Abstract
Tire and road wear particles (TRWP) are a component of ambient particulate matter (PM) produced from the interaction of tires with the roadway. Inhalation of PM has been associated with cardiopulmonary morbidities and mortalities thought to stem from pulmonary inflammation. To determine whether TRWP may contribute to these events, the effects of subacute inhalation of TRWP were evaluated in rats. TRWP were collected at a road simulator laboratory, aerosolized, and used to expose male and female Sprague-Dawley rats (n = 10/treatment group) at ~10, 40, or 100 μg/m3 TRWP via nose-only inhalation for 6 h/day for 28 days. Particle size distribution of the aerosolized TRWP was found to be within the respirable range for rats. Toxicity was assessed following OECD guidelines (TG 412). No TRWP-related effects were observed on survival, clinical observations, body or organ weights, gross pathology, food consumption, immune system endpoints, serum chemistry, or biochemical markers of inflammation or cytotoxicity. Rare to few focal areas of subacute inflammatory cell infiltration associated with TWRP exposure were observed in the lungs of one mid and four high exposure animals, but not the low-exposure animals. These alterations were minimal, widely scattered and considered insufficient in extent or severity to have an impact on pulmonary function. Furthermore, it is expected that these focal lesions would remain limited and may undergo resolution without long-term or progressive pulmonary alterations. Therefore, from this study we identified a no-observable-adverse-effect-level (NOAEL) of 112 μg/m3 of TRWP in rats for future use in risk assessment of TRWP.
Keywords::
Introduction
Occupational exposures to particulates have been long recognized as potential risk factors for the development of respiratory diseases (Rosner & Markowitz, Citation1991, Keith et al., Citation1977). More recently, however, exposure to ambient particulate matter (PM) has become a scientific focus, based on research indicating that fluctuations in daily PM concentrations in ambient air are correlated with increased cardiopulmonary mortalities and hospitalizations for cardiopulmonary morbidities (Dockery et al., Citation1992; Chen et al., Citation2004; Lin et al., Citation2005; Pope et al., Citation2006, Citation2008; Peters et al., Citation2001). Researchers have since drawn an association between both acute and chronic exposure to ambient PM and adverse health effects, with a particular focus on respiratory and cardiovascular outcomes (Dockery et al., Citation1993, U.S. EPA, Citation2009). This work has prompted regulators in many countries (e.g. USA, European Union, Canada, Japan) to establish standards for PM levels in the ambient atmosphere, based on both size and averaging time (). In an effort to meet these standards, regulating bodies have also taken steps to manage PM levels, such as instituting PM emission standards for PM sources, including industry and vehicles. To prioritize sources of ambient PM for management, work is ongoing to understand source apportionment of ambient PM, with respect to both magnitude of exposure and chemical composition/toxicity by source (U.S. EPA, Citation2009). For vehicle traffic, much of the focus to date with respect to toxicity and risk management has been on exhaust emissions, though nonexhaust emissions such as brake and tire wear have been recognized as contributors to ambient PM levels (Almeida-Silva et al., Citation2011; Keuken et al., Citation2010; Salma & Maenhaut, Citation2006; Health Effects Institute, Citation2010).
Table 1. International ambient air standards for particulate matter.
The focus of this article is on the potential toxicity associated with exposure to tire and road wear particles (TRWP), the particles formed at the friction interface of the road surface and the tire. During use, TRWP, which consist of a complex mixture of rubber, embedded minerals from the pavement, and free pavement, are dispersed into the environment. As they are generated, only a very small proportion of the TRWP (1% or less by mass) is found in the respirable range (e.g. <10 µm in diameter) (Kreider et al., Citation2010). Consistent with this low generation rate, measured concentrations of TRWP in the ambient air are typically low (<1% of total PM10) (Panko et al., Citation2012). However, as reduction strategies are implemented for vehicular exhaust emissions, the relative contribution of nonexhaust vehicle emissions will likely increase, thus increasing the need for understanding the potential risks associated with this portion of ambient PM.
To date, few studies have addressed the potential for TRWP to contribute to the adverse effects associated with exposure to ambient PM. Beretta et al. (Citation2007) and Gualtieri et al. (Citation2008, Citation2005) found that organic extracts of tire tread were cytotoxic, genotoxic, and induced oxidative stress in human lung epithelial cells (A549). Furthermore, in macrophage and lung epithelial cell lines, tread particles (TPs) extracts also caused DNA damage and induced inflammatory reactions (Karlsson et al., Citation2011, Citation2006; Lindbom et al., Citation2006; Citation2007). In vivo assessments of potential for adverse effects associated with TP are contradicting, with some authors reporting cytotoxicity and inflammation (Mantecca et al., Citation2009; Citation2010) and others reporting small transient effects on inflammation in the absence of cytotoxicity (Gottipolu et al., Citation2008) following instillation of TP. However, none of these studies evaluate the potential for toxicity associated with TRWP, the particles truly generated during tire wear, which are known to be both chemically and morphologically distinct from TP (Kreider et al., Citation2010). Furthermore, the applicability of results from those studies using organic extracts to likely effects in humans from inhalation of TRWP is questionable given the harsh nature of the extraction relative to the conditions found in the human lung.
In addition to studies in TP, research on ingredients in the tread has also implicated TRWP as a potentially toxic component of ambient PM. Zinc, a key ingredient found in tires in the form of zinc oxide, has been implicated as potential contributor to the adverse effects associated with PM exposure (Kodavanti et al., Citation2005, 2003; Adamson et al., Citation2000; Soukup et al., Citation2000, Dye et al., Citation2001). Inhalation of zinc in different forms, including zinc oxide and zinc sulfate, has been shown to cause adverse effects on respiratory and cardiovascular systems, including impaired respiratory function, increased inflammation, cytotoxicity, oxidative stress, and effects on cardiac enzymes, pathology and blood coagulation (Lam et al., Citation1988; Gordon et al., Citation1992; Amdur et al., Citation1982; Kodavanti et al., Citation2008; Gottipolu et al., Citation2008; Wallenborn et al., Citation2009, Citation2008; Huang et al., Citation2010, Gilmour et al., Citation2006). Gottipolu et al. (Citation2008) compared the cardiopulmonary effects of zinc to TP, finding that while zinc sulfate increased markers of inflammation and injury in the lung and induced markers of mitochondrial oxidative stress in the heart, no cardiac effects occurred with exposure to TP and the pulmonary effects were only transient and relatively modest with TP exposure in comparison to the zinc-only exposure, indicating that while zinc may be a key constituent with respect to adverse cardiopulmonary outcomes associated ambient PM, these effects are not likely attributable to the presence of tire tread.
As the literature is somewhat conflicting, and to date no studies have evaluated the potential effects associated with TRWP specifically, the purpose of this study was to determine whether TRWP may cause adverse effects from inhalation. While the spectrum of effects associated with PM are still being investigated, several key adverse responses have been identified in association with particulate exposure, including pulmonary inflammation, damage to pulmonary cells and tissue, and effects on blood clotting, among others (Dagher et al., Citation2005; Kodavanti et al., Citation2005; Cassee et al., Citation2005; Elder et al., Citation2004; U.S. EPA, Citation2009; Pereira et al., Citation2007). Effects on the respiratory tract, particularly pulmonary inflammation, have been hypothesized to trigger the systemic effects through an inflammatory cascade, manifesting in cardiovascular and other adverse outcomes (Scapellato & Lotti, Citation2007). Furthermore, the generation of reactive oxygen species has been identified as a potentially key mediator of the inflammatory response associated with PM (Rhoden et al., Citation2004, Citation2008, Pereira et al., Citation2007, U.S. EPA, Citation2009). Therefore, these endpoints are the focus of this study in determining the potential for TRWP to cause adverse cardiopulmonary outcomes. Results from this study can be used to understand the dose–response relationship between exposure to TRWP and adverse effects in an effort to provide useful quantitative data for the purposes of risk assessment.
Methods
Materials
TRWP were collected at a road simulator laboratory located within the Bundesanstalt für Straßenwesen (BASt), the German Federal Highway Research Institute, as previously described by Kreider et al. (Citation2010). Briefly, the laboratory used an interior drum testing system containing actual asphalt pavement in cassettes. This system was electronically programmable to mimic a variety of driving conditions by varying speed, temperature, acceleration, braking, and steering. For the TRWP collection, the pavement consisted of a standardized asphalt concrete with 6.1% proportion of bitumen (B50/70) according to ISO 10844. To prevent overheating resulting from the use of an enclosure around the drum, the road surface temperature was maintained at ~20°C during the tests. The TRWP was collected using a vacuum system mounted behind one of the simulator wheels. Both summer and winter silica based tires (Michelin Pilot Primacy 225/55 R16 95W and Pirelli Sottozero 225/55 R16 95W M+S) and a carbon-black based summer tire (Bridgestone Potenza RE 88 205/65 R15 94W) were used to generate the TRWP. Particles from each tire were combined to form a single composite (2:1:1 Bridgestone:Michelin:Pirelli) that was sieved at 150 μm to remove any large pavement pieces. This composite was used for the animal exposure described below.
Animal care
All studies complied with the applicable sections of the Final Rules of the Animal Welfare Act regulations (9 CFR Parts 1, 2, and 3) and the Guide for the Care and Use of Laboratory Animals (National Research Council, Citation1996). The animal research facilities at Lovelace Respiratory Research Institute, where the study took place, are fully accredited by the Association for Assessment and Accreditation of Laboratory Animal Care (AAALAC). For both the instillation and inhalation studies, animals were received at the treatment facility and quarantined for a minimum of 14 days before study. Animals were provided access to food and water ab libitum for the duration of the study with the exception of when the animals are outside their cage for study procedures. For this inhalation study, Sprague-Dawley rats (Charles River Laboratories, Portage, MI, USA) were used.
Aerosol generation and monitoring
TRWP were aerosolized using a rotating brush generator (RBG) into a dedicated flow past nose-only exposure system (). Target air concentrations were 10, 40, and 100 µg/m3 TRWP; to achieve these concentrations, the outlet flow rate on the RBG was 20 L/min for the low and middle exposures and 9 L/min for the high concentration. Total air flow through the system was balanced to achieve individual rodent port flows of 1.5 times the respiratory minute volume of the rat. To monitor the exposure concentration, material was collected onto Pallflex membrane filters and the samples analyzed gravimetrically to determine total aerosol concentration for each day of exposure. In addition to air concentration, particle size including mass- and number-median aerodynamic diameter (MMAD and NMAD, respectively) was also monitored three times during the study using an aerodynamic particle sizer (APS; TSI 223100).
In addition to particle characteristics, oxygen content, temperature, system pressure, ambient pressure, and flow rates were monitored throughout the study.
Treatment and sacrifice
Rats were randomized into four exposure groups (air control, low, mid, high) using body weight (five males and five females per group with two spare animals of each sex to replace sick or injured animals). All animals were acclimated to the nose-only exposure tubes four times before exposure, beginning with a 30-min acclimation and increasing to 6.5 h (6.5 h is the estimated duration in which the animals will be restrained to for preparation of exposure and exposure); the last conditioning day occurred 5 days before the start of exposure. Animals were exposed to filtered air or TRWP using the nose-only inhalation exposure system described in for 6 h/day, 7 days/week, for 4 weeks. During the study, all animals were observed a minimum of twice daily at least 6 h apart; specific attention was paid to clinical signs related to the respiratory tract including apnea, labored breathing, malaise, nasal discharge, etc. Body weight and food consumption were recorded twice weekly during the study duration.
Animals were sacrificed (overdose of Euthasol) 1 day after completion of the exposure, at which time terminal blood was collected via cardiac puncture for standard hematology, clinical chemistry, and clot analyses. All body surfaces, orifices, cranial, thoracic, and abdominal cavities were examined for abnormalities and lesions. The whole lung was harvested and weighed. The left lung lobe was tied off and perfused with 10% neutral-buffered formalin (NBF), removed from the right lung lobes, and fixed in NBF. Post-fixation, tissue sections were cut, mounted on slides, and stained with hematoxylin and eosin for microscopic evaluation. The right lung lobes were lavaged twice with phosphate-buffered saline (PBS) (3 mL) for analyses, lavagates pooled together and analyzed for cell differentials, total protein, lactate dehydrogenase (LDH) activity, alkaline phosphatase (ALP), growth-related oncogene-keratinocyte chemoattractant (GRO-KC), tumor necrosis factor-α (TNF-α), and interleukin-6 (IL-6). Following lavage, the right lung lobes were flash-frozen in liquid nitrogen for oxidative stress analyses (hemeoxygenase-1 [HO-1] and thiobarbituric acid reactive substances [TBARS]). Urine was also collected at necropsy and analyzed for occult blood, pH, protein, urobilogen, ketones, bilirubin, and specific gravity when sufficient urine volume allowed.
Assays
Aliquots of the lavage fluid were analyzed for total protein (micro assay), LDH activity, and ALP activity using a Hitachi clinical chemistry analyzer and conventional clinical reagents. Additional aliquots of the lavage fluid were assayed for a panel of cytokines, including GRO-KC, TNF-α, and IL-6 via Luminex multiplex technology. The right cranial lobe of the lung was homogenized in 10 volumes (volume/mass) of a solution of 0.5% Triton X-100 in PBS with a cocktail of proteinase inhibitors (1 mM AEBSF, 0,15 mM E-64, 0.2 mM Peptstatin A, and 5 mM 1, 10 Phenanthroline) for analysis of HO-1. HO-1 was detected in lung tissue via an ELISA (Assay Designs, Ann Arbor, MI, USA) for rat HO-1. The right accessory lobe was homogenized for analysis of TBARS according to the methods described in Sciuto et al. (Citation1998). For hematology, whole blood was collected into K3EDTA vacutainers and evaluated using an automated hematology analyzer (ADVIA 120 Hematology System; Siemens, Tarrytown, NY, USA) for standard hematology parameters including: red blood cell count, hemoglobin, hematocrit, mean corpuscular volume, mean corpuscular hemoglobin concentration, mean corpuscular hemoglobin, platelet count, percent reticulocytes, white blood cell count, neutrophils, lymphocytes, monocytes, eosinophils, basophils, and large unstained cells). For clotting parameters, including partial thromboplastin time, and prothrombin time, whole blood was collected into tubes containing sodium citrate and subsequently centrifuged to separate the plasma for analysis. Automated analyses (Amax Destiny Plus; Trinity Biotech, Jamestown, NY, USA) were used to determine clotting parameters. Serum chemistry analysis was conducted on whole blood collected into serum tubes using an antomated chemistry analyzer (Hitachi Modular Analytics Clinical Chemistry System; Roche Diagnostics, Indianapolis, IN, USA); parameters measured included: alanine aminotransferase, albumin, aspartate aminotransferase, bilirubin, blood urea nitrogen, calcium, serum chloride, total cholesterol, serum creatinine, glucose, γ-glutamyltransferase, ALP, phosphate, serum potassium, total protein, serum sodium, and triglycerides.
Statistics
One-way analysis of variance was used to assess TRWP effects in the clinical chemistry and hematology parameters, oxidative stress parameters, and lung weights. Generalized estimating equations (GEE) were used to correct the correlation of repeated measurements for the same animal for body weight analyses. Where there was a significant treatment effect (p ≤ 0.05), Dunnett’s multiple comparisons was performed to assess differences between exposed and control groups (Dunnet, Citation1955, Citation1980).
Results
Particle characteristics
outlines the characteristics of the TRWP aerosol for each target air concentration with respect to measured air concentration and particle size. For all three exposure levels, the target air concentrations were met and maintained within ~15% of the target concentration. The average mass median aerodynamic diameter and percent of mass below 3 µm indicates that the particles were predominantly inhalable for the rats (Menache et al., Citation1995; Asgharian et al., Citation2003). and B show the MMAD and NMAD, respectively, for the highest exposure level.
Table 2. Measured air concentration and average particle size for TRWP by exposure level.
Figure 2. Particle size distributions of tire and road wear particles (TRWP) used for animal exposure. (A) Representative histogram of mass median aerodynamic diameter (MMAD) particle size distribution of the 100 µg/m3 exposure group; (B) representative histogram of number-median aerodynamic diameter (NMAD) particle size distribution of the 100 µg/m3 exposure group.
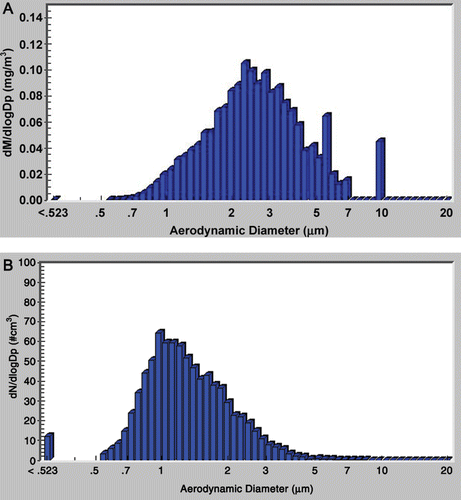
General toxicity
There were no signs of overt toxicity, such as dead or moribund animals, abnormal reactivity to stimuli, abnormal behavior, lesions, or labored or abnormal breathing, in any treatment group based on twice-daily cage-side observation of the animals. Body weight and body weight gain were unaffected by exposure to TRWP (). Similarly, there was no effect of treatment on food consumption, organ weight, or organ to body weight or brain weight ratios (data not shown). Exposure to TRWP was not associated with any changes in hematological (including clotting parameters) or clinical chemistry parameters; all statistical changes compared to control were considered spurious and associated values found to be within the typical range for the species and strain of animal used in the study.
Lavage analysis
Lavage fluid was analyzed for markers of cytotoxicity and inflammation in control and exposed animals. Cell differential profile was unchanged with exposure to TRWP (). Results for cytokine expression were consistent with the cell differential profile, indicating that TRWP did not increase expression of proinflammatory cytokines (). Markers for cytotoxicity, including LDH, ALP, and total protein, also remained unchanged with exposure to TRWP ().
Figure 4. Cell differential profile in lavage fluid with tire and road wear particles (TRWP) exposure. Data are presented as mean ± SE. n = 10 per treatment group (males and females combined).
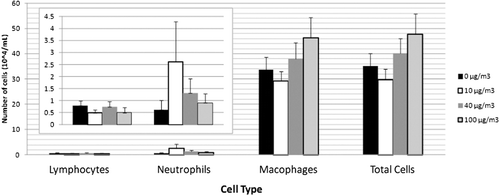
Figure 5. Inflammatory cytokine expression in lavage fluid with tire and road wear particles (TRWP) exposure. Data are presented as mean ± SE. n = 10 per treatment group (males and females combined). (A) interleukin-6 (IL-6); (B) growth-related oncogene-keratinocyte chemoattractant (GRO-KC); (C) tumor necrosis factor-α (TNF-α).
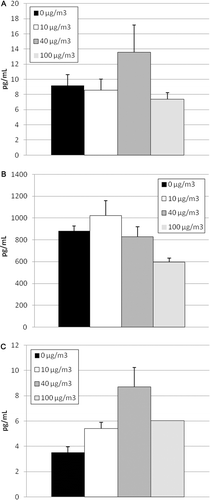
Figure 6. Markers of cytotoxicity in lavage fluid in response to tire and road wear particles (TRWP) exposure. Data are presented as mean ± SE. n = 10 per treatment group (males and females combined). (A) Total protein; (B) lactate dehydrogenase (LDH) activity; (C) alkaline phosphatase (ALP) activity.
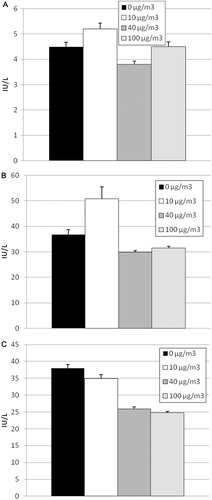
Reactive oxygen species
Lung tissue was analyzed for markers of oxidative stress. Neither HO-1 nor TBARS were elevated in lung tissue in response to TRWP exposure, indicating that TRWP has a low potential for initiating oxidative stress.
Histopathology
Lungs were normal by macroscopic examination of TRWP-exposed rats. Microscopic examination of the lung of rats exposed to 40 and 100 µg/m3 showed rare to few widely scattered minimal focal areas of subacute inflammatory cell infiltration of mononuclear cells in the alveolar wall and low numbers in alveolar spaces (). The alterations displayed a slight dose-response, observed in 3 of 10 rats exposed to 100 µg/m3 and one animal in the 40 µg/m3 group. No animals had focal lung alterations in the 0 or 10 µg/m3 exposure groups.
Figure 7. Histopathology of tire and road wear particles (TRWP) exposure. (A) Low power magnification of focal area of subacute inflammation in the lung. The perimeter is outlined by arrows to demonstrate the limited focal extent of minimal alteration. (B) Higher power magnification to illustrate infiltration of low numbers of mononuclear inflammatory cells interstitial in the thickened interalveolar wall and in alveolar spaces (asterisks) with few neutrophils (thin arrows). Hyperplasia of acinar lining epithelial cells indicated pneumocyte regeneration (thick arrows).
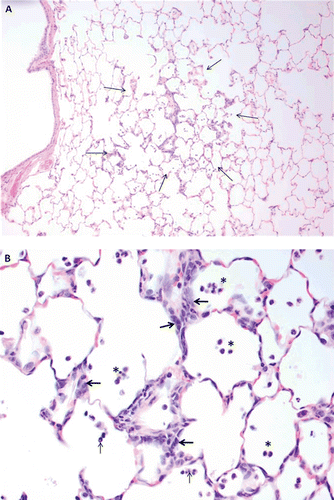
Discussion
Collectively, the results of this study indicate that TRWP is likely of low toxicity from inhalation; no adverse effects were noted on any biochemical or cell-based marker of inflammation, cytotoxicity, or oxidative stress. Furthermore, the effects noted in the lung pathology were both minor and infrequent, and therefore unlikely to lead to manifestation of impaired respiratory function or other adverse outcome in the respiratory tract from inhalation of TRWP. These results are consistent with results of an unpublished intratracheal instillation study that evaluated similar endpoints of inflammation, cytotoxicity, and oxidative stress in response to exposure to TRWP or TP. In this instillation study, neither TRWP nor TP elevated the same biochemical or cell-based markers studied in the inhalation study. In this instillation study, toxicity of TRWP and TP were also compared to particles with known proinflammatory effects, including diesel exhaust particles (NIST SRM 2975) and crystalline silica (MinUSil 5), and with particles of little inflammatory potential (titanium dioxide), in an effort to understand the relative toxicity of TRWP. is a representative example of how TRWP and TP compare to other particle types with respect to proinflammatory effects (PMN influx into lavage fluid). Similar results occurred when evaluating other endpoints of inflammation and markers of cytotoxicity and oxidative stress (data not shown). Inhalation studies are typically considered the “gold standard” for understanding the potential for toxicity from inhaled materials, but instillation studies can be useful predictors of relative toxicity (Driscoll et al., Citation2000). The results of the instillation study suggest that TRWP is a less potent inhalation toxicant than diesel exhaust (also found in ambient PM) or crystalline silica. The consistency of results across both studies provides strong support for the conclusion that TRWP is likely of low toxicity from inhalation.
Figure 8. Sample results from comparative toxicity study: Intratracheal instillation. Data are presented as mean ± SE. n = 8 per treatment group (males and females combined). *Statistically different from air control; #Statistically different from vehicle control; †Statistically different from tire and road wear particles (TRWP) (1 mg). ‡Statistically different from tread particles (TPs) (1 mg).
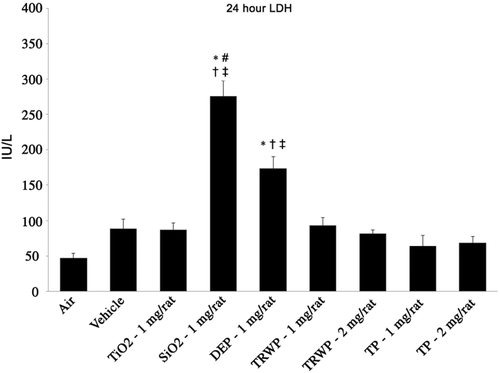
Both whole PM and components of PM have also initiated adverse events at low-dose exposures that are absent with exposure to TRWP. For instance, acute exposure (one-time 20-h exposure) to ambient air pollutants at concentrations ranging from ~20 to 200 µg/m3 PM from a heavily trafficked area in Brazil increased lipid peroxidation and inflammatory response in Wistar rats (Pereira et al., Citation2007). Hardwood smoke increased markers of cytotoxicity and inflammation at doses as low as 30 µg/m3 PM after 6 months of exposure (Seagrave et al., Citation2005). Furthermore, the U.S. EPA recently concluded that diesel exhaust particles causes adverse effects at doses as low as 100 µg/m3 (U.S. EPA, Citation2009). Exposure to diesel exhaust at 100 µg/m3 PM for 1 month increased the percentage of lymphocytes and neutrophils in the BALF and increased cytokine expression and release of cytokines from alveolar macrophages in mice (Hiramatsu et al., Citation2003; Saito et al., Citation2002). Diesel exhaust has also induced changes in clinical chemistry parameters in rats following as little as 1 week of exposure to 100 µg/m3 PM (Reed et al., Citation2004). Furthermore, at moderately higher exposure levels (~230 µg/m3) under shorter exposure durations than are used in this study, diesel exhaust has also been shown to increase markers of oxidative stress (including HO-1) (McDonald et al., Citation2004; Banerjee et al., Citation2009); inflammation (including IL-6, TNF-α and inflammatory cells) (McDonald et al., Citation2004, Banerjee et al., Citation2009); and cytotoxicity (including LDH) (Banerjee et al., Citation2009) in the lavage fluid or lung tissue. Lastly, elemental carbon, which is similar to the particulate core of diesel exhaust, has also affected cardiovascular performance in spontaneously hypertensive rats following a single exposure to 172 µg/m3, though no affects on inflammatory markers were apparent with this exposure (Upadhyay et al., Citation2008).
The potential correlation of zinc in ambient air with incidence of adverse events in the human population originally sparked the interest in TRWP as a potential contributor to the cardiopulmonary effects associated with ambient PM exposure (Adamson et al., Citation2000; Dye et al., Citation2001; Kodavanti et al., Citation2003, 2005; Soukup et al., Citation2000). The results of this study indicate that TRWP is not likely to contribute to the effects attributed zinc in the ambient environment. Zinc contributes ~1% by mass to tire tread and ~0.3% by mass to TRWP (Kreider et al., Citation2010). Tires are a considered to be a primary contributor to zinc in environment (Kreider et al., Citation2010; Lough et al., Citation2005; Councell et al., Citation2004; Adachi & Tanionosho, Citation2005). However, other sources are also known to contribute zinc to ambient PM, including combustion products, asphalt, brake wear, and industrial sources (Dye et al., Citation2001; Sjodin et al., Citation2010; Kodavanti et al., Citation2002). Gottipolu et al. Citation2008 demonstrated that bioavailability via water solubility of the zinc is an important determinant of the potency of toxic response from zinc. For TPs that had low solubility of zinc, the magnitude of response at equivalent dose levels was lower than for high solubility forms of zinc (including zinc sulfate). The percent of zinc in TRWP expected to be soluble in lung fluid is ~50% (unpublished data). Therefore, although TRWP may be a major contributor to zinc in the environment, only a fraction of that zinc is bioavailable via inhalation. Together with our results, this may provide an explanation as to why TRWP, despite being high in zinc content, may not contribute to the adverse effects from zinc-containing PM.
In addition to a basic understanding about the potential for toxicity via inhalation of TRWP, this study also provides information about the dose–response relationship between exposure to TRWP and effect. The advantage of having conducted an inhalation study (vs. previous instillation studies) is the ability to determine an effect threshold (e.g. a no or low observed adverse effect level) for the purpose of use in a future risk assessment for TRWP. Aside from the few foci of minimal inflammatory cell infiltrations scattered in the lung demonstrated by the histopathology examination, TRWP caused no effects on any other endpoint evaluated in this study, including general toxicity, cytotoxicity and inflammation in the respiratory tract, and cardiovascular endpoints, particularly hematology and clotting ability. When establishing a threshold for toxicological effects, one consideration is whether the observed effects are considered “adverse.” The U.S. EPA guidance for determining whether an effect is considered “adverse,” requires the determination of biological significance such that the observed effect (a biochemical change, a functional impairment, or a pathological lesion) is likely to impair the performance or reduce the ability of an individual to function or to respond to additional challenge from the agent (U.S. EPA, Citation2002). Biological significance is also attributed to effects that are consistent with steps in a known mode of action. Statistical significance quantifies the likelihood that the observed effect is not due to chance alone. Thus U.S. EPA guidance states that more weight is given to biological significance, and a statistically significant change that lacks biological significance is not considered an adverse response. Though no statistical evaluation of the histopathology results were performed, the effects were presumed to be related to exposure to TRWP, because of the dose–response relationship. Because the mild and infrequent inflammation observed in this study is unlikely to impair the performance of the animal, the highest dose used in this study (112 µg/m3) can be considered the no-observable-adverse-effect-level (NOAEL).
Previous studies on TPs have not permitted a clear understanding of the relative potency of TRWP compared to other known constituents of ambient air, thus it is difficult to contextualize their results. In their original study, Mantecca et al. (Citation2009) found that TPs in both the coarse and fine fractions produced macrophage-mediated inflammatory response and cytotoxicity, although a positive control was not used and therefore the magnitude of response from the tread could not be compared. In a subsequent study, Mantecca et al. (Citation2010) compared the effects of TPs to ambient PM collected in Milan. They found that, in terms of inflammatory potential, TPs were less potent than PM. These results indicate the importance of providing appropriate comparisons, particularly when the applied dose cannot be easily compared to an ambient air concentration. All other studies on TP toxicity in the respiratory system have used either intratracheal instillation techniques (with the absence of appropriate context/comparative toxicities presented) or in vitro methods (Beretta et al., Citation2007; Gottipolu et al., Citation2008; Gualtieri et al., Citation2008, Citation2005; Karlsson et al., Citation2006; Mantecca et al., Citation2009). Furthermore, because these studies only evaluated the rubber component of TRWP, the potential impact from actual tire wear particles had not yet been evaluated. Therefore, of the available literature, the results from this study provide the best available data for understanding the relationship between exposure concentration and potential for adverse effect from exposure to TRWP.
While this study is the most robust study available when evaluating the potential for TRWP-induced toxicity from inhalation, it is not without limitations. Based on material limitations, the exposure regimen used in this study was not sufficient to identify an adverse effect level of TRWP. As indicated before with other particle types, low-dose exposure regimens do not always produce consistent results with respect to potential for cardiopulmonary effects. However, because the maximum concentration used in this study is well above those detected in the environment, there is a large margin of exposure between the NOAEL and the environmental levels of TRWP, indicating that even in the presence of some uncertainty, the likelihood of TRWP causing adverse effects from inhalation in the ambient environment is low (Panko et al., Citation2012).
Conclusions
The data presented in this study supports the conclusion that TRWP is of low toxicity from inhalation. From these results, a NOAEL of 112 µg/m3 was identified for future use in a risk assessment. Based on data from a recent global air sampling campaign using a marker for rubber polymer, the air concentration of TRWP in the ambient atmosphere is low; tread concentrations in the air were <0.1 µg/m3 and contributed <1% by mass to the total PM10 in the ambient air (Panko et al., Citation2012). Therefore, the margin of exposure between the NOAEL identified from this study and environmental levels of TRWP is large, indicating a low likelihood of human health risk from inhalation of TRWP.
Supplementary Material
Download PDF (510.8 KB)Acknowledgements
The authors would like to acknowledge JeanClare Seagrave at Lovelace Respiratory Research Institute and Klaus Peter Glaeser at the BASt for their contributions to the work presented here.
Declaration of interest
This work was funded by the Tire Industry Project, an industry-wide initiative organized under the World Business Council for Sustainable Development that aims to understand the human health and environmental risks associated with the tire throughout its lifecycle.
References
- Adachi K, Tanionosho Y. 2005. Single particle characterization of size-fractionated road sediments. Appl Geochemistry 20: 849–859.
- Adamson IY, Prieditis H, Hedgecock C, Vincent R. 2000. Zinc is the toxic factor in the lung response to an atmospheric particulate sample. Toxicol Appl Pharmacol 166: 111–119.
- Almeida-Silva M, Canha N, Freitas MC, Dung HM, Dionisio I. 2011. Air pollution at an urban traffic tunnel in Lisbon, Portugal: an INAA study. Appl Radiat Isot 69: 1586–1591.
- Amdur MO, McCarthy JF, Gill MW. 1982. Respiratory response of guinea pigs to zinc oxide fume. Am Ind Hyg Assoc J 43: 887–889.
- Asgharian B, Kelly JT, Tewksbury EW. 2003. Respiratory deposition and inhalability of monodisperse aerosols in Long-Evans rats. Toxicol Sci 71: 104–111.
- Banerjee A, Trueblood MB, Zhang X, Manda KR, Lobo P, Whitefield PD, Hagen DE, Ercal N. 2009. N-acetylcysteineamide (NACA) prevents inflammation and oxidative stress in animals exposed to diesel engine exhaust. Toxicol Lett 187: 187–193.
- Beretta E, Gualtieri M, Botto L, Palestini P, Miserocchi G, Camatini M. 2007. Organic extract of tire debris causes localized damage in the plasma membrane of human lung epithelial cells. Toxicol Lett 173: 191–200.
- Cassee FR, Boere AJ, Fokkens PH, Leseman DL, Sioutas C, Kooter IM, Dormans JA. 2005. Inhalation of concentrated particulate matter produces pulmonary inflammation and systemic biological effects in compromised rats. J Toxicol Environ Health A 68: 773–796.
- Chen Y, Yang Q, Krewski D, Shi Y, Burnett RT, Mcgrail K. 2004. Influence of relative low level of particulate air pollution on hospitalization for COPD in elderly people. Inhal Toxicol 16: 21–25.
- Councell TB, Duckenfield KU, Landa ER, Callender, E. 2004. Tire-wear particles as a source of zinc to the environment. Env Sci Technol 38: 4206–4214.
- Dagher Z, Garcon G, Gosset P, Ledoux F, Surpateanu G, Courcot D, Aboukais A, Puskaric E, Shirali P. 2005. Pro-inflammatory effects of Dunkerque city air pollution particulate matter 2.5 in human epithelial lung cells (L132) in culture. J Appl Toxicol 25: 166–175.
- Dockery DW, Pope CA, Xu, X, Spengler JD, Ware JH, Fay ME, Ferris BG, Speizer FE. 1993. An Association between Air Pollution and Mortality in Six US Cities. N Engl J Med 329: 1753–1759.
- Dockery DW, Schwartz J, Spengler JD. 1992. Air pollution and daily mortality: associations with particulates and acid aerosols. Environ Res 59: 362–373.
- Driscoll KE, Costa DL, Hatch G, Henderson R, Oberdorster G, Salem H, Schlesinger RB. 2000. Intratracheal instillation as an exposure technique for the evaluation of respiratory tract toxicity: uses and limitations. Tox Sci 55: 24–35.
- Dunnet CW. 1955. A multiple comparison procedure for comparing several treatments with a control. J Am Stat Assoc 50: 1096–1121.
- Dunnet CW. 1980. Pairwise multiple comparison in the unequal variance case. J Am Stat Assoc 75: 796–800.
- Dye JA, Lehmann JR, McGee JK, Winsett DW, Ledbetter AD, Everitt JI, Ghio AJ, Costa DL. 2001. Acute pulmonary toxicity of particulate matter filter extracts in rats: coherence with epidemiologic studies in Utah Valley residents. Environ Health Perspect 109 Suppl 3: 395–403.
- Elder A, Gelein R, Finkelstein J, Phipps R, Frampton M, Utell M, Kittelson DB, Watts WF, Hopke P, Jeong CH, Kim E, Liu W, Zhao W, Zhuo L, Vincent R, Kumarathasan P, Oberdorster G. 2004. On-road exposure to highway aerosols. 2. Exposures of aged, compromised rats. Inhal Toxicol 16 Suppl 1: 41–53.
- Gilmour PS, Nyska A, Schladweiler MC, McGee JK, Wallenborn JG, Richards JH, Kodavanti UP. 2006. Cardiovascular and blood coagulative effects of pulmonary zinc exposure. Toxicol Appl Pharmacol 211: 41–52.
- Gordon T, Chen LC, Fine JM, Schlesinger RB, Su WY, Kimmel TA, Amdur MO. 1992. Pulmonary effects of inhaled zinc oxide in human subjects, guinea pigs, rats, and rabbits. Am Ind Hyg Assoc J 53: 503–509.
- Gottipolu RR, Landa ER, Schladweiler MC, Mcgee JK, Ledbetter AD, Richards JH, Wallenborn GJ, Kodavanti UP. 2008. Cardiopulmonary responses of intratracheally instilled tire particles and constituent metal components. Inhal Toxicol 20: 473–484.
- Gualtieri M, Mantecca P, Cetta F, Camatini M. 2008. Organic compounds in tire particle induce reactive oxygen species and heat-shock proteins in the human alveolar cell line A549. Environ Int 34: 437–442.
- Gualtieri M, Rigamonti L, Galeotti V, Camatini M. 2005. Toxicity of tire debris extracts on human lung cell line A549. Toxicol In Vitro 19: 1001–1008.
- Health Effects Institute. 2010. Traffic-related air pollution: A critical review of the literature on emissions, exposure, and health effects. Boston, MA: HEI Panel on the Health Effects of Traffic-Related Air Pollution.
- Hiramatsu K, Azuma A, Kudoh S, Desaki M, Takizawa H, Sugawara I. 2003. Inhalation of diesel exhaust for three months affects major cytokine expression and induces bronchus-associated lymphoid tissue formation in murine lungs. Exp Lung Res 29: 607–622.
- Huang CC, Aronstam RS, Chen DR, Huang YW. 2010. Oxidative stress, calcium homeostasis, and altered gene expression in human lung epithelial cells exposed to ZnO nanoparticles. Toxicol In Vitro 24: 45–55.
- Karlsson H, Lindbom J, Ghafouri B, Lindahl M, Tagesson C, Gustafsson M, Ljungman AG. 2011. Wear particles from studded tires and granite pavement induce pro-inflammatory alterations in human monocyte-derived macrophages: a proteomic study. Chem Res Toxicol 24: 45–53.
- Karlsson HL, Ljungman AG, Lindbom J, Moller L. 2006. Comparison of genotoxic and inflammatory effects of particles generated by wood combustion, a road simulator and collected from street and subway. Toxicol Lett 165: 203–211.
- Keith W, Morgan C, Lapp NL. 1977. Diseases of the airways and lungs. In: Key MM, Henschel AF, Butler J, Ligo RN, Tabershaw IR, Ede L. (eds.) Occupational Diseases: A Guide to Their Recognition. Washington, DC: U.S. Department of Health, Education and Welfare.
- Keuken M, Denier Van Der Gon H, Van Der Valk K. 2010. Non-exhaust emissions of PM and the efficiency of emission reduction by road sweeping and washing in the Netherlands. Sci Total Environ 408: 4591–4599.
- Kodavanti UP, Moyer CF, Ledbetter AD, Schladweiler MC, Costa DL, Hauser R, Christiani DC, Nyska A. 2003. Inhaled environmental combustion particles cause myocardial injury in the Wistar Kyoto rat. Toxicol Sci 71: 237–245.
- Kodavanti UP, Schladweiler MC, Gilmour PS, Wallenborn JG, Mandavilli BS, Ledbetter AD, Christiani DC, Runge MS, Karoly ED, Costa DL, Peddada S, Jaskot R, Richards JH, Thomas R, Madamanchi NR, Nyska A. 2008. The role of particulate matter-associated zinc in cardiac injury in rats. Environ Health Perspect 116: 13–20.
- Kodavanti UP, Schladweiler MC, Ledbetter AD, Hauser R, Christiani DC, Samet JM, McGee J, Richards JH, Costa DL. 2002. Pulmonary and systemic effects of zinc-containing emission particles in three rat strains: multiple exposure scenarios. Toxicol Sci 70: 73–85.
- Kodavanti UP, Schladweiler MC, Ledbetter AD, Mcgee JK, Walsh L, Gilmour PS, Highfill JW, Davies D, Pinkerton KE, Richards JH, Crissman K, Andrews D, Costa DL. 2005. Consistent pulmonary and systemic responses from inhalation of fine concentrated ambient particles: roles of rat strains used and physicochemical properties. Environ Health Perspect 113: 1561–1568.
- Kreider ML, Panko JM, Mcatee BL, Sweet LI, Finley BL. 2010. Physical and chemical characterization of tire-related particles: Comparison of particles generated using different methodologies. Sci Total Environ 408: 652–659.
- Lam HF, Chen LC, Ainsworth D, Peoples S, Amdur MO. 1988. Pulmonary function of guinea pigs exposed to freshly generated ultrafine zinc oxide with and without spike concentration. Am Ind Hyg Assoc J, 49: 333–341.
- Lin M, Stieb DM, Chen Y. 2005. Coarse particulate matter and hospitalization for respiratory infections in children younger than 15 years in Toronto: a case-crossover analysis. Pediatrics 116, e235–e40.
- Lindbom J, Gustafsson M, Blomqvist G, Dahl A, Gudmundsson A, Swietlicki E, Ljungman AG. 2006. Exposure to wear particles generated from studded tires and pavement induces inflammatory cytokine release from human macrophages. Chem Res Toxicol 19: 521–530.
- Lindbom J, Gustafsson M, Blomqvist G, Dahl A, Gudmundsson A, Swietlicki E, Ljungman AG. 2007. Wear particles generated from studded tires and pavement induces inflammatory reactions in mouse macrophage cells. Chem Res Toxicol 20: 937–946.
- Lough GC, Schauer JJ, Park JS, Shafer MM, Deminter JT, Weinstein JP. 2005. Emissions of metals associated with motor vehicle roadways. Environ Sci Technol 39: 826–836.
- Mantecca P, Farina F, Moschini E, Gallinotti D, Gualtieri M, Rohr A, Sancini G, Palestini P, Camatini M. 2010. Comparative acute lung inflammation induced by atmospheric PM and size-fractionated tire particles. Toxicol Lett 198: 244–254.
- Mantecca P, Sancini G, Moschini E, Farina F, Gualtieri M, Rohr A, Miserocchi G, Palestini P, Camatini M. 2009. Lung toxicity induced by intratracheal instillation of size-fractionated tire particles. Toxicol Lett 189: 206–214.
- McDonald JD, Harrod KS, Seagrave J, Seilkop SK, Mauderly JL. 2004. Effects of low sulfur fuel and a catalyzed particle trap on the composition and toxicity of diesel emissions. Environ Health Perspect 112: 1307–1312.
- Menache MG, Miller FJ, Raabe OG. 1995. Particle inhalability curves for humans and small laboratory animals. Ann Occup Hyg 39: 317–328.
- National Research Council. 1996. Guide for the Care and Use of Laboratory Animals. Washington, DC: National Academy Press.
- Panko JM, Kreider ML, Chu JJ, Unice KM. 2012. Global assessment of tire and road wear particles in the ambient air. Atmospheric Environ, submitted.
- Pereira CE, Heck TG, Saldiva PH, Rhoden CR. 2007. Ambient particulate air pollution from vehicles promotes lipid peroxidation and inflammatory responses in rat lung. Braz J Med Biol Res 40: 1353–1359.
- Peters A, Dockery DW, Muller JE, Mittleman MA. 2001. Increased particulate air pollution and the triggering of myocardial infarction. Circulation 103: 2810–2815.
- Pope CA 3rd, Muhlestein JB, May HT, Renlund DG, Anderson JL, Horne BD. 2006. Ischemic heart disease events triggered by short-term exposure to fine particulate air pollution. Circulation 114: 2443–2448.
- Pope CA, 3rd, Renlund DG, Kfoury AG, May HT, Horne BD. 2008. Relation of heart failure hospitalization to exposure to fine particulate air pollution. Am J Cardiol 102: 1230–1234.
- Reed MD, Gigliotti AP, McDonald JD, Seagrave JC, Seilkop SK, Mauderly JL. 2004. Health effects of subchronic exposure to environmental levels of diesel exhaust. Inhal Toxicol 16: 177–193.
- Rhoden CR, Ghelfi E, Gonzalez-Flecha B. 2008. Pulmonary inflammation by ambient air particles is mediated by superoxide anion. Inhal Toxicol 20: 11–15.
- Rhoden CR, Lawrence J, Godleski JJ, Gonzalez-Flecha B. 2004. N-acetylcysteine prevents lung inflammation after short-term inhalation exposure to concentrated ambient particles. Toxicol Sci 79: 296–303.
- Rosner D, Markowitz G. 1991. Deadly dust: silicosis and the politics of occupational disease in twentieth-century America. Princeton, New Jersey: Princeton University Press.
- Saito Y, Azuma A, Kudo S, Takizawa H, Sugawara I. 2002. Long-term inhalation of diesel exhaust affects cytokine expression in murine lung tissues: comparison between low- and high-dose diesel exhaust exposure. Exp Lung Res 28: 493–506.
- Salma I, Maenhaut W. 2006. Changes in elemental composition and mass of atmospheric aerosol pollution between 1996 and 2002 in a Central European city. Environ Pollut 143: 479–488.
- Scapellato ML, Lotti M. 2007. Short-term effects of particulate matter: an inflammatory mechanism? Crit Rev Toxicol 37: 461–487.
- Sciuto AM. 1998. Assessment of early acute lung injury in rodents exposed to phosgene. Arch Toxicol 72: 283–288.
- Seagrave J, Mcdonald JD, Reed MD, Seilkop SK, Mauderly JL. 2005. Responses to subchronic inhalation of low concentrations of diesel exhaust and hardwood smoke measured in rat bronchoalveolar lavage fluid. Inhal Toxicol 17: 657–670.
- Sjodin A, Ferm M, Bjork A, Rahmberg M, Gudmundsson A, Swietlicki E, Johansson C, Gustafsson M, Blomqvist G. 2010. Wear particles from road traffic- a field, laboratory, and modelling study. Goteborg, Sweden: IVL Swedish Environmental Research Institute Ltd.
- Soukup JM, Ghio AJ, Becker S. 2000. Soluble components of Utah Valley particulate pollution alter alveolar macrophage function in vivo and in vitro. Inhal Toxicol 12: 401–414.
- U.S. EPA. 2002. A review of the reference dose and reference concentration processes. Washington, DC: U.S. Environmental Protection Agency.
- U.S. EPA. 2009. Integrated science assessment for particulate matter. Research Triangle Park, NC: National Center for Environmental Assessment; Office of Research and Development.
- Upadhyay S, Stoeger T, Harder V, Thomas RF, Schladweiler MC, Semmler-Behnke M, Takenaka S, Karg E, Reitmeir P, Bader M, Stampfl A, Kodavanti UP, Schulz H. 2008. Exposure to ultrafine carbon particles at levels below detectable pulmonary inflammation affects cardiovascular performance in spontaneously hypertensive rats. Part Fibre Toxicol 5: 19.
- Wallenborn JG, Evansky P, Shannahan JH, Vallanat B, Ledbetter AD, Schladweiler MC, Richards JH, Gottipolu RR, Nyska A, Kodavanti UP. 2008. Subchronic inhalation of zinc sulfate induces cardiac changes in healthy rats. Toxicol Appl Pharmacol 232: 69–77.
- Wallenborn JG, Schladweiler MJ, Richards JH, Kodavanti UP. 2009. Differential pulmonary and cardiac effects of pulmonary exposure to a panel of particulate matter-associated metals. Toxicol Appl Pharmacol 241: 71–80.