-143: the game is not over yet. Oncotarget. 2017;8(53):91779–91794. doi:10.18632/oncotarget.2158629207684
- Jiang H, Clise-Dwyer K, Ruisaard KE, et al. Delta-24-RGD oncolytic adenovirus elicits anti-glioma immunity in an immunocompetent mouse model. PLoS One. 2014;9(5):e97407. doi:10.1371/journal.pone.009740724827739
- Kleijn A, Kloezeman J, Treffers-Westerlaken E, et al. The in vivo therapeutic efficacy of the oncolytic adenovirus Delta24-RGD is mediated by tumor-specific immunity. PLoS One. 2014;9(5):e97495. doi:10.1371/journal.pone.009749524866126
- Kleijn A, Kloezeman J, Treffers-Westerlaken E, et al. The therapeutic efficacy of the oncolytic virus Delta24-RGD in a murine glioma model depends primarily on antitumor immunity. Oncoimmunology. 2014;3(9):e955697. doi:10.4161/21624011.2014.95569725941622
- van Den Bossche WBL, Kleijn A, Teunissen CE, et al. Oncolytic virotherapy in glioblastoma patients induces a tumor macrophage phenotypic shift leading to an altered glioblastoma microenvironment. Neuro Oncol. 2018;20(11):1494–1504. doi:10.1093/neuonc/noy08229796615
- Kuryk L, Moller AW, Jaderberg M. Combination of immunogenic oncolytic adenovirus ONCOS-102 with anti-PD-1 pembrolizumab exhibits synergistic antitumor effect in humanized A2058 melanoma huNOG mouse model. Oncoimmunology. 2019;8(2):e1532763. doi:10.1080/2162402X.2018.153276330713786
- Cervera-Carrascon V, Siurala M, Santos JM, et al. TNFa and IL-2 armed adenoviruses enable complete responses by anti-PD-1 checkpoint blockade. Oncoimmunology. 2018;7(5):e1412902. doi:10.1080/2162402X.2018.149085429721366
- Woller N, Gurlevik E, Fleischmann-Mundt B, et al. Viral infection of tumors overcomes resistance to PD-1-immunotherapy by broadening neoantigenome-directed T-cell responses. Mol Ther. 2015;23(10):1630–1640. doi:10.1038/mt.2015.11526112079
- Gea Z. Abstract – interim results of a phase II multicenter study of the conditionally replicative oncolytic adenovirus DNX-2401 with pembrolizumab(Keytruda) for recurrent glioblastoma; CAPTIVE STUDY (LEYNOTE-192). Neuro-Oncology. 2018;20(suppl_6):vi6. doi:10.1093/neuonc/noy148.019
- Lang FF, Tran ND, Puduvalli VK, et al. Phase 1b open-label randomized study of the oncolytic adenovirus DNX-2401 administered with or without interferon gamma for recurrent glioblastoma. J Clin Oncol. 2017;35(15_suppl):2002. doi:10.1200/JCO.2017.35.15_suppl.2002
- Dunn J, Baborie A, Alam F, et al. Extent of MGMT promoter methylation correlates with outcome in glioblastomas given temozolomide and radiotherapy. Br J Cancer. 2009;101(1):124–131. doi:10.1038/sj.bjc.660512719536096
- Alonso MM, Gomez-Manzano C, Bekele BN, Yung WK, Fueyo J. Adenovirus-based strategies overcome temozolomide resistance by silencing the O6-methylguanine-DNA methyltransferase promoter. Cancer Res. 2007;67(24):11499–11504. doi:10.1158/0008-5472.CAN-07-531218089777
- Kleijn A, van Den Bossche W, Haefner ES, et al. The sequence of delta24-RGD and TMZ administration in malignant glioma affects the role of CD8(+)T cell anti-tumor activity. Mol Ther Oncolytics. 2017;5:11–19. doi:10.1016/j.omto.2017.02.00228480325
- Wu G, Broniscer A, McEachron TA, et al. Somatic histone H3 alterations in pediatric diffuse intrinsic pontine gliomas and non-brainstem glioblastomas. Nat Genet. 2012;44(3):251–253. doi:10.1038/ng.110222286216
- Wu G, Diaz AK, Paugh BS, et al. The genomic landscape of diffuse intrinsic pontine glioma and pediatric non-brainstem high-grade glioma. Nat Genet. 2014;46(5):444–450. doi:10.1038/ng.293824705251
- Warren KE. Diffuse intrinsic pontine glioma: poised for progress. Front Oncol. 2012;2:205. doi:10.3389/fonc.2012.0020523293772
- Martinez-Velez N, Garcia-Moure M, Marigil M, et al. The oncolytic virus Delta-24-RGD elicits an antitumor effect in pediatric glioma and DIPG mouse models. Nat Commun. 2019;10(1):2235. doi:10.1038/s41467-019-10043-031138805
- Martinez-Velez N, Marigil M, Garcia-Moure M, et al. Delta-24-RGD combined with radiotherapy exerts a potent antitumor effect in diffuse intrinsic pontine glioma and pediatric high grade glioma models. Acta Neuropathol Commun. 2019;7(1):64. doi:10.1186/s40478-019-0714-631036068
- Tejada S, Alonso M, Patino A, Fueyo J, Gomez-Manzano C, Diez-Valle R. Phase I trial of DNX-2401 for diffuse intrinsic pontine glioma newly diagnosed in pediatric patients. Neurosurgery. 2018;83(5):1050–1056. doi:10.1093/neuros/nyx50729088386
- Tejada S, Diez-Valle R, Dominguez PD, et al. DNX-2401, an oncolytic virus, for the treatment of newly diagnosed diffuse intrinsic pontine gliomas: a case report. Front Oncol. 2018;8:61. doi:10.3389/fonc.2018.0006129594041
- Aboody KS, Brown A, Rainov NG, et al. Neural stem cells display extensive tropism for pathology in adult brain: evidence from intracranial gliomas. Proc Natl Acad Sci U S A. 2000;97(23):12846–12851. doi:10.1073/pnas.97.23.1284611070094
- Lesniak MS. Targeted therapy for malignant glioma: neural stem cells. Expert Rev Neurother. 2006;6(1):1–3. doi:10.1586/14737175.6.1.116466305
- Martinez-Quintanilla J, Seah I, Chua M, Shah K. Oncolytic viruses: overcoming translational challenges. J Clin Invest. 2019;130:1407–1418. doi:10.1172/JCI12228730829653
- Yong RL, Shinojima N, Fueyo J, et al. Human bone marrow-derived mesenchymal stem cells for intravascular delivery of oncolytic adenovirus Delta24-RGD to human gliomas. Cancer Res. 2009;69(23):8932–8940. doi:10.1158/0008-5472.CAN-08-387319920199
- Sonabend AM, Ulasov IV, Tyler MA, Rivera AA, Mathis JM, Lesniak MS. Mesenchymal stem cells effectively deliver an oncolytic adenovirus to intracranial glioma. Stem Cells. 2008;26(3):831–841. doi:10.1634/stemcells.2007-075818192232
- Ahmed AU, Thaci B, Tobias AL, et al. A preclinical evaluation of neural stem cell-based cell carrier for targeted antiglioma oncolytic virotherapy. J Natl Cancer Inst. 2013;105(13):968–977. doi:10.1093/jnci/djt14123821758
- Tyler MA, Ulasov IV, Sonabend AM, et al. Neural stem cells target intracranial glioma to deliver an oncolytic adenovirus in vivo. Gene Ther. 2009;16(2):262–278. doi:10.1038/gt.2008.16519078993
- Kim CK, Ahmed AU, Auffinger B, et al. N-acetylcysteine amide augments the therapeutic effect of neural stem cell-based antiglioma oncolytic virotherapy. Mol Ther. 2013;21(11):2063–2073. doi:10.1038/mt.2013.17923883863
- Ulasov IV, Rivera AA, Sonabend AM, et al. Comparative evaluation of survivin, midkine and CXCR4 promoters for transcriptional targeting of glioma gene therapy. Cancer Biol Ther. 2007;6(5):679–685. doi:10.4161/cbt.6.5.395717404502
- Ulasov IV, Zhu ZB, Tyler MA, et al. Survivin-driven and fiber-modified oncolytic adenovirus exhibits potent antitumor activity in established intracranial glioma. Hum Gene Ther. 2007;18(7):589–602. doi:10.1089/hum.2007.00217630837
- Ulasov IV, Rivera AA, Han Y, Curiel DT, Zhu ZB, Lesniak MS. Targeting adenovirus to CD80 and CD86 receptors increases gene transfer efficiency to malignant glioma cells. J Neurosurg. 2007;107(3):617–627. doi:10.3171/JNS-07/09/061717886563
- Staba MJ, Wickham TJ, Kovesdi I, Hallahan DE. Modifications of the fiber in adenovirus vectors increase tropism for malignant glioma models. Cancer Gene Ther. 2000;7(1):13–19. doi:10.1038/sj.cgt.770010410678351
- Zheng S, Ulasov IV, Han Y, Tyler MA, Zhu ZB, Lesniak MS. Fiber-knob modifications enhance adenoviral tropism and gene transfer in malignant glioma. J Gene Med. 2007;9(3):151–160. doi:10.1002/jgm.100817351980
- Perez-Cruet MJ, Trask TW, Chen SH, et al. Adenovirus-mediated gene therapy of experimental gliomas. J Neurosci Res. 1994;39(4):506–511. doi:10.1002/jnr.4903904177884826
- Chen SH, Shine HD, Goodman JC, Grossman RG, Woo SL. Gene therapy for brain tumors: regression of experimental gliomas by adenovirus-mediated gene transfer in vivo. Proc Natl Acad Sci U S A. 1994;91(8):3054–3057. doi:10.1073/pnas.91.8.30548159705
- Barba D, Hardin J, Sadelain M, Gage FH. Development of anti-tumor immunity following thymidine kinase-mediated killing of experimental brain tumors. Proc Natl Acad Sci U S A. 1994;91(10):4348–4352. doi:10.1073/pnas.91.10.43488183911
- Trask TW, Trask RP, Aguilar-Cordova E, et al. Phase I study of adenoviral delivery of the HSV-tk gene and ganciclovir administration in patients with current malignant brain tumors. Mol Ther. 2000;1(2):195–203. doi:10.1006/mthe.2000.003010933931
- Chiocca EA, Aguilar LK, Bell SD, et al. Phase IB study of gene-mediated cytotoxic immunotherapy adjuvant to up-front surgery and intensive timing radiation for malignant glioma. J Clin Oncol. 2011;29(27):3611–3619. doi:10.1200/JCO.2011.35.522221844505
- Kieran MW, Goumnerova L, Manley P, et al. Phase I study of gene-mediated cytotoxic immunotherapy with AdV-tk as adjuvant to surgery and radiation for pediatric malignant glioma and recurrent ependymoma. Neuro Oncol. 2019;21(4):537–546. doi:10.1093/neuonc/noy20230883662
- Lowenstein PR, Castro MG. Evolutionary basis of a new gene- and immune-therapeutic approach for the treatment of malignant brain tumors: from mice to clinical trials for glioma patients. Clin Immunol. 2018;189:43–51. doi:10.1016/j.clim.2017.07.00628720549
- Desjardins A, Gromeier M, Herndon JE 2nd, et al. Recurrent glioblastoma treated with recombinant poliovirus. N Engl J Med. 2018;379(2):150–161. doi:10.1056/NEJMoa171643529943666
- Kim JW, Miska J, Young JS, et al. A comparative study of replication-incompetent and -competent adenoviral therapy-mediated immune response in a murine glioma model. Mol Ther Oncolytics. 2017;5:97–104. doi:10.1016/j.omto.2017.05.00128573184
- Yamamoto M, Curiel DT. Current issues and future directions of oncolytic adenoviruses. Mol Ther. 2010;18(2):243–250. doi:10.1038/mt.2009.26619935777
- LaRocca CJ, Han J, Gavrikova T, et al. Oncolytic adenovirus expressing interferon alpha in a syngeneic Syrian hamster model for the treatment of pancreatic cancer. Surgery. 2015;157(5):888–898. doi:10.1016/j.surg.2015.01.00625731784
- Koodie L, Robertson MG, Chandrashekar M, et al. Rodents versus pig model for assessing the performance of serotype chimeric Ad5/3 oncolytic adenoviruses. Cancers (Basel). 2019;11(2):198. doi:10.3390/cancers11020198
- Kuryk L, Moller AW, Jaderberg M. Abscopal effect when combining oncolytic adenovirus and checkpoint inhibitor in a humanized NOG mouse model of melanoma. J Med Virol. 2019;91(9):1702–1706. doi:10.1002/jmv.2550131081549